ABSTRACT
This study aims to produce evidence on the performance of horticultural systems in the peri-urban area of Buenos Aires (Argentina), based on the 10 Elements of Agroecology with an emphasis on soil health. To this end, we performed a survey with the Tool for Agroecology Performance Evaluation (TAPE) Step 1, and we measured soil physical, chemical, and biological properties in family farms self-defined as agroecological or conventional. Results reflected overall low transition-to-agroecology indices and poor soil quality in the studied area. However, self-defined agroecological systems have made significant strides toward agroecological transition with higher scores mainly affecting Elements describing management and innovation aspects of the agroecological transition but also those describing the social aspects and the enabling environment. Finally, self-defined agroecological farms exhibit better soil health scores for the indicators aggregates stability, soil organic carbon, and soil basal respiration, than conventional farms. Nevertheless, there is still potential to be exploited and constraints to be overcome to improve the transition to agroecology of horticultural systems in this area.
SUSTAINABLE DEVELOPMENT GOALS:
Introduction
In nearby urban areas, peri-urban horticulture not only fulfills environmental and socio-economic functions but also provides ecological services (Larcher Citation2017). Food production in these areas is essential for maintaining the resilience of cities (Drescher et al. Citation2021), especially in times of crisis. Particularly, the COVID-19 pandemic triggered concern about food availability along the food supply chain (Deaton and Deaton Citation2020) and highlighted the key role played by local food systems and value chains in times of crisis (Tittonell et al. Citation2021), indicating the need to maintain viable rural and peri-urban areas. However, agricultural intensification practices, which involve high levels of nonrenewable inputs, have often been associated with environmental damage in peri-urban areas around the world. Some examples include degraded ecosystem functions (Emmerson et al. Citation2016), widespread land degradation (Kopittke et al. Citation2019), and soil, water, or food contamination with pesticides (Mac Loughlin, Peluso, and Marino Citation2022), antimicrobials (Li et al. Citation2015; Margenat et al. Citation2018), and antimicrobial resistant bacteria (Pellegrini et al. Citation2022). Therefore, there is a need for improved management practices to overcome the negative impacts of agricultural intensification in peri-urban areas.
Agroecology is an alternative and systemic approach for sustainable intensification that is based on ecological principles, local farmers’ experiences, and social aspects of food systems (HLPE Citation2019). It opposes excessive use of agrochemicals and is considered a science, a set of agricultural practices and a social movement (Wezel et al. Citation2009). Agroecology has been found to have positive impacts on different levels, including the environment (Modernel et al. Citation2018), food security (Madsen et al. Citation2021), nutrition (Luna-González and Sorensen Citation2018) and household income (D’Annolfo et al. Citation2017). Such is the scope of agroecology that the Food and Agriculture Organization (FAO) promotes it as a way to address world hunger, while maintaining the correct functioning of ecosystems. The FAO identified upscaling agroecology worldwide as a top priority for achieving the Sustainable Development Goals (SDGs) by 2030 (FAO Citation2018b). Maintaining and enhancing soil health is a critical aspect of this effort, as it provides sustainable solutions to global challenges and contributes to achieving multiple SDGs (Lal et al. Citation2021). Moreover, soil health is recognized as one of the consolidated 13 principles that define agroecology by the High-Level Panel of Experts on Food Security and Nutrition (HLPE Citation2019), and one of the 10 core criteria of performance for measuring the performance of agroecology by FAO (Citation2019).
A healthy soil has the ability to sustain biological production, maintain environmental quality, and promote plant and animal health, thus ensuring food supply within ecosystem boundaries (Doran and Safley Citation2002; Kibblewhite, Ritz, and Swift Citation2008). However, this ability is affected by land use strategies and land management practices. One of the most significant risks to the availability and stability of the food supply is soil degradation brought on by changes in land use and poor management practices (Gourdji et al. Citation2013; Lobell, Schlenker, and Costa-Roberts Citation2011). In contrast, agroecological and sustainable intensification approaches contribute to regenerative processes (Pretty et al. Citation2018) by improving long-term soil health through organic matter management and enhanced soil biological activity (HLPE Citation2019). Therefore, soil health knowledge is becoming more and more important in designing sustainable agri-food systems and establishing soil conservation policies in different agricultural production systems.
In Argentina, the peri-urban areas of Buenos Aires and La Plata are the main suppliers of fresh vegetables. These vegetables are mainly grown by family farmers that rent small parcels of land, usually less than 5 ha in size, with up to 1.5 ha of greenhouse farming (Gómez, Mediavilla, and Pineda Citation2013). The main destination of these products is the domestic market, making horticulture a crucial activity for the local economy. However, the most common management system used in these areas is conventional, involving intensive use of natural resources, excessive use of external inputs and limited access to professional agronomic advice (Fernández Lozano Citation2012). As a result, natural resources have been degraded and pollution has increased. Previous studies in this area identified signs of soil degradation (Paladino et al. Citation2018), including soil alkalization, incipient salinity, loss of organic carbon and P overfertilization. Nutrient runoff is affecting the quality of local streams and groundwater (Salvioli et al. Citation2012), while the pesticides used are a major threat to the small streams and their biodiversity (Mac Loughlin, Peluso, and Marino Citation2017, Citation2022). Much like environmental pollution, signs of occupational exposure to pesticides have been reported for many years (Fitó Friedrichs et al. Citation2020; Matos et al. Citation1987). Moreover, in these farms, chicken litter is commonly used as an organic soil additive. Chicken litter from intensive farming not only provides nutrients but also introduces salts and other contaminants to the soil, while increasing pH levels. For example, recent findings (Prack McCormick et al. Citation2020) revealed that lettuce grown in soils fertilized with composted chicken litter from intensive animal production has fluoroquinolone residues.
Amid these challenges, agroecological farming is gaining attention as a sustainable alternative to ensure food security in the peri-urban area of Buenos Aires. Some farmers are adopting agroecological practices, and new marketing channels, such as farmers-to-consumers markets and delivery of seasonal vegetable bags as means for promoting their products. Unlike organic farming, there is no certification process to verify the agroecological performance of a self-defined agroecological system. Therefore, little is known about how agroecological these systems are, and if their performance is positively affecting soil health and making horticulture more sustainable. To address this gap worldwide, the Tool for Agroecology Performance Evaluation (TAPE) was developed to generate global and comparable evidence on the multidimensional performance of agroecology in any kind of production system in agriculture, hence it is also applicable to horticultural systems. TAPE comprises two main Steps: Step 1, the characterization of the agroecological transition (CAET), based on the 10 Elements of Agroecology defined by FAO (Citation2019) which allows characterization and comparing of levels of transition toward agroecology; and Step 2, which assesses performance based on 10 core criteria of performance of agroecology, where soil health is included (Mottet et al. Citation2020). TAPE was recently used in Argentina to assess the performance of horticulture in the peri-urban area of Rosario City (Lucantoni et al. Citation2022).
The present study aims to (i) characterize the level of transition to agroecology of 19 horticultural farms, self-defined as agroecological or conventional, based on the 10 Elements of Agroecology using TAPE Step 1 (CAET), and (ii) to investigate if self-defined agroecological systems have better soil health than conventional systems at physical, chemical, and biological levels. We hypothesize that self-defined agroecological systems are more advanced in agroecological transition than conventional systems and that they have better scores of soil health.
Materials and methods
Site description
This study included an area of horticultural farming taking place in Florencio Varela district, located in the south of the Green Belt of Buenos Aires, Argentina (). A total of 19 family farms under agroecological and conventional management were included in the evaluation with TAPE. The farms were classified as self-defined agroecological (A) or conventional (C) based on the self-perception of the farmer.
The climate in the area is temperate-humid without a pronounced dry season, with hot summers and temperate winters. The average annual temperature is 16°C and the frost-free period is 220 days. Irrigation is complementary in open-field cultivation systems, since rainfall in the area is between 900 and 1000 mm year−1 distributed uniformly in the four seasons (FAO Citation2019). The geomorphology of Florencio Varela corresponds to the Rolling Pampa region, with terrain characterized by gentle undulations of long and not very steep slopes. The soils are typic Argiudol (Soil Survey staff Citation2010), located in well-drained areas and have developed profiles, with deep and dark A horizons, well supplied with organic matter. Underlying B horizons have strong features of clay migration and the presence of expandable clays. Soil texture is classified as silty clay loam. Although these soils have a high productive capacity, the clay provides moderate to low permeability with high plasticity and adhesiveness, mainly in B horizons (Giménez et al. Citation1992).
Most farmers rent their land and have open field and greenhouse production (). The water used for irrigation comes from water wells, 55–60 m deep, drilled within the farms. The most common system of irrigation is drip, while fertilization is mostly performed by fertigation (Cuellas Citation2017).
Figure 2. (a) Representative diagram of the horticultural farms. (b) Representative images of sites sampled within each farm: 1) un-cultivated soils, 2) soils from open-field production and 3) soils from greenhouse production.
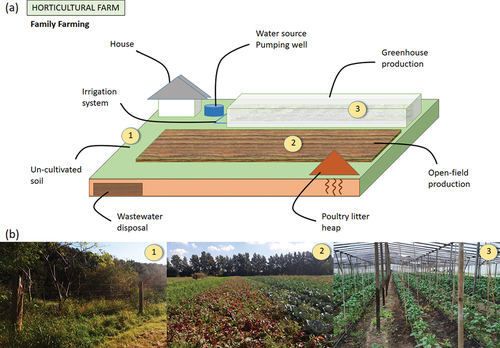
Poultry litter is applied in every farm at each cycle or every other cycle depending on the season and on the vegetable grown, and it comes from nearby facilities of intensive poultry production. The main vegetables grown in open field are, in order of importance, lettuce, chard, spinach, tomato, pepper, broccoli and cabbage, while those grown in greenhouses are tomato, spinach, lettuce and pepper (Rivas Citation2010).
Characterization of the agroecological transition (CAET)
Among the farmers included in this study, 11 self-perceived their farming systems as agroecological and eight as conventional. All the farms have more than 30 years under horticultural production. Farms classified as self-defined agroecological (A) were initially conventional and since 2011, they have been in the process of transition to agroecology. To answer the question ”How agroecological is the production system?” we performed a survey based on TAPE’s Step 1, the Characterization of the Agroecological Transition (CAET) (FAO Citation2019) based on the 10 Elements of Agroecology (FAO Citation2018a). The Elements of Diversity, Resilience, Synergies, Efficiency, and Recycling characterize the management practices and the innovation criteria implemented on the farm, while, social aspects and the enabling environment characteristics are addressed through the Elements of Human and Social Values, Culture and Food Traditions, Circular and Solidarity Economy, Co-creation and Sharing of Knowledge, and Responsible Governance. Each Element is measured through 3 or 4 indices on a descriptive scale from 0 (the least agroecological situation) to 4 (the most agroecological one), depending on the characteristics of the farm under study. As a result of this scoring, the percentage of advancement of each Element is obtained, as well as an aggregated CAET score, which is the overall level of agroecological transition on the farm assessed (Mottet et al. Citation2020). More details about CAET and the indices that describe each Element are detailed in FAO (Citation2019).
Soil health characterization
We collected soil samples from productive plots at each horticultural farm assessed in Step 1 (except for one conventional and one agroecological), from 0 to 10 cm depth to evaluate soil physical, chemical, and biological quality. The plots sampled were classified based on the approach and the cultivation system. On the one hand, the approach included plots under conventional (C; n = 10) or self-defined agroecological (A; n = 17) production. On the other hand, the cultivation system included open-field production (OF; n = 16) and greenhouse production (GH; n = 11).
In the conventional approach (C), agrochemicals including biocides and synthetic fertilizers are applied regularly. Chicken litter is incorporated into the soil at pre-sowing, while synthetic fertilizers (such as di-ammonium phosphate and NPK 15-15-15) are applied through fertigation during the sowing and on a weekly base during cultivation, hence covering the nutritional requirements. In the agroecological approach (A), the use of external inputs is reduced mainly to the poultry litter. Biocides are replaced by the use of purines, plant extracts, diatomaceous earth or increased plant diversity. Open-field production (OF) is performed in furrows in open plots of about 3000 m2 while greenhouse production (GH) is performed in wood and polyethylene gable greenhouses of 15 m × 80 m (1200 m2). In both cultivation systems, a layer of 10 mm of chicken litter is applied and incorporated with a motocultivator before sowing as an organic amendment and source of nutrients ().
Table 1. Characterization of nine poultry litter heaps present in the farms at sampling.
We also collected soil samples from uncultivated sites (NC) identified within most of the farms. Those sites were neither tilled nor used for vegetable production or poultry litter storage, to the best knowledge of the current farmer (at least 5 years). Uncultivated soil samples were used as a reference of soil quality before land use change into horticulture. summarizes the physical, chemical, and biological properties of the samples taken from the uncultivated sites.
Table 2. Characterization of uncultivated soils in the studied area from 0 to 10 cm depth.
Soil physical properties
For this study, we selected the following soil physical properties as indicators of soil physical health: bulk density (BD), gravimetric moisture (GM) and aggregate stability (AS). For these properties, three discrete soil samples were analyzed for each experimental unit. BD was determined by the cylinder method (Blake and Hartge Citation1986); GM by weight difference (Gardner Citation1986); and AS by le Bissonnais (Citation1996). Briefly, this latter method consists in exposing soil aggregates of between 3 and 5 mm to three pre-treatments to calculate the Mean Weight Diameter (MWD) of stable aggregates. The pre-treatments were as follows: fast wetting (ASfw), which is the immersion of aggregates in water to study the dry soil behavior in the face of a heavy rain like storms in summer; slow wetting (ASsw) which corresponds to a field condition of wetting under gentle rain like dry soil behavior in the face of mild rain; and stirring after pre-wetting (ASst) which is the immersion of aggregates in ethanol to test the wet mechanical cohesion of aggregates independently of slaking to study wet soil behavior in the face of a heavy rain.
Soil chemical properties
We selected the following soil chemical properties as indicators of soil chemical health: pH, electrical conductivity (EC), soil organic carbon (SOC), extractable phosphorus (EP) and total nitrogen (TN). For these properties, we analyzed one soil sample composed of 16–20 subsamples for each experimental unit. Once collected, the samples were air-dried and passed through a 2000 µm sieve. All determinations were made in triplicate. pH and EC were measured in a 1:2.5 (soil: deionized water) weight ratio, using a Hanna pH 211 microprocessor with the Hanna HI1331B pH electrode and an Orion Conductivity meter (Model 120) with the Orion conductivity cell 012010, respectively. SOC was determined according to the procedure proposed by Walkley & Black (Jackson Citation1964); EP, using the extraction method proposed by Bray & Kurtz, according to NORMA IRAM (Citation2010); and NT following the Semi-Micro Kjeldahl method according to SAMLA (Citation2004).
Soil biological property
We studied soil microbial activity through soil basal respiration (SBR), as an indicator of soil biological health. CO2 release was determined by the alkali absorption method followed by titration (Zibilske Citation1994). The method consisted in incubating 50 g of soil at 22°C for seven days in hermetic containers, by triplicate. On day seven, a tube with 25 ml of 0.25 N NaOH (the CO2 trap)was placed inside each hermetic container, and the system was incubated at 28°C for seven days more. The negative control consisted of the NaOH solution placed in a container without soil. To determine the release of CO2, 5 ml of the NaOH solution, with 3 ml of 3 N BaCl2 and 2–3 drops of phenolphthalein, were titrated with 0.25 N HCl. The result was expressed as mg of CO2 released per soil kg per day.
Statistical analyses
To compare the level of the agroecological transition between self-defined A and C, we applied the non-parametric Wilcoxon test (Mann–Whitney U) to the results from CAET (Elements and indices within Elements) considering a p < 0.05.
To assess overall soil health, we performed a principal components analysis (PCA) for every soil property measured, using the R program (R Project version 3.5.1) and the package FactoShiny. To test the effect of the two qualitative variables (factors), approach and the cultivation system or their interaction, on each of the soil properties, a variance analysis was applied using the statistical program InfoStat (di Rienzo et al. Citation2008). Significantly different means were separated using the Tukey test (p < 0.05) or the non-parametric test Kruskal Wallis (p < 0.05), and correlation between variables was calculated using Pearson’s correlation coefficients (Pearson r).
Results
Characterization of the agroecological transition (CAET)
The CAET score for the complete set of cases evaluated was 46%. The results reflect a higher level of agroecological transition in self-defined agroecological (A) systems than in the conventional (C) systems, with CAET scores of 52% (41–71) and 39% (31–57), respectively.
When analyzing the differences across the 10 Elements, Resilience, Synergies, Efficiency, Recycling, Culture and Food Traditions, and Co-creation and Sharing of Knowledge presented the most significant differences between A and C (p < 0.05, non-parametric Wilcoxon test) (). Secondly, the Elements of Diversity, Circular and Solidarity Economy, and Responsible Governance presented higher scores in A than C, however these differences were not yet statistically significant (p > 0.05, non-parametric Wilcoxon test). Finally, both A and C systems presented the same score for the Element of Human and Social Values ().
Figure 3. Mean scores of the 10 Elements of agroecology for self-defined agroecological (gray; n=11) and conventional (black; n=8) systems. Statistically significant differences: *p<0.05, **p<0.01 (non-parametric Wilcoxon test).
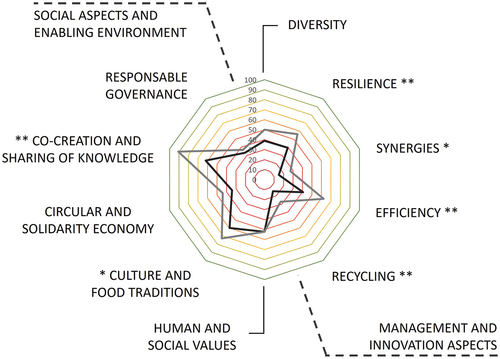
Management and innovation aspects of agroecological transitions
When zooming in on the CAET indices, between the 20 indices describing the management and innovation aspects of agroecological transitions, nine (45%) showed significantly higher scores in A compared to C, involving each of the Elements within this category (). In terms of the Diversity Element (), there were no differences between A and C regarding the overall index, but the animals index was significantly higher in A over C. The Resilience Element also showed higher scores in A, with greater stability in income and production, as well as reduced indebtedness (). The Efficiency Element had the greatest transition within the management and innovation aspects. While productivity and household’s needs index levels were similar between A and C, the A systems showed progress in the management of soil fertility and pests and diseases, as well as greater efficiency in the use of external inputs compared to the C systems (). For the Synergy Element (), we found a higher integration of trees and other perennials in the agroecosystem in A compared to C. Finally,A systems had higher recycling of nutrients and biomass than C, as well as better management of seeds and breeds for the Recycling Element ().
Figure 4. Mean scores for the indices corresponding to agroecology Elements describing management and innovation aspects for self-defined agroecological (gray; n=11) and conventional (black; n=8) systems. (a) Diversity, (b) Resilience, (c) Synergies, (d) Efficiency, and (e) Recycling. ns: non-significant, *p<0.05, **p<0.01 (non-parametric Wilcoxon test).
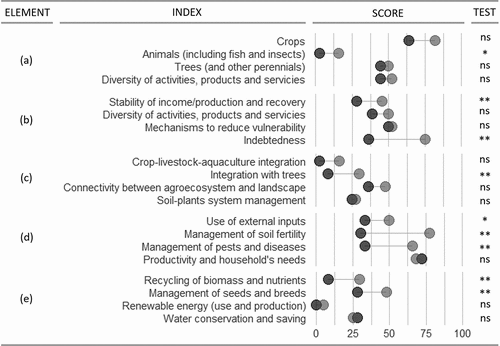
Social and enabling environment aspects of agroecological transitions
Within the social and enabling environmental aspects, between the 16 indices, three (19%) showed significantly higher scores in A compared to C, while one (6%) showed a lower score in A than in C (). For the overall Human and Social Values Element, no differences were found between A and C. However, the index women’s empowerment was the only index that showed a lower score in A compared to C (). We found a higher score in A than C for the index appropriate diet and nutritional awareness in the Culture and Food Tradition Element (). None of the indices from the Elements Circular and Solidarity Economy, and Responsible Governance had significant differences between A and C ( and respectively). However, a tendency for higher scores in group A than C was observed for networks, intermediaries, and consumers, and local food systems (). Finally, Co-creation and Sharing of Knowledge was the Element with the greatest transition progress and the highest and most remarkable difference between A and C (). We found higher scores in A systems for the indices access to and interest in agroecological knowledge as well as in participation in networks and grassroots organization ().
Figure 5. Mean scores for the indices corresponding to agroecology Elements describing the social aspects and enabling environment for self-defined agroecological (gray; n=11) and conventional (black; n=8) systems. (a) Human and social Values, (b) Culture and food Traditions, (c) Circular and Solidarity Economy, (d) Co-creation and Sharing of knowledge, and (e) Responsible Governance. ns: non-significant, *p<0.05 (non-parametric Wilcoxon test).
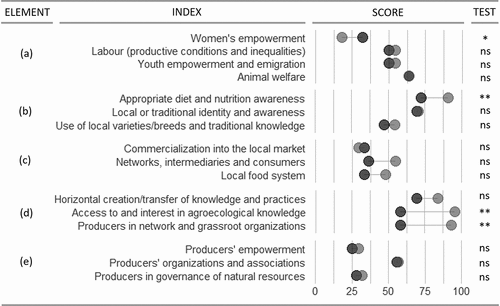
Soil health characterization
First, a descriptive analysis of soil properties was carried out through a PCA, thus obtaining an approximation of the correlation between soil properties and the qualitative variables cultivation system and approach. Then, a variance analysis showed the interaction between approach and cultivation system and their effects on each soil property. Particularly, pH and electric conductivity did not pass the normality and heteroscedasticity tests; therefore, non-parametric variance analysis was applied.
Overall variability
The first two primary components of the PCA managed to explain 60.15% of the total system variability. The third component added 11.54% to the total variability. Most of the variables evaluated defined at least one of the first two principal components. On the one hand, aggregate stability (ASfw, ASst and ASsw), pH, soil organic carbon, extractable P, total N and soil basal respiration, were the variables that defined the first component ( and ). On the other hand, gravimetric moisture, electric conductivity, extractable P and total N, defined the second component. Each of these variables had a significant correlation (p < 0.05) with the principal component it defined. Finally, bulk density was the only variable with no contribution to nor correlation with the first two principal components.
Figure 6. First factorial plane of a principal components analysis. (a) variables factor map, each vector represents a variable and the angles between variables or components indicate the correlation between them; (b) individuals factor map; (c) qualitative factor map and (d) individuals factor map where individuals are color after their category for the variable approach. Dim1: first principal component, Dim2: second principal component, BD: bulk density, GM: gravimetric moisture, ASfw: aggregates stability fast wetting, ASst: aggregates stability stirring after pre-wetting, ASsw: aggregates stability slow wetting, pH: potential hydrogen, EC: electrical conductivity; SOC: soil organic carbon, EP: extractable phosphorus, TN: total nitrogen, SBR: soil basal respiration. OF: open field production; OFC: open field conventional, OFA: open field self-defined agroecological, A: agroecological approach, C: conventional approach, GH: greenhouse production, GHC: greenhouse production conventional and GHA: greenhouse production self-defined agroecological.
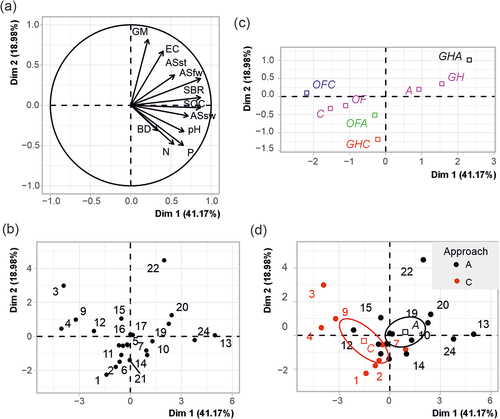
Table 3. Pearson’s correlation between the variables and the first two principal components.
When analyzing correlations between properties, we found that soil biological respiration positively correlated with both physical and chemical soil properties. On the one hand, regarding physical properties, respiration correlated with each variable related to aggregate stability (Pearson r: ASfw: 0.76; ASst: 0.43; ASsw: 0.63; p < 0.05). On the other hand, regarding chemical properties, it correlated with pH (Pearson r: 0.55, p < 0.01), soil organic carbon (Pearson r: 0.69; p < 0.01), extractable P (Pearson r: 0.47, p < 0.05) and total N (Pearson r: 0.49, p < 0.05).
Finally, according to the Wilks test p-value, the best qualitative variable to illustrate the distance between individuals on the first plane is the interaction between System and Approach (p = 4.40 e-05). Moreover, while Dim 1 had a positive correlation with the cultivation system (R2 = 0.38, p < 0.01) and the approach (R2 = 0.31, p < 0.01), the highest correlation was found for their interaction (System × Approach R2 = 0.62, p < 0.001) (, and ).
Physical indicators of soil health
Physical properties of the soil varied only depending on the approach or its interaction with the cultivation system. Concerning the approach, statistically significant differences (p < 0.05) were found for ASst. The plots under A presented higher aggregate stability than those under C (). Concerning the interaction between the approach and the cultivation system, statistically significant differences (p < 0.05) were found for ASfw (). More stable soils were observed when combining the cultivation system GH with the A approach, while the less stable corresponded to OF and C. Finally, the cultivation system alone did not affect any of the physical properties assessed.
Figure 7. Aggregate stability for the pre-treatment fast wetting; interaction between approach and cultivation system. MWD: mean weight diameter, OF: open-field production; GH: greenhouse production, C: conventional approach, A: agroecological approach. Different letters show significant differences between uses according to Tukey (p < 0.05). The vertical lines indicated the standard deviation.
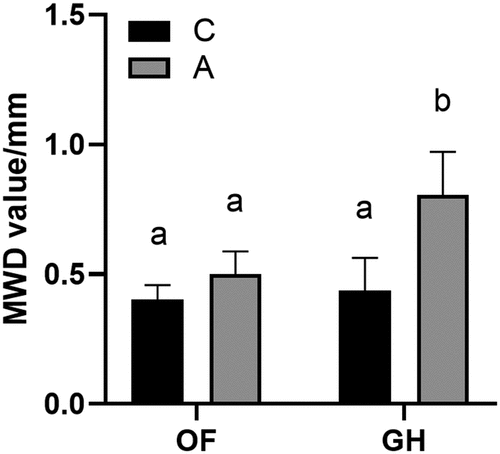
Table 4. Physical indicators of soil health without interaction between cultivation system and approach.
Chemical indicators of soil health
Chemical properties of the soil varied depending on the cultivation system and the approach. Concerning the cultivation system, statistically significant differences (p < 0.05) were found for pH, electric conductivity, and extractable P. The plots under GH presented higher levels of these three properties than OF plots (). Concerning the approach, statistically significant differences (p < 0.05) were found for soil organic carbon. Higher levels of carbon corresponded to A while lower levels corresponded to C.
Table 5. Chemical indicators of soil health, from 0 to 10 cm depth.
Biological indicators of soil health
The biological property soil basal respiration varied depending on both the cultivation system and the approach (p < 0.05). Concerning the cultivation system, plots under GH had higher soil biological respiration than plots under OF. Concerning the approach, plots under A had higher soil biological respiration than those under C ().
Table 6. Biological indicators of soil health.
Discussion
The strong specialization in vegetable production coupled with intensive agricultural practices in the green belt of Buenos Aires is reflected in generally low CAET scores (overall average of 46%), and poor soil quality. Cultivated soils have turned alkaline and have accumulated salts and P while they have lost aggregate stability, soil organic carbon, N, and soil biological activity when compared with local uncultivated soils (). Over the last decade, the interest in agroecology as a healthier and fairer way to achieve food sovereignty has grown in urban and peri-urban areas from Argentina and around the world (Einbinder et al. Citation2021; Siegner, Acey, and Sowerwine Citation2020; Simon-Rojo, Bernardos, and Landaluze Citation2018), reaching farmers, consumers, technicians, and scientists. Through this study, we give evidence that self-defined agroecological systems are advancing in the transition to agroecology with higher scores than conventional systems in Elements involving (1) management and innovation aspects of agroecological transitions as well as (2) social aspects and enabling environment. Moreover, these higher scores are coupled with better soil health observed at physical, chemical, and biological levels. Nevertheless, given the yet low level of agroecological transition, there is still much potential to be exploited and constraints to be overcome to improve the sustainability of these horticultural systems.
Characterization of the agroecological transition
Our study shows that the agricultural systems we examined in the green belt of Buenos Aires are still in the early stages of the transition to agroecology, with generally low CAET scores compared to other regions of Argentina (Álvarez et al. Citation2019; Lucantoni et al. Citation2022). For example, Álvarez et al. (Citation2019) reported that horticulture in Northern Patagonia has an average CAET value of 65% (with a range of 48–69%). However, it is important to consider that agricultural systems in Patagonia are characterized by productive diversification and strongly influenced by indigenous/peasant heritage and traditional management practices, which are similar to agroecological practices, as well as by the high biodiversity of the natural surroundings. This contrasts with the green belt of Buenos Aires, within a metropolitan area, characterized by decades of intensive production and home to almost half of Argentina’s population. Compared to Rosario, another major metropolitan area, the overall differences become less pronounced, but some Elements still differ between the two areas (Lucantoni et al. Citation2022).
Despite the low general CAET scores found in our study, self-defined agroecological systems presented higher scores than conventional systems for all of the Elements except one (with equal score), particularly those describing the management and innovation aspects of agroecological transitions. Such is the case for the Elements of Resilience, Synergies, Efficiency, and Recycling, while Diversity has yet to be improved. Within this aspect, the highest score was registered for Efficiency while Synergies and Recycling were the ones with the lowest scores. Efficiency is influenced by some of the main changes in management practices applied to the land by self-defined agroecological farmers, such as nonuse of inorganic fertilizers or pesticides. According to Lucantoni et al. (Citation2022), Efficiency had a four-fold change when comparing one representative conventional horticultural system with an agroecological one in Rosario. On the other hand, the low levels of transition accounted for Synergies and Recycling are likely influenced by the still high specialization in vegetable cropping, where diversity of crops for the market tends to be common, but the inclusion of multispecies green-manure, trees and animals within the agroecosystem is rare. As a result, there is a small opportunity for integration, a high export of the biomass produced and a low recycling rate of resources on-farm, all known causes of soil health loss (de Boer and van Ittersum Citation2018; Du et al. Citation2022). Nonetheless, our results show that self-defined agroecological systems have higher levels of tree integration, and nutrients and biomass recycling than conventional ones, but there is still much potential to be explored. Higher Resilience was also observed in terms of the indices of stability of income/production and recovery, and indebtedness. In Rosario, no significant differences were observed in Resilience, yet, the authors used the indices presented by Mottet et al. (Citation2020) where the index indebtedness is excluded.
Self-defined Agroecological systems also presented higher scores in the Elements describing the social aspects and enabling environment for agroecological transitions. Co-creation and Sharing of Knowledge is the Element with the highest transition progress within the 10 Elements. We attribute this result to positive interactions between these farm systems and institutions promoting agroecology as Universities and the National Institute of Agricultural Technology (INTA) in Argentina, as well as the participation in farmers’ organizations. Regarding the Element Culture and Food Traditions, it is noteworthy that self-defined agroecological systems scored higher for the index for appropriate diet and nutrition awareness compared to conventional systems. There is increasing evidence supporting the idea that agroecology positively impacts household food security (Bezner Kerr et al. Citation2021; Lucantoni Citation2020; Madsen et al. Citation2021). In our study, it could be due to increased interest in healthy diets going hand in hand with an interest in sustainable production (Mie et al. Citation2017), and/or increased diversity and food supply of the production system that can be either consumed by the farmers (Luna-González and Sorensen Citation2018) or sold to make other food items more affordable (Azzarri et al. Citation2022).
Self-defined agroecological systems do not yet exhibit higher scores in the Elements of Responsible Governance, Circular and Solidarity Economy, and Human and Social Values. According to Lucantoni et al. (Citation2021), the former two Elements presented very low scores, with Responsible Governance scoring 20 points lower than in horticultural systems from Rosario, Argentina (Lucantoni et al. Citation2022). This suggests a more complex environment for farmers to undergo the agroecological transition in the peri-urban zone of Buenos Aires than in Rosario. For example, long distances to the city and consumers is making it necessary to involve different intermediaries for commercialization. This happens even for methods designed for agroecological systems as selling bags with season vegetables called “bolsones” (Skill, Passero, and Farhangi Citation2022) in cooperation with National Universities. Other examples include insecurity, the bad state of the roads which are mainly unpaved (dirt)roads, the low percentage of farmers owning the land, the perception of low inclusion farmers have on decisions involving governance of natural resources.
Human and Social Values, presented the same level of transition in self-defined agroecological systems as in conventional systems, with an overall score of 52%. We attribute this result to the combination of local conditions that affect all farmers similarly. In addition to those problems described on the previous paragraph, in this peri-urban area farmers work the land with very low mechanization, depending greatly on human power. So even though they are not employees – they are their own bosses-, their working conditions are hard. This, coupled with not owning the land, likely increases youth desire to emigrate from the farm and work in different activities.
The index women’s empowerment from the Element of Human and Social Value, only scored 24.4%. The level of horticultural feminization in this area is low, with three women at the head of their farms (27%) in self-defined agroecological systems, and only one in conventional systems (12.5%). Contrary to our expectations, self-defined agroecological systems presented lower scores for this index compared to conventional ones. We consider this might be a consequence of the alignment of feminism with agroecology as a movement (Seibert et al. Citation2010; Zaremba et al. Citation2021). The higher access and interest in agroecological knowledge as well as the participation in networks and grassroot organizations of self-defined agroecological farmers is likely rising awareness of gender inequalities. Access to evidence and knowledge about the social structures and gender norms that perpetuate these inequalities can increase the sensitivity when assessing empowerment, recognizing inequalities where groups without this knowledge would not (Figueroa Vélez and Vélez Ochoa Citation2021; García Jiménez, Cala Carrillo, and Trigo Sánchez Citation2016). Overall, it is important to focus more attention on addressing gender-related issues in these horticultural systems, and even contemplate a wider perspective including dissident genders as proposed by (Leslie Citation2017; Leslie, Wypler, and Bell Citation2019).
Soil health characterization
When addressing the second objective of this study, we found that self-defined agroecological systems exhibit better soil health scores than conventional systems. Similar results were reported by Lucantoni et al. (Citation2022) in agroecological systems from the metropolitan area of Rosario especially when compared with conventional systems. Soil health depends on the physical, chemical, and biological properties of the soil (Sassenrath et al. Citation2018). Soil management practices in agroecosystems can have short-term and long-term effects on these properties (Garcia et al. Citation2019; Prack McCormick et al. Citation2022; Sokolowski et al. Citation2020), affecting development, productivity, and nutritional quality of edible crops and, furthermore, agriculture sustainability (de la Fuente and Suarez Citation2008; Paladino et al. Citation2018). Our findings reveal decline in soil health in horticultural soils based on the soil properties of aggregate stability, pH, P, soil organic carbon and microbial activity. However, we observed positive changes in self-defined agroecological systems with regard to properties associated with carbon cycling, such as aggregate stability, soil organic carbon, and microbial activity, even though the level of agroecological transition (CAET) is still low. On the other hand, soil pH, and P vary with the cultivation system, but differences in these indicators have not yet been observed between conventional and self-defined agroecological systems. The discussion below will focus on these two groups of properties.
Horticultural soil aggregates from the area under study were unstable in the face of a heavy rain but their stability is higher in self-defined agroecological systems. Agroecological management practices improved the stability of wet and dry soil aggregates in the face of a heavy rain. The only indicator for which an interaction between the approach and the cultivation system was registered was dry aggregate stability in the face of a heavy rain, with the best condition (moderately stable) found in greenhouses from self-defined agroecological systems (le Bissonnais Citation1996). Aggregate stability depends on various factors such as clay content, mineralogy, dominant cations in the exchange complex (Buckman and Brady Citation1970; Duchaufour Citation1987; Hillel Citation1998) and management strategies affecting moisture content, soil organic carbon, plant roots, soil microbial activity (Garcia et al. Citation2019; Ramesh et al. Citation2019; Sokolowski et al. Citation2020). While clay content, mineralogy and moisture remain similar between plots in this study, management strategies affecting carbon and nutrient cycling through ecological strategies scored higher in self-defined agroecological systems, according to CAET. Soil organic carbon is crucial for aggregate stability (Garcia et al. Citation2019; Six et al. Citation2004), not only by improving formation but also by reducing sensitivity to breakdown, e.g., increasing aggregate hydrophobicity (Abiven, Menasseri, and Chenu Citation2009). Additionally, soil microorganisms can also improve aggregate formation by binding soil particles with extracellular polysaccharides, and fungal hyphae can physically attach soil particles together (Cosentino, Chenu, and le Bissonnais Citation2006; Lehmann et al. Citation2020). In turn, soil organic carbon and microorganisms are also protected within soil aggregates.
The peri-urban area studied had a low supply of soil organic carbon in all farms due to more than 40 years of intensive agriculture (Gall le and García Citation2010; Vázquez and Terminiello Citation2008). However, the adoption of agroecological management practices is being followed by improvements in soil organic carbon content. Conventional horticultural practices involve intensive tillage and the use of poultry litter as the only organic amendment, which is insufficient to restore the organic matter content to its natural state (Cuellas et al. Citation2018). Nonetheless, the agroecological transition is beginning to show promise, with increased soil plant cover resulting from intercropping, reduced weeding, and fallow periods with spontaneous vegetation. These practices improve soil temperature, exudate diversity, and soil organic carbon stocks (de Tombeur et al. Citation2018; Li et al. Citation2015; Manpoong et al. Citation2021), as well as higher organic inputs and greater incorporation of residues. Additionally, the absence of inorganic nitrogen fertilization in self-defined agroecological systems can extend the half-life of crop residues with high C:N ratios.
Soil microbial activity is an essential component for the productivity and stability of agroecosystems. Basal respiration, which reflects microbial activity, can improve with the absence of soil tillage (Zhang et al. Citation2016), continuous presence of diverse plants and their roots and exudates (Bonkowski Citation2004; Griffiths et al. Citation2022; Steinauer, Chatzinotas, and Eisenhauer Citation2016), higher levels of soil organic carbon (Chen et al. Citation2017), neutral soil pH, and the absence of pollutants and agrochemicals (Fuller Citation2004). In this study, the transition to agroecological practices has led to an increase in microbial activity, accompanied by higher soil organic carbon levels. While the microbial activity and soil organic carbon levels in self-defined agroecological systems have not yet reached levels equivalent to uncultivated soils (), the paired increase in both is likely indicative of a certain ecosystem balance. Continued efforts to improve these aspects should be a priority in agroecological systems.
As part of the transition toward agroecology, some crucial soil properties, such as pH and P, which strongly influence food and environmental quality, are yet to be improved. Both properties were found to be well above their optimal range, and their values were higher in soils from GH compared to those from OF, as previously reported (Paladino et al. Citation2018). On the one hand, soil pH regulates nutrient availability for plant growth. Particularly, Fe, Cu, Mn, Zn, and Ni are tightly bound to the soil at levels as those found in our study for soils under GH (Jackson et al. Citation2018). This can negatively affect nutrient content in edible tissues decreasing food quality (Hailu et al. Citation2015) and arousing other technological debates as the need for biofortification (El-Ramady et al. Citation2022). On the other hand, P is one of the major causes of surface water eutrophication. While P accumulation is commonly detected in soils receiving manure-based nutrients (Prack McCormick et al. Citation2022), levels found in this study are extremely high. When the retention capacity of the soil is exceeded, phosphorus can be released into the environment (Xu et al. Citation2022). It can travel with water during rainstorms when the soil is easily saturated with rainwater. Runoffs are formed and discharged into surface and ground waters (Lin et al. Citation2011). Moreover, when aggregate stability is unstable to fast wetting, as is the situation in the studied area, erosion increases the sediments in water flows (le Bissonnais Citation1996) and nutrients can travel attached to soil particles.
In the previous study by Paladino et al. (Citation2018), authors discuss water irrigation quality, inorganic fertilization, and poultry litter as possible causes of the measured soil alkalization, salinization, and/or P accumulation. For this study, we assessed poultry litter samples from the farms included and found them to be extremely alkaline and a rich source of P (). Although inorganic fertilizers are not used in self-defined agroecological systems, poultry litter is commonly used in both systems, making it a major contributor to these problems. It is key to reduce the dependency on poultry litter as the main organic amendment to overcome alkalinization and P accumulation.
The main limitation of this study is that we have sampled farmers’ productive plots, hence the results do not come from a field experiment with completely controlled treatments. However, they do represent real situations from the territory.
Recommendations and future studies needed
To continue with the agroecological transition, it is necessary to work jointly with the actors involved, and in line with local needs and the socioecological context toward beneficial synergies, as well as horizontal learning and teaching (Einbinder et al. Citation2022) without forgetting the impacts that this production has on soil health.
By working within the socioecological context of the horticultural systems of Florencio Varela it is important to analyze the Elements of (i) Diversity, Synergies and Recycling and (ii) Human and Social Values, Circular and Solidarity Economy, and Responsible Governance. These Elements have indices below 40 and it is important to determine if the cause of these scores is economic or organizational in nature. For example, land tenure is a major limiting factor in the study area generating instability and preventing investments in long-term benefits. We recommend the design of strategies that provide stability of land tenure, like long-term contracts or policies that allow land purchase by farmers. There is also a need for investment in roads, the design of strategies to guarantee security and appropriate insurances for climate-driven losses. Moreover, in-depth research should be directed to identify the causes of the surprisingly low score found for women’s empowerment.
To continue improving soil health, new practices can be explored and evaluated with farmers to replace the use of polluting or nonrenewable resources and reduce the need for tillage. A network between horticultural farmers and organic or agroecological animal farmers could be formed to improve the cycling of organic carbon and nutrients locally while mitigating risks associated with intensive animal production, such as antimicrobial resistance dissemination (Prack McCormick et al. Citation2022). Self-defined agroecological farmers express their dependency on tillage and plastic mulching to control the thriving summer weeds. Therefore, research on service crops including multi-species green manures and cover crops to provide an economical and soil-friendly alternative is needed. Their implementation could be an alternative to improve the agroecological transition (Hansen et al. Citation2021). Lastly, an in-depth study of the farmers’ practices and their impact on soil biology could help improve carbon and nutrient cycling.
Conclusion
Our main findings indicate that:
Self-defined agroecological systems have made more significant strides toward agroecological transition than conventional systems; and
Self-defined agroecological farms exhibit better soil health scores than conventional systems.
The characterization of the agroecological transition with TAPE indicates that horticultural farms in the peri-urban area of Florencio Varela, Buenos Aires (Argentina) have on average a low level of transition to agroecology. However, when classifying the systems based on the perception of the farmer, those systems self-defined as “agroecological” have a higher level of transition than those perceived as conventional.
Regarding the 10 Elements of Agroecology, higher scores in agroecological systems mainly involve management and innovation aspects and secondly social aspects and enabling environment. The former one includes the Elements of Resilience, Synergies, Efficiency, and Recycling; while the latter one includes, Culture and Food Traditions, and Co-creation and Sharing of Knowledge.
Regarding soil health, horticultural soils from the area present signs of degradation, highlighting low soil organic carbon and aggregate stability, yet extremely high pH and extractable P. Altogether these signs can affect production stability, environmental stability, and food quality. According to our results, on the one hand the agroecological approach improves aggregate stability of wet soil in the face of a heavy rain, soil organic carbon and soil microbial activity, independently of the cultivation system, i.e., open field or greenhouse production. Aggregate stability in the face of a heavy rain only improves under greenhouse and the agroecological approach. On the other hand, the level of agroecological transition is still not enough to alleviate neither soil alkalization nor P overfertilization, which do vary with the cultivation system.
In peri-urban horticulture from Florencio Varela, how the farmer perceives their system correlates with the performance of such system at different levels including management and innovation aspects, social aspects and enabling environment and soil health. While better performance is starting to be noticeable, there is still much to be done and requires the cooperation of all stakeholders.
Supplemental Material
Download MS Word (34.6 KB)Acknowledgments
We are grateful to the farmers from La Capilla and Villa San Luis, Florencio Varela (Argentina) for their participation and cooperation in the study. We are also grateful to BSc in Economy and Agricultural Engineering students, Pablo Astete Castro (Universidad de Buenos Aires), Juan Manuel Monaco, Evelyn Díaz, Facundo Gonzalez, Federico Manso, Francisco Larraburu, Hernan Rivera and Juan Manuel Gonzalez (Universidad Nacional de Lomas de Zamora) for their cooperation in data collection.
Disclosure statement
No potential conflict of interest was reported by the author(s).
Supplementary material
Supplemental data for this article can be accessed online at https://doi.org/10.1080/21683565.2023.2279972.
Additional information
Funding
References
- Abiven, S., S. Menasseri, and C. Chenu. 2009. The effects of organic inputs over time on soil aggregate stability – a literature analysis. Soil Biology & Biochemistry 41 (1):1–12. doi:10.1016/j.soilbio.2008.09.015.
- Álvarez, V. E., J. A. de Pascuale Bovi, S. M. Hara, A. Cardozo, and M. Paula Ocariz. 2019. Evaluación multidimensional de la agroecología en sistemas agropecuarios de Patagonia Norte. In 1er Congreso Argentino de Agroecología, 425–29. Argentina: Universidad Nacional de Cuyo.
- Azzarri, C., B. Haile, M. Letta, and G. Kruseman. 2022. Plant different, eat different? Insights from participatory agricultural research. PloS One 17 (3):e0265947. doi:10.1371/journal.pone.0265947.
- Bezner Kerr, R., S. Madsen, M. Stüber, J. Liebert, S. Enloe, N. Borghino, P. Parros, D. M. Mutyambai, M. Prudhon, and A. Wezel. 2021. Can agroecology improve food security and nutrition? A review. Global Food Security 29:100540. doi:10.1016/j.gfs.2021.100540.
- Blake, G. R., and H. Hartge. 1986. Bulk density. Methods of soil analysis, part 1. Physical and Mineralogical Methods. American Society of Agronomy (ASA) 9:365–75.
- Bonkowski, M. 2004. Protozoa and plant growth: The microbial loop in soil revisited. The New Phytologist 162 (3):617–31. doi:10.1111/j.1469-8137.2004.01066.x.
- Buckman, H. O., and N. C. Brady. 1970. Naturaleza y propiedades de los suelos: texto de Edafología para enseñanza. Barcelona: Montaner y Simon Editores.
- Chen, Z., Y. Xu, J. Fan, H. Yu, and W. Ding. 2017. Soil autotrophic and heterotrophic respiration in response to different N fertilization and environmental conditions from a cropland in Northeast China. Soil Biology and Biochemistry 110:103–115. doi:10.1016/j.soilbio.2017.03.011.
- Cosentino, D., C. Chenu, and Y. le Bissonnais. 2006. Aggregate stability and microbial community dynamics under drying–wetting cycles in a silt loam soil. Soil Biology and Biochemistry 38 (8):2053–62. doi:10.1016/j.soilbio.2005.12.022.
- Cuellas, M. V. 2017. Horticultura peri-urbana, análisis de la fertilidad de los suelos en Invernaderos. Chilean Journal of Agricultural & Animal Sciences 33 (2). doi:10.4067/s0719-38902017005000502.
- Cuellas, M. V., M. R. Delprino, M. E. D´angelcola, O. Valenzuela, J. Czepulis, K. Del Pardo, M. Ciaponi, and M. Mitidieri. 2018. BOLETÍN “MANEJO DE LOS SUELOS HORTIFLORÍCOLAS”, Vol. 10. Argentina: INTA.
- D’Annolfo, R., B. Gemmill-Herren, B. Graeub, and L. A. Garibaldi. 2017. A review of social and economic performance of agroecology. International Journal of Agricultural Sustainability 15 (6):632–44. doi:10.1080/14735903.2017.1398123.
- Deaton, B. J., and B. J. Deaton. 2020. Food security and Canada’s agricultural system challenged by COVID-19. Canadian Journal of Agricultural Economics 68 (2). doi:10.1111/cjag.12227.
- de Boer, I. J. M., and M. K. van Ittersum. 2018. Circularity in agricultural production. The Netherlands: Wageningen University & Research.
- de la Fuente, E., and S. Suarez. 2008. Problemas ambientales asociados a la actividad humana: la agricultura. In Ecologia Austral. Argentina: Asociacion Argentina de Ecologia.
- de Tombeur, F., V. Sohy, C. Chenu, G. Colinet, and J. T. Cornelis. 2018. Effects of permaculture practices on soil physicochemical properties and organic matter distribution in aggregates: A case study of the bec-hellouin farm (France). Frontiers in Environmental Science 6 (OCT). doi: 10.3389/fenvs.2018.00116.
- di Rienzo, J. A., F. Casanoves, M. G. Balzarini, L. Gonzalez, M. Tablada, and C. W. Robledo. 2008. InfoStat Versión 2011. Argentina: Grupo InfoStat, FCA, Universidad Nacional de Córdoba. https://www.researchgate.net/publication/233842986_InfoStat_Version_2011.
- Doran, J. W., and M. Safley. 2002. Defining and assessing soil health and sustainable productivity. In Biological indicators of soil health, ed. C. Pankhurst, B. M. Doube, and V. V. S. R. Gupta, 1–27. New York: CABI Publishing. doi:10.1098/rstb.2007.2178.
- Drescher, A. W., C. Isendahl, M. C. Cruz, H. Karg, and A. Menakanit. 2021. Urban and peri-urban agriculture in the global south. doi:10.1007/978-3-030-67650-6_12.
- Duchaufour, P. 1987. Manual de Edafología. Barcelona: Toray-Masson.
- Du, X., J. Jian, C. Du, and R. D. Stewart. 2022. International soil and water Conservation Research Conservation management decreases surface runoff and soil erosion. International Soil & Water Conservation Research 10 (2):188–96. doi:10.1016/j.iswcr.2021.08.001.
- Einbinder, N., H. Morales, and Mier y, B. G. Ferguson, M. Terán Giménez Cacho, B. G. Ferguson, M. Aldasoro, & R. Nigh. 2022. Agroecology from the ground up: A critical analysis of sustainable soil management in the highlands of Guatemala. Agriculture and Human Values 39 (3):979–96. doi:10.1007/s10460-022-10299-1.
- Einbinder, N., and H. Morales, y M. M. Terán Gimenéz Cacho, M. Aldasoro, B. Ferguson, & R. Nigh. 2021. Agroecología en la periferia: un caso del territorio maya-achí, Guatemala. Desenvolvimento e Meio Ambiente 58:58. doi:10.5380/dma.v58i0.81466.
- El-Ramady, H., P. Hajdú, G. Törős, K. Badgar, X. Llana, A. Kiss, N. Abdalla, A. E. D. Omara, T. Elsakhawy, H. Elbasiouny, et al. 2022. Plant Nutrition for Human Health: A Pictorial Review on Plant Bioactive Compounds for Sustainable Agriculture. Sustainability 14(14):8329. 2022,Vol. 14, Page 8329. doi:10.3390/SU14148329.
- Emmerson, M., M. B. Morales, J. J. Oñate, P. Batáry, F. Berendse, J. Liira, T. Aavik, I. Guerrero, R. Bommarco, S. Eggers, et al. 2016. How Agricultural Intensification Affects Biodiversity and Ecosystem Services 43–97. doi:10.1016/bs.aecr.2016.08.005.
- FAO. 2018a. The 10 Elements Of Agroecology Guiding The Transition To Sustainable Food And Agricultural Systems. Rome, Italy: FAO.
- FAO. (2018b). Indicators | Sustainable Development Goals | Food and Agriculture Organization of the United Nations. https://www.fao.org/sustainable-development-goals/indicators/en/
- FAO. 2019. TOOL for AGROECOLOGY PERFORMANCE EVALUATION PROCESS of DEVELOPMENT and GUIDELINES for APPLICATION. TEST VERSION. https://www.fao.org/3/ca7407en/ca7407en.pdf.
- Fernández Lozano, J. 2012. La produccion de hortalizas en Argentina. Buenos Aires, Argentina: Secretaria de Comercio Interior, Corporación Del Mercado Central de Buenos Aires.
- Figueroa Vélez, J., and S. Vélez Ochoa. 2021. Gender sensitivity and its relation to gender equality. In Gender Equality. Encyclopedia of the UN Sustainable Development Goals, ed.W. Leal Filho, A. M. Azul, L. Brandli, A. Lange Salvia, and T. Wall. Switzerland: Springer Nature. doi:10.1007/978-3-319-70060-1_46-1.
- Fitó Friedrichs, G., G. Berenstein, S. Nasello, Y. Y. Dutra Alcoba, E. A. Hughes, S. Basack, and J. M. Montserrat. 2020. Human exposure and mass balance distribution during procymidone application in horticultural greenhouses. Heliyon 6 (1):e03093. doi:10.1016/j.heliyon.2019.e03093.
- Fuller, M. E. 2004. Pollutants - effects on microorganisms. Encyclopedia of Soils in the Environment 4. doi:10.1016/B0-12-348530-4/00170-3.
- Gall le, J., and M. García. 2010. Reestructuraciones de las periferias hortícolas de Buenos Aires y modelos espaciales ¿Un archipiélago verde? EchoGéo (11):11. doi:10.4000/echogeo.11539.
- Garcia, L., G. Damour, C. Gary, S. Follain, Y. le Bissonnais, and A. Metay. 2019. Trait-based approach for agroecology: Contribution of service crop root traits to explain soil aggregate stability in vineyards. Plant and Soil 435 (1–2):1–14. doi:10.1007/s11104-018-3874-4.
- García Jiménez, M., M. J. Cala Carrillo, and M. E. Trigo Sánchez. 2016. Conocimiento y actitudes hacia el feminismo = Knowledge and attitudes toward feminism. FEMERIS: Revista Multidisciplinar de Estudios de Género 1 (1/2):95–112. doi:10.20318/femeris.2016.3229.
- Gardner, W. H. 1986. Water content. In Inc. Soil Science Society of America (Ed.), methods of Soil analysis, part 1: Physical and mineralogical methods, 493–544. American Society of Agronomy. doi:10.2136/sssabookser5.1.2ed.c21.
- Giménez, J., M. Hurtado, M. Cabral, and M. da Silva. 1992. Estudio de suelos del partido de La Plata, 180. Argentina: Instituto de Geomorfologia de Suelos.
- Gómez, C., M. C. Mediavilla, and C. Pineda. 2013. Organizaciones de productores Bolivianos en el Cinturon Horticola Platense y el desafio de alcanzar un modelo de produccion cada vez mas inclusivo y sustentable. In Migrantes bolivianos en el periurbano bonaerense, ed. M. C. Feito. Argentina: INTA.
- Gourdji, S. M., K. L. Mathews, M. Reynolds, J. Crossa, and D. B. Lobell. 2013. An assessment of wheat yield sensitivity and breeding gains in hot environments. Proceedings of the Royal Society B: Biological Sciences 280 (1752):20122190. doi:10.1098/rspb.2012.2190.
- Griffiths, M., B. M. Delory, V. Jawahir, K. M. Wong, G. C. Bagnall, T. G. Dowd, D. A. Nusinow, A. J. Miller, and C. N. Topp. 2022. Optimisation of root traits to provide enhanced ecosystem services in agricultural systems: A focus on cover crops. Plant, Cell & Environment 45 (3):751–70. doi:10.1111/pce.14247.
- Hailu, H., T. Mamo, R. Keskinen, E. Karltun, H. Gebrekidan, and T. Bekele. 2015. Soil fertility status and wheat nutrient content in Vertisol cropping systems of central highlands of Ethiopia. Agriculture & Food Security 4 (1). doi: 10.1186/s40066-015-0038-0.
- Hansen, V., J. Eriksen, L. S. Jensen, K. Thorup-Kristensen, and J. Magid. 2021. Towards integrated cover crop management: N, P and S release from aboveground and belowground residues. Agriculture, Ecosystems and Environment 313:313. doi:10.1016/j.agee.2021.107392.
- Hillel, D. 1998. Environmental soil physics: Fundamentals, applications, and environmental considerations. United States: Academic Press.
- HLPE. 2019. Agroecological and other innovative approaches for sustainable agriculture and food systems that enhance food security and nutrition. Report No. 14, Rome, Commitee on World Food Security.
- Jackson, M. (1964). Análisis químico de suelos. https://bibliotecadigital.infor.cl/handle/20.500.12220/181
- Jackson, K., T. T. Meetei, K. Jackson, and T. T. Meetei. 2018. Influence of soil pH on nutrient availability: A Review. JETIR 5 (12):707–13. https://www.jetir.org/view?paper=JETIRDZ06090.
- Kibblewhite, M. G., K. Ritz, and M. J. Swift. 2008. Soil health in agricultural systems. Philosophical Transactions of the Royal Society B: Biological Sciences 363 (1492):685–701. doi:10.1098/rstb.2007.2178.
- Kopittke, P. M., N. W. Menzies, P. Wang, B. A. McKenna, and E. Lombi. 2019. Soil and the intensification of agriculture for global food security. Environment International 132:105078. doi:10.1016/j.envint.2019.105078.
- Lal, R., J. Bouma, E. Brevik, L. Dawson, D. J. Field, B. Glaser, R. Hatano, A. E. Hartemink, T. Kosaki, B. Lascelles, et al. 2021. Soils and sustainable development goals of the United Nations: An International Union of soil Sciences perspective. Geoderma Regional 25:e00398. doi:10.1016/j.geodrs.2021.e00398.
- Larcher, F. 2017. Urban horticulture and ecosystem services: Challenges and opportunities for greening design and management. Italus Hortus 24 (24):33. doi:10.26353/j.itahort/2017.1.3339.
- le Bissonnais, Y. 1996. Aggregate stability and assessment of crustability and erodibility: 1. Theory and methodology. European Journal of Soil Science 47 (4):425–37. doi: 10.1111/ejss.2_12311. Reflections by Y. Le Bissonnais. In European Journal of Soil Science (Vol. 67, Issue 1)
- Lehmann, A., W. Zheng, M. Ryo, K. Soutschek, J. Roy, R. Rongstock, S. Maaß, and M. C. Rillig. 2020. Fungal traits important for soil aggregation. Frontiers in Microbiology 10:10. doi:10.3389/fmicb.2019.02904.
- Leslie, I. S. 2017. Queer farmers: Sexuality and the transition to sustainable agriculture. Rural Sociology 82 (4):747–71. doi:10.1111/ruso.12153.
- Leslie, I. S., J. Wypler, and M. M. Bell. 2019. Relational agriculture: Gender, sexuality, and sustainability in U.S. Farming. Society and Natural Resources 32 (8):853–74. doi:10.1080/08941920.2019.1610626.
- Li, C., J. Chen, J. Wang, Z. Ma, P. Han, Y. Luan, and A. Lu. 2015. Occurrence of antibiotics in soils and manures from greenhouse vegetable production bases of Beijing, China and an associated risk assessment. Science of the Total Environment 521-522:101–107. doi:10.1016/j.scitotenv.2015.03.070.
- Lin, C., C. Luo, L. Pang, and S. Tu. 2011. Losses of soil and nutrients from a purplish soil on slopping lands as affected by rain intensity and farming practices. Soil Erosion Issues in Agriculture. doi:10.5772/24082.
- Lobell, D. B., W. Schlenker, and J. Costa-Roberts. 2011. Climate trends and global crop production since 1980. Science 333 (6042):616–20. doi:10.1126/science.1204531.
- Lucantoni, D. 2020. Transition to agroecology for improved food security and better living conditions: Case study from a family farm in Pinar del Río, Cuba. Agroecology & Sustainable Food Systems 44 (9):1124–61. doi:10.1080/21683565.2020.1766635.
- Lucantoni, D., M. Casella, A. Marengo, A. Mariatti, A. Mottet, A. Bicksler, M. R. Sy, and F. Escobar. 2022. Informe sobre el uso del Instrumento para la Evaluación del Desempeño de la Agroecología (TAPE) en Argentina - Resultados y discusión desde el Area Metropolitana de Rosario. Italy: FAO.
- Lucantoni, D., A. Mottet, A. Bicksler, F. De Rosa, B. Scherf, E. Scopel, S. López-Ridaura, B. Gemill-Herren, R. Bezner Kerr, J. M. Sourisseau. 2021. Évaluation des transitions vers des systèmes agricoles imentaires durables: Un outil pour l’évaluation des performances agroécologiques (TAPE). Revue AE&S 11 (1): 1–19. doi:10.54800/epa550.
- Luna-González, D. V., and M. Sorensen. 2018. Higher agrobiodiversity is associated with improved dietary diversity, but not child anthropometric status, of mayan achí people of Guatemala. Public Health Nutrition 21 (11):2128–41. doi:10.1017/S1368980018000617.
- Mac Loughlin, T. M., L. Peluso, and D. J. G. Marino. 2017. Pesticide impact study in the peri-urban horticultural area of Gran La Plata, Argentina. Science of the Total Environment 598:572–580. doi:10.1016/j.scitotenv.2017.04.116.
- Mac Loughlin, T. M., M. L. Peluso, and D. J. G. Marino. 2022. Multiple pesticides occurrence, fate, and environmental risk assessment in a small horticultural stream of Argentina. Science of the Total Environment 802:802. doi:10.1016/j.scitotenv.2021.149893.
- Madsen, S., R. Bezner Kerr, N. LaDue, I. Luginaah, C. Dzanja, L. Dakishoni, E. Lupafya, L. Shumba, and C. Hickey. 2021. Explaining the impact of agroecology on farm-level transitions to food security in Malawi. Food Security 13 (4):933–54. doi:10.1007/s12571-021-01165-9.
- Manpoong, C., Wapongnungsang, W., and S. K. Tripathi. 2021. Soil carbon stock in different land-use systems in the hilly terrain of Mizoram, Northeast India. Journal of Applied and Natural Science 13 (2):723–28. doi:10.31018/JANS.V13I2.2615.
- Margenat, A., V. Matamoros, S. Díez, N. Cañameras, J. Comas, and J. M. Bayona. 2018. Occurrence and bioaccumulation of chemical contaminants in lettuce grown in peri-urban horticulture. Science of the Total Environment 637-638:1166–1174. doi:10.1016/j.scitotenv.2018.05.035.
- Martinez, L. E., P. F. Rizzo, P. A. Bres, N. I. Riera, M. E. Beily, and B. J. Young. 2021. Compendio de métodos analíticos para la caracterización de residuos, compost y efluentes de origen agropecuario y agroindustrial. Ediciones INTA. http://repositorio.inta.gob.ar:80/handle/20.500.12123/10587.
- Matos, E. L., D. I. Lori~, N. Azbimo, N. Sobe, and E. C. de Bzcji5d. 1987. Pesticides in intensive cultivation: Effects on working conditions and workers’ health. Bulletin of the Pan American Health Organization (PAHO) 21 (4):1987. https://iris.paho.org/handle/10665.2/27212.
- Mie, A., H. R. Andersen, S. Gunnarsson, J. Kahl, E. Kesse-Guyot, E. Rembiałkowska, G. Quaglio, and P. Grandjean. 2017. Human health implications of organic food and organic agriculture: A comprehensive review. Environmental Health: A Global Access Science Source 16 (1). doi:10.1186/s12940-017-0315-4.
- Modernel, P., S. Dogliotti, S. Alvarez, M. Corbeels, V. Picasso, P. Tittonell, and W. A. H. Rossing. 2018. Identification of beef production farms in the pampas and Campos area that stand out in economic and environmental performance. Ecological Indicators 89:755–70. doi:10.1016/j.ecolind.2018.01.038.
- Mottet, A., A. Bicksler, D. Lucantoni, F. de Rosa, B. Scherf, E. Scopel, S. López-Ridaura, B. Gemmil-Herren, R. Bezner Kerr, J. M. Sourisseau, et al. 2020. Assessing transitions to sustainable agricultural and food systems: A tool for agroecology performance evaluation (TAPE). Frontiers in Sustainable Food Systems 4:4. doi:10.3389/fsufs.2020.579154.
- NORMA IRAM, Pub. L. No. 29570-1., Calidad ambiental - Calidad del suelo. Determinación de fósforo extraíble en suelos. Parte 1 - Método Bray-Kurtz 1 (2010).
- Paladino, I. R., A. C. Sokolowski, J. Irigoin, H. Rodriguez, M. C. Gagey, M. B. Barrios, J. de Grazia, S. Debelis, J. Wolski, and A. Bujan. 2018. Soil properties evaluation in horticultural farms of Florencio Varela, Buenos Aires, Argentina. Environmental Earth Sciences 77 (11):411. doi:10.1007/s12665-018-7568-2.
- Pellegrini, M. C., E. Okada, R. A. González Pasayo, and A. G. Ponce. 2022. Prevalence of Escherichia coli strains in horticultural farms from Argentina: Antibiotic resistance, biofilm formation, and phylogenetic affiliation. Environmental Science and Pollution Research 29 (16):23225–36. doi:10.1007/s11356-021-17523-1.
- Prack McCormick, B., V. A. el Mujtar, A. Cardozo, V. E. Álvarez, H. A. Rodríguez, and P. A. Tittonell. 2022. Nutrient source, management system and the age of the plantation affect soil biodiversity and chemical properties in raspberry production. European Journal of Soil Biology 111:103420. doi:10.1016/J.EJSOBI.2022.103420.
- Prack McCormick, B., M. P. Quiroga, V. E. Álvarez, D. Centrón, and P. Tittonell. 2022. Antimicrobial resistance dissemination associated with intensive animal production practices in Argentina: A systematic review and meta-analysis. Revista Argentina de Microbiología 55 (1):25–42. doi:10.1016/J.RAM.2022.07.001.
- Prack McCormick, B., and H. J. Rivera ;, M. B. Barrios ;, P. M. Palladino ;, D. E. Rojas ;, D. S. Cristos ;, & P. A. Tittonell (2020, October). Transferencia de antibióticos desde la producción avícola hacia la horticultura: la cama de pollo como vehículo. Actas – XXVII Congreso Argentino de La Ciencia Del Suelo. https://congreso2020.suelos.org.ar/actas/
- Pretty, J., T. G. Benton, Z. P. Bharucha, L. V. Dicks, C. B. Flora, H. C. J. Godfray, D. Goulson, S. Hartley, N. Lampkin, C. Morris, et al. 2018. Global assessment of agricultural system redesign for sustainable intensification. Nature Sustainability 1 (8):441–46. doi:10.1038/s41893-018-0114-0.
- Ramesh, T., N. S. Bolan, M. B. Kirkham, H. Wijesekara, M. Kanchikerimath, C. Srinivasa Rao, S. Sandeep, J. Rinklebe, Y. S. Ok, B. U. Choudhury, et al. 2019. Soil organic carbon dynamics: Impact of land use changes and management practices: A review. Advances in Agronomy 156:1–107. doi:10.1016/BS.AGRON.2019.02.001.
- Rivas, I. S. 2010. gestión ambiental para el ordenamiento territorial del partido de Florencio Varela, Area Metropolitana de Buenos Aires. Revista de La Asociacion Geologica Argentina 66 (4): 535–543.
- Salvioli, M. L., G. A. Colli, M. Cipponeri, and G. Calvo (2012, May). Gestión de cuencas: análisis comparativo en función de la calidad del agua superficial y usos del suelo de dos pequeñas cuencas urbanas. Actas VII Congreso de Medio Ambiente Dela AUGM – CoAmbiente – Consultora Ambiental. https://www.coambiente.com.ar/vii-congreso-de-medio-ambiente-dela-augm
- SAMLASistema de Apoyo Metodológico a los Laboratorios de Análisis de Suelos Agua, Vegetales y Enmiendas Orgánicas (2004).
- Sassenrath, G. F., K. Davis, A. Sassenrath-Cole, and N. Riding. 2018. Exploring the physical, chemical and biological components of soil: Improving soil health for better productive capacity. Kansas Agricultural Experiment Station Research Reports 4 (3). doi: 10.4148/2378-5977.7577.
- Seibert, I. G., A. T. Sayeed, Z. Goergieva, and A. Guerra. 2010. Without feminism, there is no agroecology. Right to Food and Nutrition Watch 1: 42–50. https://www.righttofoodandnutrition.org/files/rtfn-watch11-2019_eng-42-50.pdf.
- Siegner, A. B., C. Acey, and J. Sowerwine. 2020. Producing urban agroecology in the East Bay: From soil health to community empowerment. Agroecology & Sustainable Food Systems 44 (5):566–93. doi:10.1080/21683565.2019.1690615.
- Simon-Rojo, M., I. M. Bernardos, and J. S. Landaluze. 2018. Food movement between autonomy and coproduction of public policies: Lessons from Madrid. Nature and Culture 13 (1):47–68. doi:10.3167/nc.2018.130103.
- Six, J., H. Bossuyt, S. Degryze, and K. Denef. 2004. A history of research on the link between (micro)aggregates, soil biota, and soil organic matter dynamics. Soil and Tillage Research 79 (1):7–31. doi:10.1016/j.still.2004.03.008.
- Skill, K., S. Passero, and M. Farhangi. 2022. Cultivating agroecological networks during the pandemic in Argentina: A sociomaterial analysis. Land 11 (10):1782. doi:10.3390/land11101782.
- Soil Survey staff. 2010. Keys to Soil Taxonomy. United States: USDA-Natural Resources Conservation Service.
- Sokolowski, A. C., B. Prack McCormick, J. de Grazia, J. E. Wolski, H. A. Rodríguez, E. P. Rodríguez-Frers, M. C. Gagey, S. P. Debelis, I. R. Paladino, and M. B. Barrios. 2020. Tillage and no-tillage effects on physical and chemical properties of an Argiaquoll soil under long-term crop rotation in Buenos Aires, Argentina. International Soil & Water Conservation Research 8 (2):185–94. doi:10.1016/j.iswcr.2020.02.002.
- Steinauer, K., A. Chatzinotas, and N. Eisenhauer. 2016. Root exudate cocktails: The link between plant diversity and soil microorganisms? Ecology and Evolution 6 (20):7387–96. doi:10.1002/ece3.2454.
- Tittonell, P., M. Fernandez, V. E. el Mujtar, P. V. Preiss, S. Sarapura, L. Laborda, M. A. Mendonça, V. E. Alvarez, G. B. Fernandes, P. Petersen, et al. 2021. Emerging responses to the COVID-19 crisis from family farming and the agroecology movement in Latin America – a rediscovery of food, farmers and collective action. Agricultural Systems 190:103098. doi:10.1016/j.agsy.2021.103098.
- Vázquez, M., and A. Terminiello. 2008. Recuperación de suelos degradados de pequeños productores del cinturón hortícola del Gran La Plata. Valoración del problema y estrategias correctivas, Ed. Draghi L. FCAyF, UNLP. https://www.agro.unlp.edu.ar/sites/default/files/paginas/2008._manual_cinturon_horticola_la_plata_directora_mabel_vazquez.pdf.
- Wezel, A., S. Bellon, T. Doré, C. Francis, D. Vallod, and C. David. 2009. Agroecology as a science, a movement and a practice. Sustainable Agriculture 2 (4):503–15. doi:10.1007/978-94-007-0394-0_3.
- Xu, G., M. Yue, Y. Ren, J. Song, and X. Chen. 2022. Development of soil phosphorus storage capacity for phosphorus retention/release assessment in neutral or alkaline soils. Soil and Environment 68 (3):146–54. doi:10.17221/482/2021-PSE.
- Zaremba, H., M. Elias, A. Rietveld, and N. Bergamini. 2021. Toward a feminist agroecology. Sustainability 13 (20):11244. doi: 10.3390/su132011244. Switzerland), 13(20
- Zhang, Q., J. Wu, F. Yang, Y. Lei, Q. Zhang, and X. Cheng. 2016. Alterations in soil microbial community composition and biomass following agricultural land use change. Scientific Reports 6 (1). doi:10.1038/srep36587.
- Zibilske, L. M. 1994. Carbon mineralization. In Methods of soil analysis. Part 2: Microbiological and biochemical properties, ed. R. W. Weaver, S. Angle, P. Bottomley, D. Bezdicek, Smith, S. A. Tabatabai, and A. Wollum. United States: Soil Science Society of America, Inc. doi:10.2136/sssabookser5.2.c38.