ABSTRACT
A sustainably intensified (SI) organic vegetable rotation, employing plant-based fertilizers, more crops, reduced tillage, and cover crops was compared to common practice (CP) where plants were fertilized with animal manure, typically one crop was grown per season, soil was plowed and often left bare over winter. Second and third-year results are presented. Nitrogen (N) input obtained within the rotation from N2 fixed by legume cover crops was higher under SI (34% potential self-sufficiency) than CP (5%). Marketable yields of cabbage, celeriac, leek, lettuce, and onion were similar, and aboveground dry matter increased by 16% under SI (8.6 Mg ha−1) compared to CP (7.5 Mg ha−1). Nitrogen use efficiency (N output/ N input) was 8–16% higher under SI compared to CP, mainly due to the full year clover. Nitrogen surface balance (N input – N output) was higher for SI compared to CP, indicating increased N leaching risk under SI. Short season and shallow-rooted crops under SI left more mineral N to 2.5 m depth in autumn than deeper-rooted crops under CP. Cover crops indicated to mitigate N leaching risk. Vegetable production can be intensified sustainably using more yielding crops, cover crops, reduced tillage, and plant-based fertilizers.
Introduction
The 70%-yield gap between organic and conventional agriculture in northern Europe (de Ponti, Rijk, and van Ittersum Citation2012) indicates the need to improve and intensify organic production to meet global food demand. However, intensive agriculture leads to degraded soils and pollutes water resources when excess nutrients are leached to the environment (Drinkwater and Snapp Citation2007). Vegetable production, in particular, exhibits a high risk of nitrogen (N) leaching, due to high N inputs and the low N use efficiency (NUE) of many short-season vegetables (Tei et al. Citation2020). Yet, higher production without harmful impacts to the environment could still be obtained by employing sustainable intensification. This term defines a system where yields are increased without adverse environmental impacts and without cultivating more land (Pretty and Bharucha Citation2014). Possible methods to achieve sustainable intensification include increasing plant diversity in combination with various nutrient sources that have longer mean residence times in the soil (Drinkwater and Snapp Citation2007), as well as using cover crops for recycling nutrients and increasing yield (West, Ruark, and Shelley Citation2020).
Plant diversity can be increased by growing two crop species per season. Intercropping (i.e. growing two crop species together at the same spatial scale), can increase yields without needing to increase inputs, thus facilitating sustainable intensification (Brooker et al. Citation2015). Relay intercropping is defined as planting a second crop before the first crop reaches maturity, while strip intercropping involves the simultaneous growth of two crop species in strips (Brooker et al. Citation2015). Double cropping (i.e. growing two crop species per season consecutively across time) provides an additional way to increase yields by exploiting an extended growing season.
The aim to phase out the use of animal manure from conventional origin in organic production creates the need for alternative fertilizer sources (Oelofse, Jensen, and Magid Citation2013). Legumes have the ability to fix atmospheric N2 and can, therefore, be used as plant-based fertilizers, which can be produced on-farm. However, growing legumes over a full year comes at the price of reduced yield, due to the extra space required for growth, unless they are grown for fodder. Consequently, these crops are often under-represented in the crop rotation of stockless organic farms, especially in countries that allow input of animal manure of conventional origin (Thorup-Kristensen, Dresboll, and Kristensen Citation2012). For example, in Danish organic vegetable production, it is common practice to rely heavily on external input of nutrients to cropping systems instead of recycling nutrients by cover crops. This does not align with the principle of ecology in organic farming (IFOAM, 2020). Therefore, managers of specialized organic vegetable farms must design cropping systems that have a higher share of N input via biological N2 fixation, as this approach fosters more balanced nutrient levels (Möller Citation2018).
If two crops are grown per season, more fertilizers might be required to meet crop nutrient demand, even though total application might be below that of crops grown separately. Thus, a strategy is required to reduce N leaching losses during winter. Cover crops are an effective tool for improving N management in agricultural systems, with crop species having different effects on soil N status. Legumes and non-legumes reduce N leaching by up to 40% and 70%, respectively, compared to bare soil (Tonitto, David, and Drinkwater Citation2006). Despite the reduced efficiency of legumes in depleting the soil of mineral N, they are beneficial in adding N to agricultural systems through biological N2 fixation, potentially helping to reduce fertilizer N inputs (e.g. Hefner et al. Citation2020). The inclusion of cover crops (legumes and non-legumes) as fertility-building crops in crop rotations appears to reduce N leaching losses more than rotations without cover crops (Thorup-Kristensen, Dresboll, and Kristensen Citation2012). Moreover, cover crops reduce weed pressure and facilitate additional N input via legumes, thereby maintaining yield levels when soil tillage intensity is reduced (Wittwer et al. Citation2017).
Thus, a more holistic approach is needed to achieve sustainable intensification, whereby crop rotation sequences, soil and crop management must be included (Dore et al. Citation2011). Reducing N leaching risk in parallel to maintaining vegetable yields could only be achieved when integrating several strategies for N management (Tei et al. Citation2020). Therefore, we applied a system’s approach, in which we compared different fertilizer sources, number of crops, cover crops and tillage practices in parallel to investigate the combined effects of these management strategies on the plant and soil system.
We hypothesized that self-sufficiency in terms of N input, yields, and NUE would be improved under a sustainable intensified (SI) system, where plant-based fertilizers, more crops per season, reduced tillage and winter cover crops are employed compared with common practice (CP), where animal slurry, typically one crop per season, plowing, and few cover crops are employed. Moreover, we hypothesized that N surface balance would be smaller under SI and that N leaching risk would not differ compared to CP.
Material and methods
Field site and experimental design
A field experiment was conducted at Aarhus University Aarslev, Denmark (55°18′N, 10°27′E) over 3 years (2017–2019). The soil was sandy loam (Typic Agrudalf), and the physical and chemical properties are shown in (). Average monthly temperature and cumulative monthly precipitation during the experimental period are shown in (). The field was managed according to the Danish organic farming regulation since 1996.
Table 1. Soil properties of the experimental site.
A split-plot randomized complete block study design with three replicates (blocks) was implemented, with cropping system as the whole-plot factor and field as the sub-plot factor. Several aspects were changed between the two cropping systems being compared, making this a system’s approach instead of the more commonly used factorial set-up. The two cropping systems differed with respect to soil tillage, fertilizer type, crop species, number of crops per season and cover crops (). Cover crops were not included (except for the cabbage stubble) and fertilization was mainly import based under CP, as is common practice for organic vegetable farms in Denmark (see also O1 system in Thorup-Kristensen, Dresboll, and Kristensen Citation2012). The sub-plot factor consisted of five fields: 1) clover (CLO), 2) cabbage (CAB), 3) celeriac (CEL), 4) leek (LEE), and 5) lettuce (LET). These crops were grown at a spatial (fields) and temporal (crop rotation) scale, which meant that a total of 30 plots were managed each year (2 cropping systems × 5 fields × 3 blocks). Sub-plots were 8 × 10 m2 in size. Vegetable crops were planted at a row distance of 0.5 m. The crops and cover crops grown in each field and system are presented in ().
Table 2. Different management practices and schematic overview of crops grown in the five fields of the sustainably intensified (SI) and common practise (CP) cropping systems.
Crop management
The timing of all agricultural operations, including fertilization, planting, weeding, harvesting and harrowing, is shown in (). Seedbeds were prepared with a rotary harrow (Howard, Kongskilde, Denmark) with 0.1-m working depth, and crops were transplanted with a three-row planting machine (Checchi & Magli Wolf, Italy). Lettuce was transplanted in two rows per bed as the second crop between the three rows of onion (first crop), with the same planting machine being adapted to two rows under SI-LET with 13–34 days of overlapping growing period all three years. In 2017, summer white cabbage and leek (second crops) were also transplanted in two rows per bed between pointed cabbage and lettuce under SI-CAB and SI-LEE, respectively, with 6–12 days of overlapping growing period. However, transplanting between the existing rows resulted in damage to cabbage and lettuce (first crops) under SI-CAB and SI-LEE, respectively; thus, the second crop was transplanted to three rows immediately after the first crop was harvested in 2018 and 2019. Crop residues were chopped with a flail mower (Spearhead, UK) and were incorporated in the soil with a cultivator (Kuhn, France) to 0.15-m depth. The plots were harrowed before establishing the second crop. A specialized in-row harrow (Breviglieri, Italy) was used to cultivate the inter-rows between the rows of the first crop under SI-LET (2017–2019) and under SI-CAB and SI-LEE (2017). Weeding was conducted with an inter-row cultivator (Rath Maschinen, Austria), a weed-brush machine (Rath Maschinen, Austria) and by manual hoeing.
Table 3. Dates of agricultural management under sustainable intensified (SI) and common practice (CP) cropping systems.
Table 4. Nitrogen input (kg ha−1), including N added through N2 fixation from legumes and with fertilizers, under sustainable intensified (SI) and common practice (CP) cropping systems.
Fertilizer type and the amount of N application in all fields and years is shown in . N2 fixed by clover was applied as a fertilizer by cutting SI-CLO four times in the growing season and applying one-half and one-third of the cuttings as fresh clover fertilizer in SI-LET during May 2018 and 2019, respectively, all cuttings in SI-CEL during July 2018/2019, and half of the cutting in SI-CEL during August 2019. Clover cuttings under SI-CLO were exported outside the rotation at the start of July and in August 2018, as they did not fit in the fertilization schedule. Considering all clover cuttings, SI reached a potential self-sufficiency of 34% in terms of fertilizer N input obtained within the crop rotation (of which 37% was applied directly). The other fresh clover applications in SI-CAB, SI-CEL and SI-LEE were imported to the rotation from a 300-m nearby organic red clover field and accounted for 46% (2018) and 31% (2019) of the total fertilizer N input. The C/N ratio of all applied clover cuttings was within a range of 10 to 20. Total N input was calculated as the sum of fertilizer N, N2 fixation from legumes and atmospheric N deposition. Total N input was generally higher under SI compared to CP () to supply sufficient N to a greater number of or increased diversity of crops grown under SI in CAB, LEE and LET fields. The lower amount of N applied under CP was justified by a lower N requirement of a smaller number of crops, which aligned with the Danish fertilization regulation (Landbrugsstyrelsen Citation2020).
Table 5. Clover biomass (dry matter in Mg ha−1), N accumulation in biomass and N2 fixation (kg ha−1) from four clover cuttings and a final cut before termination of clover in November in the CLO field under sustainably intensified (SI) and common practice (CP) cropping systems.
Table 6. Nitrogen (N) output (kg ha−1), N surface balance (kg ha−1), and N use efficiency (NUE) (%).
In 2017, all plots were irrigated with a lateral moving irrigation system with 133 mm water distributed over 7 dates from 7 April to12 July. In 2018, crops were irrigated with 255 mm water distributed over 10 dates from 17 May to 7 August. Crops were not irrigated in 2019 due to sufficient precipitation. Pest control was conducted in CAB by covering cabbage with a net (2017, 2018 and 2019) and in CEL and LEE by applying a bio pesticide (DiPel; Bacillus thuringiensis) on 23 August 2019. The clover mixtures were incorporated by tillage in late November/early December to avoid preemptive competition with the subsequent crop. Winter cabbage stubble was left in the field after harvest to act as a cover crop (nitrogen catch crop) under CP. All CP plots were plowed to 0.2-m depth with a plow (Kverneland, Norway) at the start of December.
Soil and plant sampling
Soil samples were taken three times during the growing season: (1) before fertilization in spring (15 March 2017; 26–27 March and 10 April 2018; and 25–28 March 2019), (2) mid-season (12 June 2017; 10 July 2018; and 11 July 2019), and (3) at harvest (8–13 Nov 2017; 5–9 Nov 2018; and 25–29 Nov 2019). In spring and autumn, 10 sub-samples of soil were randomly taken from each subplot by a machine-driven soil piston auger with a 14-mm inner-diameter. The 0–0.5 m, 0.5–1.5 m and 1.5–2.5 m depth layers of soil were sampled with the auger, and were mixed into one composite sample per soil layer. At mid-season, nine soil sub-samples were taken with a hand-driven soil piston auger (15-mm inner diameter) in the 0–0.25 m depth layer, which was mixed to form one composite sample. Soil samples were frozen (−18°C) until analysis. For mineral N analysis, 100 g fresh weight sub-samples were taken after thawing and were immediately extracted in 1 M KCl for 1 h (1 soil: 2 solution). The soil extract was centrifuged, and the supernatant was subjected to NH4+ and NO3− analyses by standard colorimetric methods in an AutoAnalyzer 3 (Bran+Luebbe, Germany).
Clover was machine harvested (17 m2 per plot). An area of 1 m2, 5.12 m2, and 4.8 m2 was harvested by hand for barley/cover crops, celeriac, and all other crops, respectively. Yields were separated into marketable yield and crop residues, where applicable. Marketable yield was evaluated with respect to crop weight and damage by pests or disease according to the market standard. Plant material was chopped, mixed well, weighed, oven-dried at 80°C for 20 h, and weighed again to determine dry matter content. Total plant N content was analyzed by the combustion method according to the VDLUFA (Citation1991), wherein plant material was first combusted at 950°C, and molecular N was then measured by a LECO TruSpec CN (CP. Joseph, MI). Total organic carbon content (C) was determined by Dumas’ dry combustion method, wherein plant material was combusted at 1000°C, and total organic carbon content was measured by an ELTRA Helios C/S-analyzer (Haan, Germany).
Data processing and statistical analysis
The results from the first experimental year (2017) were not included in the analyses because this was the establishment year, where management operations were optimized for double cropping, and the pre-crop was the same in all fields.
Crop yields were determined as fresh weight per area. Sales units were registered according to local market standards as the number of individual crops (cabbage, lettuce, and celeriac) or bunches of three leeks and five onions per area. Above ground N accumulation of plant material was calculated by multiplying total plant dry matter per area and N content. Soil mineral N was calculated per unit area from measured N concentrations in 0–0.5 m, 0.5–1.5 m and 1.5–2.5 m soil layers and the corresponding bulk densities, which were obtained by a previous study at the same site (Kristensen & Thorup-Kristensen, 2004).
In compliance with the OECD (Citation2001) model, N surface balance was calculated as the difference between N input (N added with fertilizer and by N2 fixation of legumes grown in the fields, as well as atmospheric N deposition) and N output (N removed from field in marketable products and all clover cuttings). An empirical model (Hogh-Jensen et al. Citation2004) was used to quantify N2 fixation by clover, vetch, and grass-clover:
N2 fixation = Nshoot * Pfix * (1+ Proot +Pimmobile+Ptrans soil)
where Nshoot is the amount of N in the shoot, which was calculated based on the plant sample dry matter and respective N content. Pfix is the proportion of fixed N of total shoot N, and was set to 0.74 for clover and vetch, and to 0.95 for grass-clover according to Hogh-Jensen et al. (Citation2004). Nitrogen fixed in the shoot was then corrected with N parameters in the root (Proot), N immobilized in soil in partly decomposed organic matter (Pimmobile), and N transferred to other species via soil (Ptrans soil); specifically Proot was set to 0.25, Pimmobile was set to 0.3 for clover and vetch and 0.38 for grass-clover, and Ptrans soil was set to 0.1. Of note, Ptrans soil was only considered in the grass-clover mixture (Hogh-Jensen et al. Citation2004). Nitrogen fixation was added as input from all cuttings in the CLO field and from vetch before incorporation in the CEL field. In 2018, data on grass-clover biomass, and consequently N2 fixation, is missing. Atmospheric N deposition was set to 12 kg N ha−1 yr−1, according to Ellermann et al. (Citation2015). Nitrogen accumulation of all other cover crops was not included, due to low cover crop biomass. Nitrogen use efficiency (NUE) was calculated as the ratio between N output and N input.
Results of crop yield, sales units, above ground biomass and N accumulation were analyzed for each field separately across years. A Gaussian linear mixed model was used containing two fixed effects (cropping system and year), an interaction between these effects, and a random component representing block within year to account for spatial variation between blocks each year. Soil mineral N results were analyzed for each soil layer and year separately. Nitrogen balance and NUE were analyzed for years separately. A Gaussian linear mixed model was set up with two fixed effects (cropping system and field), an interaction between these effects, and a random component accounting for the split-plot design (representing the block and the whole-plot). Data were logarithmically transformed when assumptions of homogeneity of variance and normal distribution of residuals were not met.
The statistical analyses were performed in R software, version 3.4.2 (R Core Team, 2017). The mixed models were defined with the R-package lme4 (Bates et al., 2015). Nested models (e.g., a large model containing the interaction and a reduced model containing additive main effects) were compared with likelihood ratio tests for generalized linear mixed models implemented in the ‘anova()’ R-function to detect significant interactions between fixed factors. Post-hoc analyses were conducted using the correction for multiple comparisons based on the method of control of False Discovery Rate (FDR, see Benjamini and Yekutieli, 2001) with the R-package ‘pairwiseComparisons.’ Mean values are reported followed by standard errors
Results
Climate
Cumulative precipitation was lower in 2018 (531 mm) compared to 2019 (767 mm), with particularly low values in May–July 2018 (). Average daily temperature in April–October was 14.9°C in 2018 and 13.4°C in 2019.
Clover productivity and N2 fixation
Clover biomass was higher when full-year clover was cut four times per season under SI (15 Mg ha−1 dry weight) in contrast to under-sown grass-clover under CP (1 Mg ha−1 dry weight) (). Likewise, N2 fixation was higher under SI (300 kg N ha−1 yr−1) compared to CP (33 kg N ha−1 yr−1). The sum of N input in CAB, CEL, LEE and LET fields was 863 and 860 kg N ha−1 in 2018 and 2019 (). Averaged for both years, 34% of this N input was obtained by N2 fixation of full year clover under SI. However, only 107 kg N ha−1 (averaged for both years; accounting for 37% of N2 fixed by all clover cuttings in CLO) was applied within SI, due to poor timing of N availability and crop demand. Consequently, fertilization was supplemented with clover from a nearby field. Self-sufficiency, in terms of N input by N2 fixation from clover cuttings applied within the crop rotation, was 12%, and increased to 19% when including the incorporated legume cover crops in the CLO and CEL fields. In CP, only 5% of the 635 kg N ha−1 N input was supplied by N2 fixation of under-sown grass-clover in 2019.
Crop yield, sales units and N accumulation
Above ground dry matter biomass averaged across all fields increased by 16% under SI (8.6 Mg ha−1) when compared with CP (7.5 Mg ha−1). In particular, the above ground biomass of CLO, LEE and LET (2018/2019) increased under SI compared with CP; however, the aboveground biomass of CAB (2018/2019) and CEL (2019) decreased ().
Figure 2. Above ground biomass (dry matter; left column) and N accumulation (right column) of five crop fields across two years. SI = sustainable intensified system, CP = common practice. CLO = clover (SI) and spring barley under-sown with clover (CP); CAB: pointed cabbage followed by white cabbage (SI) and white cabbage (CP); CEL: celeriac (SI and CP); LEE: lettuce followed by leek (SI) and leek (CP); LET: green onion intercropped with lettuce (SI) and lettuce followed by lettuce (CP). Bars indicate standard error (n = 3). Different lower-case letters indicate significant differences between cropping systems for each field separately (P < .05).
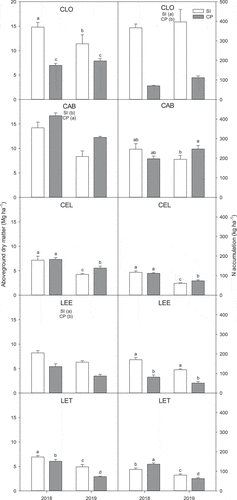
Marketable yields averaged across all four vegetable fields (CAB, CEL, LEE, LET) were maintained under SI when compared with CP. Marketable yields of LEE (2018/2019) and LET (2019) were 157–191% and 34% higher, respectively, under SI compared to CP. However, the marketable yield of CEL (2019) was 30% lower under SI compared to CP (). In all other cases, marketable yields were comparable between systems.
Figure 3. Marketable yield (left column) and sales units (right column) of four fields over two years. SI = sustainable intensified system, CP = common practice. CAB: pointed cabbage followed by summer white cabbage (SI) and autumn white cabbage (CP); CEL: celeriac (SI and CP); LEE: lettuce followed by leek (SI) and leek (CP); LET: green onion intercropped with lettuce (SI) and lettuce followed by lettuce (CP). Bars indicate standard error (n = 3). Different lower-case letters indicate significant differences between cropping systems for each field separately (P < .05).
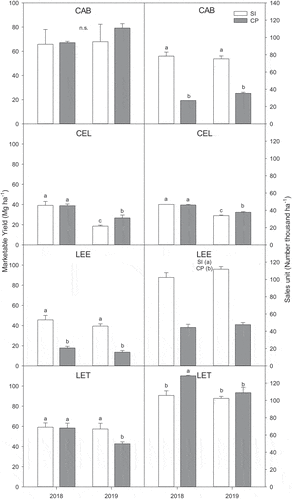
Sales units averaged across fields (excluding CLO) increased under SI compared to CP. In CAB and LEE fields, two crops were grown per season under SI in contrast to one crop under CP, increasing sales units under SI (). Sales units of CEL (2018/2019) and LET (2019) were comparable between systems, whereas sales units of LET (2018) were lower under SI (bunches of five onions followed by lettuce) compared to CP (lettuce followed by lettuce).
Above ground N accumulation averaged across all fields was 63% higher under SI (168 kg N ha−1) compared to CP (114 kg N ha−1). In particular, N accumulation of CLO (2018/2019), LEE (2018/2019), and LET (2019) increased under SI. In contrast, N accumulation of CEL (2019) and LET (2018) decreased under SI by 19% and 17%, respectively ().
Fewer plant residues remained in the field under SI (2.8 and 1.6 Mg ha−1) compared to CP (3.4 and 1.9 Mg ha−1) in 2018 and 2019, respectively, mainly due to lower cabbage and onion/lettuce residues (results not shown). Nitrogen accumulation in residues was equal between systems in 2018 (53 kg N ha−1), but was lower under SI (37 kg N ha−1) compared to CP (48 kg N ha−1) in 2019 (results not shown).
Nitrogen input, output, surface balance and use efficiency
Nitrogen input was 77–79 kg N ha−1, 142–144 kg N ha−1, and 43–62 kg N ha−1 higher under SI compared to CP in CAB, LEE and LET fields, respectively (). Higher N input was paralleled by higher organic matter input, as plant-based fertilizers were applied. In 2018, SI-CAB had higher N output compared to CP-CAB; however, this difference was not observed in 2019 (). In contrast, N surface balance (N input – N output) for CAB did not differ between SI and CP in 2018, but was higher under SI in 2019. In LEE, N output and N surface balance were higher under SI compared to CP in both years. Nitrogen output and NUE (N output per N input) were highest for SI-CLO compared to all other fields and cropping systems, whereas N surface balance (N input – N output) was lowest ().
Among fields, NUE was generally lower for LEE and LET (16%–38%), intermediate for CAB (45–77%) in both years and CEL in 2018 (41–42%), and highest for SI-CLO (120–127%) in both years (). Nitrogen use efficiency was higher under SI compared to CP for CLO in both years, lower under SI compared to CP for CAB in 2019, but comparable between cropping systems in all other fields and years. Mean NUE across fields was 8–16% higher under SI compared to CP ().
Soil mineral N
Growing clover over winter under SI-CLO reduced soil mineral N content at 1.5–2.5 m soil depth in the spring of 2018 and at 0.5–1.5 m soil depth in the spring of 2019 compared with bare soil under CP-CLO (). In contrast, soil mineral N was higher following two short-season cabbages under SI compared to one long-season cabbage under CP in CAB at 0–1.5 m soil depth in autumn 2018 and at 1.5–2.5 m soil depth in autumn 2019 (). Soil mineral N content was similar between systems in LEE fields (supplementary material), but differences appeared in the following spring under LET, where soil mineral N content was higher under CP compared to SI at 1.5–2.5 m depth in 2018 and 0.5–2.5 m depth in 2019 (). In contrast, higher soil mineral N was recorded in autumn in LET under SI compared to CP at 0.5–1.5 m soil depth (2018/2019) ().
Figure 4. Soil mineral N in three soil layers (0–0.5 m, 0.5–1.5 m, 1.5–2.5 m) of selected fields in the spring and autumn of 2018 and 2019. SI = sustainable intensified system, CP = common practice. Bars indicate standard error (n = 3). * indicates significant differences for sampling time and soil depth separately (P < .05). Soil mineral N of all fields is provided as supplementary material (S1).
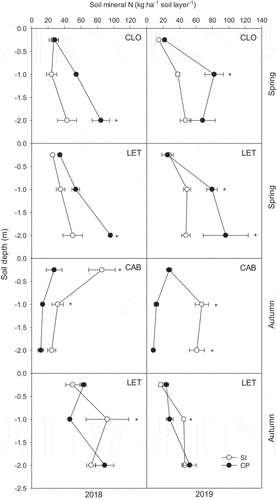
Discussion
Nitrogen input and self-sufficiency
Despite N input being higher under SI, 34% of N input within the crop rotation was obtained from N2 fixed by clover and legume cover crops (of which just 37% was applied). In contrast, CP mainly depended on external N input from animal manure (). Nitrogen accumulated by red and white clover under SI (366–397 kg N ha−1) was slightly lower than the N accumulated by pure red clover (500 kg N ha−1) or white clover (400 kg N ha−1) cut four times in a previous study at the same site (Sorensen and Grevsen Citation2016). N2 fixed under CLO (277–300 kg N ha−1 yr−1) that was cut and removed four times during the growing season was slightly lower than the N2 fixed by a mixture of perennial ryegrass and red and white clover (336–376 kg N ha−1 yr−1) cut three times and left on the soil in another study conducted in Denmark (Pandey et al. Citation2017). The lower N2 fixation of full year clover documented in our study could be explained by lower N2 fixation of legumes grown in pure stands compared to legumes grown in mixtures with non-legumes, because the competition for soil mineral N in mixtures stimulates the N2 fixation of legumes (Nyfeler et al. Citation2011).
Nitrogen input was generally higher in SI compared to CP (), because a greater number or variety of crops in CAB, LEE and LET required higher N input. Nitrogen input also increased under SI, because the N transfer rate from fertilizer to crops is lower for leguminous green manure crops (0.6) compared to liquid animal manure (0.8) (Benke et al. Citation2017), justifying the need for a higher N application rate of plant-based fertilizers under SI.
Thirty-four percent of the total N input was obtained through N2 fixation by clover under SI (). Only 37% of this N (accounting for 12% of total N input) was applied within the crop rotation, due to the poor timing of N availability in relation to N demand. However, the potential of storing this N source via ensiling could facilitate N application at times when crop demand is high, as proposed by Möller (Citation2018). The redistribution of N from one year to the next via ensiling increased N accumulation in the cropping system, as N can be applied when crop need is high (Raberg, Carlsson, and Jensen Citation2018). We showed that replacing one-fifth of the crop rotation with full year clover under SI potentially decreased N import from external sources by one-third if unused cuttings are preserved as silage. Therefore, we supported our hypothesis that self-sufficiency, in terms of N input, increased to a greater extend under SI (34%) compared to CP (5%). Similarly, the strategic use of fertility-building crops, such as legumes, in a crop rotation reduced the dependence on imported nutrients (Thorup-Kristensen, Dresboll, and Kristensen Citation2012).
The self-sufficiency of the SI system could have been improved further by including more legumes in the crop rotation as cover crops or intercrops. Iannetta et al. (Citation2016) showed that if legume crops are grown for half the year of a crop rotation, N input through N2 fixation is maximized, reducing the need for external N input. Moreover, using leguminous green manure contributes to a more balanced overall input of nutrients by importing just N without any other elements (Möller Citation2018). However, increased risks of rotational diseases in legumes need to be taken into account.
Yield response to cropping system
We hypothesized that above ground biomass and marketable yield would be higher under SI compared to CP, due to double- and intercropping crops under SI. We confirmed that the overall above ground biomass under SI was 16% higher than that under CP. This phenomenon was partly attributed to the biological N2 fixation of full year clover grown in the CLO field, which provided an additional source of N input and biomass production. Full-year grass-clover adds considerable amounts of N to the system through N2 fixation (Pandey et al. Citation2017). Moreover, growing two crops instead of one crop in the LEE field increased above ground biomass by 52–82%. This rise was mainly attributed to the extended growing season (175 vs. 107 days) with a higher temperature sum (2668 °Cd vs. 1770 °Cd). It is particularly important to utilize temperature during the extended growing season in Nordic climates, where low temperatures limit plant growth. Average daily temperature from April to October was higher in 2018 (14.9°C) compared to 2019 (13.4°C), and positively affected vegetable yields. Furthermore, diversifying crops grown under LET also increased above ground biomass by 14–68% under SI, in which onion and lettuce were intercropped compared with double cropped lettuce under CP. The increased above ground biomass under LET was an effect of higher onion biomass (4–5 Mg ha−1) compared with lettuce biomass (1–3 Mg ha−1).
Although overall above ground biomass was higher under SI, overall marketable yield was similar between SI and CP, due to the absence of marketable yield under SI-CLO. Marketable yield under SI-LEE sufficiently increased to counterbalance the reduced marketable yield under SI-CLO. Thus, we partly supported our hypothesis, because SI increased above ground biomass and maintained marketable yield compared with CP. The ability to maintain overall marketable yield under SI despite the introduction of a full year clover in the crop rotation showed the potential of this system for application in practice where full year legumes are often replaced by part year legumes in vegetable crop rotations (Thorup-Kristensen, Dresboll, and Kristensen Citation2012). In addition, overall increased sales units, in particular in CAB and LEE, demonstrated the advantage of the SI system. Similarly, marketable yields of an organic crop rotation based on fertility-building crops with reduced import of animal manure were similar to that obtained for organic rotation based on the common practice of using animal manure and no cover crops in another study conducted at the same location (Thorup-Kristensen, Dresboll, and Kristensen Citation2012).
Interestingly, the marketable yield of CAB was similar between cropping systems, regardless of whether two short-season cabbage crops or one long-season cabbage crop were grown. However, above ground biomass declined by 15–32% and N accumulation by 25% under SI in 2019. This finding indicates that double cropping two crops incurs slower nutrient uptake during the early growing phase, which contrasted with higher biomass production and nutrient uptake of a long-season crop under CP. Likewise, deep roots of a long-season white cabbage increased access to soil mineral N in deep layers (Kristensen and Thorup-Kristensen Citation2007), explaining the higher N accumulation of CAB under CP found in our study. Reduced celeriac yield under SI-CEL compared to CP-CEL in 2019 was attributed to delayed plant development due to delayed transplanting under SI by 14 days caused by high precipitation, which prevented machinery traffic. In 2018, transplanting conditions and yields were similar.
Nitrogen dynamics
Nitrogen use efficiency was comparable between systems for all fields, except CLO 2018/2019 and SI-CAB 2019 (). This finding indicated that the generally higher N outputs (obtained for CAB 2018, LEE 2018/2019, and LET 2019) justified higher N inputs under SI (). When including CLO in the average NUE across all fields, NUE was higher under SI compared to CP (). Full -ear clover improved the NUE of the whole crop rotation under SI, because of high N output (366–376 kg N ha−1, ) per N input via N2 fixation (300–357 kg N ha−1, ). The overall higher NUE under SI showed that the intensification of organic vegetable production could be achieved sustainably, when full year clover is included in the crop rotation. We confirmed part of our hypothesis that SI improved NUE due to higher N outputs compared with CP.
A generally higher N surface balance under SI than CP was found for LET 2019, CAB 2018, CEL 2019, and LEE 2018/2019 (Table 8). This phenomenon was attributed to higher N inputs under SI (), which was necessary to supply the greater number of crops grown under SI. Average N surface balance across fields was also higher under SI than CP, which was explained by the high N input through N2 fixation under SI-CLO (). Similarly, De Notaris et al. (Citation2018) found that crop rotations including full year green manure had the highest N surplus, which was attributed to the N2 fixation of legumes. Thus, we rejected our hypothesis that N surface balance would be lower under SI compared to CP.
Excess N under SI presents a risk for higher N losses through denitrification, volatilization, and leaching compared with CP, and requires the implementation of measures to reduce N losses. Tei et al. (Citation2020) suggested well-designed crop rotations, growing cover crops and employing inter-cropping as useful management tools to reduce N losses. The calculation of N surface balance in our study did not include the contribution of cover crops grown over winter, because they were considered to recycle N internally. Including cover crops in crop rotations was effective to reduce N leaching (Hefner et al. Citation2020; Thorup-Kristensen, Dresboll, and Kristensen Citation2012). Thus, N taken up by cover crops during autumn might have compensated for the higher N surface balance under SI, since cover crops were only grown under SI but not CP. However, growing two vegetable crops per season requires early sowing in spring and late harvesting in autumn, which might not coincide with timely cover crop management and efficiency of cover crops to reduce N leaching risk. Dabney, Delgado, and Reeves (Citation2001) showed that biomass and N accumulation of cover crops declined with delayed sowing date.
Nitrogen leaching risk
Vegetable residues present a high risk of N leaching because of the high quantities of N in their residues and low C/N ratios (Congreves and Eerd Citation2015; De Neve et al. Citation2000). The amount of crop residues (mainly cabbage and onion/lettuce) left in the field at harvest was smaller under SI compared to CP in both years, with less N accumulated in residues during 2019. This phenomenon indicated a smaller risk of N leaching from vegetable residues over the winter under SI.
However, increased risk of N leaching under SI was detected under CAB (), in which two short-season cabbages were grown compared with one long-season cabbage under CP. The ca. 78 kg ha−1 higher fertilizer N application under SI compared to CP () contributed to the increased risk of N leaching. Moreover, the deeper and more extensive root system of long-season cabbage, reaching 2.4 m depth (Kristensen and Thorup-Kristensen Citation2007) under CP might have improved soil N uptake, especially from deeper soil layers, potentially reducing the risk of N leaching. This phenomenon was detected in a previous study in Denmark where deep rooted white cabbage (2 m) was better at reducing soil mineral N in soil layers of 1–2.5 m depth by more than 100 kg N ha−1 compared with shallow rooted leek (0.5 m) (Thorup-Kristensen Citation2006). Similarly, N leaching risk was higher under SI compared to CP in LET, probably due to 24–63 kg ha−1 higher N application and more shallow onion roots compared to lettuce roots. Still, the inclusion of hairy vetch/black oat and red clover/white clover cover crops in SI probably were effective tools to reduce N leaching risk as indicated by lower soil mineral N in spring in CLO-SI. Tonitto, David, and Drinkwater (Citation2006) also showed that legumes can reduce N leaching risk by 40% compared with bare soil.
From a crop rotation perspective, focus is required on the low NUEs of LEE and LET (16%–38%), as they present a particularly high risk for N leaching during winter, whereas the NUEs of CAB and CLO were higher (). Interestingly, soil mineral N content in autumn was similar between SI and CP under LEE, but was lower in deeper soil layers under SI under LET the following spring (). This result indicates the positive effect of reducing N leaching by growing lettuce before leek, and of using winter rye cover crops after the leek harvest. In particular, Kristensen and Thorup-Kristensen (Citation2007) suggested that knowledge about differences in root growth between species is required to design crop rotations with high NUEs.
Nitrogen output included N removed in harvested products and legume cuttings. Nitrogen surface balance was calculated as the difference between N input (N in fertilizer + N2 fixed by legumes () + atmospheric N deposition) and N output. NUE was calculated as the percentage of N output per N input. SI = sustainably intensified system, CP = common practice. Mean values are presented with standard error (n = 3). Different superscript letters indicate significant differences for years separately (P < .05).
Growing clover over winter under SI reduced the risk of N leaching compared with bare soil under CP in CLO. This phenomenon was evidenced by the lower soil mineral N content the following spring (). Reduced N leaching losses in cover-cropped systems are mainly the effect of increased root exploitation by cover crops in contrast to bare soil, which improve N retention (Thorup-Kristensen, Dresboll, and Kristensen Citation2012).
Our findings support our hypothesis that N leaching risk was comparable between systems, because N taken up by cover crops grown over winter compensated for higher N surplus under SI. This phenomenon was recorded in all fields, except for CAB and LET, possibly due to the shallower root depth of short-season cabbage and onion compared with long-season cabbage and lettuce under CP. Therefore, the crop rotation of the SI system could be improved by incorporating knowledge about differences in root growth between species, cropping duration and the earlier establishment of cover crops (e.g. by under-sowing).
Conclusion
Designing a cropping system that has an increased number of crops, using plant-based fertilizers, cover crops, and reducing tillage facilitated the sustainable intensification of organic vegetable production. Even though N input was higher under SI compared to CP, 19% of N input was obtained within the crop rotation from N2 fixed by clover and legume cover crops. Potentially 34% self-sufficiency could have been obtained if all clover cuttings had been applied. In contrast, CP mainly depended on external N input from animal manure. Above ground biomass was 16% higher under SI compared to CP, due to high clover production and growing a greater number of crops. Marketable yield was generally comparable between systems, despite the absence of marketable yield in full-year clover under SI. This was because the higher marketable yield of double cropped lettuce and leek under SI counterbalanced the yield disadvantage of full year clover. Nitrogen use efficiency was comparable between systems for all vegetable fields, and was, even, improved, when including full year clover, indicating an advantage of the SI system. However, N surface balance was higher for SI compared to CP, mainly due to higher N inputs under SI, which increased the risk of N leaching. In particular, N leaching risk was higher following two short-season cabbages under SI compared to long-season cabbage under CP, as well as onion under SI compared to lettuce under CP. This phenomenon might be attributed to lower N uptake from the deeper soil layers of shallower rooted crops under SI. But the inclusion of cover crops in the rotation indicated a reduced N leaching risk under SI. In conclusion, the SI system could be implemented to realize the sustainable intensification of vegetable production; however, focus is required on managing N surplus in the system, e.g. by including cover crops for N recycling.
Abbreviations
Supplemental Material
Download EPS Image (183 KB)Acknowledgments
This study was funded by Green Development and Demonstration Program (GUDP) under the Danish Ministry of Environment and Food (GUDP project #34009-16-1134) and the Organic RDD3 programme under the International Centre for Research in Organic Food Systems as part of the DoubleCrop project (2017-2020). The funding bodies did not influence the study design, data analysis and writing of the report. We thank Lasse Vesterholt, Knud Erik Pedersen, Astrid Bergmann, Jens Elkjær, Jens Barfod, Asger Jarle Olsen, and Simon Gabriel Cazalis Fenger for their invaluable work in conducting the field management.
Disclosure statement
A potential competing interest exists, as Richard de Visser is employed as an advisor at the company HortiAdvice A/S, where he advises organic farmers on how to improve vegetable production.
Supplementary material
Supplemental data for this article can be accessed online at https://doi.org/10.1080/21683565.2022.2104419
Additional information
Funding
References
- Benke, A. P., A. M. Rieps, I. Wollmann, I. Petrova, S. Zikeli, and K. Moller. 2017. Fertilizer value and nitrogen transfer efficiencies with clover-grass ley biomass based fertilizers. Nutrient Cycling in Agroecosystems 107 (3):395–411. doi:10.1007/s10705-017-9844-z.
- Brooker, R. W., A. E. Bennett, W. F. Cong, T. J. Daniell, T. S. George, P. D. Hallett, C. Hawes, P. P. M. Iannetta, H. G. Jones, A. J. Karley, et al. 2015. Improving intercropping: A synthesis of research in agronomy, plant physiology and ecology. New Phytology 206 (1):107–17. doi:10.1111/nph.13132.
- Congreves, K. A., and L. L. Eerd. 2015. Nitrogen cycling and management in intensive horticultural systems. Nutrient Cycling in Agroecosystems 102 (3):299–318. doi:10.1007/s10705-015-9704-7.
- Dabney, S. M., J. A. Delgado, and D. W. Reeves. 2001. Using winter cover crops to improve soil and water quality. Communications in Soil Science and Plant Analysis 32 (7–8):1221–50. doi:10.1081/Css-100104110.
- De Neve, S., G. Hofman, A. P. Benke, A.-M. Rieps, I. Wollmann, I. Petrova, S. Zikeli, and K. Möller. 2000. Influence of soil compaction on carbon and nitrogen mineralization of soil organic matter and crop residues. Biology and Fertility of Soils 30 (5–6):544–49. doi:10.1007/s003740050034.
- De Notaris, C., J. Rasmussen, P. Sorensen, and J. E. Olesen. 2018. Nitrogen leaching: A crop rotation perspective on the effect of N surplus, field management and use of catch crops. Agriculture, Ecosystems and Environment 255:1–11. doi:10.1016/j.agee.2017.12.009.
- de Ponti, T., B. Rijk, and M. K. van Ittersum. 2012. The crop yield gap between organic and conventional agriculture. Agricultural Systems 108:1–9. doi:10.1016/j.agsy.2011.12.004.
- Dore, T., D. Makowski, E. Malezieux, N. Munier-Jolain, M. Tchamitchian, and P. Tittonell. 2011. Facing up to the paradigm of ecological intensification in agronomy: Revisiting methods, concepts and knowledge. European Journal of Agronomy 34 (4):197–210. doi:10.1016/j.eja.2011.02.006.
- Drinkwater, L. E., and S. S. Snapp. 2007. Nutrients in agroecosystems: Rethinking the management paradigm. In Advances in agronomy, ed. D. L. Sparks, Vol. 92, 163-+. doi:10.1016/s0065-2113(04)92003-2.
- Ellermann, T., R. Bossi, J. Christensen, P. Løfstrøm, C. Monies, L. Grundahl, and C. Geels. 2015. Atmosfærisk deposition 2013. Videnskabelig rapport fra DCE. DCE: Nationalt Center for Miljø og Energi. NOVANA. Aarhus Universitet.
- Hefner, M., M. T. Gebremikael, S. Canali, F. X. Sans Serra, K. K. Petersen, J. N. Sorensen, S. De Neve, R. Labouriau, and H. L. Kristensen. 2020. Cover crop composition mediates the constraints and benefits of roller-crimping and incorporation in organic white cabbage production. Agriculture, Ecosystems and Environment 296:106908. doi:10.1016/j.agee.2020.106908.
- Hogh-Jensen, H., R. Loges, F. V. Jorgensen, F. P. Vinther, and E. S. Jensen. 2004. An empirical model for quantification of symbiotic nitrogen fixation in grass-clover mixtures. Agricultural Systems 82 (2):181–94. doi:10.1016/j.agsy.2003.12.003.
- Iannetta, P. P. M., M. Young, J. Bachinger, G. Bergkvist, J. Doltra, R. J. Lopez-Bellido, M. Monti, V. A. Pappa, M. Reckling, C. F. E. Topp, et al. 2016. A comparative nitrogen balance and productivity analysis of legume and non-legume supported cropping systems: The potential role of biological nitrogen fixation. Frontiers in Plant Science 7. doi:10.3389/fpls.2016.01700.
- IFOAM Organics International, 2020. https://www.ifoam.bio/why-organic/shaping-agriculture/four-principles-organic (accessed July 27, 2021.
- Kristensen, H. L., and K. Thorup-Kristensen. 2007. Effects of vertical distribution of soil inorganic nitrogen on root growth and subsequent nitrogen uptake by field vegetable crops. Soil Use and Management 23 (4):338–47. doi:10.1111/j.1473-2743.2007.00105.x.
- Landbrugsstyrelsen, 2020. Danish ministry for food, fisheries and agriculture. Vejledning om gødsknings- og harmoniregler planperioden 1. August 2020 til 31. juli 2021 1. revision,september 2020. accessed July 15, 2021 https://lbst.dk/fileadmin/user_upload/NaturErhverv/Filer/Landbrug/Goedningsregnskab/Vejledning_om_goedsknings-_og_harmoniregler_2020_2021.pdf
- Möller, K. 2018. Soil fertility status and nutrient input–output flows of specialised organic cropping systems: A review. Nutrient Cycling in Agroecosystems 112 (2):147–64. doi:10.1007/s10705-018-9946-2.
- Nyfeler, D., O. Huguenin-Elie, S. Matthias, E. Frossard, and A. Luscher. 2011. Grass-legume mixtures can yield more nitrogen than legume pure stands due to mutual stimulation of nitrogen uptake from symbiotic and non-symbiotic sources. Agriculture, Ecosystems and Environment 140 (1–2):155–63. doi:10.1016/j.agee.2010.11.022.
- OECD. 2001. Environmental indicators for agriculture, methods and results. Paris, France: Organization for Economic Cooperation and Development.
- Oelofse, M., L. S. Jensen, and J. Magid. 2013. The implications of phasing out conventional nutrient supply in organic agriculture: Denmark as a case. Organic Agriculture 3 (1):41–55. doi:10.1007/s13165-013-0045-z.
- Pandey, A., F. C. Li, M. Askegaard, and J. E. Olesen. 2017. Biological nitrogen fixation in three long-term organic and conventional arable crop rotation experiments in Denmark. European Journal of Agronomy 90:87–95. doi:10.1016/j.eja.2017.07.009.
- Pretty, J., and Z. P. Bharucha. 2014. Sustainable intensification in agricultural systems. Annals of Botany 114 (8):1571–96. doi:10.1093/aob/mcu205.
- Raberg, T., G. Carlsson, and E. S. Jensen. 2018. Nitrogen balance in a stockless organic cropping system with different strategies for internal N cycling via residual biomass. Nutrient Cycling in Agroecosystems 112 (2):165–78. doi:10.1007/s10705-018-9935-5.
- Sorensen, J. N., and K. Grevsen. 2016. Strategies for cut-and-carry green manure production. Acta Horticulturae 1137:1139–45. doi:10.17660/ActaHortic.2016.1137.6.
- Tei, F., S. De Neve, J. de Haan, and H. L. Kristensen. 2020. Nitrogen management of vegetable crops. Agricultural Water Management 240:106316. doi:10.1016/j.agwat.2020.106316.
- Thorup-Kristensen, K. 2006. Effect of deep and shallow root systems on the dynamics of soil inorganic N during 3-year crop rotations. Plant and Soil 288 (1–2):233–48. doi:10.1007/s11104-006-9110-7.
- Thorup-Kristensen, K., D. B. Dresboll, and H. L. Kristensen. 2012. Crop yield, root growth, and nutrient dynamics in a conventional and three organic cropping systems with different levels of external inputs and N re-cycling through fertility building crops. European Journal of Agronomy 37 (1):66–82. doi:10.1016/j.eja.2011.11.004.
- Tonitto, C., M. B. David, and L. E. Drinkwater. 2006. Replacing bare fallows with cover crops in fertilizer-intensive cropping systems: A meta-analysis of crop yield and N dynamics. Agriculture, Ecosystems and Environment 112 (1):58–72. doi:10.1016/j.agee.2005.07.003.
- VDLUFA, 1991. Bestimmung von leicht löslichen (pflanzenverfügbaren) Haupt- und Spurennährstoffen. Die Untersuchung von Böden. Verband Deutscher Landwirtschaftlicher Untersuchungs- und Forschungsanstalten e.V. VDLUFA, Verlag, Darmstadt, Germany.
- West, J. R., M. D. Ruark, and K. B. Shelley. 2020. Sustainable intensification of corn silage cropping systems with winter rye. Agronomy for Sustainable Development 40. doi:10.1007/s13593-020-00615-6.
- Wittwer, R. A., B. Dorn, W. Jossi, and M. G. A. van der Heijden. 2017. Cover crops support ecological intensification of arable cropping systems. Scientific Reports 7 (1). doi: 10.1038/srep41911.