Abstract
Background: MRI-guided transurethral ultrasound ablation (TULSA) has been evaluated for organ-confined prostate cancer (PCa). The purpose of this study was to assess the safety and toxicity, accuracy and short-term evolution of cell-death after lesion-targeted TULSA.
Methods: This prospective, registered, Phase-I treat-and-3-week-resect-study enrolled six patients with MRI-visible-biopsy-concordant PCa. Lesions were targeted using TULSA with radical intent, except near neurovascular bundles (NVB). Robot-assisted-laparoscopic-prostatectomy (RALP) was performed at 3 weeks. Post-TULSA assessments included MRI (1 and 3 weeks), adverse events and quality-of-life (QoL) to 3 weeks, followed by RALP and whole-mount-histology. Treatment accuracy and demarcation of thermal injury were assessed using MRI and histology.
Results: Six patients (median age = 70 years, prostate volume = 60 ml, PSA = 8.9 ng/ml) with eight biopsy-confirmed MRI-lesions (PIRADS ≥3) were TULSA-treated without complications (median sonication and MRI-times of 17 and 117 min). Foley-catheter removal was uneventful at 2–3 days. Compared to baseline, no differences in QoL were noted at 3 weeks. During follow-up, MRI-derived non-perfused-volume covered ablated targets and increased 36% by 3 weeks, correlating with necrosis-area on histology. Mean histological demarcation between complete necrosis and outer-limit-of-thermal-injury was 1.7 ± 0.4 mm. Coagulation necrosis extended to capsule except near NVB, where 3 mm safety-margins were applied. RALPs were uncomplicated and histopathology showed no viable cancer within the ablated tumor-containing target.
Conclusions: Lesion-targeted TULSA demonstrates accurate and safe ablation of PCa. A significant increase of post-TULSA non-perfused-volume was observed during 3 weeks follow-up concordant with necrosis on histology. TULSA achieved coagulation necrosis of all targeted tissues. A limitation of this treat-and-resect-study-design was conservative treatment near NVB in patients scheduled for RALP.
Introduction
Standard therapies for intermediate- and high-risk localized prostate cancer (PCa), radical prostatectomy and radiotherapy offer proven cancer control but carry the risk of adverse effects to genitourinary and bowel function [Citation1–4]. There is an unmet need for effective cancer treatments that have minimal impact on quality-of-life (QoL) [Citation5].
MRI provides visualization of PCa, enabling a platform for image-guided and lesion-targeted therapies [Citation6,Citation7]. MRI-guided transurethral ultrasound ablation (TULSA) treats prostate tissue with high intensity directional (but not focused) ultrasound by rapidly raising and maintaining elevated tissue temperatures above 55 °C within the targeted region, utilizing real-time magnetic resonance (MR) thermometry to monitor and control ablation [Citation8,Citation9]. As opposed to a series of brief small volume exposures in HIFU, directional ultrasound has distinct patterns of thermal dose and temperature deposition and subsequent tissue damage due to the use of continuous heating with an unfocused ultrasound beam. The ablated volume is visualized post-treatment on contrast enhanced MRI (CE-MRI) as a non-perfused-volume (NPV) indicating complete cell death [Citation10–12]. Target tissue undergoes acute coagulation necrosis [Citation13], followed by delayed necrosis, which is mainly dependent on cumulative thermal dose [Citation14]. Correlation between a thermal dose of 240 cumulative equivalent minutes at 43 °C (CEM) measured on MR thermometry, with delayed migration of the outer limit of thermal injury up to 3 mm beyond the region of acute coagulation necrosis within 48 hours after ablation, has been demonstrated in pre-clinical studies [Citation15–17]. In human prostates, the demarcation of thermal injury (DTI) between complete necrosis and the outer limit of thermal injury at delayed time points after TULSA treatment has not yet been reported.
The feasibility of ablating prostate tissue using TULSA has been demonstrated using prototype systems in two treat-and-immediate-resect-studies and using the now CE-marked commercial TULSA system in a Phase I study with 12-month follow-up after conservative whole-gland-ablation to a 3 mm safety margin in mainly low-risk PCa [Citation18–20]. Only one treat-and-immediate-resect study with a TULSA prototype has demonstrated the capability of ablation reaching the prostate capsule [Citation19]. However, the majority of PCa locations are subcapsular, some infiltrating the capsule. Therefore, it is paramount to determine if TULSA is not only accurate with sharp demarcation of ablation boundaries avoiding damage to surrounding tissues, but if it can ablate into the capsule, and on demand beyond, to ensure cancer control. Data on the feasibility of lesion-targeted TULSA is scarce, and no short-term radiology-histology analyses exploring delayed necrosis and demarcation of thermal injury near the prostate capsule have been reported.
The primary objectives of the current study were to assess safety and toxicity, histological and radiological treatment accuracy and DTI after lesion-targeted TULSA. A treat-and-resect study design with a 3-week interval between TULSA and robot-assisted laparoscopic prostatectomy (RALP) enabled assessment of early adverse events and QoL, treatment accuracy with histology and NPV as reference, the evolution of NPV and its correlation to histology and DTI. Pre-planned post-TULSA RALP enabled inclusion of higher-risk tumors and thorough evaluation of ablation extent at the prostate capsule on whole-mount histology.
Materials and methods
Study design
This was a prospective, registered (NCT03350529), single-center Phase I study with treatment followed by resection at 3 weeks. The study protocol was approved by the local Ethics committee of the Hospital District of Southwest Finland and written informed consent was obtained from all participants.
Patient eligibility, selection and consenting
Men with newly-diagnosed MRI-visible lesions concordant with biopsy-confirmed localized histologically significant PCa who planned to undergo RALP were eligible for the study. Patients were included only if they had undergone MRI-targeted biopsies (MRI-TBx) from MRI-visible lesions with PI-RADS version 2 score ≥3 [Citation21] in addition to systematic transrectal 10–12-core ultrasound-guided biopsies (TRUSgb). Spatial concordance was achieved by cognitive registration of lesions on MRI with TRUSgb.
Histologically significant PCa lesions were defined based on MRI-TBx as follows: Gleason score (GS) ≥7 or high-volume GS6 disease (cancer core length >6 mm, or cancer involvement >50% in any core, or number of positive MRI-TBx cores >2). For detailed description of eligibility criteria, see Supplementary Appendix 1.
After consenting, baseline investigations were performed (Supplementary Appendix 1). If still eligible, the participant was referred to TULSA.
Description of the study intervention
The study intervention was delivered using a previously described TULSA system (TULSA-PRO, Profound Medical Inc., Toronto, Canada) integrated into a 3 T MRI (Ingenia 3.0 T, Philips Healthcare, Best, Netherlands) [Citation18].
The treatment plan targeted ablation of all MRI-visible biopsy-concordant PCa lesions. The ablative effect was planned to cover the lesion(s) with a 5 mm overlap up to the prostate capsule when feasible, to account for MRI underestimation of tumor size [Citation22]. For this treat-and-resect-study where patients do not receive benefits from TULSA treatment, it was paramount to minimize the risk of any negative functional impact on genitourinary function related to the study intervention prior to nerve-sparing RALP. Therefore, in the vicinity of the neurovascular bundles (NVB), safety margins up to 3 mm were applied regardless of tumor extent, based on the concern that necrosis may migrate beyond the region of acute coagulation necrosis [Citation16] (Supplementary Figure S2a). Details of TULSA method and lesion-targeted TULSA treatment delivery are described in Supplementary Appendix 2 and Supplementary Figure S1.
Endpoints and post-TULSA follow-up
Primary endpoints were safety and toxicity, accuracy and histological DTI of lesion-targeted TULSA. The secondary endpoint was to assess the evolution of NPV during 3 weeks follow-up. Exploratory endpoints included assessment of ablation efficacy using radiology and histology.
Follow-up visits were scheduled as follows: 2–3 days, removal of catheter; 1 and 3 weeks, PSA and multiparametric MRI. Adverse events and pain were reviewed at every visit, QoL was measured at baseline and 3 weeks post-TULSA using IPSS, IIEF-5, UCLA-PCI-UFI, ICIQ-SF and EPIC26-BD questionnaires.
POST-TULSA RALP and histopathology
RALPs were performed by a urologist with 10-year experience of this procedure. During surgery, additional attention was paid to tissue effects caused by prior TULSA. RALP specimens were formalin fixed and cut into 5 mm sections. Whole-mount 5 μm sections were examined by a uropathologist with 10 years of experience (Supplementary Figure S2b).
Two thermal damage boundaries were contoured: (1) the outer boundary of complete necrosis and (2) the outer limit of thermal injury, outside which there was no visual evidence of thermal damage [Citation16]. Hematoxylin-eosin-stained histological slides were digitized for volumetric calculation and subsequent comparison to MRI-data (Supplementary Figure S2b).
Measurements of targeting and treatment accuracy
Measurements of thermal targeting accuracy derived from MRI temperature maps and planning images obtained during TULSA treatment included volumetric thermal target coverage and overlap, as well as linear targeting accuracy and precision. Isotherm calculations and comparisons were executed in Matlab (R2018a, Mathworks Inc., Natick, MA). Detailed description of targeting measurements are shown in Supplementary Table S4.
MRI-based prostate volume, lesion volumes, NPV and histology-based complete necrosis volume were measured using AW Server (GE Healthcare, Chicago, IL) by calculating the contoured area multiplied by the plane thickness (Supplementary Figure S3).
Histological and radiological treatment accuracies were evaluated by comparing the size and shape of MR-thermometry volumes as well as the immediate, 1- and 3-week NPV, with both the complete necrosis volume on histology and the target volume on the treatment plan [Citation23,Citation24]. The mean distance between the complete necrosis boundary and the outer limit of thermal injury was measured to determine DTI.
Determination of treatment efficacy
Within targeted regions, the 3-week radiological and histological treatment efficacies were determined using 3-level structured destruction score classifications [Citation12]. For MRI destruction scores, targeted regions completely included within the NPV without focal enhancement were classified as successful treatment (score 0), targeted regions not totally included within the NPV as targeting failure (score 1) and targeted regions within the NPV but demonstrating residual focal enhancement as ablative failure (score 2). For histological destruction scores, targeted regions completely included in the necrosis volume with no vital cancer observed inside the necrosis volume were classified as successful treatment (score 0), targeted regions not completely included in the necrosis volume with no foci of vital cancer inside the necrosis volume were classified as targeting failure (score 1) and targeted regions totally included in the necrosis volume but with foci of residual vital cancer inside the ablated volume were classified as ablative failure (score 2). MRI destruction scores were not applied to regions of viable cancer that were not part of the treatment plan due to either MRI-invisibility or to 3 mm safety margins near the NVB required for this treat-and-resect study.
Results
Patients and targeted lesions
Between August 2017 and May 2018, six men were enrolled and all completed the study. The characteristics of the study population are presented in . All six study patients were Caucasians and had normal performance status (ECOG = 0). The median baseline PSA was 8.9 ng/ml.
Table 1. Baseline patient, disease and tumor characteristics on MRI.
Table 2. TULSA for targeted ablation of PCa-lesions: Multimodality based volumetric correlations.
Eight lesions in the six study patients were ablated. MRI-TBx from five patients presented histology of Gleason pattern 4, and from one patient Gleason pattern 3 with high-volume disease based on MRI and histology ( and ). Patient 6 presented with bilobar GS 3 + 4 = 7 lesions, and patient 5 had bifocal left lobe GS 4 + 4 = 8 disease. Both of their lesions were covered with a single treatment sector.
Figure 1. Location of the targeted lesions on baseline MRI. Note the red arrow pointing to the lesion on each image sequence. The first four patients had only one lesion, the last two patients also had a secondary lesion. Every lesion involved MRI-TBx-proven histologically significant PCa. On treatment planning, lesions were contoured with 5 mm of overlap when feasible, except near the NVB where a posterolateral 3 mm safety margin was ethically required for this treat-and-resect study, regardless of lesion coverage.
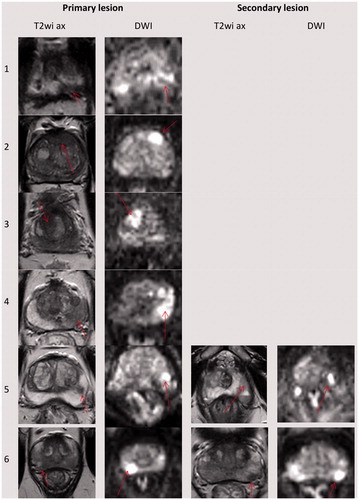
Study intervention
Targeted ablation was successful in every study patient, with target volumes of 7–19 ml and radii up to 33.6 mm in prostates ranging from 42–82 ml. Median sonication time and in bore MRI time were 17 (range = 11–52) min and 117 (range = 82–185) min, respectively (Supplementary Table S1).
The study intervention was performed under spinal anesthesia in one patient and general anesthesia in the others. A guidewire was used for secure insertion of the ultrasound applicator, without cystoscopy. In contrast with other reports of similar technology suprapubic catheterization was avoided; having no urinary drainage during the procedure did not compromise the procedure [18]. Two patients were discharged on the same day and four patients admitted overnight for logistical reasons. The median hospital stay was 23 h (range = 10.5–33) (Supplementary Table S1). Catheterization time was 2–3 days and there were no differences between pre- and post-TULSA uroflowmetries (Supplementary Table S2).
Safety and toxicity
Over 3 weeks follow-up, none of the study patients had any treatment-related complications. Mean VAS pain score during hospitalization was 2, decreasing to 1 at catheter removal visit, and then to 0 at weeks 1 and 3. There were no differences in any of the QoL outcomes between baseline and 3 weeks after TULSA. Three patients having baseline erection firmness sufficient for penetration had penetrations post-TULSA with normal antegrade ejaculations. All had normal continence at 3 weeks after TULSA. None of the study patients reported any bowel symptoms (Supplementary Table S3).
Post-TULSA RALP
The RALP procedures were uneventful. Minor localized inflammatory effects with periprostatic adhesions were noted; however, these did not compromise oncological radicality or nerve-sparing technique in any of the study patients. All study patients presented negative margins.
Targeting accuracy and evolution of ablation extent
Details of thermal targeting accuracy measurements are presented in Supplementary Table S4. Mean targeting accuracy was –0.5 ± 1.4 mm, with 95 ± 2% thermal coverage indicating minor undertreatment.
Post-TULSA MRI indicated a 29% (mean) increase in total prostate volume at 1 week, decreasing at 3 weeks but remaining 4% (mean) greater than baseline. NPV increased gradually over 3 weeks with a mean change of 36% (). Locality and morphology of the 3-week NPV correlated with the respective complete necrosis on histology in all patients (). 3-week NPV was 19% (mean) larger than complete necrosis volume on histology after accounting for average total prostate volume shrinkage on histology of 26%.
Figure 2. Lesion-targeted TULSA treatment (a) and 3-week follow-up (b) for patient number 2. (a) Intra-procedural MR images, with immediate post-treatment contrast-enhanced overlay images from active elements showing high spatial concordance between target volume (top row), 55 °C isotherm volume (cytocidal temperature, second row), thermal dose coverage (third row), and non-perfused-volume (NPV, fourth row). (b) Baseline imaging identified an anterior 3 cc PIRADS 5 lesion with a biopsy-concordant high-volume Gleason 3 + 3 PCa (MRI-TBx 4/4 positive cores, systematic biopsies 6 + 6 negative). Post-treatment, 1- and 3-week NPV and 3-week whole-mount hematoxylin-eosin-stained slide demonstrate high volumetric and morphometric concordance. Note that complete necrosis reached the capsule and thermal damage was well-confined. NPV covered the entire PIRADS 5 lesion and no vital cancerous tissue was reported in the vicinity of the targeted lesion. In final histopathology of the whole prostate specimen, only a posteroapical MRI-negative microfocus of vital histologically insignificant Gleason pattern 3 PCa was detected.
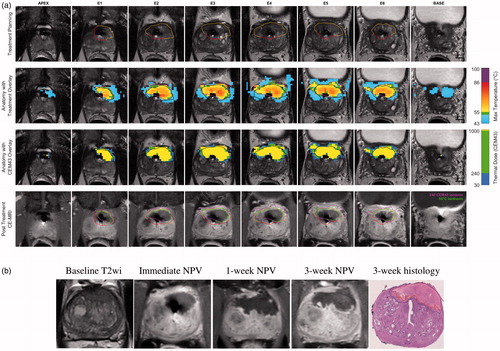
Table 3. TULSA for targeted ablation of PCa-lesions: The outcomes of treatment efficacy for targeted regions at 3 weeks
Immediate NPV was consistently smaller than the planned target volume. The 240 CEM isodose boundary extended beyond the target volume and was larger than the 3-week NPV. The volume of the 57 °C isotherm correlated best with the 3-week NPV ().
The gradual growth of NPV and disappearance of the peripheral rim of enhancement between 1 and 3 weeks on CE-MRI indicated that some of the peripheral rim of enhancement surrounding the initial NPV was devascularized. On whole-mount histology acquired 3 weeks post-TULSA, the mean DTI between complete necrosis and the outer limit of thermal injury across all study patients was 1.7 ± 0.4 mm, in close agreement with previous same-day treat-and-resect studies [Citation20].
Treatment efficacy
Radiological assessment of 3-week treatment efficacy indicated no ablative or targeting failures. Histological assessment of in-field treatment efficacy indicated no ablative failures and no targeting failures. However, as expected based on the conservative treatment plan required in this treat-and-resect-study, 4/6 patients had residual cancer outside of the planned ablation volume, inside the pre-planned 3 mm safety margin near the NVB at the prostate capsule (). While these regions were excluded from the planned ablation volume to minimize the impact on subsequent possible nerve-sparing prostatectomy in this treat-and-resect study, they would be targeted if TULSA was administered with curative intent.
Discussion
This is the first study reporting short-term safety, toxicity, and QoL outcomes after lesion-targeted ablation with TULSA. Also, no other TULSA studies have evaluated short-term radiological and histological evolution of ablative effects and demarcation of thermal injury, including delayed cell death. Furthermore, no other study has demonstrated thoroughly that ablation to the prostate capsule can be achieved with this treatment method. We report here our initial experience of lesion-targeted TULSA followed by RALP at 3 weeks and demonstrated safe, painless, accurate and efficacious in-field ablation of PCa-lesions.
In contrast with the Phase 1 study reported by Chin et al. [Citation18], no urinary tract infections or urinary retentions occurred in our study patients, possibly related to the smaller ablation volumes with lesion-targeted TULSA compared to the whole-gland approach. Other reasons might be that cystoscopy was not performed, suprapubic catheterization was avoided and catheterization time was considerable shorter; 2–3 days compared to 2 weeks.
Previous treat-and-resect-studies investigating similar technology had immediate rather than delayed post-TULSA prostatectomy and therefore did not evaluate delayed thermal effects or the evolution of NPV [Citation16,Citation17]. The interval of 3 weeks between TULSA and surgery was chosen to avoid excessive delay before definitive treatment while enabling evaluation of delayed thermal effects. Importantly, post-TULSA NPV gradually increased in size, by an average of 36% at 3 weeks, which is likely to have substantial clinical implications on both oncological and functional outcomes. Also, TULSA showed sharp DTI on histology. This new understanding of the evolution of lesion size and DTI may be utilized in treatment planning for future trials and for ongoing commercial TULSA treatments under CE Mark.
Our study population included solely MRI–visible biopsy-concordant PCa, representing 50% intermediate-risk and 50% high-risk disease, differing from previous studies exploring similar technology in mainly low-risk disease [Citation18–20,Citation25]. Our study confirms the tissue-type independence of TULSA ablation, demonstrating that predominant Gleason pattern 4 tumors respond to TULSA with complete cell death verified on histopathology.
After these first six treatments it was evident that TULSA was feasible and safe for lesion-based ablation. There was obvious morphometric and volumetric concordance between radiology and histology in all targeted tumors. In terms of quantitative accuracy measurements, the primary endpoint, the results of the mid-term analysis of the first six study patients were also consistent. Therefore, we decided not to continue recruiting the preplanned amount of 10 patients; for this treat-and-resect-study in which participants receive no additional oncological benefit beyond the planned RALP, it would not have been ethically sustainable.
While the treat-and-3-week-resect-study design had several advantages enabling new understanding of TULSA, it also introduced several limitations. The study population was small, although typical for a treat-and-resect study design that offers no benefit to patients, and mixed with both intermediate- and high-risk tumors. Longer-term toxicity could not be explored, although favorable 3-week safety and QoL may predict longer-term functional outcomes. Importantly, the study design precluded evaluation of longer-term oncological outcomes. However, the long-term safety and efficacy of whole-gland TULSA are being assessed in larger cohorts [Citation18,Citation26]. Another limitation is that, due to the treat-and-resect study design, a conservative treatment strategy was ethically required near the NVB, contributing to residual cancer found on histology in four patients, all outside the intended targeted region within the 3 mm safety margins. This was an expected result and concordant with treatment planning, demonstrating accurate targeting and sharp demarcation of thermal damage. If TULSA was delivered as a definitive treatment without RALP, the treatment strategy would have been more radical also near the NVB, where all residual cancers were observed. Given the 30 mm typical ablation depth achievable with the commercial TULSA system, ablation of posterolateral lesions is expected to be feasible in nearly all prostates. While a more radical approach to ablation may result in better cancer eradication, its impacts on functional outcomes are unknown. Furthermore, while more aggressive treatment near the NVB could cause more genitourinary morbidity, consistency between planned and observed necrosis boundaries with sharp DTI suggests that ablation of lesions extending into the NVB could be achieved in a controlled manner. Although capsular/extracapsular ablation near NVB was not investigated in the present study, we want to highlight that this is the first TULSA study that demonstrates the capability of ablation to reach the prostate capsule and beyond in all other parts of the gland, which has significant clinical implications.
Additional limitations of this study stem from the challenges inherent to direct quantitative comparison of imaging with histology. First, it was not possible to obtain precise spatial concordance between post-TULSA MRI and RALP sections, partially because the TULSA instrumentation influences the morphology of the prostate. Second, histopathological processing substantially alters the specimen volume, configuration and composition, hampering the ability to make precise comparisons [22]. Third, thermal damage and subsequent biological response over the 3-week follow-up period have a non-uniform effect on both the prostate and target volumes. Therefore, direct quantitative comparisons were limited to measurements of treatment-day targeting accuracy. Radiological and histological accuracy at delayed time points were assessed based on measures of volumetric and morphometric concordance. In this study we reported that 3-week NPV was 19% larger than the delayed necrosis volume, which is consistent with previously reported results with similar technology [Citation23,Citation24].
Conclusion
Lesion-targeted TULSA appears feasible, enabling personalized and flexible treatment with a potentially lower toxicity profile than whole-gland TULSA. While short-term follow-up resulted in no adverse effects or compromise to function up to 3 weeks, long-term impacts are unknown, especially when extending ablation into the NVB. Long-term oncologic outcomes after lesion-targeted TULSA are unknown, but our study demonstrates the feasibility of accurate and efficient targeted ablation of PCa lesions. Combined with encouraging data on the feasibility and safety of whole-gland TULSA, these results warrant continuation into Phase II studies of definitive targeted TULSA for localized PCa lesions with radical intent.
Revised_Supplemental_material.docx
Download MS Word (10.8 MB)Disclosure statement
No conflicts of interest to be declared.
References
- Hamdy FC, Donovan JL, Lane JA, et al. 10-Year outcomes after monitoring, surgery, or radiotherapy for localized prostate cancer. N Engl J Med. 2016;375(15):1415–1424.
- Wilt TJ, Jones KM, Barry MJ, et al. Follow-up of prostatectomy versus observation for early prostate cancer. N Engl J Med. 2017;377(2):132–142.
- Bill-Axelson A, Holmberg L, Garmo H, et al. Radical prostatectomy or watchful waiting in early prostate cancer. N Engl J Med. 2014;370(10):932–942.
- Donovan JL, Hamdy FC, Lane JA, et al. Patient-reported outcomes after monitoring, surgery, or radiotherapy for prostate cancer. N Engl J Med. 2016;375(15):1425–1437.
- Valerio M, Cerantola Y, Eggener SE, et al. New and established technology in focal ablation of the prostate: a systematic review. Eur Urol. 2017;71(1):17–34.
- Ahmed HU, El-Shater Bosaily A, Brown LC, et al. Diagnostic accuracy of Multi-parametric MRI and TRUS biopsy in prostate cancer (PROMIS): a paired validating confirmatory study. Lancet. 2017;389(10071):815–822.
- Kasivisvanathan V, Rannikko AS, Borghi M, et al. MRI-targeted or standard biopsy for Prostate-cancer diagnosis. N Engl J Med. 2018;378(19):1767–1777.
- Ishihara Y, Calderon A, Watanabe H, et al. A precise and fast temperature mapping using water proton chemical shift. Magn Reson Med. 1995;34(6):814–823.
- Chopra R, Tang K, Burtnyk M, et al. Analysis of the spatial and temporal accuracy of heating in the prostate gland using transurethral ultrasound therapy and active MR temperature feedback. Phys Med Biol. 2009;54(9):2615.
- Böni RA, Sulser T, Jochum W, et al. Laser ablation-induced changes in the prostate: findings at endorectal MR imaging with histologic correlation. Radiology. 1997;202(1):232–236.
- Rouvière O, Lyonnet D, Raudrant A, et al. MRI appearance of prostate following transrectal HIFU ablation of localized cancer. Eur Urol. 2001;40:265–274.
- Rosset R, Bratan F, Crouzet S, et al. Can pre- and postoperative magnetic resonance imaging predict recurrence-free survival after whole-gland high-intensity focused ablation for prostate cancer? Eur Radiol. 2017;27(4):1768–1775.
- Boyes A, Tang K, Yaffe M, et al. Prostate tissue analysis immediately following magnetic resonance imaging guided transurethral ultrasound thermal therapy. J Urol. 2007;178.(3):1080–1085.
- Sapareto SA, Dewey WC. Thermal dose determination in cancer therapy. Int J Radiat Oncol Biol Phys. 1984;10(6):787–800.
- Chu KF, Dupuy DE. Thermal ablation of tumours: biological mechanisms and advances in therapy. Nat Rev Cancer. 2014;14(3):199–208.
- Burtnyk M, Hill T, Cadieux-Pitre H, et al. Magnetic resonance image guided transurethral ultrasound prostate ablation: a preclinical safety and feasibility study with 28-day followup. J Urol. 2015;193(5):1669–1675.
- Siddiqui K, Chopra R, Vedula S, et al. MRI-guided transurethral ultrasound therapy of the prostate gland using Real-time thermal mapping: initial studies. Urology. 2010;76(6):1506–1511.
- Chin JL, Billia M, Relle J, et al. Magnetic resonance imaging-guided transurethral ultrasound ablation of prostate tissue in patients with localized prostate cancer: a prospective phase 1 clinical trial. Eur Urol. 2016;70(3):447–455.
- Ramsay E, Mougenot C, Staruch R, et al. Evaluation of focal ablation of magnetic resonance imaging defined prostate cancer using magnetic resonance imaging controlled transurethral ultrasound therapy with prostatectomy as the reference standard. J Urol. 2017;197(1):255–261.
- Chopra R, Colquhoun A, Burtnyk M, et al. MR imaging-controlled transurethral ultrasound therapy for conformal treatment of prostate tissue: initial feasibility in humans. Radiology. 2012;265(1):303–313.
- Weinreb JC, Barentsz JO, Choyke PL, et al. PI-RADS prostate imaging – Reporting and data system: 2015, version 2. Eur Urol. 2016;69(1):16–40.
- Priester A, Natarajan S, Khoshnoodi P, et al. Magnetic resonance imaging underestimation of prostate cancer geometry: use of patient specific molds to correlate images with whole mount pathology. J Urol. 2017;197(2):320–326.
- Lindner U, Lawrentschuk N, Weersink RA, et al. Focal laser ablation for prostate cancer followed by radical prostatectomy: validation of focal therapy and imaging accuracy. Eur Urol. 2010;57(6):1111–1114.
- Bomers JGR, Cornel EB, Futterer JJ, et al. MRI-guided focal laser ablation for prostate cancer followed by radical prostatectomy: correlation of treatment effects with imaging. World J Urol. 2017;35(5):703–711.
- Napoli A, Anzidei M, De Nunzio C, et al. Real-time Magnetic Resonance–guided High-intensity focused ultrasound focal therapy for localised prostate cancer: preliminary experience. Eur. Urol. 2013;63(2):395–398.
- Eggener S, Penson D, Pavlovich C, et al. LBA-26 Pivotal trial of MRI-guided transurethral ultrasound ablation in men with localized prostate cancer. J. Urol. 2019;20:e1004.