ABSTRACT
Transitioning to more sustainable agricultural practices is a key goal in agroecology. Before practices are adopted, however, farmers must weigh a complex set of biophysical and socioeconomic tradeoffs. Tarping is a weed control practice gaining popularity in New England, but many of its biophysical impacts remain unclear to farmers. Here, we used participatory action research to engage in mutual learning with farmers around the tradeoffs of tarping for weed control. We collected quantitative biophysical data with a field study and qualitative data on biophysical and socioeconomic factors by interviewing farmers. We found tarping has a number of benefits, challenges, and uncertainties, though most farmers had positive overall perceptions of the practice. Many of our biophysical results matched farmers’ experiences, including that tarping dramatically heated soils, suppressed weeds, and increased crop yields. However, our mixed results for the effects of tarping on soil nitrate contrasted farmers’ perception that tarping increases soil nitrate availability. Engaging in participatory and mixed methods research was an effective approach to unveil complex tradeoffs around tarping and ensure our research was relevant to farmer interests. Future research on long-term effects of tarps will be valuable to inform the sustainability of this practice.
Introduction
Increasing evidence shows that industrial agricultural practices are unsustainable and perpetuate injustice within social-ecological systems (Patel Citation2013; Tilman Citation1998). In response, many farmers, activists, and scientists have united around agroecology as a channel for fundamental shifts in our food systems. Originally defined as the application of ecological concepts and principles to the management of agricultural systems and landscapes (Altieri Citation1987; Vandermeer Citation1995; Wezel et al. Citation2009), some agroecologists have embraced a transdisciplinary approach that, in addition to including a range of academic disciplines (e.g. natural science, social science, and the humanities), also seeks to integrate different forms of knowledge (e.g. Indigenous, practical, etc.) (Mason et al. Citation2021; Méndez, Bacon, and Cohen Citation2013). While a major goal in agroecology is to transition food systems to more sustainable alternatives (Gliessman Citation2016), such agroecological transitions come with great uncertainty (Anderson et al. Citation2021; Magda et al. Citation2019).
Farmers considering transitioning to new land management practices weigh tradeoffs of social, economic, and biophysical criteria (Dendoncker et al. Citation2018; van Zonneveld, Turmel, and Hellin Citation2020). For instance, innovative practices may have tangible benefits, like reducing weed pressure or increasing crop marketability, as well as non-material benefits, such as if a practice aligns with farmers’ value systems or maintains a preferred farm aesthetic (Adams and Morse Citation2019; Ribeiro, Correia, and Paracchini Citation2016). Farmers must weigh these benefits against potential challenges, such as additional labor or infrastructure costs. Agricultural practices also have the potential to lead to negative ecological impacts, such as losses in biodiversity or ecosystem function, which are especially concerning if they negatively affect farm function (Fischer, Lindenmayer, and Manning Citation2006; Tscharntke et al. Citation2012). While farmers can easily track some of these considerations (like weed pressure or implementation costs), others are more difficult to quantify (such as impacts on biodiversity).
To understand complex dynamics on their farms, farmers often work with academic researchers. Researchers may validate farmers’ observations, provide insight to causal mechanisms, or uncover more “invisible” elements within their systems (such as nutrient levels and difficult-to-see communities like microorganisms and arthropods). However, applied academic research can take many forms and interpreting such research should warrant caution. While applied scientific research should be done for the benefit and interests of stakeholders (e.g. small-scale farmers), it sometimes runs the risk of being pushed too far into the theoretical realm, diverging from farmer interests, or being designed in a way that is unrealistic (Marabelli and Vaast Citation2020; Sharma and Bansal Citation2020; Teague et al. Citation2013). Therefore, results of such studies can waver in their relevance to real-life systems, ranging from being extremely helpful to misleading, and sometimes creating more uncertainty.
The complexity and uncertainty around agroecological transitions call for novel and innovative research methodologies (López-García et al. Citation2021). A key approach in agroecology is participatory action research (PAR) (Méndez, Bacon, and Cohen Citation2013; Utter et al. Citation2021). PAR seeks for all participants in the process (including researchers, community stakeholders, etc.) to fully engage in all aspects of research, from co-creating research questions to interpreting data. Ideally, all actors in this process would also engage in reflection and the action or implementation of initiatives informed by the research (McTaggart Citation1991; Méndez et al. Citation2017). PAR encourages mutual learning, or a reciprocity of knowledge sharing between academics and non-academics (Méndez, Bacon, and Cohen Citation2013; Méndez et al. Citation2017; Polk and Knutsson Citation2008; Utter et al. Citation2021). By promoting trust, accountability, collective power, and humility (Méndez et al. Citation2017), PAR stands in contrast to traditional extractive research methods (Smith Citation2021), which are especially present in agricultural studies (Menconi, Grohmann, and Mancinelli Citation2017). Ultimately, academic research generated through PAR can benefit local decision-making by being realistic, relevant, and applicable (Luján Soto, de Vente, and Cuéllar Padilla Citation2021). Despite its immense value (Caswell et al. Citation2021), there are still relatively few studies in agriculture that fully engage with PAR (Sachet et al. Citation2021). Factors limiting PAR include the lack of formal training in PAR and skills needed for PAR (e.g., facilitation, humility, and communication). The long-term nature of PAR projects is also challenging in that it does not align with common funding structures or fast-paced publishing expectations in academia (Whitmer et al. Citation2010; Méndez et al. Citation2017).
Given its transdisciplinary focus (Fernández González, Ollivier, and Bellon Citation2021; Méndez, Bacon, and Cohen Citation2013), PAR aligns with mixed methods research. Mixed methods research is the practice of combining quantitative and qualitative methodologies, such as ecological field data and textual interview data (Creswell and Creswell Citation2017), analogous to the integration of academic and non-academic knowledge systems in PAR. Both mixed methods and PAR allow researchers to view the world through multiple lenses, furthering knowledge co-creation and poising collaborators to better understand and solve complex environmental and societal issues, like agroecological transitions (Bennett et al. Citation2017; Gaba and Bretagnolle Citation2020; Hermans et al. Citation2021; López-García et al. Citation2021; Palmer Citation2012). By drawing on multiple perspectives and types of knowledge, the integration of PAR and mixed methods can help clear up points of uncertainty around agroecological transitions.
In this study, we used PAR and mixed methods to engage in mutual learning around the tradeoffs associated with agricultural tarping. Tarping is an agricultural practice in which farmers cover fields with impermeable plastic sheets generally for several weeks. We focus on the use of tarping for weed control, though tarping is also used for pest suppression and cover crop or perennial grass termination (Coelho, Chellemi, and Mitchell Citation1999; Elmore et al. Citation1997; Lounsbury et al. Citation2018). Tarping has risen in popularity on small-scale vegetable farms in the northeastern USA and is seen as a potential sustainable practice because it reduces tillage and herbicide needs (Lounsbury et al. Citation2022; Rylander et al. Citation2020). Many farmers have adopted or experimented with tarping, but uncertainties remain. For instance, while there is a multitude of evidence (both academic and anecdotal) that tarping effectively suppresses weeds (Elmore, Roncoroni, and Giraud Citation1993; Lounsbury et al. Citation2022; Rylander et al. Citation2020), other impacts remain unclear, including impacts on nutrients and soil biodiversity.
This project grew out of the collaborative interests of farmers and researchers, and the biophysical research questions and study variables were shaped through iterative engagement (; Méndez et al. Citation2017). We additionally conducted interviews with our farmer partners. The interviews supplement our quantitative results with anecdotal evidence around tarping while also documenting farmers’ reactions to our quantitative results, which contextualizes and increases the credibility of our quantitative findings by checking it against local knowledge. These qualitative interviews are not regionally generalizable due to their low sample size (8 interviews conducted with 4 interviewees) and nonrandom method of choosing participants (the interview participants are our PAR partners). Instead, the interviews should be seen as a tool to build PAR relationships while also formally demonstrating the co-creation of knowledge between the academic and farmer partners of this study. Our overall research objectives were to 1) assess the tradeoffs of tarping using both quantitative and qualitative methods; 2) assess how our quantitative biophysical results align with farmer perceptions; and 3) demonstrate the value of PAR for understanding emerging agricultural practices.
Methods
PAR process and methodology
This study was made possible due to ongoing relationships between the Agroecology and Livelihoods Collaborative (ALC) and local farmers in Chittenden County, Vermont, USA. The ALC is a community of practice within the Department of Plant and Soil Science at the University of Vermont. It brings together students, researchers, extension personnel, farmers, and other food systems actors. The mission of the ALC is to co-create evidence and knowledge with farmers and other actors to cultivate socially just and ecologically sound food systems. Three out of the four farmers included in this study (“farmer partners”) have worked with the ALC for over a decade through an Advanced Agroecology undergraduate course, where students work and learn on the farms (Horner et al. Citation2021). We embarked on this research together with these farmer partners due to their initial interest in the effects of tarping, their willingness to participate in the study (including lending a portion of their land for our plots), and the long-term relationships and trust built between them and the ALC, which facilitated healthy and productive collaboration.
Our research design weaved together academic and farmer knowledge at many points (). The research topic was motivated by informal conversations with the farmer partners (the “preflection” phase; ). Through additional conversations, the farmer partners revealed a particular interest in the effects of tarping on soil nutrients and biodiversity. We created a research plan that integrated the farmers’ interests with our own, which included soil arthropods, weeds, and mixing quantitative and qualitative data. We brought this research plan back to farmers and through multiple, iterative conversations the farmers commented on and helped revise the research plan. One major focus of these conversations was choosing and refining our tarping and control treatments and management decisions, with the goal that our methods mimicked realistic farm practices and would yield useful and applicable results. After we reached consensus on the biophysical study design, we began fieldwork in May 2021. In June 2021 we conducted our first round of interviews with farmers, which focused on farmers’ experiences and perceptions of tarping. After our fieldwork ended in August 2021, we compiled our preliminary biophysical results into a presentation. We gave this presentation to each farmer before conducting a second interview, in which the farmers responded to our results. We used this second interview to deliver knowledge back to our farmer partners (an important tenant of PAR; Méndez et al. Citation2017) while also formalizing their responses and feedback. We used the results of this second interview, which highlighted variables that farmers were particularly interested in or uncertain of, to inform additional variables to include in our final version of the results and to shape discussion points. In this way, the quantitative and qualitative research elements informed one another, thus following a “bidirectional synthesis” design (Kinnebrew et al. Citation2020).
Biophysical research design
Fieldwork took place in the summer of 2021 on three small-scale certified organic vegetable farms in Chittenden County, Vermont. We refer to these farms as Farm A, Farm B, and Farm C. All three farms are relatively close (<10 km) to one another and to the city of Burlington. They are all embedded in semi-natural habitats: Farm A is surrounded by a mix of temperate forest and grassland habitat, and Farms B and C are situated near a network of recreational forested areas within the floodplains of the Winooski River. One key difference among sites is that Farm A’s soils are sandy, Farm B’s soils are sandy loam, and Farm C’s soils are loam. Each farm also had a different composition of major weeds (). We used irrigation and fertilizer schemes typical to each farm (rather than controlling management among farms) because these practices are honed to each farm’s environment, reflect the operational capacities of each farm, and represent realistic scenarios ().
Table 1. Biophysical and management differences between the three study farms.
In June 2021, when tarps were applied, this region had a mean precipitation of 0.2 cm per day, a mean maximum temperature of 28°C, and a mean minimum temperature of 16°C (these conditions were hotter and drier than historical means). In July, after tarps were removed, the region experienced wetter and cooler weather than historical means, with a mean precipitation of 0.5 cm per day, a mean maximum temperature of 25°C, and a mean minimum temperature of 16°C (NOAA, Citation2021).
We tested tarping with two types of plastic tarps, silage tarps and clear plastic tarps, along with a hoed control. Our silage tarps were 5 mil (0.13 mm) thick polyethylene with a black upper-facing side and white lower-facing side (Klerks Hyplast Inc., Chester, South Carolina, USA). Our clear plastic tarps were 6 mil (0.15 mm) thick transparent polyethylene (Poly-Ag Corp, San Diego, California, USA) and were previously used as a hoophouse cover. We specifically used repurposed clear plastic because this reflects a common practice on farms (Birthisel et al. Citation2019).
We laid out plots in a completely randomized block design, with each farm including six replicates of each of the three treatments on each farm (18 plots per farm; 54 plots total). Treatment plots were 4.5 m by 1.5 m (1.5 m was the approximate width of the crop beds), with a buffer space of 0.5 m between plots.
Before the tarping treatments were applied, all fields were prepared with disc harrows (20–25 cm depth), amended with farm-specific fertilizers (), and then lightly tilled to incorporate the fertilizer (10–15 cm depth). We irrigated the fields 1–2 days before applying the tarps (irrigation amounts in ) to stimulate weed germination and increase tarp effectiveness for killing weeds (irrigating before tarping is a common practice amongst farmers). We applied the tarps in late May 2021 and sealed the tarps’ edges by burying them. The tarps were left on the fields for 25 days. During this time, we hoed the control plots weekly to create stale seed beds and simulate a common organic weed-control practice.
Within a week after tarp removal, we direct seeded lettuce (Lactuca sativa; Encore Lettuce Mix, Product ID: 2366G from Johnny’s Selected Seeds; Winslow, ME, USA) in each plot using a Jang seeder (Jang Automation Co., LTD, South Korea), calibrated to deposit 2 seeds every 2.54 cm. We seeded two rows, spaced at least 0.3 m in from the edge of the tarp, and 0.3 m to 0.6 m from each other within the plot. We did not remove weeds in any plots (tarped or control) after tarps were removed.
Biophysical field data collection
Before tarps were applied, we used composite sampling to collect soil from each farm and assess farm baseline soil conditions, including measurements of pH, soil organic matter, phosphorus, and soil texture (SI 1). During tarp application, we measured soil temperature using iButtons (Thermochron, Baulkham Hills, NSW, Australia), which logged temperatures every 30 minutes. Two iButtons were buried in each plot: one at the soil surface (buried under approximately 1 cm of soil) and one 10 cm below the surface. We additionally manually measured soil moisture using a TDR-350 soil probe (Spectrum Technologies, Inc., Aurora, IL) 3 weeks into tarp cover by briefly uncovering tarps (16 June 2021). Finally, we measured soil nitrate-N 2–3 days before and after tarp treatment, using composite sampling in each plot.
We sampled arthropods 5 times throughout the field season: 1 week before tarping (to get a baseline of arthropod diversity), 3 weeks into the tarp treatment, and 1, 3, and 5 weeks after tarps were removed. We sampled arthropods in each plot using two methods: pitfall traps (for surface and litter organisms) and the Berlese funnel method (for belowground organisms) (see SI 2 for additional information). We then classified specimens into morphospecies and calculated richness (separately for pitfall and Berlese funnel samples).
We surveyed crops and weeds weekly after tarps were removed. We conducted these surveys in 1 m by 2 m quadrats which were positioned in the center of each treatment plot (we refer to this area as the “plot” when discussing the crop and weed surveys). For crops, we randomly chose 10 lettuce individuals per plot and for each measured the number of leaves and the height and width of the largest leaf. We harvested the lettuce after 5 weeks of growth, and within each plot we counted the number of lettuce individuals and weighed total lettuce biomass (crop yield). To survey weeds, we visually estimated percent cover for each present weed species and calculated weed species richness and total coverage of weeds for each plot. Additional details of all biophysical sampling procedures are included in the Supplemental Materials (SI 2).
Interviews
We interviewed four farmers (one farmer each from Farm A, Farm B, and Farm C, and one additional farmer from Farm D, another small-scale vegetable farm in Chittenden County, Vermont) twice during the summer of 2021 using semi-structured interviews. All four farmers identified as White, were between the ages of 30–55, and had at least an undergraduate education (the farmer at Farm D additionally had a master’s degree). The interviewed farmers at Farm A and Farm D had been farming for around 5 years at the time of the interview, while the interviewed farmers at Farm B and C had been farming for over two decades.
All interviews were recorded with consent from the farmers, and each farmer is referred to using pseudonyms. The first interview focused on farmers’ experiences with tarping, including the perceived benefits and challenges of tarping. The second interview, which took place following a 30-minute presentation on the biophysical results of tarping, assessed to what extent our field results affirmed their experiences with tarping, whether the results provided any shift in perception about tarping, whether there were additional analyses farmers wanted to see from the data, and whether academic results are a convincing form of knowledge. Interview protocols are listed in the Supplemental Materials (SI 3).
Quantitative analyses
We tested how tarp treatments affected biophysical variables using analysis of variance tests (ANOVAs). For soil moisture, soil nitrate-N (measured as change in soil nitrate-N from before to after tarping), number of crop individuals per plot, and crop yield, we tested significance using linear mixed effects models with farm as a random factor. For data that we collected over multiple time periods, including soil temperature, soil arthropod richness, weed coverage and richness, and crop growth parameters (number of leaves per plant and leaf sizes), we tested significance using repeated measures ANOVAs, again with farm as a random factor for most variables. For crop parameters, we used plot nested in farm as a random factor due to taking 10 measurements per plot. We additionally analyzed extra variables, including the impact of tarping on specific weed species and arthropod groups, which were variables of farmer interest revealed from the second interviews. These data were also analyzed using repeated measures ANOVAs.
For all analyses, we removed extreme outliers and visually checked for normality. As needed, we log transformed data to better achieve normality of residuals. Models were fit using the lmer function in the lme4 package (Bates et al. Citation2015) and multiple comparisons were made using the lsmeans function or, for repeated measures ANOVAs, the emmeans function in the lsmeans package (Lenth Citation2016). All analyses were done in R version 4.0.4 (R Core Team Citation2021).
Qualitative analyses
Interviews were transcribed using Descript (Descript.com) and open coded by CM, SWW, and EK. Applying a modified constant comparative method, there were multiple stages of coding and analysis. Using the pre-identified interest in tradeoffs as a sensitizing concept (Bowen Citation2006), codes were developed and grouped in an iterative process that enabled identification of important themes relevant to the research questions (Creswell and Creswell Citation2017). All interviews were coded multiple times by a minimum of two authors using NVivo (released in March 2020, https://www.qsrinternational.com/nvivo-qualitative-data-analysis-software/home).
Results
Biophysical variables
Soil temperatures at both the surface and 10 cm below the surface were significantly higher in the tarped treatments than in the control and were hotter under the clear plastic tarps than the silage tarps (all P < .0001; ). The highest recorded surface temperature under the clear plastic tarps was 59°C, compared to 52°C in the silage tarp and 43°C in the control plots. At 10 cm below the soil surface, there was a maximum temperature of 48°C in the clear plastic tarp treatment, compared to 34°C in the control. In general, temperatures 10 cm below the soil surface were around 8°C hotter in the clear plastic tarp treatment than the control while the silage tarp was around 3°C hotter than the control ().
Figure 2. Impacts of silage tarps and clear plastic tarps on soil surface temperature (A), soil temperature (10 cm below the surface); B), soil moisture, measured 16 June 2021 (C), and soil nitrate-N (measured as after – before tarping; D). Temperatures are averaged among farms and demonstrate trends during five days of tarp application in 2021; low temperaturess on June 14th were due to cool, rainy conditions. Soil moisture and soil nitrate-N are separated by farm to highlight site differences.
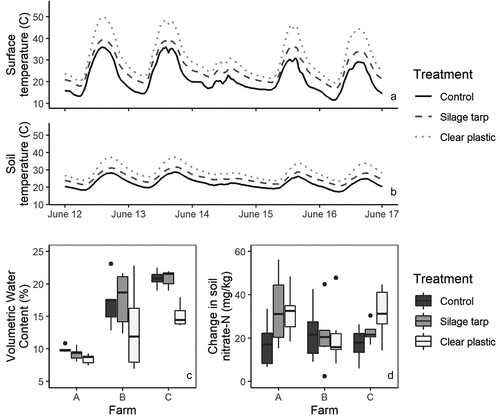
Soil moisture was significantly lower under the clear plastic tarps compared to the silage tarp and control plots (both P < .01), but was similar between the control and silage tarp plots (P = .86; ). Soil nitrate-N results varied by farm. In a model with all three farms, clear plastic tarp plots had a slightly more positive change in nitrate availability than the control plots, but this difference was not significant (P = .09; ). One farm, Farm B, saw particularly little difference between treatments. In a model without Farm B, there was a significant difference between the clear plastic tarp and control plots (P = .02).
During the tarp treatment, aboveground arthropod richness values were significantly lower in the two tarped treatments than in the control (both P < .001), with around 8 fewer morphospecies (). Aboveground arthropod richness remained lower in the silage tarp plots than the control plots 1 week after tarp removal (P = .04). At 3 and 5 weeks after tarp removal, there were no differences between treatments for aboveground richness (). Belowground soil arthropod richness values were much lower than the aboveground richness values (). During the tarp treatment, there was a mean of around 2.5 morphospecies per plot in the control plots, 1.8 morphospecies per plot in the silage tarp plots (P = .19 from the control), and less than 1 morphospecies per plot in the clear plastic tarp plots (P < .001 from the control). There were no differences at 1 and 3 weeks after tarp removal, but 5 weeks after tarp removal both tarped treatments had lower belowground arthropod richness than control plots (silage tarp – control P = .013; clear plastic – control P = .003; ).
Figure 3. Impacts of silage tarps and clear plastic tarps on aboveground arthropod richness (A), belowground arthropod richness (B), flea beetle (Coleoptera: Chrysomelidae) abundance (C), crop yields (D), weed coverage (E), and purslane (Portulaca oleracea) coverage (F). Significance (p < .05) is indicated by an asterisk (*) for differences between silage tarp and control plots, † for differences between clear plastic tarps and control plots, and § for differences between silage tarps and clear plastic tarps. NS indicates no significant differences between treatments. In A-C, negative numbers in the x axis represent number of weeks before tarps were applied and positive numbers in the x axis represent number of weeks after the tarps were removed. Plots C and F represent specific variables in which farmers expressed interest in the second interview (Section 3.4).
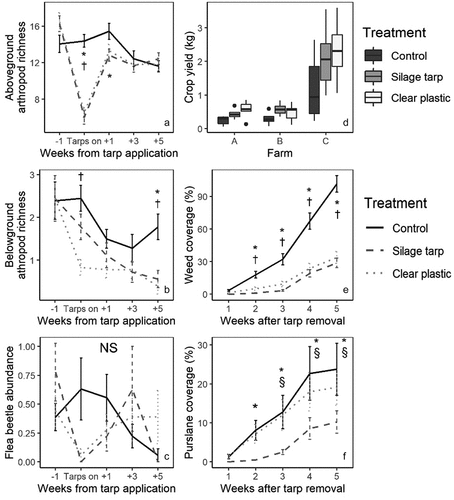
The impact of tarping on pest species was of high interest to farmers (see Section 3.4). Flea beetles (Coleoptera: Chrysomelidae), which were the most mentioned pest in the interviews, were not very abundant in our samples. While there was low flea beetle abundance in the tarp treatments during tarp application, there was no significant difference between treatments (). Other pest species, including weevils (Coleoptera: Curculionoidea), click beetles (Coleoptera: Elateridae), or aphids (Hemiptera: Aphidoidea), had similar abundances among treatments (no significant differences). Among common natural enemy species, we found that silage tarps boosted the abundance of ground beetles (Coleoptera: Carabidae; P = .04 compared to the control), but clear plastic plots had fewer wolf spiders (Araneae: Lycosidae) than control plots (P = .03) during tarp cover.
Tarping had positive effects on crops, with crop yields in the tarped treatments being twice those in the control plots (both P < .002; ). Similarly, lettuce plants had significanfly more leaves in the tarped treatments than in the control (clear plastic – control P < .001; silage tarp – control P = .04). As well, tarping caused slight increases to leaf size: crop leaf width 4 weeks after planting was larger by 5–8 mm in silage tarps plots than control plots (P = .05). However, the number of lettuce individuals per plot at harvest did not differ among treatments. Crop yields negatively related to weed cover (P = .001) but did not relate to available soil nitrate-N (P = .517; SI 4). Crop yields were also highest in Farm C (where available phosphorus levels were over three times the levels in Farm A and B; SI 1).
Tarping suppressed weed cover. While all treatments had less than 5% weed cover at the time of tarp removal, by 2 weeks after tarp removal the tarped treatments had significantly less weed coverage than control plots (clear plastic – control: P = .034; silage tarp – control: P = .003). Weed coverage remained much lower in the tarped treatments for the remainder of the experiment (P < .001 for all comparisons between tarped treatments and control; ). By 5 weeks after tarp removal, control plots had an average of around 90% weed coverage while the tarped plots had around 30% weed coverage.
Weed species richness values were also generally lower in the tarped treatments than in the control plots, though this difference decreased through time. For instance, while richness values were lower in both tarped treatments from 1 to 4 weeks after tarp removal (all P < .001), by 5 weeks after tarp removal silage tarps did not significantly differ from control plots (P = .14), and clear plastic plots were less significantly different than control plots (P = .015). At 5 weeks after tarp removal, all plots had an average of just under 6 weed species, though the maximum number of weeds found in a plot was 10 species (in a control plot).
Finally, while most weed species were suppressed by tarping, purslane (Portulaca oleracea) provided a major exception (a point of interest among farmers, see Section 3.4). We found that silage tarp plots had lower purslane cover than control plots starting at 2 weeks after tarp removal (P = .047), but clear plastic tarps were ineffective at controlling purslane (). Purslane cover never differed between control plots and clear plastic tarp plots and, from 3 to 5 weeks after tarp removal, purslane cover was significantly higher in clear plastic plots than in silage tarp plots. At the end of the experiment, clear plastic plots had an average of 19% purslane coverage, compared to 24% in control plots, and 10% in silage tarp plots.
Farmer perceptions of tarping tradeoffs
Overall experiences
The four farmers we interviewed included “Ana” (Farm A), “Adam” (Farm B), “Hayley” (Farm C), and “Elliot” (Farm D). The farmers all used or had used silage tarps. While one farm had experimented with clear plastic tarps, they had only used it on a small scale. Therefore, the results from the first interview relate only to silage tarps.
Of the four farmers, Hayley and Adam regularly used tarping in their rotation, Elliot used tarping as needed, and Ana was largely taking a break from tarping. The three farmers who were currently using tarping had very positive perceptions of tarping. Ana had less positive opinions, largely due to tarping’s ineffectiveness at suppressing purslane, a main weed on her farm. Overall, we found major themes within all interviews pertaining to benefits, challenges, and uncertainties with tarping (), which we describe below.
Benefits of tarping
The main benefits of tarping fell into three main subthemes: weed suppression, soil health, and non-material benefits (). Reducing weed pressure is one of the main desired outcomes of tarping, and most farmers confirmed that tarping was effective for this. Elliot noted that tarping functioned as a “reset” button on his farm, clearing problematic weeds and creating a new weed-thin baseline. This reduction in weed pressure had a number of related benefits, including saving time. Hayley, reflecting on the past when her farm didn’t use tarping, noted that the “hand weeding was just endless.” Adam also noted that lower weed prevalence increased the quality of crops and led to easier harvest and better commercial sale.
Farmers also expressed a variety of anecdotal evidence on the biophysical benefits of tarping. For example, Hayley found that tarping increased crop yields: “we think our stuff has looked good and healthier and more robust.” Farmers also reflected on tarping’s benefits to soil health, and all farmers mentioned that they thought tarping increased soil nitrate availability. Elliot noted that the general quality of his farm’s soil improved after tarping, mentioning increases in organic matter, tilth, water retention, and earthworm populations. However, Hayley expressed an opposite experience, noting that tarped soils were crustier, wetter, and harder to work with, highlighting that farmers did not always have the same experience with tarping. Such differences in soil conditions following tarping may relate to management practices, such as the extent to which farmers watered the fields before tarp application.
Finally, some farmers noted non-material benefits of tarping. Tarping helped support farmers’ visions for their farms or healthy food systems more broadly, for example by reducing tillage or herbicide use. Ana also noted a major education-based benefit of tarping:
A lot of students that come through [the farm] … are going on to start their own farms and may not necessarily have the capital to invest in a big tractor or might be starting really small-scale and not be doing any tillage. So, anyways, we wanted to model that … and the tarps kind of tied in with that.
Thus, for Ana, whose farm hosted a farmer training program, tarping helped model a cheap, simple, and small-scale practice that new farmers could easily adopt. Hayley also noted the benefit of tarping to boost morale, noting, “Mentally, it feels better to know that there’s an end to the hand weeding or it’s like a more spot treatment.” Thus, tarping provided an emotional benefit for the farmers at her farm because it made weed control seem more manageable.
Challenges of tarping
When asked about the challenges of tarping, all farmers brought up labor requirements (). Tarps are physically large and all farmers reported that moving them requires substantial strength and several people. Throughout tarping, the tarps often become heavier due to accumulation of water, dirt, and in some cases bird feces, which makes removing the tarps “messy,” “smelly,” and “muddy.” Several farmers also reported that securing the tarps was particularly arduous, and Adam described the process of filling sandbags to hold down tarps the “most time-consuming part of tarping.” Hayley similarly noted on the process of handling tarps: “it’s sort of absurd and ridiculous, and you’re just like, what are we even doing?,” which well summarizes the common frustration amongst farmers.
Another main challenge with tarping was adjusting to new planning schedules. Tarps are generally on the fields from 2–4 weeks, as opposed to tilling, which can be done in an afternoon. Therefore, farmers had to learn to plan ahead, establish an entirely new organization or “rhythm” of farm activities, and even sacrifice part of the growing period. Furthermore, there are a variety of practices on the farm believed to increase the efficacy of tarping, notably watering the fields (or waiting for rain) before tarping to stimulate germination. Farmers posited that when they forgot this step or planned incorrectly with the weather, tarping was not as effective.
Finally, while weed suppression was one of the main benefits of tarping, the farmers also expressed challenges with tarping being ineffective for certain weeds, being less effective in certain locations on their farm, or losing effectiveness over time. One farmer noted that while tarping was effective for lamb’s quarters (Chenopodium album), redroot pigweed (Amaranthus retroflexus), and hairy galinsoga (Galinsoga quadriradiata), it was less effective for crabgrass (Digitaria grasses) and, especially, purslane. The ineffectiveness of silage tarps against purslane was a huge challenge for Ana:
We were finding just like carpets and carpets of purslane. So that was a bummer … at least in our systems the tarp wasn’t effective against purslane.
Ultimately, this struggle with purslane, a major weed at Ana’s farm, was a main factor in her deciding not to continue with tarping. Ana noted:
It’s so dependent on just your system and what you’ve got going on, what your soil is like … Tarps could work great and they could not work at all.
This quote keenly summarizes the context dependency of tarping’s efficiency.
Uncertainties with tarping
Among the benefits and challenges of tarping, farmers felt a considerable amount of uncertainty around this practice, especially around tradeoffs with other alternative methods, and they had questions about how tarping mechanistically functions ().
While tarping is used to replace other more intensive practices, like tilling and herbicide use, farmers had doubts that using large amounts of plastics on their farms was actually advantageous. Hayley noted:
We’re not running the tractor as much, but then I’m like, okay, … we bought all this plastic. … I don’t know which one is worse or which one is better. I mean, you can’t reuse diesel, you can reuse the silage tarps.
Ana also grappled with the difference between using plastic and herbicides, while also bringing up the concern that microplastics from tarps might enter the soil and affect soil functioning. Thus, the farmers shared a general concern over whether using plastics was the most sustainable choice in their agricultural systems.
Farmers also had many questions about the mechanisms of tarping. Adam stated:
What’s going on under there? Is it that the weed seeds are germinating and then they’re starved for light? Is it that they’re germinating and then they expire because it’s too hot? … Is it that the tarping is actually accelerating certain biological communities that are then decomposing the seeds?
Here, Adam highlights that farmers still do not exactly know why tarping functions. Both Adam and Elliot expressed that better understanding tarping’s exact mechanisms would be not only exciting but also extremely valuable in advising their future use of tarping (e.g., informing how long to leave tarps on the field during different times of the growing season).
Farmer responses to our results
Farmers had a variety of responses to our presentation of biophysical results. Many of our results affirmed farmers’ experiences, especially results relating to increased temperatures under the tarps, reduced overall weed pressure, lower effectiveness of clear plastic tarps controlling purslane, and higher crop yields. Consequently, several farmers noted that our results would not lead to drastic differences in their tarp application, but rather validated their use of tarping by increasing their confidence in its benefits.
However, other results were less validating to farmers. In particular, farmers were perplexed by our soil nitrate results. Adam noted of nitrate:
Certainly that’s something that a number of people … advocate for as a major benefit of tarps. [Tarps’] nitrate preservation intuitively makes sense. But if it’s not really what happened … it’s good to know that.
Like Adam, several of the farmers had predicted a more positive effect of tarping on soil nitrate availability, and, in response, offered suggestions as to why our results did not match their expectations. For example, some farmers suggested different experimental designs that may better capture trends, including measuring soil nitrate availability continuously for several weeks after removing tarps.
Our soil arthropod results generated surprise and interest amongst farmers. While farmers listed functional and/or ethical reasons for preserving soil biodiversity, this ecological group was particularly unknown to them (with the exception of earthworms). For Ana, our arthropod results provided a “good surprise,” in particular that aboveground arthropod populations rebounded after an initial diversity decline with tarping (). In fact, Ana noted that if we had found more negative results on tarping’s arthropod effects it would have been the “nail in the coffin” for her use of tarping. These arthropod results, mixed with our other biophysical results showing benefits of tarping, slightly shifted Ana’s opinions on tarping. Despite being fairly negative toward tarps in her first interview, in her second interview she noted “Maybe [I’ll] give them another chance.” Thus, our research found enough positive outcomes of tarping for Ana to express an opinion change.
However, the soil arthropod results also generated more questions than answers for some farmers. For instance, Hayley found it difficult to conceptualize the biodiversity results:
Do we know every single time we run the cultivator through those beds what that does to arthropod populations? Is that just as bad or is it better?
This highlights that decision-making around tarping cannot happen without understanding how the effects of tarping compare to those of other practices. Many farmers also raised questions around arthropod pests, noting that some of the arthropods lost to tarping could be pests, which could offer agronomic benefits. This highlights that diversity data without indication of species’ ecosystem roles may have only limited value to farmers. Accordingly, most of the farmers interviewed expressed the value of determining the effects of tarping specifically for pest or natural enemy species.
Similarly, when shown the effects of tarping on specific weeds, most farmers commented only on the results for purslane. While farmers were not particularly surprised by our result that purslane was unaffected by clear plastic tarps, it nevertheless garnered concern and questions, such as about purslane’s growth mechanisms and how climate change will affect purslane’s success.
Finally, when asked to what extent farmers take into account or trust scientific research, they all expressed a similar sentiment: academic research is especially helpful when it affirms or builds on farmer observations. Hayley noted:
I have a lot of faith in our observations, but I know that we don’t practice great science … And there’s so many factors that could influence things. So it’s really nice to see methodically done research that lines up with our thoughts.
Adam also noted that a main value of academic research for him was to illuminate mechanisms of practices that he already used, with this knowledge helping him make that practice even more effective. These responses highlight that academic research is valuable to farmers, but they use it to support their operations and affirm their own knowledge systems rather than as an authoritative guide.
Discussion
Overview of results
We assessed tradeoffs of agricultural tarping using a biophysical field study and farmer interviews. Our quantitative results demonstrated that tarping has key benefits, including dramatically suppressing weeds and boosting crop yields. The interviews reiterated these benefits and elucidated additional social and economic benefits of tarping, including saving time and modeling low-input techniques. The farmers validated many of our results but raised concerns or questions about certain findings (discussed below). Through PAR and our mixed methods research design, we engaged in mutual learning around tarping and deepened our relationships with our farmer partners.
Exploring topics of confusion and interest
Our results that tarping increased temperatures, decreased weed pressure, and increased crop yields matched farmer experiences and are supported by many academic studies (Birthisel and Gallandt Citation2019; Birthisel et al. Citation2019; Cohen and Rubin Citation2007; Elmore, Roncoroni, and Giraud Citation1993; Riemens et al. Citation2007; Rylander et al. Citation2020). Other elements relating to our research, such as our soil nitrate results, mechanisms of weed suppression (including poor efficiency of clear plastic tarps at controlling purslane), and the possibility of tarping to be used for pest and disease control, garnered confusion or interest amongst farmers.
Our results showed only limited evidence that tarping increased soil nitrate availability, but farmers expressed anecdotal evidence that tarping does increase the availability of soil nutrients. This sparked uncertainty and put into question whether our experiment could have been designed differently to better capture nitrate trends. Supporting farmers’ experiences, other studies have found that tarping and plastic mulch increased plant available soil nitrogen (Birthisel et al. Citation2019; Filipović et al. Citation2016; Rylander et al. Citation2020), likely due to higher temperatures under tarps catalyzing nitrification or tarp cover decreasing nitrate leaching events (Jarvis et al. Citation1996; Sintim et al. Citation2021). Our results, which were inconsistent among our farm sites, may result from each farm sites using a different organic fertilizer (tailored to each farm’s soil and nutrient needs; ). Because the different source ingredients and soluble versus insoluble N percentages in each farm’s fertilizers () can affect nitrate release rates and interactions with temperature and moisture (Gaskell and Smith Citation2007; Hartz and Johnstone Citation2006), sampling at multiple time points after tarps were removed (such as in Birthisel et al. Citation2019) may have uncovered different nitrogen dynamics.
While both tarps suppressed weeds at similar rates, the mechanisms by which these two tarps suppress weeds differs. A general theory might dictate that clear plastic tarps kill weed seeds through extreme temperatures (depleting the seed bank), while silage tarps prohibit germination both through increased temperatures and light absence, which could simply delay the prevalence of weeds (Birthisel and Gallandt Citation2019; Standifer, Wilson, and Porche-Sorbet Citation1984). However, weed suppression is a complex phenomenon, representing many different mechanisms, and is further complicated by the individuality of each plant species’ response to environmental conditions. For example, some plant seeds have drastically higher heat tolerances than others (Egley Citation1990), which may allow them to survive in the hot conditions under tarps. Also, while some seeds need light to germinate, others do not (Travlos et al. Citation2020; Yan and Chen Citation2020). Thus, while some seeds may remain dormant under silage tarps, others may germinate and ultimately perish due to persistent dark and hot conditions.
One plant which was not similarly impacted by silage tarps and clear plastic tarps was purslane. Purslane’s ability to survive under hot clear plastic tarps likely relates to its extreme resilience and even preference for hot conditions (Feng et al. Citation2015). In a paper testing 7 weed species in extreme temperatures, purslane had the highest tolerance, with 82% of seeds surviving at 7 days of 50°C heat (Egley Citation1990). Purslane’s ability to germinate and survive in high temperatures may be due to its physiology – purslane is a C4 plant, which can photosynthesize while its stomata are closed (Edwards et al. Citation2001). However, the lack of purslane in silage tarp plots indicates that the dark conditions were not hospitable to growth, supporting other studies (Feng et al. Citation2015; Zimmerman Citation1976).
Finally, farmers expressed interest in tarping for pest and disease suppression. While we did not find any major effects of tarping on arthropod pests, effects were difficult to discern due to a low prevalence of pests in our sites (). However, there is a long history of farmers using tarps to heat soils for pest and disease suppression, often termed “solarization” in relation to the use of clear plastic tarps (Elmore et al. Citation1997; Hansen, Johnson, and Winter Citation2011). Both black and clear tarps have been successful at suppressing beetle pests, parasitic nematodes, and major crop pathogens including Verticillium fungi (Verticillium wilt), Rhizoctonia solani (root rot), Athelia rolfsii (southern blight), and Phytophthora oomycetes (Coelho, Chellemi, and Mitchell Citation1999; Elmore et al. Citation1997; Katan Citation1981; McFarlane Citation1989; McSorley and McGovern Citation2000; Stapleton and DeVay Citation1986). In the context of our study, 3.5 weeks of tarping with maximum daily soil temperatures between 30–48°C under clear plastic tarp plots () would likely kill many but not all soil pests (Elmore et al. Citation1997), and greater temperatures and longer tarping time would increase pest and disease suppression. However, we encourage farmers to be wary of simultaneous negative effects on beneficial organisms (such as on the soil microbiome) and possible unintended long-term environmental consequences (Birthisel et al. Citation2019; Kanaan et al. Citation2018; Schreiner, Ivors, and Pinkerton Citation2001).
Remaining uncertainties around tarping tradeoffs
While farmers agreed on many of the benefits, challenges, and uncertainties around tarping (especially the messy and laborious process of handling tarps), the interviews indicated that farmers were still actively observing, judging, and making sense of tarping’s effects. This was highlighted by the large number of uncertainties and questions that farmers listed (see SI 5 for a full list of farmers’ questions around tarping). For instance, Hayley’s difficulty in controlling redroot pigweed the year of the interviews highlighted the context dependency of the effectiveness of tarping and dampened her enthusiasm for the practice. Conversely, sharing our experiment’s results with Ana renewed her interest in tarping. A major reason for farmers’ flexible opinions could be that tarping is still a new practice, which makes its long-term effects and context-specific efficiency unclear. We want to again highlight that these qualitative findings relate to the experiences of only four farmers and a higher sample size would be needed to increase the generalizability of our interview findings.
As well, while many of the benefits and challenges that farmers discussed in their interviews represent short-term effects of tarping, their stated uncertainties more often represented unknown long-term effects. Better understanding of the long-term effects of tarping will be essential to assessing the sustainability of this practice and allowing farmers to make more informed decisions (Steinmetz et al. Citation2016). One major future consideration of tarping is whether weeds will remain resistant to tarps’ effects. Weeds can undergo rapid evolution to better survive disturbances, including disturbances associated with agricultural management (Neve, Vila-Aiub, and Roux Citation2009), and farmers and researchers should stay alert to this possibility. Another potential long-term effect is the release of microplastics or chemical compounds from plastics into the soil. Although there is no evidence of this yet for tarping, microplastics from plastic mulch are a major issue (Steinmetz et al. Citation2016) and can decrease crop yields (Qi et al. Citation2018). Going forward, future research on ecosystem services or life-cycle analyses of tarp plastic may help farmers better understand the tradeoffs of tarping.
Mutual learning and knowledge co-creation
PAR can support mutual learning and increase the applicability of research. In our experiment, PAR was essential for guiding the direction of our research and maintaining positive, productive, and collaborative relationships with our partner farmers. Without engaging in PAR, we argue that we would not have achieved as robust an understanding of the context-dependent factors that determine the efficacy, suitability, and sustainability of this practice.
A major goal of our research was to identify the biophysical impacts of tarping for farmers. Some of the impacts we assessed were variables that farmers were already keenly aware of, such as impacts on weed prevalence, and our research helped to affirm and enumerate this information. However, it was particularly rewarding to share new information and unexpected results with farmers. For instance, the farmers all noted that while they valued soil biodiversity in some way, they had little understanding of which soil fauna lived on their farm and how tarping would affect them. Farmers’ unfamiliarity with soil arthropods was not a surprise – despite soil fauna’s value to agroecosystems, many farmers around the world have very limited knowledge around them and are mostly aware of soil fauna that are especially beneficial or harmful (Pauli et al. Citation2016, Citation2012). Our research helped shed light on this particularly “invisible” community, giving farmers more context about the life they work amongst and hopefully encouraging more consideration of this group in decision-making.
Through the PAR process, farmers also contributed to our knowledge in valuable ways. Farmers not only inspired this research idea, but also informed the research process by advising our methodological design which helped our experiment mimic realistic on-farm practices and create more relevant results. Because of the trust and respect that we established with the farmers, we also benefited from frequent informal conversations with farmers in the field. The anecdotal experiences that farmers shared taught us more about the tarping system than is available in academic literature. Finally, the interviews provided a more formal method of learning from the farmers, in which we gained knowledge around the variety of factors that weigh into farmers’ decision-making and learned which elements of our research were most valuable to document. The farmers’ feedback has also inspired future research, such as a more thorough study into tarping’s effects on nutrient availability.
Conclusions
Tarping is a promising alternative practice in agriculture that shows positive effects for weed suppression and boosts in crop yields while reducing tillage and herbicide needs. However, more research is needed to understand the context dependency of tarping’s effectiveness and to discover possible long-term effects. We recommend future researchers keep in mind a key finding from the interviews – while farmers valued academic knowledge, they also heavily relied on their own observational knowledge and experiences. This finding supports the value of approaches that foster knowledge co-creation, like PAR, to make research more informative, relevant, and approachable to decision-makers.
Ethics declaration
This study was reviewed by the Research Protections Office at the University of X (Study#: 0001633) and was determined to fall into the exemption category 2. All human subject protection regulations were followed and no problems arose during the experiment that needed to be raised with the office.
Supplemental Material
Download MS Word (179.2 KB)Acknowledgments
We are extremely grateful to all farmers at our partner farms for their support and guidance throughout this project. Thank you as well to Macie Millistein, Sarah Brickman and Marie English for assistance in the field or lab.
Disclosure statement
No potential conflict of interest was reported by the author(s).
Data availability statement
All data associated with this paper are available at https://doi.org/10.6084/m9.figshare.c.6298920.
Supplementary material
Supplemental data for this article can be accessed online at https://doi.org/10.1080/21683565.2022.2146254.
Additional information
Funding
References
- Adams, A., and J. Morse. 2019. Non-material matters: A call for integrated assessment of benefits from ecosystems in research and policy. Land Use Policy 80:400–02. doi:10.1016/j.landusepol.2018.04.031.
- Altieri, M. A. 1987. Agroecology: The scientific basis of alternative agriculture. Boulder, CO: Westview Press.
- Anderson, C. R., J. Bruil, M. J. Chappell, C. Kiss, and M. P. Pimbert. 2021. Agroecology now!: Transformations towards more just and sustainable food systems. Cham, Switzerland: Springer Nature.
- Bates, D., M. Mächler, B. Bolker, and S. Walker. 2015. Fitting linear mixed-effects models using lme4. Journal of Statistical Software 67:1–48. doi:10.18637/jss.v067.i01.
- Bennett, J. A., H. Maherali, K. O. Reinhart, Y. Lekberg, M. M. Hart, and J. Klironomos. 2017. Plant-soil feedbacks and mycorrhizal type influence temperate forest population dynamics. Science 355:181–84. doi:10.1126/science.aai8212.
- Birthisel, S. K., and E. R. Gallandt. 2019. Trials evaluating solarization and tarping for improved stale seedbed preparation in the northeast USA. Organic Farming 5:52–65. doi:10.12924/of2019.05010052.
- Birthisel, S. K., G. A. Smith, G. M. Mallory, J. Hao, and E. R. Gallandt. 2019. Effects of field and greenhouse solarization on soil microbiota and weed seeds in the northeast USA. Organic Farming 5:66–78. doi:10.12924/of2019.05010066.
- Bowen, G. A. 2006. Grounded theory and sensitizing concepts. International Journal of Qualitative Methods 5:12–23. doi:10.1177/160940690600500304.
- Caswell, M., R. Maden, N. McCune, V. E. Mendez, G. Bucini, J. Anderzén, V. Izzo, S. E. Hurley, R. K. Gould, and J. W. Faulkner. 2021. Amplifying agroecology in Vermont: Principles and processes to foster food systems sustainability. Burlington, Vermont, USA: Agroecology and Livelihoods Collaborative, University of Vermont.
- Coelho, L., D. O. Chellemi, and D. J. Mitchell. 1999. Efficacy of solarization and cabbage amendment for the control of Phytophthora spp. in north Florida. Plant Disease 83:293–99. doi:10.1094/PDIS.1999.83.3.293.
- Cohen, O., and B. Rubin. 2007. Soil solarization and weed management. In Non-Chemical weed management: principles, ed. M. K. Upadhyaya and R. E. B. R. E. U.M.K., Blackshaw, 177–200. Wallingford: Concepts and Technology. CABI. doi:10.1079/9781845932909.0177.
- Creswell, J. W., and J. D. Creswell. 2017. Research design: Qualitative, quantitative, and mixed methods approaches. Thousand Oaks, California, USA: SAGE Publications, Inc.
- Dendoncker, N., F. Boeraeve, E. Crouzat, M. Dufrêne, A. König, and C. Barnaud. 2018. How can integrated valuation of ecosystem services help understanding and steering agroecological transitions? Ecology and Society 23: doi: 10.5751/ES-09843-230112.
- Edwards, G. E., R. T. Furbank, M. D. Hatch, and C. B. Osmond. 2001. What does it take to be C4? Lessons from the evolution of C4 photosynthesis. Plant Physiology 125:46–49.
- Egley, G. H. 1990. High-temperature effects on germination and survival of weed seeds in soil. Weed Science 38:429–35. doi:10.1017/S0043174500056794.
- Elmore, C., J. Roncoroni, and D. Giraud. 1993. Perennial weeds respond to control by soil solarization. California Agriculture 47:19–22. doi:10.3733/ca.v047n01p19.
- Elmore, C. L., J. J. Stapleton, C. E. Bell, and J. E. DeVay. 1997. Soil solarization: A nonpesticidal method for controlling, diseases, nematodes, and weeds. Oakland: Univ. Calif. Agric. Nat. Res. Publ.
- Feng, L., G.-Q. Chen, X.-S. Tian, H.-M. Yang, M.-F. Yue, and C.-H. Yang. 2015. The hotter the weather, the greater the infestation of Portulaca oleracea: Opportunistic life-history traits in a serious weed. Weed Research 55:396–405. doi:10.1111/wre.12151.
- Fernández González, C., G. Ollivier, and S. Bellon. 2021. Transdisciplinarity in agroecology: Practices and perspectives in Europe. Agroecology and Sustainable Food Systems 45:523–50. doi:10.1080/21683565.2020.1842285.
- Filipović, V., D. Romić, M. Romić, J. Borošić, L. Filipović, F. J. K. Mallmann, and D. A. Robinson. 2016. Plastic mulch and nitrogen fertigation in growing vegetables modify soil temperature, water and nitrate dynamics: Experimental results and a modeling study. Agricultural Water Management 176:100–10. doi:10.1016/j.agwat.2016.04.020.
- Fischer, J., D. B. Lindenmayer, and A. D. Manning. 2006. Biodiversity, ecosystem function, and resilience: Ten guiding principles for commodity production landscapes. Frontiers in Ecology and the Environment 4:80–86. doi:10.1890/1540-9295(2006)004[0080:BEFART2.0.CO;2.
- Gaba, S., and V. Bretagnolle. 2020. Social–ecological experiments to foster agroecological transition. People and Nature 2:317–27. doi:10.1002/pan3.10078.
- Gaskell, M., and R. Smith. 2007. Nitrogen Sources for Organic Vegetable Crops. HortTechnology 17:431–41. doi:10.21273/HORTTECH.17.4.431.
- Gliessman, S. 2016. Transforming food systems with agroecology. Agroecology and Sustainable Food Systems 40:187–89. doi:10.1080/21683565.2015.1130765.
- Hansen, J. D., J. Johnson, and D. A. Winter. 2011. History and use of heat in pest control: A review. International Journal of Pest Management 57:267–89. doi:10.1080/09670874.2011.590241.
- Hartz, T. K., and P. R. Johnstone. 2006. Nitrogen availability from high-nitrogen-containing organic fertilizers. HortTechnology 16:39–42. doi:10.21273/HORTTECH.16.1.0039.
- Hermans, T. D. G., A. J. Dougill, S. Whitfield, C. L. Peacock, S. Eze, and C. Thierfelder. 2021. Combining local knowledge and soil science for integrated soil health assessments in conservation agriculture systems. Journal of Environmental Management 286:112192. doi:10.1016/j.jenvman.2021.112192.
- Horner, C. E., C. Morse, N. Carpenter, K. L. Nordstrom, J. W. Faulkner, T. Mares, E. Kinnebrew, M. Caswell, V. Izzo, V. E. Méndez, et al. 2021. Cultivating pedagogy for transformative learning: A decade of undergraduate agroecology education. Frontiers in Sustainable Food Systems 5: doi: 10.3389/fsufs.2021.751115.
- Jarvis, S. C., E. A. Stockdale, M. A. Shepherd, and D. S. Powlson. 1996. Nitrogen mineralization in temperate agricultural soils: Processes and measurement. Advances in Agronomy 57:187–235. doi:10.1016/S0065-2113(08)60925-6.
- Kanaan, H., S. Frenk, M. Raviv, S. Medina, and D. Minz. 2018. Long and short term effects of solarization on soil microbiome and agricultural production. Applied Soil Ecology 124:54–61. doi:10.1016/j.apsoil.2017.10.026.
- Katan, J. 1981. Solar heating (solarization) of soil for control of soilborne pests. Annual Review of Phytopathology 19:211–36. doi:10.1146/annurev.py.19.090181.001235.
- Kinnebrew, E., E. Shoffner, A. Farah‐Pérez, M. Mills‐Novoa, and K. Siegel. 2020. Approaches to interdisciplinary mixed methods research in land-change science and environmental management. Conservation Biology 35:130–41. doi:10.1111/cobi.13642.
- Lenth, R. V. 2016. Least-squares means: The R package lsmeans. Journal of Statistical Software 69:1–33. doi:10.18637/jss.v069.i01.
- López-García, D., M. Cuéllar-Padilla, A. de Azevedo Olival, N. P. Laranjeira, V. E. Méndez, Y. Peredo, S. Parada, C. A. Barbosa, C. Barrera Salas, M. Caswell, et al. 2021. Building agroecology with people: Challenges of participatory methods to deepen on the agroecological transition in different contexts. Journal of Rural Studies 83:257–67. doi:10.1016/j.jrurstud.2021.02.003.
- Lounsbury, N. P., S. K. Birthisel, J. Lilley, and R. Maher. 2022. Tarping in the Northeast: A guide for small farms, 1075. Orono, Maine, USA: The University of Maine Cooperative Extension.
- Lounsbury, N. P., N. D. Warren, S. D. Wolfe, and R. G. Smith. 2018. Investigating tarps to facilitate organic no-till cabbage production with high-residue cover crops. Renewable Agriculture and Food Systems 1–7. doi:10.1017/S1742170518000509.
- Luján Soto, R., J. de Vente, and M. Cuéllar Padilla. 2021. Learning from farmers’ experiences with participatory monitoring and evaluation of regenerative agriculture based on visual soil assessment. Journal of Rural Studies 88:192–204. doi:10.1016/j.jrurstud.2021.10.017.
- Magda, D., N. Girard, V. Angeon, C. Cholez, N. Raulet-croset, R. Sabbadin, N. Salliou, C. Barnaud, C. Monteil, and N. Peyrard. 2019. A plurality of viewpoints regarding the uncertainties of the agroecological transition Agroecological Transitions: From Theory to Practice in Local Participatory Design, edited byJ.-E. Bergez, E. Audouin, and O. Therond, 99–120. Cham, Switzerland: Springer Nature.
- Marabelli, M., and E. Vaast. 2020. Unveiling the relevance of academic research: A practice-based view. Information and Organization 30:100314. doi:10.1016/j.infoandorg.2020.100314.
- Mason, R. E., A. White, G. Bucini, J. Anderzén, V. E. Méndez, and S. C. Merrill. 2021. The evolving landscape of agroecological research. Agroecology and Sustainable Food Systems 45:551–91. doi:10.1080/21683565.2020.1845275.
- McFarlane, J. A. 1989. Preliminary experiments on the use of solar cabinets for thermal disinfestation of maize cobs and some observations on heat tolerance in Prostephanus truncatus (Horn). Coleoptera: Bostrichidae). Tropical agriculture.
- McSorley, R., and R. J. McGovern. 2000. Effects of solarization and ammonium amendments on plant-parasitic nematodes. Journal of Nematology 32:537–41.
- McTaggart, R. 1991. Principles for participatory action research. Adult Education Quarterly 41:168–87. doi:10.1177/0001848191041003003.
- Menconi, M. E., D. Grohmann, and C. Mancinelli. 2017. European farmers and participatory rural appraisal: A systematic literature review on experiences to optimize rural development. Land Use Policy 60:1–11. doi:10.1016/j.landusepol.2016.10.007.
- Méndez, V. E., C. M. Bacon, and R. Cohen. 2013. Agroecology as a transdisciplinary, participatory, and action-oriented approach. Agroecology and Sustainable Food Systems 37:3–18. doi:10.1080/10440046.2012.736926.
- Méndez, V. E., M. Caswell, S. R. Gliessman, and R. Cohen. 2017. Integrating agroecology and participatory action research (PAR): lessons from Central America. Sustainability 9:705. doi:10.3390/su9050705.
- Neve, P., M. Vila-Aiub, and F. Roux. 2009. Evolutionary-thinking in agricultural weed management. New Phytologist 184:783–93. doi:10.1111/j.1469-8137.2009.03034.x.
- NOAA National Climatic Data Center. 2021. Monthly summaries of the global historical climatology network - Daily (GHCN-D). [Station location: Burlington International Airport, VT US]. accessed 11.14.21.
- Palmer, M. A. 2012. Socioenvironmental sustainability and actionable science. BioScience 62:5–6. doi:10.1525/bio.2012.62.1.2.
- Patel, R. 2013. The Long Green Revolution. The Journal of Peasant Studies 40:1–63. doi:10.1080/03066150.2012.719224.
- Pauli, N., L. K. Abbott, S. Negrete-Yankelevich, and P. Andrés. 2016. Farmers’ knowledge and use of soil fauna in agriculture: A worldwide review. Ecology and Society 21: doi: 10.5751/ES-08597-210319.
- Pauli, N., E. Barrios, A. J. Conacher, and T. Oberthür. 2012. Farmer knowledge of the relationships among soil macrofauna, soil quality and tree species in a smallholder agroforestry system of western Honduras. Geoderma 189–190:186–98. doi:10.1016/j.geoderma.2012.05.027.
- Polk, M., and P. Knutsson. 2008. Participation, value rationality and mutual learning in transdisciplinary knowledge production for sustainable development. Environmental Education Research 14:643–53. doi:10.1080/13504620802464841.
- Qi, Y., X. Yang, A. M. Pelaez, E. Huerta Lwanga, N. Beriot, H. Gertsen, P. Garbeva, and V. Geissen. 2018. Macro- and micro- plastics in soil-plant system: Effects of plastic mulch film residues on wheat (Triticum aestivum) growth. Science of the Total Environment 645:1048–56. doi:10.1016/j.scitotenv.2018.07.229.
- R Core Team. 2021. R: A Language and Environment for Statistical Computing. Vienna, Austria: R Foundation for Statistical Computing.
- Ribeiro, S. M. C., T. P. Correia, and M. L. Paracchini. 2016. Addressing the social landscape dimensions: The need for reconciling cross scale assessments for capturing Land Use Policy, Addressing the social landscape dimensions: The need for reconciling cross scale assessments for capturing. Cultural Ecosystem Services (CES) 53:1–2. doi:10.1016/j.landusepol.2015.12.022.
- Riemens, M., R. Van Der Weide, P. Bleeker, and L. Lotz. 2007. Effect of stale seedbed preparations and subsequent weed control in lettuce (cv. Iceboll) on weed densities. Weed Research 47:149–56. doi:10.1111/j.1365-3180.2007.00554.x.
- Rylander, H., A. Rangarajan, R. M. Maher, M. G. Hutton, N. W. Rowley, M. T. McGrath, and Z. F. Sexton. 2020. Black plastic tarps advance organic reduced tillage I: Impact on soils, seed seed survival, and crop residue. HortScience 55:819–25. doi:10.21273/HORTSCI14792-19.
- Sachet, E., O. Mertz, J.-F. Le Coq, G. S. Cruz-Garcia, W. Francesconi, M. Bonin, and M. Quintero. 2021. Agroecological transitions: A systematic review of research approaches and prospects for Participatory Action Methods. Frontiers in Sustainable Food Systems 5. doi:10.3389/fsufs.2021.709401.
- Schreiner, P. R., K. L. Ivors, and J. N. Pinkerton. 2001. Soil solarization reduces arbuscular mycorrhizal fungi as a consequence of weed suppression. Mycorrhiza 11:273–77. doi:10.1007/s005720100131.
- Sharma, G., and P. (Tima) Bansal. 2020. Cocreating rigorous and relevant knowledge. AMJ 63:386–410. doi:10.5465/amj.2016.0487.
- Sintim, H. Y., S. Bandopadhyay, M. E. English, A. Bary, Y. Liquet, J. E. González, J. M. DeBruyn, S. M. Schaeffer, C. A. Miles, and M. Flury. 2021. Four years of continuous use of soil-biodegradable plastic mulch: Impact on soil and groundwater quality. Geoderma 381:114665. doi:10.1016/j.geoderma.2020.114665.
- Smith, L. T. 2021. Decolonizing methodologies: Research and indigenous peoples. New York, USA: Bloomsbury Publishing.
- Standifer, L. C., P. W. Wilson, and R. Porche-Sorbet. 1984. Effects of solarization on soil weed seed populations. Weed Science 32:569–73. doi:10.1017/S0043174500059580.
- Stapleton, J. J., and J. E. DeVay. 1986. Soil solarization: A non-chemical approach for management of plant pathogens and pests. Crop Protection 5:190–98. doi:10.1016/0261-2194(86)90101-8.
- Steinmetz, Z., C. Wollmann, M. Schaefer, C. Buchmann, J. David, J. Tröger, K. Muñoz, O. Frör, and G. E. Schaumann. 2016. Plastic mulching in agriculture: Trading short-term agronomic benefits for long-term soil degradation? Science of the Total Environment 550:690–705. doi:10.1016/j.scitotenv.2016.01.153.
- Teague, R., F. Provenza, U. Kreuter, T. Steffens, and M. Barnes. 2013. Multi-paddock grazing on rangelands: Why the perceptual dichotomy between research results and rancher experience? Journal of Environmental Management 128:699–717. doi:10.1016/j.jenvman.2013.05.064.
- Tilman, D. 1998. The greening of the Green Revolution. Nature 396:211–12. doi:10.1038/24254.
- Travlos, I., I. Gazoulis, P. Kanatas, A. Tsekoura, S. Zannopoulos, and P. Papastylianou. 2020. Key factors affecting weed seeds’ germination, weed emergence, and their possible role for the efficacy of false seedbed technique as weed management practice. Frontiers in Agronomy. doi:10.3389/fagro.2020.00001.
- Tscharntke, T., Y. Clough, T. C. Wanger, L. Jackson, I. Motzke, I. Perfecto, J. Vandermeer, and A. Whitbread. 2012. Global food security, biodiversity conservation and the future of agricultural intensification. Biological Conservation 151:53–59. doi:10.1016/j.biocon.2012.01.068.
- Utter, A., A. White, V. E. Méndez, and K. Morris. 2021. Co-creation of knowledge in agroecology. Elementa: Science of the Anthropocene 9:00026. doi:10.1525/elementa.2021.00026.
- Vandermeer, J. 1995. The ecological basis of alternative agriculture. Annual Review of Ecology and Systematics 26:201–24. doi:10.1146/annurev.es.26.110195.001221.
- van Zonneveld, M., M.-S. Turmel, and J. Hellin. 2020. Decision-making to diversify farm systems for climate change adaptation. Frontiers in Sustainable Food Systems 4: doi: 10.3389/fsufs.2020.00032.
- Wezel, A., S. Bellon, T. Doré, C. Francis, D. Vallod, and C. David. 2009. Agroecology as a science, a movement and a practice. A review. Agronomy for Sustainable Development 29:503–15. doi:10.1051/agro/2009004.
- Whitmer, A., L. Ogden, J. Lawton, P. Sturner, P. M. Groffman, L. Schneider, D. Hart, B. Halpern, W. Schlesinger, S. Raciti, et al. 2010. The engaged university: Providing a platform for research that transforms society. Frontiers in Ecology and the Environment 8:314–21. doi:10.1890/090241.
- Yan, A., and Z. Chen. 2020. The control of seed dormancy and germination by temperature, light and nitrate. Botanical Review 86:39–75. doi:10.1007/s12229-020-09220-4.
- Zimmerman, C. A. 1976. Growth characteristics of weediness in Portulaca oleracea L. Ecology 57:964–74. doi:10.2307/1941061.