ABSTRACT
This work evaluates and discusses the greenhouse gas (GHG) emissions reduction potential of agroecological policies implemented in public food procurement for school canteens. Using a life cycle analysis (LCA) methodology (cradle-to-grave approach) and the information gathered from the Red Municipal de Comedores Escolares (Local Network of School Canteens) in the municipality of Ames (A Coruña, Galicia, Spain), the results show how organic production, dietary change (less animal protein), seasonal consumption, and packaging reduction, among other measures, can mitigate the climate impact of school meals. The food policies implemented in Ames have allowed mitigating the GHG emissions of local school canteens by 13.4%. Under realistic conditions, this reduction could rise to 40.6% should the agroecological transformation of the food system continue to advance.
Introduction
Across the planet, many peasants, farmers, consumers and social actors, as well as political and educational organizations, are fighting against the socioeconomic crisis and the unsustainability of the globalized model by encouraging agroecological transition processes toward sustainable food systems (Méndez, Bacon, and Cohen Citation2013). However, and despite the increasing number of successful experiences, massification and the scaling of agroecology are still two of the main challenges in that fight (Gliessman Citation2018). In scientific literature, the debate on agroecological scaling strategies is intense (see, for example, Ilieva and Hernández Citation2018; Mier y Terán Giménez Cacho et al. Citation2018). Scaling agroecology does not mean identifying the “good practices” of a territory to replicate them somewhere else (Wigboldus et al. Citation2016), but transforming the whole agrifood system at different scales from a political and structural perspective. On the one hand, the scaling out of agroecology refers to the need to expand it both quantitatively and geographically – more farmers and families producing and consuming agroecological products in more places – (Gliessman Citation2014), i.e., to increase the size of sustainable food systems. On the other hand, the scaling up of agroecology involves a shift in the logic of institutional policies (Altieri and Nicholls Citation2005) from sectoral policies to food policies (IPES Food Citation2019), i.e., policies that act on the economy, education, market regulation, evaluation systems, etc. (Gliessman Citation2019; Rosset and Altieri Citation2017).
National, regional, even local governments are institutions with a great capacity for action and responsibility on the provision of food that guarantees healthy and fair conditions for all citizens and that is environmentally friendly (Benvenuti et al. Citation2016; Moragues-Faus, Sonnino, and Marsden Citation2017). They have the legislative and executive capacity and competence to implement the fiscal, educational, health and other policies required. For instance, governments may foster changes in food marketing or goods labeling systems, procure food grown or manufactured under environmentally and health-friendly criteria, etc. (Sönnichsen and Clement Citation2020; Wirsenius, Hedenus, and Mohlin Citation2011). They may even forbid damaging production practices or food components that are suspected of having negative impacts on people’s health or the environment. Among other political tools available to governments to promote sustainable food and, therefore, contribute to the scaling of agroecology, we can highlight public food procurement (Parsons and Hawkes Citation2018; Swensson and Tartanac Citation2020). Sustainable public food procurement is one of the Sustainable Development Goals that could clearly contribute to the generalization of local zero-carbon food systems based on diets that minimize all negative impacts on health (Gaddis and Coplen Citation2018; UN Citation2018).
In this sense, it is hardly surprising that public food procurement for school canteens is receiving special attention from the field of agroecology (Kleine and Das Graças-Brightwell Citation2015; Petersen and Silveira Citation2017). The agroecological design of public food procurement may contribute to the achievement of such global objectives as food sovereignty and the right to food, as well as to the reinforcement of environmental education for action in schools (Powell and Wittman Citation2018). In this sense, policies that encourage food procurement according to the following factors are especially relevant: a) “how” food is produced, supporting sustainable agriculture based on local resources (Borsatto et al. Citation2019; Nuutila, Risku-Norja, and Arolaakso Citation2019); b) “where” food is produced, promoting the purchase of proximity food and favoring the territorial distribution of income, as well as local development (Gliessman Citation2015; Soares, Caballero, and Davó-Blanes Citation2017); c) “when” food is consumed, fostering a reduction in the consumption of non-seasonal produce (Francis et al. Citation2003); and d) “what” is consumed, normalizing diets that help mitigate environmental impacts and damage to people’s health (Aleksandrowicz et al. Citation2016; Conrad et al. Citation2018). Therefore, the scaling of agroecology through public food procurement, in addition to political will, requires that purchasing decisions stop being guided by the “lowest price” economistic principle and include other criteria and indicators that reflect, to the extent that it is possible, the various impacts and benefits of all processes along the food supply chain (Filippini et al. Citation2018; Goggins and Rau Citation2016).
Although it is true that the regulation framework currently in force across Europe and, consequently, in Spain does not hinder the generalization of more transformative public procurement policies and the consideration of non-economistic criteria (Galli et al. Citation2014), the actual political practice is rarely heading in that direction. In addition, within this framework, no clear scientific criteria defining, for instance, healthy diets or sustainable production systems (Conrad et al. Citation2018; Springmann et al. Citation2016) have been established, not even thresholds for strong environmental impact indicators that can be used to design policies for improvement (Cerutti et al. Citation2017; Galli et al. Citation2014). In this line, new indicators are needed to quantify the existing nexus between the prevailing diets and their impact on human health and the environment (IPES Food Citation2017; Tilman and Clark Citation2014), while allowing the establishment of reliable criteria to advance in food sustainability. Life cycle analysis (LCA) is an international methodology that enables assessing, in consonance with the agroecological proposal, the environmental impact of the agrifood system as a whole, from input production to waste management (ISO Citation2006), given that agroecology is the ecology of the whole food system, from the field to the table, including all processes taking place in between (Gliessman Citation2020). LCA makes it possible to evaluate and monitor public policies, both sectoral and food-based: not only because it sheds light, using a scientific and institutionally approved language, on the critical aspects that require intervention, but because it has the potential to highlight the environmental benefits of implementing agroecological food policies that, among other effects, can mitigate the climate impact of food (Giacomo et al. Citation2019; IPES Food Citation2016).
LCA has been used to assess the individual impact of many crops (Clune, Crossin, and Verghese Citation2017), but also to analyze the environmental pressure of conventional diets (Batlle-Bayer et al. Citation2020) and alternative diets, showing that the latter can have positive effects on health and prevent chronic diseases, while reducing GHG emissions, species loss and deforestation (Esteve-Llorens et al. Citation2019; González-García et al. Citation2018). The LCA has shown how vegetarian diets can have a lower impact than diets rich in animal protein (Corrado et al. Citation2019; Poore and Nemecek Citation2018; Westhoek et al. Citation2014), or how organic and Mediterranean diets, which are more aligned with health-based dietary guidelines, can mitigate climate pressure (Batlle-Bayer et al. Citation2019; Treu et al. Citation2017). In relation to school canteens, previous studies have focused mostly on estimating the carbon footprint of school meals (Caputo, Ducoli, and Clementi Citation2014; De Laurentiis et al. Citation2019; De Laurentiis, Hunt, and Rogers Citation2017). Thus, for Wickramasinghe et al. (Citation2016; Citation2017) have shown how, in the English case, diets that are low in salt, saturated fats and sugar produce less GHG emissions throughout their life cycle. The work of Cerutti et al. (Citation2017) is especially interesting, because it assesses the effect of different policies aimed at reducing the climate impact of school canteens in Italy through dietary change, sustainable production, seasonal consumption, etc. Recently, Martínez et al. (Citation2020) have calculated the carbon footprint of school meals in Spain that follow the Spanish school dietary guidelines, and assessed six alternative menus. Despite these relevant precedents, there are no previous analyses of the success, in terms of environmental impact reduction, of implementing agroecological public policies in the area of education.
In the European Union, public procurement of goods and services accounts for almost 20% of the GDP (COM Citation2013), while the budget of the whole social food service market, where education services are included, amounts to EUR 82,000 million. In Spain, public procurement of goods and services also represents over 20% of the GDP (CNMV Citation2019). According to estimates made by VSF (Citation2013), Spanish public administrations spend around EUR 2,000–3,000 million in food purchasing. In addition to their economic relevance, public schools in Spain have more than 2.2 million users (MEYFP Citation2020), 73% of whom usually eat in the school canteen an average of 165 days per year (ME Citation2016). In Galicia, around 120,000 children study in primary schools, and most of them (approximately 70%) (MEYFP – Ministerio de Educación y Formación Profesional Citation2020) do it in public schools. The annual public expenditure on school canteens e non-school meals in Galicia amounts to EUR 28.8 million of the regional budget (CEUFP Citation2020).
The Spanish Ministry of Education has issued some dietary guidelines for the design of school menus according to the students’ developmental stage and nutritional needs (ME Citation2016). However, this school guide does not include any environmental sustainability guidance (Martínez et al. Citation2020). On the contrary, multimillion-euro tenders are called where economistic decision-making criteria prevail and economic margins are relatively small. Consequently, economies of scale are essential to ensure businesses viability. Usually, school catering services are managed in a centralized way, using refrigeration systems to preserve conventional food cooked in kitchens outside the school, sometimes far from it and therefore long before it is consumed. This centralized food production and supply model is dominant in Galicia and, overall, in Spain (Muñoz-Rico Citation2018). In this model, seasonal consumption, energy efficiency, or the fight against climate change are little, if at all, considered along the supply chain.
In this context, our analytical proposal focuses on the experience of collective food consumption in pre-school and primary education centers as part of a program developed by the Rede Municipal de Comedores (Local School Canteen Network) in the municipality of Ames (Galicia, Spain). The starting point for this Network was similar to the above-described situation (conventional produce, little seasonality, etc.), but differed in that the catering services were provided by local kitchens supervised by local authorities. However, from 2005 onwards, this network has promoted agroecological policies for the purpose of establishing a more direct connection with those who produce food under the agroecological principles of equity, democracy and justice (Gliessman Citation2015). In a context of unusual population growth,Footnote1 the local government decided to support food system transformation in local schools, fostering the purchase of food that is locally, organically and seasonally produced for the meals of 1,456 children in five public schools, i.e., 195,000 school meals per year.Footnote2
The main objective of this work is to assess the GHG emissions reduction potential of agroecological policies implemented in public food procurement, more specifically, for school canteens. In order to focus the discussion, we have analyzed the global warming potential in 100 years (GWP 100a) of the complete life cycle of school canteens in the Concello de Ames (Municipality of Ames), in the province of A Coruña. This LCA considers five phases of the agrifood system. As a starting point, we analyzed the environmental behavior of school canteens before any agroecological public policy was implemented (baseline scenario). After this first assessment was made, the main hotspots in the daily operation of the school canteens were identified and the GHG emissions reduction potential was calculated according to eight drivers related to: 1) how the products are produced (shift from conventional to organic agriculture); 2) where the products are produced and consumed (search for greater proximity and transportation efficiency); 3) when and how the products are consumed (greater seasonal consumption, packaging reduction, use of green electricity); and 4) what products are consumed (dietary change). Taking into account these results, we built three “agroecological transition” scenarios toward low-carbon agrifood systems. The first scenario reflects the current GHG emissions of school canteens in Ames (after the local policies in force today were implemented), while the other two scenarios perform the reduction potential associated with the structural transformation of the food system through the implementation and intensification of agroecological policies in public food procurement.
Materials and methods
System boundary, functional unit and data collection
The GWP (100a) of school canteens in Ames was estimated by performing an LCA (cradle-to-grave approach) (ISO Citation2006) that considered the following phases of the life cycle of food consumed therein: 1) in-farm production (direct and indirect emissions associated with the use of inputs and capital); 2) packaging (plastics, tin containers, etc.); 3) transportation (direct emissions); 4) Primary energy used in schools (food preservation and cooking); 5) auxiliary material (tableware, etc.); and 6) waste management (organic waste and others), according to two functional units: the yearly total and the average meal. For this purpose, information was gathered on: a) annual food consumption (more than 80 products); b) packaging; c) distances and transportation modes; d) energy expenditure (electricity and other sources); e) material consumption (napkins, waste bags, tableware, cleaning products, etc.); and f) waste production (both organic and inorganic).
A significant part of this information was drawn from in-depth inventories taken by the people responsible for the school canteens (a, d and e), while the rest was gathered from secondary sources and/or estimates (b and c). In the case of packaging, the plastic, cardboard and tin containers of the main products consumed in the school canteens were weighed to calculate their share of the total waste produced. For transportation, the average behaviors, for both distances and modes, reflected in the information available for Spain were taken to represent the behaviors in this case. Thus, the import/export data provided by the FAO (Citation2020) were used to calculate the degree of food dependency by product, while data on distances and transportation modes were obtained from Pérez-Neira et al. (Citation2016), who analyzed international food transportation (travels within the exporting country were, however, not considered, which led to an underestimation of the impact that is assumed in the present work). For food produced in Spain, the origin was considered, distinguishing Galicia from the rest of Spain on the basis of the information collected from the inventories. When this information was lacking, the Spanish region that is the largest producer of a specific product was taken as its place of origin (for instance, Andalusia for olive oil). By using geographical information systems, the minimum travel distances were estimated (place of origin/manufacture–Ames). For the final phase of transportation (retailer–school), the information was gathered from the inventories, where the expenditure on fuel and the type of vehicle used were registered. Finally, the management of organic waste (f) was estimated through technical food waste coefficients (Moreiras et al. Citation2005).
Estimation of the environmental impact (GWP): Baseline, drivers and agroecological transition scenarios
Once the information was gathered, the GWP was estimated by implementing a CML-IA baseline version 3.05 methodology using SimaPro software and the Ecoinvent 3.5 and Agribalyse 3.0 databases, from which environmental data were drawn for 95% of the inputs studied (food, packaging, transportation, cooking, auxiliary material, and waste). The impact of the remaining 5% was calculated from data collected from specialized literature. In the case of olive oil and fish, the works of Cappelletti et al. (Citation2014), Iribarren et al. (Citation2010) and Clune, Crossin, and Verghese (Citation2017) were used to complete the SimaPro estimates. Specialized literature was also consulted to calculate the impact of some organic products. More specifically, the work of Astier et al. (Citation2014) was used to estimate the GWP of organic avocados; that of Roibás, Elbehri, and Hospido (Citation2016) to estimate that of bananas; Hospido et al. (Citation2009), Aguilera, Guzmán, and Alonso (Citation2013), and Pérez-Neira and Grollmus (Citation2018) for vegetables; Vitali et al. (Citation2018) for beef; Taylor, Omed, and Edwards-Jones (Citation2014) for organic and free-range eggs; Michos et al. (Citation2012) for peaches; Pergola et al. (Citation2013) for oranges; Inci et al. (Citation2016) for chicken meat; Pérez-Neira et al. (Citation2020b) for organic chocolate. Likewise, for transportation electrification, the works by Delgado-Cabeza et al. (Citation2015) and Pérez-Neira, Rodriguez-Fernandez, and Hidalgo-Gonzalez (Citation2020a), which report specific information on the Spanish case, were also used. From the inventory and the model design, a first, baseline scenario (conventional) was estimated to reflect the climate behavior of the local school canteens before any agroecological public policy was implemented. This first scenario was defined by the parameters shown in .
Table 1. Description and scope of the baseline scenario reflecting the behavior of school canteens in Ames before the implementation of agroecological public policies
Once the environmental impact of the baseline scenario was calculated and its main hotspots were identified, the potential of nine agroecological sustainability drivers to mitigate GHG emissions in the local school canteens was calculated: procurement of organic products (S1) and local products (S2a); electrification of the transportation modes used for the distribution of food from stores to schools (S2b); consumption of seasonal vegetables and fruit (S3a); reduction of food packaging (S3b); procurement of green (renewable) electricity (S3c); increase in the consumption of inshore (Galician) fish rather than frozen fish (S4a); substitution of fish for beef (S4b); and reduction in the consumption of animal products (meat, fish and milk) (S4c). The description of each driver, as well as their scope and capacity to modify the baseline scenario, are synthesized in . The assumptions of improvement have been defined using pragmatical criteria. In other words, based on knowledge of the context, we have assessed the reduction of emissions associated with changes that can be implemented by the school canteens in the short/medium term. These changes comprise procuring up to 65% of organic food, increasing the consumption of Galician foodstuffs by 40%, or including 70% of seasonal vegetable products in the meals according to the local production capacity, among others.
Table 2. Description and scope of the drivers of change applied to the baseline model
Subsequently, using these drivers, we built three scenarios that performed different phases of the agroecological transition toward low-carbon agrifood systems (). The first one (AT1) estimates the current environmental impact of school canteens in Ames, i.e., the impact once the local policies meant to improve the sustainability of school canteens were implemented. The other two scenarios (AT2 and AT3) aim at capturing the GHG emissions reduction potential associated with a more intense agroecological transformative impulse of the policies initiated by the local government of Ames. The road from AT1 to AT3 involves organizing food trade and transportation in an equitable manner and avoiding all environmental impacts. It also involves supporting local food production (Vaarst et al. Citation2018), which is threatened by globalization and by the monopolization of genetic diversity and the ever-increasing homogenization of diets associated with it (Tittonell Citation2015).
Table 3. Agroecological transition scenarios in the school canteens of Ames
Results
Climate impact of school canteens in Ames: from cradle to grave
Total GHG emissions of school canteens in the municipality of Ames as in the baseline scenario were estimated at 266 t of CO2-eq, which corresponds to 1.36 kg of CO2-eq per meal (). Consuming only conventional food reinforces the environmental impact of the food system: production is the phase in the life cycle of school meals that has a larger impact on the total (68.9%), followed by transportation (11.7%), packaging (5.1%), primary energy use in schools (8.3%), waste management (3.9%), and auxiliary material (2.0%) ().
Table 4. GHG emissions of school canteens in Ames (Cradle to grave approach)
From farm to school
shows the quantities of foodstuffs of the ten main food groups used in school meals and the emissions caused by their production/manufacture, packaging and transportation, which accounted for 85.7% of the total GHG emissions. Thus, during the schoolyear, school canteens in Ames purchased 138 t of food, which amounted to 0.71 kg per meal, and 0.50 kg per meal if bottled water was excluded. The group of bottled water and other products (chocolate, salt, sugar, species, paprika, etc.) was the one with the largest share of the total (30.3%), mainly due to the purchase of water (96.4% within the group). The group of vegetables, fruit, meat and fish was next in importance, representing 25.4%, 14.0%, 7.3%, and 5.2%, respectively, of the total food weight (). From a nutritional perspective, cereals and potatoes, fruit, and vegetables accounted for 25.5%, 21.0% and 23.0% of the menu, respectively, whereas protein-based products contributed 25.6%, and dairy products the remaining 4.9%. Of the protein-based products consumed in Ames, 93% were of animal origin.
Table 5. Quantities of foodstuffs of the ten main food groups consumed in the school canteens of Ames and GHG emissions caused by their production/manufacture, packaging and transportation (baseline scenario) (cradle-to-school approach)
Figure 2. Quantities and GHG emissions of the main food groups (a), and distribution according to their share in the production, packaging and transportation phases (b) (cradle-to-school approach) (%)
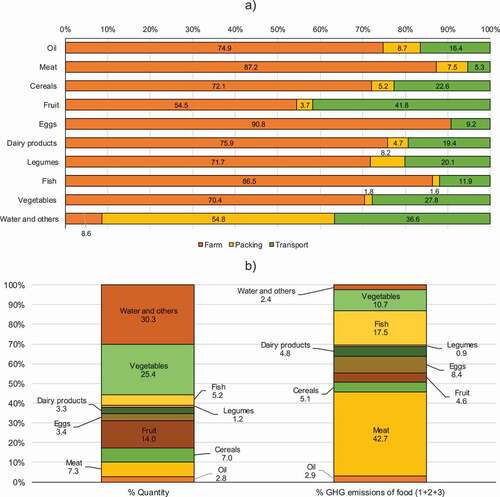
In the baseline scenario, animal products are responsible for the largest environmental impact of the school canteens: they produced 73.4% of the emissions, despite accounting for only 19.2% of the food weight ( and ). Meat and fish are the groups with the largest impacts (42.7% and 17.5%), followed by vegetables, eggs and cereals (10.7%, 8.4% and 5.1% of the total emissions). It is worth underlining how the absence of seasonality criteria leads to the impact of vegetable consumption being relatively high (10.7% of the total) due to greater consumption of greenhouse products. Up to 50% of the impact of packaging is related to the use of plastic, while the remaining 45% and 5% are associated with glass and tin. Food consumed in Ames traveled an average distance of 1,250 km. Up to 36.7% of the emissions caused by food transportation was associated with local travels (from store to school), whereas 57.6% and 5.7% were due to the regional, country-wide or international mobility of the products (by truck and/or boat) ().
From school to dumping site
The environmental impact associated with planning and cooking the school meals, and managing the resulting waste, amounted to 14.3% of the GHG emissions. Electricity (light, refrigeration) accounted for 30% of the final energy consumed in the school canteens, while the use of fossil fuel (butane and gas) represented the remaining 70.0%. Within the group of auxiliary material, cleaning products (78.1% of this subtotal) are the most relevant item, while the rest corresponds to the use and replacement of tableware (21.8%). Organic and inorganic waste management accounted for 3.9% of the total emissions.
GHG emissions reduction potential: drivers and transition scenarios
As observed on , purchasing organic food instead of conventional food is the driver with the greater potential to mitigate GHG emissions: up to 15.3%, should 65% of the food consumed in the school canteens be organic (S1). The substitution of legumes, vegetables and fruit for 30% of meat and fish (S4c), and the substitution of animal products with a lower impact, like inshore fish, for 50% of beef (S4b), would favor reductions of 11.1% and 6.7%, respectively. The decrease in the use of plastic (by 50%) (S3b) resulting from the implementation of more sustainable practices such as purchasing non-laminated vegetables, as well as legumes and cereals in bulk, or substituting tap water for bottled water and fresh food for processed food, would, in the case analyzed, cut the emissions by 5.1%, 1.7% of which would correspond to the phase of waste management. Procuring 50% of renewable electricity would reduce emissions by 1.5% (S3c). Prioritizing seasonal consumption (S3a) would lead to a reduction of 6.5% of the total emissions – if the consumption of seasonal vegetables and eggs increased from 30% to 70% –, while purchasing local fish (S4a) instead of imported or offshore fish (50%) would cut the emissions by 5.0%. Likewise, procuring local food (reducing tkm by 31%) (S2a) would favor a decrease of around 2.2% of the emissions, while the improvement of efficiency (S2b) in the distribution of foodFootnote3 would cut them by 1.7%.
Figure 3. Reduction of GHG emissions following the implementation of various measures of the agroecological policies analyzed (% of total emissions)
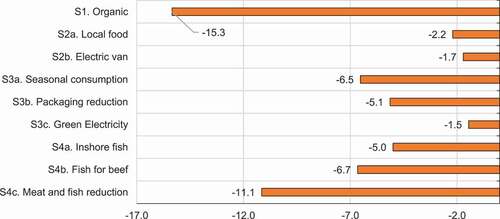
With respect to the transition scenarios analyzed (details in ), the changes introduced at different levels in the school canteens of Ames (organic, local, seasonal purchases, etc.) have allowed – in addition to improving the quality of the menus – mitigating the climate impact by 13.4% in relation to the starting point (scenario AT1 vs. baseline scenario) (). Furthering public policies to reach an advanced stage of the agroecological transition, as reflected in scenario AT2, could lead, under specific and realistic conditions, to a larger reduction of up to 40.6% (AT2 vs. baseline scenario). Likewise, in a highly advanced agroecological transition scenario (AT3), where production and consumption are almost 100% organic and respectful of local environmental conditions; where most food is regionally produced (considering there is no single maximum distance); where the use of plastic and other packaging is minimized or replaced by alternative preservation systems; where electricity comes from renewable sources and the consumption of animal proteins and processed food is significantly reduced, the environmental impact could drop an additional 47.3% in relation to the present situation (AT3 vs. AT1) (). In addition, the impact could decrease by an extra 10.4% should the diet be 100% ovo-dairy vegetarian, in addition to complying with the remaining agroecological principles/drivers mentioned before.
Discussion
From the whole to the parts. Agroecological drivers to reduce the climate impact of school canteens
The climate impact of the school canteens in Ames is similar to those calculated by previous studies on school meals (between 1.02 and 1.67 kg of CO2-eq per meal) (Laurentiis et al. Citation2017, or Cerutti et al. Citation2017), including the estimate made by the Spanish dietary guidelines (±1.22 kg of CO2-eq per meal) (Martínez et al. Citation2020). Public policies that take into account the critical aspects of the conventional food model may encourage the transition toward agroecological food systems (Dumont, Gasselin, and Baret Citation2020), which would allow decreasing the GWP of food consumed in public schools (Benvenuti et al. Citation2016). In this sense, some regional or local governments and initiatives, like the one carried out in Ames, are using public food procurement to foster change in “how” food is produced, encouraging the purchase of organic and/or peasant food (Borsatto et al. Citation2019; Nuutila, Risku-Norja, and Arolaakso Citation2019) because in-farm production is the phase in the life cycle of school meals creating the most environmental pressure (GHG emissions) (Laurentiis et al. Citation2019; Jungbluth, Keller, and König Citation2016). Incorporating the technical and economic principles of agroecology (Sevilla-Guzmán and Soler Citation2010) has the potential of not only mitigating GHG emissions, but favoring biodiversity conservation (Altieri Citation2002), increasing soil organic matter (Simón, Montero, and Bermúdez Citation2020), decreasing dependency on fossil fuels (SOCLA Citation2015), maintaining ecosystem services, and reducing pollution associated with agricultural practices, among other effects. (Aguilera et al. Citation2020).
However, a change in management is not enough. If the commercial distribution (when and how the food is purchased), the consumption patterns (which food is consumed and when) and the institutional framework (how the income is distributed) do not change as well, there is a risk of replicating the conventional agricultural model in the new niches of the organic market (Poux and Aubert Citation2018). In fact, green markets can guarantee an almost full substitution of organic food for conventional food, and yet the relative price of each foodstuff may hinder a change in the diet (González de Molina Citation2013). In this sense, the modification of the consumption patterns (dietary change) has been pointed out as the main driver when it comes to reducing the environmental impact of food (Rockström et al. Citation2019), and some public policies are also pointing in that direction. Diets rich in vegetables (for example, vegetarian or Indian diets) have a better environmental and health profile (for instance, in lowering diet-related mortality) than those rich in animal products (González-García et al. Citation2018; Springmann et al. Citation2016). Consumption patterns that exclude or minimize the intake of animal products show a great capacity to reduce diet-related GHG emissions (Corrado et al. Citation2019; Hallström, Carlsson-Kanyama, and Börjesson Citation2015; Poore and Nemecek Citation2018). In addition, they require a smaller use of land (76%, including arable land, i.e., 19% less than other diets), and help mitigate the environmental pressure caused by other impact indicators, such as acidification, eutrophication and scarcity-weighted freshwater (Poore and Nemecek Citation2018). In spite of this, some authors argue that the complete elimination of animal products from the diet may not ensure an adequate supply of certain important micro-nutrients (for instance, calcium or vitamin D) (González-García et al. Citation2018).
Excessive consumption of animal proteins is one of the main characteristics of the conventional agrifood system (Esteve-Llorens et al. Citation2019), which is replicated in school canteens (Caputo, Ducoli, and Clementi Citation2014; Martínez et al. Citation2020). The menu served in the public schools of Ames, even if sticking to the Spanish dietary guidelines, contains an excess of protein-based foodstuffs (93% of which are of animal origin) when compared, for instance, with the “one plate” model proposed by the United States Department of Agriculture (Citation2017) (25.6% vs. 20% recommended by the UDSA). The regular inclusion of beef, lamb, cheese, butter or pork in the diet increases the climate pressure of school canteen meals (Clune, Crossin, and Verghese Citation2017; Wickramasinghe et al. Citation2016, Citation2017). Consequently, menus that cut the consumption of those products, or replace them with others, will have an enormous potential to diminish the climate impact of school canteens (as shown by S4c, but also by S4b), as well as to reduce the risk of diseases associated with the intensive consumption of this type of food (Springmann et al. Citation2016). The advancement toward healthier and more sustainable diets in school canteens also involves the application of seasonal consumption criteria (Cerutti et al. Citation2017; Francis et al. Citation2003). Consuming vegetables out of season is associated with the use of greenhouses and a higher consumption of industrial inputs, water, and energy, which certainly lead to more GHG emissions (Clune, Crossin, and Verghese Citation2017). In addition, lack of seasonal consumption can indirectly generate more waste through the use of more packaged or processed food.
The production of packaging, particularly made of plastic, is a global environmental problem. Some public procurement options have focused in the reduction of plastic use (Neto Citation2020), to prevent both waste and possible endocrine problems (WHO Citation2013). Often packages are larger than necessary – due to packaging technology and marketing – and, in many products, this could be avoided (Nemat et al. Citation2020). For instance, in Ames, the LCA has shown how replacing bottled water with tap water may reduce plastic waste by 47%. This is a controversial issue, because packaging is a phase in the process that usually allows farmers to obtain a higher value added for their seasonal produce, as well as to preserve the food better and protect it so it can travel longer distances. In this sense, packaging reduction is also connected to “where” the products are purchased. Thus, another important discussion around agroecology and the development of food alternatives focuses on the extent to which the relocation of production and a distribution based on short-channel marketing and endogenous development (Aubry and Kebir Citation2013) can reduce food-related GHG emissions (Coelho, Coelho, and Egerer Citation2018; IPES Food Citation2017).
In the case of Ames, the overall distance traveled by the food used in a conventional menu was 400% longer than the maximum distance that can be traveled within Galicia, which would take us into the sea or even France if we intended to cover it within Spain. In this sense, local food clearly reduces the distances traveled, especially compared with globalized food (Kissinger et al. Citation2019). However, the geographical shortening of supply chains may not automatically alleviate the environmental pressure. In fact, shorter distances can produce contradictory results when the modal distribution is inefficient (Coley, Howard, and Winter Citation2009). Debates around local food and short marketing circuits cannot be limited to the impact of transportation (Coelho, Coelho, and Egerer Citation2018). The literature also points to the environmental inefficiency of local food associated with problems of logistics, the size of distribution experiences, economies of scale, occupancy rate of vehicles, etc. (Loiseau et al. Citation2020; Mundler and Rumpus Citation2012). As seen in the case of Ames, transportation is the driver with the least capacity to reduce emissions among all those analyzed. The use and efficiency of renewable energy in food storage and cooking technology, as well as the new cooking habits, are also relevant drivers of the environmental manage of the supply chain (Caputo et al. Citation2017; Corrado et al. Citation2019; Scherhaufer et al. Citation2018) that are not always considered in agroecological proposals. Finally, it is important to mention the creation of school vegetable gardens and organic matter reuse programs (like the ones implemented in Ames) as contributing not only to closing nutrient cycles but to mitigating GHG emissions (De Sadeleer, Brattebø, and Callewaert Citation2020).
From the parts to the whole: Agroecological food policies for public food procurement
Partially changing one or the other component of the agrifood system, no matter how important they are, turn out to be insufficient. A thorough transformation of the food system (Rockström et al. Citation2019) involves changes and efforts at many levels, demanding participation from many social actors along the food supply chain. Therefore, policies should give up their exclusively industry-based approaches to introduce global perspectives that consider the food system as a whole and contribute in a more effective manner to agroecological scaling (Ferguson et al. Citation2019; Rosset and Altieri Citation2017). As in other public food procurement experiences (Borsatto et al. Citation2019; Kleine and Das Graças-Brightwell Citation2015), the scaling out of individual consumption actions is achieved by involving more actors through the scaling up of agroecological initiatives under a policy-making umbrella with a global approach (Benvenuti et al. Citation2016; Kleine and Das Graças-Brightwell Citation2015). In Ames, mothers, fathers, teachers, complementary services staff and local policy-makers have developed an ongoing dialogue dynamic for the construction of a public system of school canteens that responds to various objectives: family-work balance, social integration, environmental education and local rural development.
Thus, in this town, after the introduction of modest measures at different levels (organic, local and seasonal food purchase, etc.), articulated through the participatory management of the school canteens, not only has the quality of school meals improved, but their climate impact has dropped by 13.4% in relation to the starting point (scenario AT1 vs. baseline scenario, ). This reduction could reach 40.6% under quite realistic conditions. The effect of the initial measures shows the potential for environmental (and social) progress that can be achieved when the political will to improve the food situation of school canteen users is strong. Successful experiences like that of Ames, where public policies favor the production and consumption of agroecological food (for instance, the public procurement of food for school canteens has progressively incorporated more seasonal food through the participation of local producers in the design of the menus), allow advancing the scaling out and scaling up of agroecology, and furthering agroecological transition processes (Ilieva and Hernández Citation2018; Mier y Terán Giménez Cacho et al. Citation2018). Should the transformation of public food procurement for the local school canteens started in Ames be generalized to, for instance, the 120,000 children studying in Galician primary schools, the aggregated GHG emissions reduction effect might reach, under the same conditions considered herein, between 3,900 and 11,700 t of GHG (AT1 and AT2 scenarios). As part of this debate, it would also be important to determine whether cooking the meals locally, in the school kitchens, as it is done in Ames, is a better option than doing it in large centralized kitchens where thousands of meals can be cooked and distributed to hundreds of schools daily. Our work does not compare the environmental impact of the two systems, but it enables us to affirm that the direct participation of local food actors (families, producers, etc.) is giving rise to transformative dynamics in relation to food habits and having positive impacts in the rural context where this innovative initiative is developed.
Limits of the study and future prospects
The present work has limits and we will now mention some of them. From an exclusively environmental point of view, it focuses on one impact category (the global potential warming of GHG emissions), omitting the other impact categories assessed in the LCA. Some information is underestimated, as is the case with the indirect emissions of transportation, and/or is not accurately reflected because of lack of data, as with organic waste or the total distance traveled by imported food. In addition, the study does not analyze other socioeconomic benefits that may derive from agroecological policies like the ones implemented in Ames, such as income flows, employment, or progress in agroecological governance (Giraldo and Rosset Citation2017; López-García et al. Citation2020). Neither does it consider the limits to the actual implementation of those policies (lack of production, poor logistics, high prices, etc.) (Filho et al. Citation2019; Filippini et al. Citation2018). This opens the field for new research works that may complete and further explain the results obtained in the present work.
Final reflections
As discussed throughout this work, public authorities, especially local authorities, may play an important role in advancing the development of sustainable agrifood systems by implementing strategies where other public actors and certain private actors (in our case, educational communities and local producers) are also involved. The objective of those strategies is to keep environmentally damaging practices under control, and to restore and protect the integrity of the Earth systems on which our lives depend. Thus, a shift toward an agroecological approach of food procurement policies may have a significant potential to encourage the transformation of the food system and the scaling of sustainable agrifood systems. Achieving these goals requires the active intervention of the State and other public administrations to adopt and develop the political instruments necessary to protect the ecosystems (by reinforcing organic, agroecological and/or peasant agriculture), reduce food waste through education and better infrastructures, and promote vegetable-based diets, while bearing in mind the social and economic aspects of agroecology.
The experience of Ames illustrates all this and may be considered an agroecological reference that proves that scaling not only is possible but has positive effects on the improvement of environmental and social sustainability. Certainly, reducing GHG emissions by 13.4% is not enough, if the guidelines of the main international agreements on climate change are considered. Yet, it is not to be dismissed for it represents the beginning of a necessary transition process. As seen in the different scenarios built for this work, public food procurement could be much more ambitious and transformative in terms of fighting climate change and developing agrifood alternatives. Repoliticizing school canteens from an agroecological perspective could help channel the efforts of educational communities, food producers and political decision-makers to build a different future, where agroecology is one of the main drivers of sustainable production and consumption decisions.
Acknowledgments
This work has been developed within the framework of a project entitled “La construcción social de la calidad alimentaria: Mediaciones entre la producción y el consumo en una economía basada en el conocimiento” (“The social construction of food quality: Mediation between production and consumption in a knowledge-based economy”) (Ref. CSO2017-85188-r), which has benefitted from the Aid to RDI Projects initiative of the Programa Estatal de Investigación, Desarrollo e Innovación orientada a los Retos de la Sociedad (National Plan for Research, Development and Innovation focused on Societal Challenges), developed by the Spanish Ministry of Economy, Industry and Competitiveness.
Additional information
Funding
Notes
1. Ames tripled its population between 1991 and 2017, and had over 30,000 inhabitants on the latter year.
2. Among other transformative initiatives, the local government of Ames is developing projects based on sustainable food production such as the Huertas Urbanas Sostenibles y Solidarias (Sustainable and Solidarity-based Urban Gardens) for registered local citizens (90 plots, 14% of which are reserved for social emergency cases, and another 14% for older people over 65), and a community compost plan for the processing of organic waste generated by local kitchens and canteens. In addition, there are specific programs to facilitate work-home balance and the integration of women in the labor market, which also include the provision of meals: the “Bos Días Cole” (“Good day, school”) program (for 475 children, before the school day starts) and the “Tardes divertidas” (“Happy evenings”) program (for 310 children, after the canteen is closed and until 9 pm).
3. It is important to remember that, in calculating the impact of food transportation, travels within the exporting countries were not considered due to lack of information. Consequently, the emission reduction capacity of local food is also underestimated.
References
- Aguilera, E., C. Díaz-Gaona, R. García-Laureano, C. Reyes-Palomo, G. Guzmán, L. Ortolani, M. Sánchez-Rodríguez, and V. Rodríguez-Estévez. 2020. Agroecology for adaptation to climate change and resource depletion in the Mediterranean region. A Review. Agricultural Systems 181:102809.
- Aguilera, E., G. Guzmán, and A. Alonso. 2013. Greenhouse gas emissions from conventional and organic cropping systems in Spain. I. Herbaceous Crops. Agronomy for Sustainable Development 35:713–24.
- Aleksandrowicz, L., R. Green, E. J. Joy, P. Smith, and A. Haines. 2016. The impacts of dietary change on greenhouse gas emissions, land use, water use, and health: A systematic review. PLoS One 11:11.
- Altieri, M. A. 2002. Agroecology: The science of natural resource management for poor farmers in marginal environments. Agriculture, Ecosystems & Environment 93:1–24.
- Altieri, M. A., and C. Nicholls. 2005. Agroecology and the search for a truly sustainable agriculture. México: United Nations Environment Programme.
- Astier, M., J. Merlín-Uribe, L. Villamil-Echeverri, A. Garciarreal, M. E. Gavito, and O. Masera. 2014. Energy balance and greenhouse gas emissions in organic and conventional avocado orchards in Mexico. Ecological Indicators 43:281–87.
- Aubry, C., and L. Kebir. 2013. Shortening food supply chains: A means for maintaining agriculture close to urban areas? The case of the French metropolitan area of Paris. Food Policy 41:85–93.
- Batlle-Bayer, L., A. Bala, I. García-Herrero, E. Lemaire, G. Song, R. Aldaco, and P. Fullana-i-Palmer. 2019. The Spanish dietary guidelines: A potential tool to reduce greenhouse gas emissions of current dietary patterns. Journal of Cleaner Production 213:588–98.
- Batlle-Bayer, L., A. Bala, J. Albertí, R. Xifré, R. Aldaco, and P. Fullana-i-Palmer. 2020. Food affordability and nutritional values within the functional unit of a food LCA. An application on regional diets in Spain. Resources, Conservation and Recycling 160:104856.
- Benvenuti, L., A. De Santis, F. Santesarti, and L. Tocca. 2016. An optimal plan for food consumption with minimal environmental impact: The case of school lunch menus. Journal of Cleaner Production 129:704–13.
- Borsatto, R. S., M. A. Altieri, H. C. Duval, and J. Perez-Cassarino. 2019. Public procurement as strategy to foster organic transition: Insights from the Brazilian experience. Renewable Agriculture and Food Systems 35:1–9.
- Cappelletti, G. M., G. Ioppolo, G. Martino Nicoletti, and C. Russo. 2014. Energy requirement of extra virgin olive oil production. Sustainability 6:4966–74.
- Caputo, P., C. Ducoli, and M. Clementi. 2014. Strategies and tools for eco-efficient local Food supply scenarios. Sustainability 6:631–51.
- Caputo, P., M. Clementi, C. Ducoli, S. Corsi, and G. Scudo. 2017. Food Chain Evaluator, a tool for analyzing the impacts and designing scenarios for the institutional catering in Lombardy (Italy). Journal of Cleaner Production 140 (2):1014–26.
- Cerutti, A., F. Ardente, S. Contu, D. Donno, and G. L. Beccaro. 2017. Modelling, assessing, and ranking public procurement options for a climate-friendly catering service. International Journal of Life Cycle Assessment 23:95.
- CEUFP – Consellería de Educación, Universidade e Formación Profesional. 2020. Orzamentos da comunidade autónoma galega do ano 2020.
- Clune, S., E. Crossin, and K. Verghese. 2017. Systematic review of greenhouse gas emissions for different fresh food categories. Journal of Cleaner Production 140:766–83.
- CNMV. 2019. E/CNMC/004/18 Radiografía de los procedimientos de contratación pública en España. https://www.cnmc.es/sites/default/files/2314114_2.pdf.
- Coelho, F. C., E. M. Coelho, and M. Egerer. 2018. Local food: Benefits and failings due to modern agriculture. Scientia Agricola 1 (75):84–94.
- Coley, D., M. Howard, and M. Winter. 2009. Local food, food miles and carbon emissions: A comparison of farm shop and mass distribution approaches. Food Policy 34:150–54.
- COM. 2013. Communication from the commission to the European parliament, the council, the European economic and social committee and the committee of the regions. European Commission. https://ec.europa.eu/environment/nature/ecosystems/docs/green_infrastructures/1_EN_ACT_part1_v5.pdf
- Conrad, Z., M. T. Niles, D. A. Neher, E. D. Roy, N. E. Tichenor, and L. Jahns. 2018. Relationship between food waste, diet quality, and environmental sustainability. PLoS ONE 13 (4):0195405.
- Corrado, S., G. Luzzani, M. Trevisan, and L. Lamastra. 2019. Contribution of different life cycle stages to the greenhouse gas emissions associated with three balanced dietary patterns. Science of the Total Environment 660:622–30.
- De Laurentiis, V., D. V. L. Hunt, and C. D. F. Rogers. 2017. Contribution of school meals to climate change and water use in England. Energy Procedia 123:204–11.
- De Laurentiis, V., D. V. L. Hunt, S. E. Lee, and C. D. F. Rogers. 2019. EATS: A life cycle-based decision support tool for local authorities and school caterers. The International Journal of Life Cycle Assessment 24:1222–38.
- De Sadeleer, I., H. Brattebø, and P. Callewaert. 2020. Waste prevention, energy recovery or recycling - Directions for household food waste management in light of circular economy policy. Resources, Conservation and Recycling 160:104908.
- Delgado-Cabeza, M., C. Márquez-Guerrero, D. Coq-Huelva, D. Pérez-Neira, C. Rodríguez-Morillas, A. Sanz-Alduán, P. Vega-Pindado, and M. Mateos-Arribas. 2015. Las cuentas integradas del transporte en Andalucía. Dirección técnica de la Consejería de Fomento y Vivienda, Junta de Andalucía.
- Dumont, A. M., P. Gasselin, and P. V. Baret. 2020. Transitions in agriculture: Three frameworks highlighting coexistence between a new agroecological configuration and an old, organic and conventional configuration of vegetable production in Wallonia (Belgium). Geoforum 108:98–109.
- Esteve-Llorens, X., M. T. Moreira, G. Feijoo, and S. González-García. 2019. Linking environmental sustainability and nutritional quality of the Atlantic diet recommendations and real consumption habits in Galicia (NW Spain). Science of the Total Environment 683:71–79.
- FAO. 2020. Faostat. Online: http://www.fao.org/faostat/en/#home.
- Ferguson, B. G., M. Aldasoro-Maya, O. Giraldo, M. Mier y Terán Giménez-Cacho, H. Morales, and P. Rosset. 2019. Special issue editorial: What do we mean by agroecological scaling? Agroecology and Sustainable Food Systems 43 (7–8):722–23.
- Filho, W. L., A. Skouloudis, A. L. Brandli, A. L. Salvia, L. Veiga Avil, and L. Rayman-Bacchus. 2019. Sustainability and procurement practices in higher education institutions: Barriers and drivers. Journal of Cleaner Production 231:1267–80.
- Filippini, R., I. De Noni, S. Corsi, R. Spigarolo, and E. Bocchi. 2018. Sustainable school food procurement: What factors do affect the introduction and the increase of organic food? Food Policy 76:109–19.
- Francis, C., G. Lieblein, S. Gliessman, A. T. Breland, N. Creamer, R. Harwood, L. Salomonsson, J. Helenius, D. Rickerl, S. Salvador, et al. 2003. Agroecology: The ecology of food systems. Journal of Sustainable Agriculture 22 (3):99–118.
- Gaddis, J., and A. K. Coplen. 2018. Reorganizing school lunch for a more just and sustainable food system in the US. Feminist Economics 24 (3):89–112.
- Galli, F., G. Brunori, F. D. Iacovo, and S. Innocenti. 2014. Co-producing sustainability: Involving parents and civil society in the governance of school meal services. A case study from Pisa, Italy. Sustainability 6:1643–66.
- Giacomo, M. R., F. Testa, F. Iraldo, and M. Formentini. 2019. Does green public procurement lead to life cycle costing (LCC) adoption? Journal of Purchasing and Supply Management 25:100500.
- Giraldo, F. O., and P. Rosset. 2017. Agroecology as a territory in dispute: Between institutionalist and social movements. The Journal of Peasant Studies 45 (3):545–64.
- Gliessman, S. R. 2014. Introduction. Agroecology: A global movement for food security and sovereignty. In FAO (ed). Agroecology for food security and nutrition proceedings of the FAO International Symposium 18–19 September 2014, Rome, Italy.
- Gliessman, S. R. 2015. Agroecology: The ecology of sustainable food systems, 371. Third ed. Taylor & Francis: New York.
- Gliessman, S. R. 2018. Scaling-out and scaling-up agroecology. Agroecology and Sustainable Food Systems 42 (8):841–42.
- Gliessman, S. R. 2019. Scaling agroecology through policy change. Agroecology and Sustainable Food Systems 43 (4):361–361.
- Gliessman, S. R. 2020. Transforming food and agriculture systems with agroecology. Agriculture and Human Values 37:547–48.
- Goggins, G., and H. Rau. 2016. Beyond calorie counting: Assessing the sustainability of food provided for public consumption. Journal of Cleaner Production 112:257–66.
- González de Molina, M. 2013. Agroecology and politics. How to get sustainability? About the necessity for a political agroecology. Agroecology and Sustainable Food Systems 37 (1):45–59.
- González-García, S., X. Esteve-Llorens, M. T. Moreira, and G. F. Feijoo. 2018. Carbon footprint and nutritional quality of different human dietary choices. Science of the Total Environment 644:77–94.
- Hallström, E., A. Carlsson-Kanyama, and P. Börjesson. 2015. Environmental impact of dietary change: A systematic review. Journal of Cleaner Production 91:1–11.
- Hospido, A., L. L. Milà i Canals, S. McLaren, M. Truninger, G. Edwards-Jones, and R. Clift. 2009. The role of seasonality in lettuce consumption: A case study of environmental and social aspects. International Journal of Life Cycle Assessment 14:381–91.
- Ilieva, R. T., and A. Hernández. 2018. Scaling-Up Sustainable Development Initiatives: A Comparative Case Study of Agri-Food System Innovations in Brazil, New York, and Senegal. Sustainability 10 (11):4057.
- Inci, H., B. Sogut, O. Gokdogan, G. Ayasan, and T. Sengul. 2016. Determining the energy usage efficiency and economic analysis of broiler chickens raised under organic conditions. Indian Journal of Animal Sciences 86 (11):1323–27.
- IPES Food. 2016. From uniformity to diversity: A paradigm shift from industrial agriculture to diversified agroecological systems. International Panel of Experts on Sustainable Food Systems. http://www.ipes-food.org/_img/upload/files/UniformityToDiversity_FULL.pdf).
- IPES Food. 2017. Unravelling the food–health nexus: Addressing practices, political economy, and power relations to build healthier food systems. The Global Alliance for the Future of Food and IPES-Food. http://www.ipes-food.org/_img/upload/files/Health_FullReport(1).pdf
- IPES Food. 2019. Towards a common food policy for the European Union. International Panel of Experts on Sustainable Food systems. http://www.ipes-food.org/_img/upload/files/CFP_FullReport.pdf
- Iribarren, D., I. Vázquez-Rowe, A. Hospido, M. T. Moreira, and G. Feijoo. 2010. Estimation of the carbon footprint of the Galician fishing activity (NW Spain). Science of the Total Environment 408:5284–94.
- ISO. 2006. Environmental management life cycle assessment principles and framework. Geneva: The International Organization for Standardization. ISO 14040.
- Jungbluth, N., R. Keller, and A. König. 2016. ONE TWO WE—life cycle management in canteens together with suppliers, customers and guests. International Journal of Life Cycle Assessment 21 (5):646–53.
- Kissinger, M., C. Sussmann, C. Dorward, and K. Mullinix. 2019. Local or global: A biophysical analysis of a regional food system. Renewable Agriculture and Food System 34 (6):523–33.
- Kleine, D., and M. Das Graças-Brightwell. 2015. Repoliticising and scaling-up ethical consumption: Lessons from public procurement for school meals in Brazil. Geoforum 67:135–47.
- Loiseau, E., M. Colin, A. Alaphilippe, G. Coste, and P. Roux. 2020. To what extent are short food supply chains (SFSCs) environmentally friendly? Application to French apple distribution using life cycle assessment. Journal of Cleaner Production 276:124166.
- López-García, D., V. García-García, S. Sampedro-Ortega, A. Pomar-León, G. Tendero-Acin, A. Sastre-Morató, and A. Correro-Humanes. 2020. Exploring the contradictions of scaling: Action plans for agroecological transition in metropolitan environments. Agroecology and Sustainable Food Systems 44 (4):467–89.
- Martínez, S., M. M. Delgado, R. Martínez-Marín, and S. Álvarez. 2020. Carbon footprint of school lunch menus adhering to the Spanish dietary guidelines. Carbon Management 11 (4):427–39.
- ME (Ministerio de Educación). 2016 Statistics. https://www.educacionyfp.gob.es/portada.html.
- Méndez, E., C. M. Bacon, and R. Cohen. 2013. Agroecology as a transdisciplinary. Participatory, and Action-oriented Approach. Agroecology and Sustainable Food Systems 37 (1):3–18.
- MEYFP – Ministerio de Educación y Formación Profesional. 2020. Resultados detallados de centros educativos de la estadística de las enseñanzas no universitarias del curso 2018-2019.
- Michos, M., A. P. Mamolos, G. C. Menexes, C. A. Tsatsarelis, V. Tsirakoglou, and K. Kalburtji. 2012. Energy inputs, outputs and greenhouse gas emissions in organic, integrated and conventional peach orchards. Ecological Indicators 13:22–28.
- Mier y Terán Giménez Cacho, M., O. F. Giraldo, M. Aldasoro, H. Morales, B. G. Ferguson, P. Rosset, A. Khadse, and C. Campos. 2018. Bringing agroecology to scale: Key drivers and emblematic cases. Agroecology and Sustainable Food Systems 42: 637–665.
- Moragues-Faus, A., R. Sonnino, and T. Marsden. 2017. Exploring European food system vulnerabilities: Towards integrated food security governance. Environmental Science & Policy 75:184–215.
- Moreiras, O., A. Carbajal, L. Cabrera, and C. Cuadrado. 2005. Tablas de composición de alimentos. Pirámide. Madrid, Ed.
- Mundler, P., and L. Rumpus. 2012. The energy efficiency of local food systems: A comparison between different modes of distribution. Food Policy 37:609–15.
- Muñoz Rico, A. 2018. Los comedores escolares en España: Del diagnóstico a las propuestas de mejora. Del Campo al Cole. https://www.observatoriodelainfancia.es/ficherosoia/documentos/5587_d_Informe-Comedores-Escolares.pdf
- Nemat, B., M. Razzaghi, K. Bolton, and K. Rousta. 2020. The potential of food packaging attributes to influence consumers’ decisions to sort waste. Sustainability 12:2234.
- Neto, B. 2020. Analysis of sustainability criteria from European public procurement schemes for foodservices. Science of the Total Environment 704:135300.
- Nuutila, J., H. Risku-Norja, and A. Arolaakso. 2019. Public kitchen menu substitutions increase organic share and school meal sustainability at equal cost. Organic Agriculture 9:117–26.
- Parsons, K., and C. Hawkes. 2018. Connecting food systems for co-benefits: How can food systems combine diet-related health with environmental and economic policy goals? European Observatory on Health Systems and Policies. Denmark: World Health Organization.
- Pérez-Neira, D., D. Copena, L. Armengot, and X. Simón. 2020b. Transportation can cancel out the ecological advantages of producing organic cacao: The carbon footprint of the globalized agrifood system of Ecuadorian chocolate. Journal of Environmental Management 276:111306.
- Pérez-Neira, D., and D. Grollmus-Venegas. 2018. Life-cycle energy assessment and carbon footprint of peri-urban horticulture. A comparative case study of local food systems in Spain. Landscape and Urban Planning 172:60–68.
- Pérez-Neira, D., P. Rodriguez-Fernandez, and C. Hidalgo-Gonzalez. 2020a. The greenhouse gas mitigation potential of university commuting: A case study of the University of Leon (Spain). Journal of Transport Geography 82:102550.
- Pérez-Neira, D., X. Simón Fernández, D. Copena Rodríguez, M. Soler Montiel, and M. Delgado Cabeza. 2016. Analysis of the transport of imported food in Spain and its contribution to global warming. Renewable Agriculture and Food Systems 31 (1):37–48.
- Pergola, M., M. D’Amico, G. Celano, A. M. Palese, A. Scuderi, G. Di Vita, G. Pappalardo, and P. Inglese. 2013. Sustainability evaluation of Sicily’s lemon and orange production: An energy, economic and environmental analysis. Journal of Environmental Management 128:674–82.
- Petersen, P., and L. Silveira. 2017. Agroecology, public policies and labor-driven intensification: Alternative development trajectories in the Brazilian semi-arid region. Sustainability 9 (4):535.
- Poore, J., and T. Nemecek. 2018. Reducing food’s environmental impacts through producers and consumers. Science 360:987–92.
- Poux, X., and P.-M. Aubert 2018. An agroecological Europe in 2050: Multifunctional agriculture for healthy eating. Findings from the Ten Years For Agroecology (TYFA) modelling exercise, Iddri-AScA, Study No. 09/18, Paris, France, 74 p.
- Powell, L. J., and H. Wittman. 2018. Farm to school in British Columbia: Mobilizing food literacy for food sovereignty. Agriculture and Huma Values 35:193–206.
- Rockström, J., B. Loken, M. Springmann, T. Lang, S. Vermeulen et al . 2019. Food in the Anthropocene: the EAT–Lancet Commission on healthy diets from sustainable food systems. The Lancet Commissions 393 (10170): 447–492.
- Roibás, L., A. Elbehri, and A. Hospido. 2016. Carbon footprint along the Ecuadorian banana supply chain: Methodological improvements and calculation tool. Journal of Cleaner Production 112 (2016):2441–51.
- Rosset, P. M., and M. Altieri. 2017. Agroecology: Science and politics. Fernwood Publishing.
- Scherhaufer, S., M. Moates, H. Hartikainen, K. Waldron, and G. Obersteiner. 2018. Environmental impacts of food waste in Europe. Waste Management 77:98–113.
- Sevilla-Guzmán, E., and M. Soler-Montiel. 2010. Agroecología y soberanía alimentaria: Alternativas a la globalización alimentaria. Instituto de Patrimonio Histórico 311:258–83.
- Simón, X., M. Montero, and O. Bermúdez. 2020. Advancing food security through agroecological technologies: The implementation of the biointensive method in the Dry Corridor of Nicaragua. Sustainability 12:844.
- Soares, P., P. Caballero, and M. C. Davó-Blanes. 2017. Compra de alimentos de proximidad en los comedores escolares de Andalucía, Canarias y Principado de Asturias. Gaceta Sanitaria 32:446–52.
- SOCLA. 2015. Agroecology: Key concepts, principles and practices. Malaysia: Third World Network.
- Sönnichsen, S. D., and J. Clement. 2020. Review of green and sustainable public procurement: Towards circular public procurement. Journal of Cleaner Production 245:118901.
- Springmann, M., H. C. J. Godfray, M. Rayner, and P. Scarborough. 2016. Analysis and valuation of the health and climate change co-benefits of dietary change. PNAS 113:4146–51.
- Swensson, L. F. J., and F. Tartanac. 2020. Public food procurement for sustainable diets and food systems: The role of the regulatory framework. Global Food Security 25:100366.
- Taylor, R. C., R. Omed, and G. Edwards-Jones. 2014. The greenhouse emissions footprint of free-range eggs. Poultry Science 91 (1):231–37.
- Tilman, D., and M. Clark. 2014. Global diets link environmental sustainability and human health. Nature 515:518–32.
- Tittonell, P. 2015. Food security and ecosystem services in a changing world: It is time for Agroecology. In FAO (ed): Agroecology for food security and nutrition. Proceedings of the FAO International Symposium, Roma.
- Treu, H., M. Nordborg, C. Cederberg, T. Heuer, E. Claupein, E. Hoffmann, and G. Berndes. 2017. Carbon footprints and land use of conventional and organic diets in Germany. Journal of Cleaner Production 161:127–42.
- UN (United Nations). 2018. Sustainable Development Goal 2. https://sdgs.un.org/goals/goal2
- USDA 2017. Choose my plate. Retrieved from United States Department of Agriculture. www.choosemyplate.gov/ (06-12-2020).
- Vaarst, M., A. Getz-Escudero, M. J. Chappell, C. Brinkley, R. Nijbroek, N. Arraes, L. Andreasen, A. Gattinger, G. Fonseca, D. Bossio, et al. 2018. Exploring the concept of agroecological food systems in a city-region context. Agroecology and Sustainable Food Systems 42:686–711.
- Vitali, A., G. Grossi, G. Martino, U. Bernabucci, A. Nardone, and N. Lacetera. 2018. Carbon footprint of organic beef meat from farm to fork: A case study of short supply chain. Journal of the Science of Food and Agriculture 98 (14):5518–24.
- VSF (Veterinarios Sin Fronteras) 2013. Compra pública en sistemas alimentarios locales: Impactos sociales, ambientales y económicos. https://d3cra5ec8gdi8w.cloudfront.net/uploads/documentos/documentos_informecomprapblica_d9a40ea8.pdf.
- Westhoek, H., J. P. Lesschen, T. Rood, S. Wagner, A. De Marco, D. Murphy-Bokern, A. Leip, H. Van Grinsven, M. A. Sutton, and O. Oenema. 2014. Food choices, health and environment: Effects of cutting Europe’s meat and dairy intake. Global Environmental Change 26:196–205.
- WHO. 2013. State of the science of endocrine disrupting chemicals 2012. Geneva, Switzerland: United Nations Environment Programme and the World Health Organization.
- Wickramasinghe, K., M. Rayner, M. Goldacre, N. Townsend, and P. Scarborough. 2016. Contribution of healthy and unhealthy primary school meals to greenhouse gas emissions in England: Linking nutritional data and greenhouse gas emission data of diets. European Journal of Clinical Nutrition 70:1162–67.
- Wickramasinghe, K., M. Rayner, M. Goldacre, N. Townsend, and P. Scarborough. 2017. Environmental and nutrition impact of achieving new School Food Plan recommendations in the primary school meals sector in England. BMJ Open 7.
- Wigboldus, S., L. Klerkx, C. Leeuwis, M. Schut, S. Muilerman, and H. Jochemsen. 2016. Systemic perspectives on scaling agricultural innovations. A Review. Agronomy for Sustainable Development 36:46.
- Wirsenius, S., F. Hedenus, and C. Mohlin. 2011. Greenhouse gas taxes on animal food products: Rationale, tax scheme and climate mitigation effects. Climatic Change 108:159–84.