Abstract
The mucosal barriers of catfish (Ictalurus spp) constitute the first line of defense against pathogen invasion while simultaneously carrying out a diverse array of other critical physiological processes, including nutrient adsorption, osmoregulation, waste excretion, and environmental sensing. Catfish depend more heavily on mucosal barriers than their terrestrial counterparts as they are continuously interacting with the aquatic microbiota. Our understanding of these barriers, while growing, is still limited relative to that of mammalian model systems. Nevertheless, a combination of molecular and cellular studies in catfish over the last few decades, and particularly within the last few years, has helped to elucidate many of the primary actors and pathways critical to their mucosal health. Here we describe aspects of innate and adaptive immune responses in the primary mucosal tissues (skin, gill, and intestine) of catfish, focusing on mucus-driven responses, pathogen recognition, soluble mediators, and immunoglobulin and T-cell derived immunity. Modulation of mucosal barriers will be critical moving forward for crafting better diets, improving vaccine delivery, enhancing water quality, and ensuring sustainable production practices in catfish.
Introduction
The importance of mucosal barriers as a first line of defense against pathogens cannot be overstated in the aquatic environment. There, in an ideal medium for microorganismal growth, aquatic vertebrates are constantly colonized by a variety of commensals and opportunistic and primary pathogens along their exposed gill and skin surfaces as well as through the gut. These surfaces are dynamic interfaces which mediate critical physiological functions such as nutrient and oxygen absorption and waste secretion while also sensing, sampling, and screening a diverse microbiome. In spite of these challenges, teleost fishes have been a success story of adaptation and diversification, representing over half of extant vertebrates, and thriving in varying environments ranging from stagnant pools to fast-running rivers with associated wide variation in tolerated water chemistry parameters (e.g. dissolved oxygen, temperature, salinity, etc.). Our knowledge of the cellular actors and mechanisms governing mucosal barriers is limited to a handful of teleost species. Among the better studied of these is the channel catfish, the focus of this review.
Channel catfish, Ictalurus punctatus, is an important aquaculture species in the United States, but is also one of the better-studied immune models among teleost fish.Citation1 Given its role as a cultured food fish, immune responses in catfish have been studied in the context of important pathogens known to cause widespread disease. These include parasites which encyst on gill epithelia, intracellular and extracellular bacterial pathogens which invade through the skin, gill, nares, and gut, and herpesviruses which be transmitted horizontally. While the etiology of these pathogens have been studied for 30-40 years, we have only recently focused intensively on the invasive strategies and associated host defense responses at the sites of initial entry. Much of the recent knowledge of mucosal barriers in catfish in this regard have been gained through transcriptome studies of impacted mucosal tissues, as reviewed below, accompanied by infection challenge models which help to dissect the early stages of pathogenesis. These studies have revealed a variety of novel and conserved actors at these barriers relative to mammalian paradigms. As antibody reagents and gene-modification technologies (e.g., CRISPRs) become more accessible in catfish, in-depth functional studies will be needed to confirm the putative roles of these genes and to analyze the functional of cellular subsets.
In catfish and other cultured fishes, our study of the physiology and immunology of mucosal barriers goes beyond a comparative approach aimed at understanding structure and function to the very real need for development of improved culture practices, diets, vaccines, and therapeutants for aquaculture. Almost all of the most urgent areas of aquaculture research require an understanding of mucosal barriers, from improving vaccine delivery via feeding or immersion to ameliorating soy diet-induced enteritis to development of prebiotic and probiotic additives to modulate beneficial microflora. The mucosal barriers are the interfaces where nutrition, social cues, water quality, host immunity, and the microbiome intersect to integrate their signals, with far-reaching consequences on pathogen prevalence, fish growth and fish health.Citation2 Throughout this review, we will attempt to discuss the catfish mucosal system in the light of this continuous, dynamic interplay occurring at these surfaces and point out applications of this knowledge which may improve fish health in aquaculture settings. As a final introductory note, this review will focus primarily on the gill, skin, and gut barriers of catfish, given the relatively minimal study to-date of the more minor barriers such as reproductive tissues and the nares.
Structural overview of major mucosal barriers in catfish
Gill
Indicative of the rapid growth of knowledge in the area, aspects of physiology and mucosal immunity shared among teleost fishes have been covered in-depth recently by several excellent reviews and a book edited by the authors of this review.Citation2-7 We will focus, therefore, here on aspects and research bearing more directly on channel catfish.
The gills of catfish play important roles in respiration, osmoregulation, and nitrogenous waste secretion, in addition to their immune roles as a mucosal tissue. In teleosts, each of the 2 gills have 4 gill arches and 5 slits. Each arch contains a septum and 2 rows of filaments termed the primary lamellae. Arches and filaments are a complex tissue of cartilage, connective tissue, blood vessels, nerves, and immune cells. Although relatively unexplored, recent research has described a gill associated lymphoid tissue (GiALT) or inter-branchial lymphoid tissue with intraepithelial aggregations of T cells.Citation8,9
Skin
The skin of the catfish, also referred to as the integumentary system, covers the outer surface of the fish with 3 layers, the epidermis, dermis, and the hypodermis (closest to muscle). An aqueous mucus layer covers the epidermal surface. The uppermost layer of the epidermis, termed the superficial stratum, lies over intermediate and basal epidermal cells, and contains microridges retaining mucus and antibacterial substances continuously renewed by goblet cells in the intermediate layer. Also in the intermediate layer of epidermis are found various types of cells, including unicellular glands (mucus cells and club cells), sensory cells, ionocytes, immune cells, pigment cells, and undifferentiated cells. The basal part of the epidermis is a single-cell layer which is attached to the basement membrane via hemidesmosomes. Additionally, the basal layer tightly links the epidermis to dermis. The dermis is composed of a collagenous matrix while the hypodermis contains loosely organized collagen and vasculature. The majority of catfish species, including those in the genus Ictalurus do not have scales.Citation2,10-13 The skin of channel catfish is particularly noted for its extensive innervation by sensory cells, including taste buds which cover most of the tissue surface, and ampullary organs and neuromasts controlling electroreception and lateral line sensory systems.Citation14,15 While relatively little is known about immunity in fish skin, recent studies have established intriguing similarities between the skin-associated lymphoid tissue (SALT) and that of the gut.Citation16
Gut
The gut barriers of fish are responsible for nutrition absorption, sodium and water balance, neuroendocrine actions, and immune barrier functions. The gastrointestinal tracts of teleost fish share many common characteristics with corresponding mucosal surfaces in mammals. The intestine of the channel catfish can be divided into a thick ascending segment, a descending segment, a thin convoluted segment and a thicker terminal segment, the rectum. The ascending segment of the intestine is composed of numerous mucosal folds containing branched villi. The descending and convoluted segments have fewer folds with shorter and less-branching villi. The descending and convoluted segments account for 80% of the total length of the intestine. An approximately 4-fold increase in the thickness of the tunica muscularis of the terminal segment of the intestine has been reported.Citation17,18 The mucosal epithelium is generally composed of a simple lamina propria, blood vessels, nerves, collagenous matrices and gut-associated lymphoid tissue (GALT) including lymphocytes populations, macrophages, dendritic cells, as well as other intraepithelial immune cells.Citation3,19,20 As with other mucosal tissues, fish intestinal mucosal surfaces are covered by a mucosal gel layer secreted by mucus-secreting goblet cells, forming the outermost barrier of innate defense and providing a matrix for immunoglobulins secreted by GALT-analog plasma cells.Citation21,22
Innate Immunity at Catfish Mucosal Barriers
The role of mucus
Mucins in the gill
Catfish gill epithelium, like other mucosal epithelia, secretes mucus, predominantly made up of mucins from mucus or goblet cells distributed throughout the llamellar epithelium. Mucins are high-molecular weight glycoproteins saturated with O-linked sugars. Mucus cell numbers have been shown to be sensitive to dietary changes, stress and handling in other Ictalurus species and to water temperature in channel catfish. Citation23,24 Work by our group has identified expression patterns of MUC2, MUC5AC, MUC19 and related isoforms in catfish gill. Examination of fish susceptible to the common freshwater pathogen, Flavobacterium columnare, revealed basally higher levels of goblet cells () and mucin expression, a pattern maintained after infection.Citation25 Interestingly, F. columnare shows a strong chemotactic attraction to catfish mucus, where it appears to associate with goblet cells.Citation26-28 Mucin levels are also altered by short-term fasting, a nutritional state previously associated with higher F. columnare mortality.Citation29-31 Goblet cell proliferation appears to be under the control of anterior gradient 2 protein (AGR2) in zebrafish.Citation32 Similarly, we have observed changes in AGR2 due to diet and immune status.Citation25,30 Quantity and composition (e.g. sialomucin vs. sulfomucin) of mucus is likely critical for dynamics of pathogen invasion, successful attachment to host receptors, and persistence, and, therefore, is a target of immuno-sculpting by fish pathogens.Citation33 A recent study of F. columnare biofilm formation highlighted the importance of factors such as salinity, water hardness, and the presence of sugars such as mannose, all directly relevant to mucus production.Citation34-37
Figure 1. Channel catfish featuring different susceptibilities (panel A, resistant fish; panel B, susceptible fish) to Flavobacterium columnare (columnaris disease) show disparate numbers of mucus-producing goblet cells in the gill (indicated by black arrows). 200X magnification. Periodic acid Schiff technique.
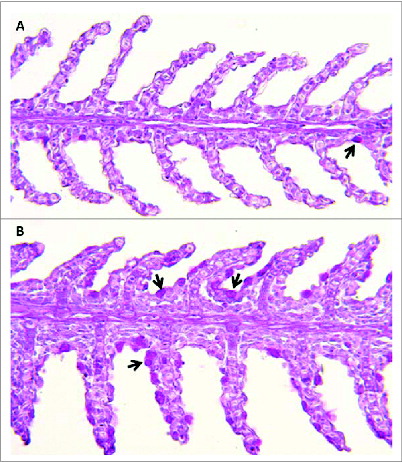
Mucins in the skin
The skin in fish is constantly processing complex signals from the aquatic milieu, sensing and integrating environmental, nutritional, and health cues. In fish, mucus is also continuously secreted by goblet cells in the skin epidermis, playing indispensable roles in shedding pathogen and dead cells away to maintain host health. As mentioned above, short-term feed deprivation in channel catfish altered mucin expression in both skin and gill, potentially through changes in gene expression of AGR2.Citation30 A similar fasting study in blue catfish revealed drastically different levels of mucin expression, potentially altering the host-pathogen dynamics in host fish.Citation29 In recent years a virulent strain of Aeromonas hydrophila capable of producing a motile aeromonad septicemia (MAS) infection, has caused widespread mortality in the US catfish industry. Our previous work utilized high-density Affymetrix microarrays to examine gene expression profiles in both channel and blue catfish skin upon A. hydrophila infection. Intriguingly, in experimental challenge, only disruption (scraping) of skin mucus prior to immersion challenge could reliably produce A. hydrophila mortality. Microarray analyses revealed differential expression patterns of MUC5AC, MUC5B at early timepoints following challenge in the more susceptible channel catfish but not in blue catfish.Citation38,39 The gaps in our understanding of mucin dynamics in catfish skin are numerous. Mucin secretion in the skin, like the gill, undoubtedly responds to complex signals from environment, host, pathogen, and the commensal microbiome. In brook charr, stressful conditions modulate skin mucus microbiota, decreasing probiotic-like bacteria and increasing the abundance of pathogenic bacteria.Citation40 Mucus secretion patterns dictate not only rates of bacterial shedding but also production of enzymes, antimicrobial peptides, and secreted immunoglobulins.Citation16 In Atlantic salmon, food deprivation has been shown to cause rapid changes in the abundance and composition of skin mucus cells, accompanied by shifts in diversity of microbiota.Citation41,42 Further work in catfish is needed to chart these interactions in skin and to more clearly delineate cause and effect between environmental perturbations, commensal shifts, and host immunity.
Mucins in the gut
A histological and flow cytometric study of cell types in the catfish intestine revealed goblet cells distributed throughout all gut segments, with highest numbers and copious amounts of mucin found in the distal rectal segment.Citation43 Our RNA-seq analysis of intestinal immune responses following Edwardsiella ictaluri infection identified perturbed expression of mucins in the gut (MUC2, MUC5B), but further work is needed to clarify the roles of these mucins.Citation44 Probiotics and prebiotics, known to positively impact mucus secretion and gut health in mammals, are increasingly added to fish diets, including catfish.Citation45 However, few studies have examined their impact on mucin expression, goblet cell numbers or mucus secretion (see refs. 46 for an exception to this). In a recent study examining response to fed prebiotics (yeast cell wall components), we noted higher mucin expression in the gill both before and after F. columnare challenge, potentially indicating cross-presentation and priming across the catfish mucosal system (Unpublished results).
Mucosal Secretions
Fish mucosal secretions are known to contain a variety of antimicrobial peptides, complement proteins, proteases, and lysozyme.Citation3 A series of RNA-seq expression studies in catfish gill exposed to F. columnare or following feed deprivation have cataloged a diverse innate repertoire likely secreted into the mucus.Citation25,30,47 These include complement factor D, C1, C7, C1q, NK-lysin, bacterial permeability-increasing protein (BPI) and several fish toxin proteins including natterin and toxin-1 precursor, lysozyme C, and lysozyme G. In the intestine, we have additionally detected H2 antimicrobial peptides, serine proteases, granzyme and abundant metalloproteinases.Citation44 Of these, lysozyme C appears to be a particularly good marker of immune readiness, particularly in the skin and gill of catfish. Lysozyme C also displayed consistently higher expression in resistant catfish gill than that observed in susceptible fish. Citation25 Plasma lysozyme levels have been studied for several decades in the context of fish immunity, but relatively little attention has been given to the level and roles of lysozyme in mucosal surfaces.Citation48,49 In mammals, lysozymes are among the most abundant secreted mucosal enzymes from the epithelium as well as a major component of granules of professional phagocytes. They help to kill bacterial pathogens through enzymatic and antimicrobial activity.Citation50 In catfish mucosa, the cellular origins of lysozyme are not known. Using a monoclonal antibody specific for lysozyme, Marsh and Rice showed that myeloid cells, presumptively identified as granulocytes and macrophages, isolated from the anterior kidney expressed lysozyme.Citation51 In Atlantic salmon, intestinal eosinophilic granular cells showed immunoreactivity to an antibody targeting lysozyme which was localized to the secretory granules of these cells; similar to that observed in mammalian Paneth cells.Citation52 In previous studies in catfish challenged with Edwardsiella ictaluri, plasma lysozyme dynamics differed between resistant and susceptible fish, with a faster response and elevated lysozyme levels characterizing resistant catfish strains.Citation53,54 Also supporting the potential importance of high mucosal levels of lysozyme for disease resistance is research from zebrafish. Yazawa et al. Citation55 established a transgenic zebrafish strain expressing a chicken lysozyme gene under the control of a keratin promoter which resulted in a 65% survival rate against F.columnare compared to 0% survival in wild-type fish.Citation25 We have also observed suppression of skin/gill lysozyme C levels following short-term feed deprivation Citation30 and elevated lysozyme levels in gills following feeding a diet supplemented with mannan-oligosaccharides (unpublished results).
Pathogen recognition at mucosal barriers
Like mammals, teleost fish rely on pathogen recognition receptors (PRRs) for detecting and responding to pathogen-associated molecular patterns (PAMPs). However, as with other aspects of fish immunology, diversification of these receptors (often via tandem duplication) has generated a vast arsenal of genes capable of recognizing non-self. Many of these lack clear mammalian orthologues and await further functional study. Most of our understanding of PRRs in mucosal tissues in catfish again comes from transcriptome studies (RNA-seq and microarray). Here we will review our knowledge of 3 major groups of PRRs known to be active in catfish mucosal immune responses: TLRs, NLRs, and lectins.
TLRs
Toll-like receptors (TLRs) are by far the best characterized group of PRRs in vertebrates. In humans, TLR1-10 provide various PAMP-recognition specificities.Citation56 Teleost TLR repertoires are larger, potentially reflecting the diversity of pathogens encountered in the aquatic environment.Citation57 Catfish have one of the larger sets of TLRs described from fish, with 19 TLRs described by Quiniou et al.Citation58 and 20 TLRs described by Zhang et al.Citation59 These represent both structural orthologs of human TLRs and fish-specific TLRs. Direct evidence for ligand specificity has only been shown in a handful of fish TLRs, leaving us to speculate upon the exact molecular patterns recognized by most catfish TLRs.Citation57 However, expression evidence in the context of disease, points to a role for TLRs in mucosal immunity.
Zhao et al.Citation60 examined TLR responses in several tissues (including skin and gill) following infection with the ciliate parasite Ichthyophthirius multifiliis. They reported significant up-regulation of TLR1 in skin, and TLR2, TLR9, and TLR19 in skin and gill. Interestingly, in their work characterizing catfish TLRs, Zhang et al. Citation59 found that TLR4, responsible for LPS recognition in mammals, had highest expression in skin, gill, and intestine. TLR4-like molecules in zebrafish, however, cannot sense LPS, and protein characterization in catfish has revealed that catfish TLR4 also likely lacks the necessary structural features for this ligand.Citation58 In work by our group focused on immune responses in mucosal tissues, we have detected differential expression of only 2 TLRs—TLR5S and TLR21. TLR5S showed significant down-regulation (>10-fold) at 3 examined timepoints (2 h, 8 h, and 12 h) in the skin of catfish infected with virulent A. hydrophila as part of a transcriptome profile supporting broad immune evasion and suppression by the pathogen.Citation38 TLR5S was modestly up-regulated at 3 d following E. ictaluri infection in the catfish intestine. TLR21 was also modestly induced in the gill of catfish susceptible to F. columnare soon after infection. The majority of expression studies by our group have focused on early timepoints following infection (e.g., within 24 h post-challenge), a period where one would expect TLR-mediated recognition would be critical in initiating downstream immune cascades. Our failure to detect significant TLR-driven responses in several studies and the small handful of TLRs involved may point to a relatively minor role for TLRs in catfish mucosal tissues when compared with secondary lymphoid organs such as spleen or head kidney. Lectins and other PRRs may be playing a far more important role in surveillance at initial sites of pathogen infection and entry.
NLRs
The NLRs (nucleotide-binding domain and leucine-rich repeat containing family receptors) are a recently identified family of pattern recognition receptors in vertebrates. Several subfamilies of NLRs have been characterized in human, mouse, and zebrafish, but relatively little is known of their function in catfish or other species. NLRs are intracellular sensors recognizing PAMPs, like TLRs, through diverse leucine-rich repeat domains. NLRs interact with inflammatory caspases, forming a complex termed the inflammasome, and activating pro-inflammatory cytokine signaling (often IL-1β or IL-18; reviewed in fish by Hansen et al. Citation56 Two recent studies characterized the NLR family in catfish.Citation61,62 The latter study, the more comprehensive of the 2, identified 22 NLRs including 6 members of the NLR-A subfamily (NODs), 2 members of the NLR-B subfamily, and 11 members of the NLR-C subfamily. Selected NLRs were found to be ubiquitously expressed, including in skin, gill, and intestine and to be down-regulated in the intestine following E. ictaluri infection.Citation63 By contrast, work by our group has found broad NLR-C subfamily upregulation in the catfish intestine following E. ictaluri infection.Citation44 A NLRC3-like gene was upregulated by F. columnare infection in the catfish gill Citation47 and was expressed more highly in F. columnare susceptible fish.Citation25 A NLRC1-like gene was upregulated upon A. hydrophila infection in catfish skin, but was also found to be more highly expressed in the gill of F. columnare resistant fish. Members of the NLR family appear to be important early mediators of innate mucosal responses in catfish, but further functional studies are needed to more precisely determine their roles.
Lectins
A subset of PRRs, rather than remaining in association with a particular cell type, are secreted. Among these are lectins, a group carbohydrate-binding proteins that recognize specific carbohydrate moieties expressed on cell surfaces. Lectins are classified into several families depending on their sequence similarities and sugar binding specificities including C, I, P, and F-type lectins, pentraxins, rhamnose binding lectin, galectins, mannose binding lectins, calnexin and calreticulin, endoplasmic reticulum ER-Golgi intermediate compartment protein (ERGIC-53) and vesicular integral protein (VIP-36), discoidins, fibrinogen-type, and fucolectins.Citation64 In vertebrates and invertebrates, lectin repertoires not only participate in recognition of pathogens, but also play distinct biological roles in both innate and adaptive immunity.Citation65 Expression studies by our group have found that lectins are rapidly and strongly regulated in response to a variety of infections in the gill, skin, and intestine, pointing to a critical role for these molecules in early pathogen detection and binding. Many of these lectins may be secreted directly into the mucus where they are held by binding to mucin glycoproteins and where they can provide protection and surveillance against foreign pathogens.Citation66,67 A rise in mucin secretion, therefore, may lead to a rise in the presence of certain mucus-bound lectins.
Mannose binding lectin is an important member of the collectin family and one of the better studied lectins in catfish, albeit with few studies examining its roles in mucosal barriers.Citation68-70 MBLs are important for induction of the complement system via mannose-binding lectin-associated serine proteases (MASPs). MBLs have been identified in the proteome of skin mucus from Atlantic cod, indicating they may be present constitutively at barrier defenses.Citation71 In catfish, we have observed induction of MASP2 in catfish gill following F. columnare infection,Citation47 higher levels of MBL1 in channel and blue catfish skin following A. hydrophila infection,Citation38,39 and 86-fold higher expression of MBL2b in resistant channel catfish gill tissue compared to susceptible gill at 1 h following F. columnare challenge.Citation25
Galectins constitute a lectin family defined by their binding specificity for β-galactoside sugars. Three families of galectins (proto, chimera, and tandem-repeat) have been identified in teleost fish. Since their first description in the mid-1970s, galectins have been assigned to several roles including embryogenesis, host early development and response to infection through the IFN-induced antiviral pathway.Citation72,73 In contrast to other fish species where functional studies on galectins have been carried out (see review by Ogawa et al. Citation74, no comprehensive assessment of galectins has been carried out in catfish. However, our recent expression analyses again indicate the presence and regulation of these receptors before and soon after bacterial infection in mucosal tissues. Galectin-4 was observed to be downregulated by short-term feed deprivation in channel catfish.Citation30 Particularly strong galectin responses to A. hydrophila infection were also detected, including a 13-fold up-regulation of galectin-3 in channel catfish skin at 2 h post-infection, and a 63-fold downregulation of galectin-9 at 24 h post-infection in blue catfish.
Rhamnose-binding lectins (RBLs) have been reported from a variety of fish species as well as several invertebrates.Citation74,75 They have well-characterized roles in fish eggs as well as being pattern recognition receptors in innate immunity.Citation76-79 Our group has recently utilized channel catfish (Ictalurus punctatus) and F. columnare as a host-pathogen model to understand teleost mucosal dynamics outside the gut. Global transcriptome profiling of the channel catfish gill revealed a rhamnose-binding lectin whose expression was induced greater than 100-fold at 3 h following F. columnare experimental infection.Citation47 Saturation of the RBL with its ligands, L-rhamnose or D-galactose, lowered its expression and decreased F. columnare adhesion and catfish mortality in a dosage-dependent manner in a subsequent challenge infection.Citation35 Additionally, RBL expression was found to be inversely correlated with host resistance to F. columnare infection.Citation25 Taken together, these results suggested that the host lectin is mediating F. columnare binding to the surface mucosa. We next asked if changes in feeding, previously tied to F. columnare susceptibility, may impact RBL expression.Citation80 Indeed, a period of 7 d fasting upregulated RBL expression in the catfish gill greater than 120-fold.Citation35 We have subsequently characterized the broader RBL family in channel catfish, identifying 6 RBL genes, all of which showed some degree of homeostatic expression in mucosal tissues.Citation81 Further research is needed to better delineate the function and signaling of these diverse lectins. Ongoing work in our labs is focused on identifying means of harnessing and/or manipulating rhamnose-based host-pathogen adhesion for altering F. columnare attachment and enhancing the performance of existing vaccines. In this vein, a recent feeding trial with mannan-oligosaccharides also lowered RBL levels, and resulted in lower mortality following F. columnare challenge (unpublished results).
Cell junctions
While not classically categorized under pathogen recognition, discussion of junctional machinery is warranted here as the altered expression of genes related to junctional regulation has repeatedly been documented in recent years; particularly at early timepoints following microbial infections. Clearly, pathogens seek to disrupt the cellular junctions present in host epithelia in an effort to facilitate host invasion. The full complement of genes and their protein products related to the maintenance of cell junctions is not completely understood in the catfish model system. Across a range of pathogenic insults, we and others have observed marked dysregulation in junctional components after pathogen encounter; namely the apical junction complex comprised of the tight junction, adherens junction, and the desmosome. One example group of junctional regulators worth mentioning here are the claudins which selectively regulate permeability of epithelial barriers. To date, in channel catfish, 52 claudin genes have been identifiedCitation82. Hypoxic stress has been shown to alter the expression of claudins in the gill,Citation82 while in the intestine, a downregulation of claudin gene expression was revealed soon after encountering E. ictaluri.Citation44 However, most other transcripts representing junctional proteins were up-regulated (oftentimes only modestly), including cadherins, desmoplakin, and MAGI3 among others, potentially a result of pathogen-induced cytoskeletal rearrangements.Citation44 Relatedly, after infection with Aeromonas hydrophila, several components of desmosomes including desmocollin 1a, desmoglein and plakoglobin were perturbed in the skin of channel catfish.Citation38 Transcriptomic profiling of gill and skin after an experimental exposure to Flavobacterium columnare showed rapid (by 1 hr) and robust alterations in the expression of factors related to junctional processes.Citation25 Intriguingly, a comparison of naïve fish obtained from columnaris resistant and susceptible families revealed that some claudin family members in resistant fish were expressed at levels >100 fold lower than resistant fish.Citation25 While further work is clearly needed, constitutively higher/lower levels of these and other junctional elements may play a role in the initiation or development of various diseases.
Soluble mediators of inflammation
The Importance of IL-17
Following pathogen recognition by receptors on the epithelial surface and/or leukocytes, a diverse set of cytokines and chemokines are released to channel and direct the inflammatory process. In the channel catfish gill epithelium, these responses include both pro-inflammatory (IL-8, IL-17, IL-22, TNFα) and anti-inflammatory cytokines (IL-13, IL-4, TGFβ) as well as reactive oxygen species mediators. In RNA-seq studies, we have observed a consistent correlation between high IL-17 expression in the gill and rapid F. columnare mortality.Citation25,47 Interleukin (IL) 17 family cytokines are important mediators of mucosal immune responses, tightly regulated by signals from the complex milieu of pathogenic and commensal microbes, epithelial cells and innate and adaptive leukocytes found at tissue barriers. In mammals, IL17 ligand expression has been linked not only to protective immunity but also excessive tissue inflammation and damage in the gut and lungs.Citation83 Recent work by our group identified and characterized 7 IL17 ligands and 4 IL17 receptor (IL17R) homologues from transcriptomic and genomic databases of channel catfish. To gain insight into the mucosal actions of the IL17A/Fs-associated pathway in inflammatory processes, the expression profiles of 3 IL17A/Fs and their putative receptors IL17RA and IL17RC in mucosal tissues of catfish following experimental challenge with E. ictaluri and F. columnare were investigated. Bacterial challenge induced higher expression of the catfish IL17A/Fs as early at 4 h post-infection, particularly in gill tissue. In contrast, in the catfish intestine, where IL17 function is best understood in mouse models, IL17A/F expression showed minimal early responses to E. ictaluri infection. Instead, a significant upregulation of IL17 ligands and receptors was observed in the intestine at 7 d, highlighting species and tissue-specific regulation of the IL17 family.Citation84 Given the importance of IL-17 to mucosal immunity, efforts are underway to generate the antibody reagents necessary for studying IL17+ cell subsets in catfish.
iNOS and Gill Mucosal Immunity
Production and release of nitric oxide also appears to be critical for deciding immune readiness, polarization, and disease outcomes in the catfish mucosal tissues, particularly the gill. We found that catfish gill has constitutively high expression of inducible nitric oxide synthase (iNOS; the most highly expressed of all captured genes; Peatman et al.Citation25, similar to reports in human respiratory epithelium.Citation85 The strongest down-regulation after fasting in channel catfish and blue catfish surface mucosa was seen in inducible nitric oxide synthase 2b (iNOS2b), previously established as the most likely teleost ortholog of mammalian iNOS,Citation29,30 while iNOS2b levels in resistant fish were 5-15-fold higher before and after challenge with F. columnare when compared with susceptible fish.Citation25 Macrophages utilize the NOS pathway for increased bacterial killing and disruption of pathogen virulence mechanisms. iNOS catalyzes the production of nitric oxide (NO) from L-arginine, and, therefore, is often an indicator of the readiness of mononuclear phagocytes to handle infection, or their activation state. Arginine synthesis and metabolism pathways were also dysregulated in fasted and columnaris-susceptible catfish in a manner likely altering macrophage activation states and compromising immune readiness.Citation25,29,30 Further functional studies are clearly needed to examine the complex interrelationships between feed deprivation, arginine metabolism, nitric oxide production and bacterial disease susceptibility in catfish.Citation30
Enigmatic Catfish Mucosal Factors
Across multiple published and unpublished RNA-seq studies in catfish mucosal tissues, several poorly-defined factors have stood out for their consistent presence and significant differential expression at early timepoints following bacterial infection. We briefly highlight 3 such factors here which deserve more attention in the future as we seek to better understand teleost mucosal immunology.
MFAP4
All mucosal studies to-date in catfish by our group have identified differential expression in microfibrillar-associated protein 4 (MFAP4) genes, including studies of skin, gill, and intestine. The lectin pathway of the complement system is characterized by mannose-binding lectins (MBLs) and ficolins, which both serve to recognize and bind carbohydrates in pathogens and activate complement, leading to opsonization, leukocyte activation, and direct pathogen killing. Previous characterization of MFAP4 genes in our lab noted structural similarities between MFAP4 and ficolin (unreported in teleosts), suggesting that MFAP4 genes may be carrying out similar roles in early pathogen recognition.Citation86 While the function of MFAP4 in mammals is poorly understood, it is known to bind to pulmonary surfactant protein A (SP-A), an oligomeric collectin that recognizes lipid and carbohydrate moieties present on broad range of micro-organisms, and mediates microbial lysis and clearance.Citation87 Additionally, in zebrafish, it is recognized as a specific marker for macrophages.Citation88 Future studies in catfish should utilize zebrafish MFAP4 reagents to better understand the role of these genes in mucosal immune responses.
Pancreatic glycoprotein 2 (GP2)
The equivalent of antigen-transporting M cells, critical to mammalian mucosal paradigms have not been identified in teleost fish.Citation6 However, interestingly, the most powerful marker of M cells in mammals, glycoprotein 2 (GP2), is a common signature of early responses to pathogens in the gill, skin, and gut of channel catfish. For example, GP2 was one of the most highly upregulated genes at 2 h post infection (7.70-fold) in A. hydrophila infected blue catfish.Citation39 GP2 is the major zymogen granule membrane protein, with reports finding that GP2 takes up bacteria through M cells within the Peyer's patches and lymphoid follicles to initiate the mucosal immune response.Citation89 Mucosal vaccine strategies are currently targeting antigen to Peyer's patch M cells through conjugation to GP2.Citation90 However, no study to-date in fish has examined what cell types express GP2 and whether these cells may be involved in antigen delivery. Of particular interest, would be the examination of antigen delivery capacity of catfish goblet cells, given reports of this capacity in mice.Citation91
IgGFc-binding protein (FcgammaBP)
A final notable mucosal factor in catfish is IgGFc-binding protein (FcgammaBP). In humans, this protein was first reported to be expressed in colonic epithelial cells and to exhibit a mucin-like structure.Citation92 However, the functions of this protein have remained largely unexplored, even in mammals. We have again consistently observed differential expression of this gene in skin, gill, and intestine of catfish following fasting and/or bacterial infection. Recent HIV vaccine trial data has revealed that Fcgbp is part of a net-like scaffold within the mucosa of the cervix and colon, and that it is an important component of the innate immune system with the ability to bind the Fc of IgG antibodies.Citation93 The author finds evidence that this mechanism traps the virus within the mucus, preventing it from contacting and infecting the epithelium. Further research is needed to understand how catfish Fcgbp may interact with mucosal immunoglobulin in capturing bacterial antigens.
Adaptive Immunity at Catfish Mucosal Barriers
Humoral adaptive immunity
As reviewed above, the innate immune system plays an important role in detecting and activating an early response to infection. However, it is often the adaptive immune system and its effector cells that ultimately eliminate or minimize pathogenic organisms. As with mammals, teleost fish produce different immunoglobulins (Ig). In catfish, there are 2 Ig isotypes; IgM and IgD (no catfish mucosal Ig has been identified). In this section, we will discuss studies using the channel catfish to characterize the humoral immune system and comment on the importance of antibody to help protect the mucosal sites.
In the late 1960s and early 1970s there was an abundance of research using different teleost fish models to understand the similarity to their mammalian counterparts.Citation94-98 It had already been surmised that there were specific gamma globulin proteins in the serum of different fish species that were responsible for humoral immunity;Citation96,97 and soon after these components would be described as IgM fractions that are similar to the molecular structure found in mammals.Citation99,100
Early studies identified similar immune-reactive IgM fractions in mucus as seen in the serum, suggesting that this antibody (Ab) protection moved beyond being a serum-only component.Citation101,102 During this time, labs were initiating different Ab-mediated immunity through the use of simple and complex antigens. In two such studies, catfish were immunized either with bovine albuminCitation101 or killed Salmonella paratyphiCitation102 through intraperitoneal injection, and therefore a systemic immune response was the likely the outcome. Di Conza and Halliday Citation101 did not detect BA specific mucosal Ab and reasoned that under normal conditions the immune response would need to be initiated in the skin and Ab would be produced locally. Ourth however was able to detect S. paratyphi specific Abs in both the serum and mucus and inferred that they had likely transudated from the serum;Citation102 however he did not rule out the possibility that some lymphocytes in the epithelial of skin could have also locally responded to some circulating antigen. Later studies would address the production of mucosal Ab after bath immersion with conjugated DNP-Horse serum albumin.Citation103 This work demonstrated that a local Ab response had occurred within the skin, because anti-DNP Abs resolved from the mucus were not also found in the serum. This work further suggested that a T-dependent response occurred in the mucosal tissues; other work in teleost fish had already established a hapten-carrier effect can result from systemic immunization, and it had also been shown that catfish have separate B and T cell populations.Citation104-106
In the late eighties, new methodologies were established to characterize and identify B cells and Ab responses in the channel catfish.Citation107,108 The development of different catfish IgM monoclonal Abs would allow for more quantitative monitoring of humoral immune responses. Most of these studies have used fish pathogens as models for activating adaptive immune responses in the channel catfish. One of these studies evaluated the serum and mucosal Ab response after immersion with the gram negative bacterium, E. ictaluri.Citation109 After 14 days, the serum Ig was 20-fold above pre-immune values and the mucus Ig at 27 days was 6-fold above pre-immune values. The authors cited a positive correlation between total Ig and the E. ictaluri observed Ab in the serum among the animals, suggesting de novo synthesis of serum Ig in response to the infection. The authors also concluded that independent production of Ab in the mucus had occurred because their data did not show a correlation between production of serum and mucus Ig within the same animals. This would suggest that perhaps some of the animals underwent a mucosal response and then produced antibody locally, while others initiated systemic Ab responses that then did not correlate with their production of mucus Abs. Another study using the fish pathogen F. columnare immunized catfish through intraperitoneal injection. This study was different than those discussed above, in that, for this study, skin was excised from immunized animals and put into tissue culture, and ELISAs were conducted to assess for the production of F. columnare specific Abs. The authors concluded that the Abs detected had been made locally and were not transudated from the serum.Citation31
I. multifiliis (Ich) is a common skin parasite of channel catfish caused by a ciliated protozoan and serves as a good model to study the mucosal humoral defense. To assess the protective capability of mucus-derived Abs, one study housed Ich-immune fish with non-immune animals and then exposed all of them to theronts (free-living stage of Ich) and assessed for their rate of infectivity. Their study showed that Anti-Ich Abs produced by immune fish could offer moderate protection to non-immune animals, and that these Abs could be detected in the water of the challenge system.Citation110 Another study using Ich took another approach in that they immunized catfish through intraperitoneal injection, and then, rather than simply evaluate whether Ich Abs had been made, they quantified the number of Ab-secreting cells in the skin and head kidney.Citation111 They initially showed a roughly 20-fold increase in the number of ASC present in the skin of immune versus the non-immune catfish, while there was little to no increase in ASC in the head kidney. They also were able to show that Ich-specific ASC were present among the skin, but represented a small portion of the total ASC population. Another experiment showed that skin PBMC induced with LPS developed into ASC in culture. These combined data suggest that B cells that recognize Ich antigens have undergone activation and developed into ASC in the skin. Because these Ich-specific ASC are also present in the head kidney and result in serum Ab to Ich, it would seem that both the systemic and mucosal humoral immunity have been activated through intraperitoneal immunization protocol. Because skin B cells can also give rise to ASC independent of the systemic immune system (LPS experiments), it would seem that mucosal Ab immunity can occur independently, as well as maintain memory to Ich in the skin even after several years.Citation112
We would be remiss if we did not at least briefly discuss what is known about the only other Ig isotype identified in catfish. There are 3 types of IgD+ cells: IgM+/IgD+ B cells, IgM-/IgD+ B cells, and an IgD+ granulocyte population that express exogenously-derived IgD by means of a putative IgD-binding receptor and are negative for IgD, IgM, and TCR transcript.Citation113,114 Intriguingly, analysis of secreted IgD transcripts shows the absence of a V domain, and IgM-/IgD+ B cells are thought to expand in response to certain pathogens and the Fc region serves as a pattern recognition molecule, similar to human IgD. Currently no work has been carried out examining the role of this B cell subset in the mucosal sites of the channel catfish. There has been a study using rainbow trout that characterized a similar B-cell subset (IgM-/IgD+/CCR7+) that is predominantly found in the gill and undergoes migration to the head kidney during a viral infection.Citation115
We don't currently have a complete view on how the mucosal adaptive immune system operates in teleost fish. It would be of interest to investigate B-cell lineage development at the mucosal sites in response to antigen both under normal and disease conditions, including clarifying the nature of expected mucosal Ig isotypes in channel catfish. There remains a disparity between activation of systemic and mucosal, or mucosal alone, adaptive immunity in the channel catfish, and this is something that will need to be further evaluated in future work. In this regard, transcriptomic studies by our group provide evidence of pathogen-induced expression of polymeric Ig receptor (pIgR) genes in mucosal tissues. In mammals, pIgR is expressed by mucosal epithelia and is responsible for binding and transcytosis of IgM and IgA.Citation6 Short-term fasting in channel catfish down-regulated expression of pIgR in skin and gill, as part of a broader suppression of innate immune factors.Citation30 However, pIgR was induced (>5-fold) following A. hydrophila infection in the skin. Future binding assays with catfish pIgR may help to more clearly delineate mucosal Ig subtypes.Citation116
Mucosal T-cell mediated immunity
The development of immune reagents such as antibodies specific for surface markers displayed by catfish leukocytes has allowed for great gains in knowledge of immune cell abundance and localization patterns under homeostatic and pathological conditions. Likewise, the rising utilization of next-generation sequencing platforms such as RNA-seq has propelled the rate of discovery in the catfish model system. In this section, we highlight some fundamental discoveries and key knowledge gaps related to T cells in catfish mucosa, with more recent discoveries characterizing transcriptional signatures in mucosal barrier tissues at rest and after encountering pathogenic insults.
αβ T cells
The existence of T cells in channel catfish was alluded to some time ago in a series of in vitro studies which focused on the ability of surface Ig- cells to provide “help” in generating primary antibody responses to thymus-dependent antigens in mixed leukocyte cultures (namely Ig+ cells and monocyte/macrophages).Citation117,118 Soon following, monoclonal antibodies were developed which reacted with T lymphocytes (although in some cases cross-reacting with other cell types) which formed the basis for flow cytometry-based investigations.Citation108,119,120 The unequivocal confirmation of T cells in channel catfish can be ascribed to the cloning of TCRα and TCRβ genes and the establishment of clonal T cell lines.Citation121-124 Indeed, much of what is known regarding catfish T cells arose from the development of these T cell lines, which were produced by limiting dilutions of peripheral blood lymphocytes (PBL) isolated from a single fish that had been immunized repeatedly with the catfish autonomous B cell line 3B11.Citation122 While not focused on mucosal populations, this landmark work on catfish cytolytic cells highlighted populations of CTLs based on alloreactivity and TCRαβ expression. The lines are classified as non-autonomous because occasional stimulation with the allogeneic 3B11 cells is required.Citation124 The vast majority of the clones were CTLs; however, one clone, which expressed TCRαβ, did not exhibit cytolytic activity but exerted a proliferative response to the presence of 3B11 cells and was classified as a putative alloantigen-specific T helper cell.Citation122
In mammals and birds, αβ T cell subsets are defined by CD4 or CD8 expression, which signify T helper cells and cytotoxic T cells, respectively. CD4 acts as a co-receptor to facilitate the engagement of the TCR with the MHC II-peptide displaying complex, while CD8 functions similarly through the context of MHC I. Further, both co-receptors initiate T cell signaling processes through phosphorylation events mediated by protein tyrosine kinases. In channel catfish, 2 CD4-like genes, CD4-1 and CD4-2 have been described.Citation125 Catfish CD4L molecules differ in the number of Ig domains with CD4L-1 consisting of 4 Ig domains, like that of mammals and chickens, with CD4L-2 comprised of 3 Ig domains. Conventional RT-PCR of several tissues revealed that levels of CD4L-1 transcripts were among the highest expressed in the gill and lowly expressed in the intestine. The levels of CD4L-2 message were similar across PBL, thymus, spleen, pronephros, mesonephros, and gill and slightly lower in the intestine. Generally speaking, if these transcriptional patterns are correlative at the cellular level, the resident patterns of CD4 cells differ from that highlighted in murine models. Whole body analyses of naïve mice showed high numbers of CD4 cells in the spleen and lymph nodes; however, after specific antigen exposures via the blood, a large increase in CD4 cells was observed in the lung and gut.Citation126 In mammals, CD8 molecules are typically found as heterodimers composed of disulfide-linked CD8a and CD8b chains. Homologs of CD8α and CD8β have recently been identified in channel catfish.Citation121 Unsurprisingly, the thymus expressed the highest levels of CD8 message; however, the gill appeared to have the second greatest levels of CD8 expression (nearly equivalent to spleen), which did vary between individual fish.Citation121
As described throughout this work, external barriers such as the gill must elicit tightly coordinated and carefully metered responses that balance protection from pathogens and host-derived inflammatory cascades. Emerging evidence suggests that the immune milieu of the gill of some teleosts may be skewed toward a Th2/Treg phenotype.Citation8,127 In salmonids, IL-4, GATA2, and LAG3 were constitutively expressed in the gill and skin, which is suggestive of an environment skewed toward Th2 responses. Citation126 The authors suggest that this immune status could offer protection against parasites while tempering overexuberent pro-inflammatory Th1 and Th17 responses. Further, the presence of the viral mimetic poly I:C failed to induce IFN-gamma production, and enhanced production of IL-4/13A reflecting a strong Th2 bias. The teleost gill features an elongated intraepithelial segment termed intrabranchial lymphoid tissue comprised of numerous CD3+ T cells and scant B cells.Citation8,128 Taken together with the shared patterns of cytokine expression; the gill lymphoid tissue and thymus are thought to be closely-related from an evolutionary perspective.Citation127
Building on the basic understanding established in the above studies, the transcriptome of channel catfish gill was examined in 2 populations of catfish with known differential susceptibilities to columnaris disease caused by the Gram negative pathogen F. columnare. The results indicated a basal polarization in mucosal immune status at rest, before a disease challenge. Among numerous differences in innate and adaptive immune effectors and messengers (many of which are highlighted elsewhere in this review), the gill derived from naïve susceptible fish showed that a suite of components related to T cell activation and stimulation, predominately cytokine receptors, were found to be constitutively expressed at higher levels than naïve resistant fish including IL-1R, IL-2R, IL-10R, IL-13R, IL-17A/F2 and IL-17RA, and MHC II. By and large, the expression of these genes remained elevated over resistant fish levels following infection with F. columnare. In contrast, resistant fish showed higher levels of IL-8 and IL-22R which mammalian models link to T cell chemotaxis and immunoregulation at mucosal barriers, respectively. Clearly, more information is needed to understand these signatures, both at steady state and after challenge. It is important to note here, that another layer of complexity exists, in that cohorts from these same 2 families of fish were shown to be inversely sensitive to another important pathogen of catfish, E. ictaluri, which causes Enteric Septicemia of Catfish (ESC).Citation129 The pathogenesis of these 2 problematic microbes is strikingly different. Columnaris disease principally affects ectopic barrier tissues, chiefly the gill and skin, while ESC causes a widespread systemic disease affecting multiple tissues. Thus, the immune phenotype exhibited by columnaris-resistant fish may be highly appropriate in delivering a measured response to the presence of F. columnare yet ineffective against inhibiting E. ictaluri colonization. Relatedly, while it is well known that E. ictaluri can gain access to the catfish host via the gastrointestinal system, bioluminescent imaging of channel catfish challenged with E. ictaluri bearing a luciferase reporter construct showed heavy loads of bacteria in the gill, a phenotype that was shortly preceded death.Citation130 Future studies, particularly those pairing global transcriptional profiles with targeted validation at the protein level (e.g., through immunohistochemical approaches) will be crucial in understanding the adaptive mechanisms that govern disease resistance/susceptibility.
γδ T cells
In humans and mice, T cells bearing the γδ TCR represent a small proportion of circulating T cells, but are found in high numbers in mucosal barriers where they exert potent cytolytic and immunoregulatory functions. Unlike their γδ T cell counterparts, γδ T cells are capable of directly recognizing and responding to antigens without the need for MHC-dependent antigen presentation or priming. Collectively, these properties have led to the classification of γδ T cells as a “bridge” between innate and adaptive immunity. Their precise function depends on a number of factors including anatomical location (e.g.,, intraepithelial lymphocytes), subset type, and state/method of activation. A variety of antigens are recognized by γδ cells and range from and microbial products and intermediates to stress determinants expressed by malignantly transformed cells. Despite substantial research progress in recent years, γδ T cells remain enigmatic in the mammalian situation, and even more so in ectothermic vertebrates. Of farmed fish species, the European sea bass (Dicentrarchus labrax) is one of the better studied species with respect to TCRγ diversity and the in vitro and in vivo functional attributes of γδ T cells. The interferon modulator poly I:C was found to induce a potent up-regulation of TCR gamma message in a mixed fraction of leukocytes cultured in vitro. Following a betanodavirus challenge, TCRγ transcripts were rapidly downregulated in the intestine and later in the kidney, presumably through changes in γδ T cell localization or trafficking patternsCitation131; similar to that described in non-human primate models of simian immunodeficiency virus.Citation131,132
One of the most intriguing and unsolved mysteries of γδ T cell biology lies in the tremendous disparity between γδ T cell diversity and abundance; with mice and primates exhibiting “low” peripheral representation, while sheep, cattle, rabbits, and chickens show “high” levels of circulating γδ-T cells.Citation133 Broadly, those species possessing low numbers of peripheral γδ T cells typically are armed with a higher number of variable TCR and Ig genes pivotal to adaptive responses. Thus, while speculative, the life history strategy of an animal, and its predilection for microbe interaction may be correlative of γδ T cell constitution; both numerically and from the standpoint of repertoire diversity. More specifically, murine and primate omnivores have a reduced need to maintain peace with a diverse population of microbiota in comparison to cows, sheep, rabbits, and chickens; wherein microbes play crucial roles in digestion and metabolism.Citation133
Evidence for γδ T cells in catfish, was hinted at in Stuge et al. Citation122 where 2 TCR αβ- clones showed varying degrees of allogeneic cell lysis. These cells were discussed as NK-like and the possibility of TCR γδ expression was not ruled out.Citation120 More recently, new insights into the diversity of γδ T cells in channel catfish were revealed by Moulana et al.Citation134. By using a combination of BLAST analyses, RACE protocols, and primer walking on bacterial artificial chromosomes (BAC), 3 Vγ families and 2 Vδ families were identified.Citation132 Efforts are currently underway to develop antibody resources to better assess the tissue localization patterns and role of these cells under homestatic conditions and disease perturbation.
The Next Mucosal Frontier—Microbiome Studies
For nearly a century, investigators have sought to characterize the microbial communities that reside upon and within fish.Citation135,136 These microbial populations have been historically referred to as the “microflora” or “microbiota” but more recently the term “microbiome” has emerged.Citation2 Irrespective of the moniker used, resident microbes comprise a select group of bacteria, and when under homeostatic situations act as symbionts. Microbiota at the interface between host and environment can serve many crucial functions including inhibiting pathogen colonization, stimulating innate immunity, and providing a protective layer to thwart environmental stressors. The composition of the microbial community represents the coevolution between host and microbes, resulting in a balanced state that is mutually beneficial for both.Citation137 The microbiota benefit by occupying a niche within the host to acquire nutrients and ensure their preservation; in turn, the microbiota contribute to host nutrition and energy balance, and to the maintenance of a functional immune system.Citation137 However, when this delicate homeostasis is breached, a dysbiosis of microbial communities can result which can dramatically alter host physiology and immunity.Citation138 In mammals, recent studies have shed light on immunological processes that participate in maintenance of homeostasis with the microbiota and on how disturbance of host immunity or the microbial ecosystem can result in disease-provoking dysbiosis.Citation137
The present understanding of microbiota in fish is largely derived from bacterial culture-based approaches, particularly in the gut, which reveal a narrow picture of microbial diversity and offer little insight into the functional roles of the isolated microbes.Citation137 Culture-based surveys greatly underestimate the diversity of microbiota, as an estimated <10% of bacteria can be isolated or cultured in laboratory settings.Citation139,140 The interaction between the assemblages of microbes in fish and host health is a topic that has been out of reach until the advent of recent technological advances such as next generation sequencing, which enable investigators to deeply profile microbial communities in host tissues of interest. High-throughput sequencing approaches based upon 16s rRNA, have brought about a new appreciation and interest in the diversity and function of the microbiota in different groups of vertebrates.Citation139
Little is known regarding the microbiome in the catfish model, particularly lacking is a basic characterization of microbial communities and how these communities shift due to changes in health status. Recently however, by utilizing 454 pyrosequencing of 16S rRNA, Larsen et al. Citation141 compared gastrointestinal microbiota across 3 commercially important species of fish (channel catfish; bluegill, Lepomis macrochirus; and largemouth bass, Micropterus salmoides) inhabiting the same lake and consuming natural food items. While several differences were documented; broadly, the gut microbial communities of all species examined were dominated by the phylum Fusobacteria, particularly the species Cetobacterium somerae. Surprisingly, the human pathogens Plesiomonas shigelloides, Fusobacterium mortiferum, and members of the genus Aeromonas, were well-represented suggesting these species are normal inhabitants of the gut. Research in other agricultural animals has been focused on controlling the microbiota, particularly within the gut, in an effort to identify or select for microbial populations or probiotic species that could yield improvements in metrics such as feed conversion, disease resistance, and overall production.Citation142 Beneficial microbes present within the gut of fish can serve as microbial barriers to infection, outcompeting pathogenic microbes for space and nutrients needed for proliferation or creating conditions inhospitable to pathogen growth.Citation143 Understanding the microbiome in catfish consuming natural prey items could be useful in developing probiotics for improving catfish growth on artificial diets. Probiotics are live microbes that can be fed as supplements to benefit the host animal by improving intestinal balance.Citation143,144 Probiotic species can function to inhibit pathogen colonization through production of antagonistic compounds, competition for attachment sites and nutrients, immune stimulation, or through nutritional benefits such as improving feed digestibility and feed utilization.Citation143
Another area in need of study is to better understand how pathogenic bacteria of catfish, such as F. columnare, can persist on mucosal surfaces of healthy fish.Citation145,146 Interestingly, F. columnare has been shown to be easily outcompeted by other species of bacteria.Citation147 However, when fish develop columnaris disease, the mucosal surfaces become burdened with high loads of F. columnare which often leads to death. Determining the extent to which F. columnare is affected by microbiome composition, and in turn, how this debilitating pathogen can alter the population composition or density of the microbiota could unlock vital clues toward identifying bacterial species that can be exploited to improve fish health.
Conclusions
Studies of catfish mucosal immunity pose challenges but also offer tremendous opportunities for both improving our understanding of teleost immunity and for making measurable improvement in the health of cultured fish. As illustrated in , a complex web of interactions shapes catfish mucosal immune responses but also provides input points for manipulating outcomes. Changing water quality, for example, in a culture system can impact the rate and nature of mucus secretions, shifting the microbiome, impacting host immunity, and changing the magnitude of responses to mucosal vaccination. Understanding and better delineating these interactions is critical for evaluating the impact of a change in culture practices on fish health and growth. With the molecular (genome and transcriptome), cellular (antibodies and cell lines), and culture resources available in channel catfish, the future looks bright for multidisciplinary studies aimed at both basic and applied studies of catfish mucosal barriers.
Figure 2. The complex interactions of environment, host, pathogen, and microbiome in catfish aquaculture pose challenges, but also offer numerous points of manipulation for research and production improvements. Arrows illustrate numerous connections and feedback mechanisms among the biotic and abiotic factors impacting catfish mucosal barriers.
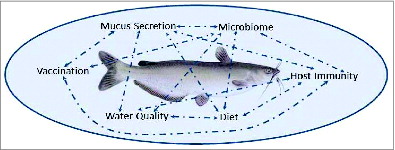
Looking beyond catfish, future discoveries may additionally help reshape mammalian immune paradigms and benefit human medicine. For example, discoveries of IgM- IgD+ subsets in catfish led to identification of similar subsets in human respiratory mucosa.Citation113,148 Similarly, the discovery of phagocytic B cells in fish, including catfish, has recently led to a re-examination of the characteristics and evolution of mammalian B-1 and B-2 cells.Citation7 On a molecular scale, functional studies of gene components critical to mucosal immune responses in catfish, will likely highlight unexplored, yet conserved, roles of orthologous genes in higher vertebrates (e.g. MFAP4, FCgammaBP, Ig-like family proteins, immune receptors.Citation149 Further development and testing of mucosal vaccines for several bacterial and parasitic pathogens of catfish,Citation150 now ongoing, may also provide valuable insights into increasing the efficacy of human vaccines delivered through nasal or oral route. All of which serves to point to one final conclusion: Catfish are an important (and delicious) mucosal model organism!
Disclosure of Potential Conflicts of Interest
No potential conflicts of interest were disclosed.
References
- Bengten E, Clem LW, Miller NW, Warr GW, Wilson M. Channel catfish immunoglobulins: repertoire and expression. Dev Comp Immunol 2006; 30:77-92; PMID:16153707; http://dx.doi.org/10.1016/j.dci.2005.06.016
- Beck BH and Peatman E eds. Mucosal health in aquaculture. Academic Press 2015; 408 pp. eBook ISBN: 9780124171930. Print Book ISBN: 9780124171862
- Gomez D, Sunyer JO, Salinas I. The mucosal immune system of fish: the evolution of tolerating commensals while fighting pathogens. Fish Shellfish Immunol 2013; 35:1729-39; PMID:24099804; http://dx.doi.org/10.1016/j.fsi.2013.09.032
- Lazado CC, Caipang CM. Mucosal immunity and probiotics in fish. Fish Shellfish Immunol 2014; 39:78-89; PMID:24795079; http://dx.doi.org/10.1016/j.fsi.2014.04.015
- Pérez T, Balcázar JL, Ruiz-Zarzuela I, Halaihel N, Vendrell D, de Blas I, Múzquiz JL. Host-microbiota interactions within the fish intestinal ecosystem. Mucosal Immunol 2010; 3:355-60; http://dx.doi.org/10.1038/mi.2010.12
- Rombout JH, Yang G, Kiron V. Adaptive immune responses at mucosal surfaces of teleost fish. Fish Shellfish Immunol 2014; 40:634-43; PMID:25150451; http://dx.doi.org/10.1016/j.fsi.2014.08.020
- Sunyer JO. Fishing for mammalian paradigms in the teleost immune system. Nat. Immunol 2013; 14:320-6; PMID:23507645; http://dx.doi.org/10.1038/ni.2549
- Koppang EO, Fischer U, Moore L, Tranulis MA, Dijkstra JM, Kollner B, Aune L, Jirillo E, Hordvik I. Salmonid T cells assemble in the thymus, spleen and in novel interbranchial lymphoid tissue. J Anat 2010; 217:728-39; PMID:20880086; http://dx.doi.org/10.1111/j.1469-7580.2010.01305.x
- Salinas I, Zhang YA, Sunyer JO. Mucosal immunoglobulins and B cells of teleost fish. Dev Comp Immunol 2011; 35:1346-65; PMID:22133710; http://dx.doi.org/10.1016/j.dci.2011.11.009
- Ángeles Esteban M. An overview of the immunological defenses in fish skin. ISRN Immunology 2012; 2012:29pp. doi:10.5402/2012/853470.
- Chang WJ, Hwang PP. Development of zebrafish epidermis. Birth Defects Res, Part C 2011; 93:205-14; http://dx.doi.org/10.1002/bdrc.20215
- Hawkes J. The structure of fish skin. Cell Tissue Res 1974; 149:159-72; PMID:4424316; http://dx.doi.org/10.1007/BF00222271
- Konrádsdóttir F, Loftsson T, Sigfússon SD. Fish skin as a model membrane: structure and characteristics. J. Pharm. Pharmacol 2009; 61:121-4
- Northcutt RG. Taste bud development in the channel catfish. J Comp Neurol 2005; 482:1-16; PMID:15612020; http://dx.doi.org/10.1002/cne.20425
- Northcutt RG, Holmes PH, Albert JS. Distribution and innervation of lateral line organs in the channel catfish. J Comp Neurol 2000; 421:570-92; PMID:10842214; http://dx.doi.org/10.1002/(SICI)1096-9861(20000612)421:4%3c570::AID-CNE7%3e3.0.CO;2-6
- Xu Z, Parra D, Gomez D, Salinas I, Zhang YA, von Gersdorff Jorgensen L, Heinecke RD, Buchmann K, LaPatra S, Sunyer JO. Teleost skin, an ancient mucosal surface that elicits gut-like immune responses. Proc Natl Acad Sci U S A 2013; 110:13097-102; PMID:23884653; http://dx.doi.org/10.1073/pnas.1304319110
- Grizzle JM, Rogers WA. Anatomy and histology of the channel catfish. 1976.
- Sis RF, Ives PJ, Jones DM, Lewis DH, Haensly WE. The microscopic anatomy of the oesophagus, stomach and intestine of the channel catfish, Ictalurus punctatus. J Fish Biol 1979; 14:179-86; http://dx.doi.org/10.1111/j.1095-8649.1979.tb03508.x
- Vogel WO, Claviez M. Vascular specialization in fish, but no evidence for lymphatics. Z Naturforsch C 1981; 36:490-2
- Wardle CS. New Observations on the Lymph System of the Plaice Pleuronectes Platessa and other Teleosts. J Mar Biol Assoc U K 1971; 51:977-90; http://dx.doi.org/10.1017/S0025315400018099
- Flajnik MF. All GOD's creatures got dedicated mucosal immunity. Nature Immunol 2010; 11:777-9; http://dx.doi.org/10.1038/ni0910-777
- Rašković BS, Stanković MB, Marković ZZ, Poleksić VD. Histological methods in the assessment of different feed effects on liver and intestine of fish. J Agric Sci, Belgrade 2011; 56:87-100; http://dx.doi.org/10.2298/JAS1101087R
- Zuchelkowski EM, Pinkstaff CA, Hinton DE. Mucosubstance histochemistry in control and acid-stressed epidermis of brown bullhead catfish, lctalurus nebulosus (LeSueur). Anat Rec 1985; 212:327-35; PMID:4073548; http://dx.doi.org/10.1002/ar.1092120402
- Quiniou S-A, Bigler S, Clem L, Bly J. Effects of water temperature on mucous cell distribution in channel catfish epidermis: a factor in winter saprolegniasis. Fish Shellfish Immunol 1998; 8:1-11; http://dx.doi.org/10.1006/fsim.1997.0115
- Peatman E, Li C, Peterson BC, Straus DL, Farmer BD, Beck BH. Basal polarization of the mucosal compartment in Flavobacterium columnare susceptible and resistant channel catfish (Ictalurus punctatus). Mol Immunol 2013; 56:317-27; PMID:23895942; http://dx.doi.org/10.1016/j.molimm.2013.04.014
- Klesius PH, Pridgeon JW, Aksoy M. Chemotactic factors of Flavobacterium columnare to skin mucus of healthy channel catfish (Ictalurus punctatus). FEMS Microbiol Lett 2010; 310:145-51; PMID:20695896; http://dx.doi.org/10.1111/j.1574-6968.2010.02060.x
- Klesius PH, Shoemaker CA, Evans JJ. Flavobacterium columnare chemotaxis to channel catfish mucus. FEMS Microbiol Lett 2008; 288:216-20; PMID:18801048; http://dx.doi.org/10.1111/j.1574-6968.2008.01348.x
- Olivares-Fuster O, Bullard SA, McElwain A, Llosa MJ, Arias CR. Adhesion dynamics of Flavobacterium columnare to channel catfish Ictalurus punctatus and zebrafish Danio rerio after immersion challenge. Dis Aquat Organ 2011; 96:221; PMID:22132500; http://dx.doi.org/10.3354/dao02371
- Li C, Beck BH, Peatman E. Nutritional impacts on gene expression in the surface mucosa of blue catfish (Ictalurus furcatus). Dev Comp Immunol 2014; 44:226-34; PMID:24378224; http://dx.doi.org/10.1016/j.dci.2013.12.014
- Liu L, Li C, Su B, Beck BH, Peatman E. Short-term feed deprivation alters immune status of surface mucosa in channel catfish (Ictalurus punctatus). PloS one 2013; 8:e74581; PMID:24023952; http://dx.doi.org/10.1371/journal.pone.0074581
- Shoemaker C, Klesius P, Lim C, Yildirim M. Feed deprivation of channel catfish, Ictalurus punctatus (Rafinesque), influences organosomatic indices, chemical composition and susceptibility to Flavobacterium columnare. J Fish Dis 2003; 26:553-61; PMID:14575373; http://dx.doi.org/10.1046/j.1365-2761.2003.00489.x
- Chen YC, Lu YF, Li IC, Hwang SP. Zebrafish Agr2 is required for terminal differentiation of intestinal goblet cells. PLoS One 2012; 7:e34408; PMID:22514630; http://dx.doi.org/10.1371/journal.pone.0034408
- Hertzog PJ, Mansell A, van Driel IR, Hartland EL. Sculpting the immune response to infection. Nat Immunol 2011; 12:579-82; PMID:21685948; http://dx.doi.org/10.1038/ni0711-579
- Cai W, De La Fuente L, Arias CR. Biofilm formation by the fish pathogen Flavobacterium columnare: development and parameters affecting surface attachment. Appl Environ Microbiol 2013; 79:5633-42; PMID:23851087; http://dx.doi.org/10.1128/AEM.01192-13
- Beck BH, Farmer BD, Straus DL, Li C, Peatman E. Putative roles for a rhamnose binding lectin in Flavobacterium columnare pathogenesis in channel catfish Ictalurus punctatus. Fish Shellfish Immunol 2012; 33:1008-15; PMID:22960031; http://dx.doi.org/10.1016/j.fsi.2012.08.018
- Paz HB, Tisdale AS, Danjo Y, Spurr-Michaud SJ, Argüeso P, Gipson IK. The role of calcium in mucin packaging within goblet cells. Exp Geront 2003; 77:69-75
- Roberts SD, Powell MD. Comparative ionic flux and gill mucous cell histochemistry: effects of salinity and disease status in Atlantic salmon (Salmo salar L.). Comp Biochem Physiol, Part A: Mol Integr Physiol 2003; 134:525-37; PMID:12600661; http://dx.doi.org/10.1016/S1095-6433(02)00327-6
- Li C, Wang R, Su B, Luo Y, Terhune J, Beck B, Peatman E. Evasion of mucosal defenses during Aeromonas hydrophila infection of channel catfish (Ictalurus punctatus) skin. Dev Comp Immunol 2013; 39:447-55; PMID:23219904; http://dx.doi.org/10.1016/j.dci.2012.11.009
- Li C, Beck B, Su B, Terhune J, Peatman E. Early mucosal responses in blue catfish (Ictalurus furcatus) skin to Aeromonas hydrophila infection. Fish Shellfish Immunol 2013; 34:920-8; PMID:23337110; http://dx.doi.org/10.1016/j.fsi.2013.01.002
- Boutin S, Bernatchez L, Audet C, Derôme N. Network analysis highlights complex interactions between pathogen, host and commensal microbiota. PLoS One 2013; 8:e84772; PMID:24376845; http://dx.doi.org/10.1371/journal.pone.0084772
- Landeira-Dabarca A, Álvarez M, Molist P. Food deprivation causes rapid changes in the abundance and glucidic composition of the cutaneous mucous cells of Atlantic salmon Salmo salar L. J Fish Dis 2014; 37:899-909; PMID:24117614; http://dx.doi.org/10.1111/jfd.12184
- Landeira-Dabarca A, Sieiro C, Álvarez M. Change in food ingestion induces rapid shifts in the diversity of microbiota associated with cutaneous mucus of Atlantic salmon Salmo salar. J Fish Biol 2013; 82:893-906; PMID:23464550; http://dx.doi.org/10.1111/jfb.12025
- Hébert P, Ainsworth AJ, Boyd B. Histological enzyme and flow cytometric analysis of channel catfish intestinal tract immune cells. Dev Comp Immunol 2002; 26:53-62; http://dx.doi.org/10.1016/S0145-305X(01)00044-1
- Li C, Zhang Y, Wang R, Lu J, Nandi S, Mohanty S, Terhune J, Liu Z, Peatman E. RNA-seq analysis of mucosal immune responses reveals signatures of intestinal barrier disruption and pathogen entry following Edwardsiella ictaluri infection in channel catfish, Ictalurus punctatus. Fish Shellfish Immunol 2012; 32:816-27; PMID:22366064; http://dx.doi.org/10.1016/j.fsi.2012.02.004
- Hardy H, Harris J, Lyon E, Beal J, Foey AD. Probiotics, prebiotics and immunomodulation of gut mucosal defences: homeostasis and immunopathology. Nutrients 2013; 5:1869-912; PMID:23760057; http://dx.doi.org/10.3390/nu5061869
- Torrecillas S, Makol A, Benítez-Santana T, Caballero MJ, Montero D, Sweetman J, Izquierdo M. Reduced gut bacterial translocation in European sea bass (Dicentrarchus labrax) fed mannan oligosaccharides (MOS). Fish Shellfish Immunol 2011 Feb;30(2):674-81; http://dx.doi.org/10.1016/j.fsi.2010.12.020
- Sun F, Peatman E, Li C, Liu S, Jiang Y, Zhou Z, Liu Z. Transcriptomic signatures of attachment, NF-κB suppression and IFN stimulation in the catfish gill following columnaris bacterial infection. Dev Comp Immunol 2012; 38:169-80; PMID:22669032; http://dx.doi.org/10.1016/j.dci.2012.05.006
- Bergsson G, Agerberth B, Jörnvall H, Gudmundsson GH. Isolation and identification of antimicrobial components from the epidermal mucus of Atlantic cod (Gadus morhua). FEBS J 2005; 272:4960-9; PMID:16176269; http://dx.doi.org/10.1111/j.1742-4658.2005.04906.x
- Nigam AK, Kumari U, Mittal S, Mittal AK. Comparative analysis of innate immune parameters of the skin mucous secretions from certain freshwater teleosts, inhabiting different ecological niches. Fish Physiol Biochem 2012; 38:1245-56; PMID:22350522; http://dx.doi.org/10.1007/s10695-012-9613-5
- Davis KM, Weiser JN. Modifications to the peptidoglycan backbone help bacteria to establish infection. Infect Immun 2011; 79:562-70; PMID:21041496; http://dx.doi.org/10.1128/IAI.00651-10
- Marsh MB, Rice CD. Development, characterization, and technical applications of a fish lysozyme-specific monoclonal antibody (mAb M24-2). Comp Immunol Microbiol Infect Dis. 2010;33:e15-23; PMID:19900707; http://dx.doi.org/10.1016/j.cimid.2009.10.002
- Sveinbjornsson B, Olsen R, Paulsen S. Immunocytochemical localization of lysozyme in intestinal eosinophilic granule cells (EGCs) of Atlantic salmon, Salmo salar L. J Fish Dis. 1996;19:349-355; http://dx.doi.org/10.1111/j.1365-2761.1996.tb00373.x
- Bilodeau AL, Waldbieser GC. Activation of TLR3 and TLR5 in channel catfish exposed to virulent Edwardsiella ictaluri. Dev Comp Immunol 2005; 29:713-21; PMID:15854683; http://dx.doi.org/10.1016/j.dci.2004.12.002
- Bilodeau-Bourgeois L, Bosworth BG, Peterson BC. Differences in mortality, growth, lysozyme, and toll-like receptor gene expression among genetic groups of catfish exposed to virulent Edwardsiella ictaluri. Fish Shellfish Immunol 2008; 24:82-9; PMID:18023209; http://dx.doi.org/10.1016/j.fsi.2007.09.001
- Yazawa R, Hirono I, Aoki T. Transgenic zebrafish expressing chicken lysozyme show resistance against bacterial diseases. Transgenic Res 2006; 15:385-91; PMID:16779653; http://dx.doi.org/10.1007/s11248-006-0009-0
- Hansen JD, Vojtech LN, Laing KJ. Sensing disease and danger: A survey of vertebrate PRRs and their origins. Dev Comp Immunol 2011; 35:886-97; PMID:21241729; http://dx.doi.org/10.1016/j.dci.2011.01.008
- Palti Y. Toll-like receptors in bony fish: from genomics to function. Dev Comp Immunol 2011; 35:1263-72; PMID:21414346; http://dx.doi.org/10.1016/j.dci.2011.03.006
- Quiniou SM, Boudinot P, Bengtén E. Comprehensive survey and genomic characterization of Toll-like receptors (TLRs) in channel catfish, Ictalurus punctatus: identification of novel fish TLRs. Immunogenetics 2013; 65:511-30; PMID:23558557; http://dx.doi.org/10.1007/s00251-013-0694-9
- Zhang J, Liu S, Rajendran KV, Sun L, Zhang Y, Sun F, Kucuktas H, Liu H, Liu Z. Pathogen recognition receptors in channel catfish: III phylogeny and expression analysis of Toll-like receptors. Developmental and comparative immunology 2013; 40:185-94; PMID:23396097; http://dx.doi.org/10.1016/j.dci.2013.01.009
- Zhao F, Li YW, Pan HJ, Shi CB, Luo XC, Li AX, Wu SQ. Expression profiles of toll-like receptors in channel catfish (Ictalurus punctatus) after infection with Ichthyophthirius multifiliis. Fish Shellfish Immunol 2013; 35:993-7; PMID:23742868; http://dx.doi.org/10.1016/j.fsi.2013.05.023
- Rajendran KV, Zhang J, Liu S, Kucuktas H, Wang X, Liu H, Sha Z, Terhune J, Peatman E, Liu Z. Pathogen recognition receptors in channel catfish: I. Identification, phylogeny and expression of NOD-like receptors. Dev Comp Immunol 2012; 37:77-86; PMID:22200599; http://dx.doi.org/10.1016/j.dci.2011.12.005
- Sha Z, Abernathy JW, Wang S, Li P, Kucuktas H, Liu H, Peatman E, Liu Z. NOD-like subfamily of the nucleotide-binding domain and leucine-rich repeat containing family receptors and their expression in channel catfish. Dev Comp Immunol 2009; 33:991-9; PMID:19414032; http://dx.doi.org/10.1016/j.dci.2009.04.004
- Rajendran KV, Zhang J, Liu S, Peatman E, Kucuktas H, Wang X, Liu H, Wood T, Terhune J, Liu Z. Pathogen recognition receptors in channel catfish: II. Identification, phylogeny and expression of retinoic acid-inducible gene I (RIG-I)-like receptors (RLRs). Dev Comp Immunol 2012; 37:381-9; PMID:22387588; http://dx.doi.org/10.1016/j.dci.2012.02.004
- Vasta GR. Roles of galectins in infection. Nat Rew Immunol 2009; 7:424-38
- Vasta GR, Nita-Lazar M, Giomarelli B, Ahmed H, Du S, Cammarata M, Parrinello N, Bianchet MA, Amzel LM. Structural and functional diversity of the lectin repertoire in teleost fish: relevance to innate and adaptive immunity. Dev Comp Immunol 2011;35:1388-99; PMID:21896283; http://dx.doi.org/10.1016/j.dci.2011.08.011
- Al-Banaw A, Kenngott R, Al-Hassan JM, Mehana N, Sinowatz F. Histochemical analysis of glycoconjugates in the skin of a catfish (Arius tenuispinis, day). Anat Histol Embryol 2010;39:42-50; PMID:19839984; http://dx.doi.org/10.1111/j.1439-0264.2009.00977.x
- Tsutsui S, Yamaguchi M, Hirasawa A, Nakamura O, Watanabe T. Common skate (Raja kenojei) secretes pentraxin into the cutaneous secretion: The first skin mucus lectin in cartilaginous fish. J Biochem 2009; 146:295-306; PMID:19416957; http://dx.doi.org/10.1093/jb/mvp069
- Ourth DD, Narra MB, Simco BA. Comparative study of mannose-binding C-type lectin isolated from channel catfish (Ictalurus punctatus) and blue catfish (Ictalurus furcatus). Fish Shellfish Immunol 2007; 23:1152-60; PMID:17950622; http://dx.doi.org/10.1016/j.fsi.2007.03.014
- Peterson BC, Peatman E, Ourth DD, Waldbieser GC. Effects of a phytogenic feed additive on growth performance, susceptibility of channel catfish to Edwardsiella ictaluri and levels of mannose binding lectin. Fish Shellfish Immunol 2015;44:21-5; PMID:25659231; http://dx.doi.org/10.1016/j.fsi.2015.01.027
- Zhang NN, Yao L, Zhuang MQ, Wang GC, Chen TT, Yang YJ, Zhang J, Lv M, Jin L. ; Association between mannose-binding lectin 2 gene and protein kinase C-beta 1 gene polymorphisms and type 2 diabetic macrovascular complications in northern Chinese Han population.. Zhonghua Yi Xue Yi Chuan Xue Za Zhi. 2012; 29:709-14; PMID:23225056
- Rajan B, Fernandes JM, Caipang CM, Kiron V, Rombout JH, Brinchmann MF. Proteome reference map of the skin mucus of Atlantic cod (Gadus morhua) revealing immune competent molecules. Fish Shellfish Immunol 2011; 31:224-31; PMID:21609766; http://dx.doi.org/10.1016/j.fsi.2011.05.006
- Vasta GR, Ahmed H, Odom EW. Structural and functional diversity of lectin repertoires in invertebrates, protochordates and ectothermic vertebrates. Curr Opin Struct Biol 2004; 14:617-30; PMID:15465324; http://dx.doi.org/10.1016/j.sbi.2004.09.008
- Verrier ER, Langevin C, Benmansour A, Boudinot P. Early antiviral response and virus-induced genes in fish. Dev Comp Immunol 2011; 35:1204-14; PMID:21414349; http://dx.doi.org/10.1016/j.dci.2011.03.012
- Ogawa T, Watanabe M, Naganuma T, Muramoto K. Diversified carbohydrate-binding lectins from marine resources. J Amino Acids 2011; 2011:838914; PMID:22312473; http://dx.doi.org/10.4061/2011/838914
- Watanabe Y, Tateno H, Nakamura-Tsuruta S, Kominami J, Hirabayashi J, Nakamura O, Watanabe T, Kamiya H, Naganuma T, Ogawa T, et al. The function of rhamnose-binding lectin in innate immunity by restricted binding to Gb3. Dev Comp Immunol 2009; 33:187-97; PMID:18809432; http://dx.doi.org/10.1016/j.dci.2008.08.008
- Bah CS, Fang EF, Ng TB, Mros S, McConnell M, Bekhit Ael D. Purification and characterization of a rhamnose-binding chinook salmon roe lectin with antiproliferative activity toward tumor cells and nitric oxide-inducing activity toward murine macrophages. J Agric Food Chem 2011; 59:5720-8; PMID:21456624; http://dx.doi.org/10.1021/jf2004578
- Shiina N, Tateno H, Ogawa T, Muramoto K, Saneyoshi M, Kamiya H. Isolation and characterization of L-rhamnose-binding lectins from chum salmon (Oncorhynchus keta) eggs. Fisheries Sci 2002; 68:1352-66; http://dx.doi.org/10.1046/j.1444-2906.2002.00575.x
- Tateno H, Ogawa T, Muramoto K, Kamiya H, Saneyoshi M. Rhamnose-binding lectins from steelhead trout (Oncorhynchus mykiss) eggs recognize bacterial lipopolysaccharides and lipoteichoic acid. Biosci Biotech Bioch 2002; 66:604-12; http://dx.doi.org/10.1271/bbb.66.604
- Tateno H, Ogawa T, Muramoto K, Kamiya H, Saneyoshi M. Distribution and molecular evolution of rhamnose-binding lectins in Salmonidae: isolation and characterization of two lectins from white-spotted Charr (Salvelinus leucomaenis) eggs. Biosci Biotech Bioch 2002; 66:1356-65; http://dx.doi.org/10.1271/bbb.66.1356
- . Klesius P, Lim C, Shoemaker C. Effect of feed deprivation on innate resistance and antibody response to Flavobacterium columnare in channel catfish, Ictalurus punctatus. B Eur Assoc Fish Pat 1999; 19:156-8
- Thongda W, Li C, Luo Y, Beck BH, Peatman E. L-rhamnose-binding lectins (RBLs) in channel catfish, Ictalurus punctatus: Characterization and expression profiling in mucosal tissues. Dev Comp Immunol 2014; 44:320-31; PMID:24480296; http://dx.doi.org/10.1016/j.dci.2014.01.018
- Sun L, Liu S, Bao L, Li Y, Feng J, Liu Z. Claudin multigene family in channel catfish and their expression profiles in response to bacterial infection and hypoxia as revealed by meta-analysis of RNA-Seq datasets. Comp Biochem Physiol Part D Genomics Proteomics. 2015;13:60-9; PMID:25681604; http://dx.doi.org/10.1016/j.cbd.2015.01.002
- McAleer JP, Kolls JK. Directing traffic: IL-17 and IL-22 coordinate pulmonary immune defense. Immunol Rev. 2014;260:129-44; PMID:24942687; http://dx.doi.org/10.1111/imr.12183
- Wang X, Li C, Thongda W, Luo Y, Beck B, Peatman E. Characterization and mucosal responses of interleukin 17 family ligand and receptor genes in channel catfish Ictalurus punctatus. Fish Shellfish Immunol 2014; 38:47-55; PMID:24602926; http://dx.doi.org/10.1016/j.fsi.2014.02.020
- Guo FH, De Raeve HR, Rice TW, Stuehr DJ, Thunnissen FB, Erzurum SC. Continuous nitric oxide synthesis by inducible nitric oxide synthase in normal human airway epithelium in vivo. Proc Natl Acad Sci U S A 1995;92:7809-13; PMID:7544004; http://dx.doi.org/10.1073/pnas.92.17.7809
- Niu D, Peatman E, Liu H, Lu J, Kucuktas H, Liu S, Sun F, Zhang H, Feng T, Zhou Z, et al. Microfibrillar-associated protein 4 (MFAP4) genes in catfish play a novel role in innate immune responses. Dev Comp Immunol 2011; 35:568-79; PMID:21232551; http://dx.doi.org/10.1016/j.dci.2011.01.002
- Schlosser A, Thomsen T, Shipley JM, Hein PW, Brasch F, Tornøe I, Nielsen O, Skjødt K, Palaniyar N, Steinhilber W, et al. Microfibril-associated protein 4 binds to surfactant protein A (SP-A) and colocalizes with SP-A in the extracellular matrix of the lung. Scand J Immunol. 2006;64:104-16; PMID:16867155; http://dx.doi.org/10.1111/j.1365-3083.2006.01778.x
- Zakrzewska A, Cui C, Stockhammer OW, Benard EL, Spaink HP, Meijer AH. Macrophage-specific gene functions in Spi1-directed innate immunity. Blood. 2010;116:e1-11; PMID:20424185; http://dx.doi.org/10.1182/blood-2010-01-262873
- Hase K, Kawano K, Nochi T, Pontes GS, Fukuda S, Ebisawa M, Kadokura K, Tobe T, Fujimura Y, Kawano S, et al. Uptake through glycoprotein 2 of FimH(+) bacteria by M cells initiates mucosal immune response. Nature 2009; 462:226-30; PMID:19907495; http://dx.doi.org/10.1038/nature08529
- Shima H, Watanabe T, Fukuda S, Fukuoka S, Ohara O, Ohno H. A novel mucosal vaccine targeting Peyer's patch M cells induces protective antigen-specific IgA responses. Int Immunol 2014; 26:619-25; PMID:24908678; http://dx.doi.org/10.1093/intimm/dxu061
- McDole JR, Wheeler LW, McDonald KG, Wang B, Konjufca V, Knoop KA, Newberry RD, Miller MJ. Goblet cells deliver luminal antigen to CD103+ dendritic cells in the small intestine. Nature 2012; 483:345-9; PMID:22422267; http://dx.doi.org/10.1038/nature10863
- Harada N, Iijima S, Kobayashi K, Yoshida T, Brown WR, Hibi T, Oshima A, Morikawa M. Human IgGFc binding protein (FcgammaBP) in colonic epithelial cells exhibits mucin-like structure. J Biol Chem 1997; 272:15232-41; PMID:9182547; http://dx.doi.org/10.1074/jbc.272.24.15232
- Schwartz JL. Fcgbp - A Potential Viral Trap in RV144. Open AIDS J 2014; 8:21-4; PMID:25246998; http://dx.doi.org/10.2174/1874613601408010021
- Bradshaw CM, Clem LW, Sigel MM. Immunologic and immunochemical studies on the gar, Lepisosteus platyrhincus. I. Immune responses and characterization of antibody. J Immunol 1969; 103:496-504; PMID:4979778
- Clem LW. Functional aspects of fish antibodies. Transplant Proc 1970; 2:260-2; PMID:4107301
- Clem LW, Small PA, Jr. Phylogeny of immunoglobulin structure and function. V. Valences and association constants of teleost antibodies to a haptenic determinant. J Exp Med 1970; 132:385-400; PMID:4109109; http://dx.doi.org/10.1084/jem.132.3.385
- Leslie GA, Clem LW. Production of anti-hapten antibodies by several classes of lower vertebrates. J Immunol 1969; 103:613-7; PMID:4185045
- Sigel MM, Clem LW. Antibody response of fish to viral antigens. Ann N Y Acad Sci 1965; 126:662-77; PMID:4287087; http://dx.doi.org/10.1111/j.1749-6632.1965.tb14312.x
- Hall SJ, Evans EE, Dupree HK, Acton RT, Weinheimer PF, Bennett JC. Characterization of a teleost immunoglobulin: the immune macroglobulin from the channel catfish, Ictalurus punctatus. Comp Biochem Physiol B Comp Biochem 1973; 46:187-97; http://dx.doi.org/10.1016/0305-0491(73)90060-6
- Mestecky J, Kulhavy R, Schrohenloher RE, Tomana M, Wright GP. Identification and properties of J chain isolated from catfish macroglobulin. J Immunol 1975; 115:993-7; PMID:809510
- Di Conza JJ, Halliday WJ. Relationship of catfish serum antibodies to immunoglobulin in mucus secretions. Aust J Exp Biol Med Sci 1971; 49:517-9; PMID:4110769; http://dx.doi.org/10.1038/icb.1971.56
- Ourth DD. Secretory IgM, lysozyme and lymphocytes in the skin mucus of the channel catfish, Ictalurus punctatus. Dev Comp Immunol 1980; 4:65-74; PMID:6768604; http://dx.doi.org/10.1016/S0145-305X(80)80009-7
- Lobb CJ. Secretory immunity induced in catfish, Ictalurus punctatus, following bath immunization. Dev Comp Immunol 1987; 11:727-38; PMID:3440500; http://dx.doi.org/10.1016/0145-305X(87)90060-7
- Lobb CJ, Clem LW. Fish lymphocytes differ in the expression of surface immunoglobulin. Dev Comp Immunol 1982; 6:473-9; PMID:6813154; http://dx.doi.org/10.1016/S0145-305X(82)80033-5
- Lobb CJ, Olson MO, Clem LW. Immunoglobulin light chain classes in a teleost fish. J Immunol 1984; 132:1917-23; PMID:6421929
- Yocum D, Cuchens M, Clem LW. The hapten-carrier effect in teleost fish. J Immunol 1975; 114:925-7; PMID:46263
- Klesius PH. Effect of size and temperature on the quantity of immunoglobulin in channel catfish, Ictalurus punctatus. Vet Immunol Immunopathol 1990; 24:187-95; PMID:2110694; http://dx.doi.org/10.1016/0165-2427(90)90021-J
- Miller NW, Bly JE, van Ginkel F, Ellsaesser CF, Clem LW. Phylogeny of lymphocyte heterogeneity: identification and separation of functionally distinct subpopulations of channel catfish lymphocytes with monoclonal antibodies. Dev Comp Immunol 1987; 11:739-47; PMID:3326758; http://dx.doi.org/10.1016/0145-305X(87)90061-9
- Zilberg D, Klesius PH. Quantification of immunoglobulin in the serum and mucus of channel catfish at different ages and following infection with Edwardsiella ictaluri. Vet Immunol Immunopathol 1997; 58:171-80; PMID:9336885; http://dx.doi.org/10.1016/S0165-2427(97)00033-0
- Xu DH, Klesius PH. Protective effect of cutaneous antibody produced by channel catfish, Ictalurus punctatus (Rafinesque), immune to Ichthyophthirius multifiliis Fouquet on cohabited non-immune catfish. J Fish Dis 2003; 26:287-91; PMID:12962237; http://dx.doi.org/10.1046/j.1365-2761.2003.00463.x
- Zhao X, Findly RC, Dickerson HW. Cutaneous antibody-secreting cells and B cells in a teleost fish. Dev Comp Immunol 2008; 32:500-8; PMID:18045689; http://dx.doi.org/10.1016/j.dci.2007.08.009
- Findly RC, Zhao X, Noe J, Camus AC, Dickerson HW. B cell memory following infection and challenge of channel catfish with Ichthyophthirius multifiliis. Dev Comp Immunol 2013; 39:302-11; PMID:23041614; http://dx.doi.org/10.1016/j.dci.2012.08.007
- Edholm ES, Bengten E, Stafford JL, Sahoo M, Taylor EB, Miller NW, Wilson M. Identification of two IgD+ B cell populations in channel catfish, Ictalurus punctatus. J Immunol 2010; 185:4082-94; PMID:20817869; http://dx.doi.org/10.4049/jimmunol.1000631
- Edholm ES, Bengten E, Wilson M. Insights into the function of IgD. Dev Comp Immunol 2011; 35:1309-16; PMID:21414345; http://dx.doi.org/10.1016/j.dci.2011.03.002
- . Castro R, Bromage E, Abós B, Pignatelli J, González Granja A, Luque A, Tafalla C. CCR7 is mainly expressed in teleost gills, where it defines an IgD+IgM- B lymphocyte subset. J Immunol 2014; 192:1257-66; PMID:24353268; http://dx.doi.org/10.4049/jimmunol.1302471
- Hamuro K, Suetake H, Saha NR, Kikuchi K, Suzuki Y. A teleost polymeric Ig receptor exhibiting two Ig-like domains transports tetrameric IgM into the skin. J Immunol 2007; 178:5682-9; PMID:17442951; http://dx.doi.org/10.4049/jimmunol.178.9.5682
- Miller NW, Sizemore R, Clem L. Phylogeny of lymphocyte heterogeneity: the cellular requirements for in vitro antibody responses of channel catfish leukocytes. J Immunol 1985; 134:2884-8; PMID:2580005
- Sizemore R, Miller N, Cuchens M, Lobb C, Clem L. Phylogeny of lymphocyte heterogeneity: the cellular requirements for in vitro mitogenic responses of channel catfish leukocytes. J Immunol 1984; 133:2920-4; PMID:6491279
- Ainsworth AJ, Dexiang C, Greenway T. Characterization of monoclonal antibodies to channel catfish, Ictalurus punctatus, leucocytes. Vet Immunol Immunopathol 1990; 26:81-92; PMID:2251768; http://dx.doi.org/10.1016/0165-2427(90)90134-E
- Ellsaesser CF, Bly JE, Clem L. Phylogeny of lymphocyte heterogeneity: the thymus of the channel catfish. Dev Comp Immunol 1988; 12:787-99; PMID:3264795; http://dx.doi.org/10.1016/0145-305X(88)90053-5
- Quiniou SM, Sahoo M, Edholm E-S, Bengten E, Wilson M. Channel catfish CD8α and CD8β co-receptors: characterization, expression and polymorphism. Fish Shellfish Immunol 2011; 30:894-901; PMID:21272650; http://dx.doi.org/10.1016/j.fsi.2011.01.011
- Stuge TB, Wilson MR, Zhou H, Barker KS, Bengtén E, Chinchar G, Miller NW, Clem LW. Development and analysis of various clonal alloantigen-dependent cytotoxic cell lines from channel catfish. J Immunol 2000; 164:2971-7; PMID:10706684; http://dx.doi.org/10.4049/jimmunol.164.6.2971
- Wilson M, Zhou H, Bengten E, Clem L, Stuge T, Warr G, Miller NW. T-cell receptors in channel catfish: structure and expression of TCR α and β genes. Mol Immunol 1998; 35:545-57; PMID:9809582; http://dx.doi.org/10.1016/S0161-5890(98)00037-6
- Zhou H, Stuge TB, Miller NW, Bengten E, Naftel JP, Bernanke JM, Chinchar VG, Clem LW, Wilson M. Heterogeneity of channel catfish CTL with respect to target recognition and cytotoxic mechanisms employed. J Immunol 2001; 167:1325-32; PMID:11466349; http://dx.doi.org/10.4049/jimmunol.167.3.1325
- Edholm E-S, Stafford JL, Quiniou SM, Waldbieser G, Miller NW, Bengtén E, Wilson M. Channel catfish, Ictalurus punctatus, CD4-like molecules. Dev Comp Immunol 2007; 31:172-87; PMID:16844219; http://dx.doi.org/10.1016/j.dci.2006.05.012
- Reinhardt RL, Khoruts A, Merica R, Zell T, Jenkins MK. Visualizing the generation of memory CD4 T cells in the whole body. Nature 2001; 410:101-5; PMID:11242050; http://dx.doi.org/10.1038/35065111
- Takizawa F, Koppang EO, Ohtani M, Nakanishi T, Hashimoto K, Fischer U, Dijkstra JM. Constitutive high expression of interleukin-4/13A and GATA-3 in gill and skin of salmonid fishes suggests that these tissues form Th2-skewed immune environments. Mol Immunol 2011; 48:1360-8; PMID:21489635; http://dx.doi.org/10.1016/j.molimm.2011.02.014
- Haugarvoll E, Bjerkås I, Nowak BF, Hordvik I, Koppang EO. Identification and characterization of a novel intraepithelial lymphoid tissue in the gills of Atlantic salmon. J Anat 2008; 213:202-9; PMID:19172734; http://dx.doi.org/10.1111/j.1469-7580.2008.00943.x
- LaFrentz BR, Shoemaker CA, Booth NJ, Peterson BC, Ourth DD. Spleen index and mannose-binding lectin levels in four channel catfish families exhibiting different susceptibilities to Flavobacterium columnare and Edwardsiella ictaluri. J Aquat Anim Health 2012; 24:141-7; PMID:22870893; http://dx.doi.org/10.1080/08997659.2012.675936
- Karsi A, Menanteau-Ledouble S, Lawrence ML. Development of bioluminescent Edwardsiella ictaluri for noninvasive disease monitoring. FEMS Microbiol Lett 2006; 260:216-23; PMID:16842347; http://dx.doi.org/10.1111/j.1574-6968.2006.00310.x
- Buonocore F, Castro R, Randelli E, Lefranc M-P, Six A, Kuhl H, Reinhardt R, Facchiano A, Boudinot P, Scapigliati G. Diversity, molecular characterization and expression of T cell receptor γ in a teleost fish, the sea bass (Dicentrarchus labrax, L). PloS one 2012; 7:e47957; PMID:23133531; http://dx.doi.org/10.1371/journal.pone.0047957
- Kosub DA, Durudas A, Lehrman G, Milush JM, Cano CA, Jain MK, Sodora DL. Gamma/Delta T cell mRNA levels decrease at mucosal sites and increase at lymphoid sites following an oral SIV infection of macaques. Curr HIV Res 2008; 6:520; PMID:18991617; http://dx.doi.org/10.2174/157016208786501490
- Kalyan S, Kabelitz D. Defining the nature of human γδ T cells: a biographical sketch of the highly empathetic. Cell Mol Immunol 2013;10:21-9; PMID:23085947; http://dx.doi.org/10.1038/cmi.2012.44
- Moulana M, Taylor EB, Edholm E-S, Quiniou SM, Wilson M, Bengtén E. Identification and characterization of TCRγ and TCRδ chains in channel catfish, Ictalurus punctatus. Immunogenetics 2014; 66:545-61; PMID:25129471; http://dx.doi.org/10.1007/s00251-014-0793-2
- Reed GB, Spence CM. The Intestinal and slime flora of the haddock: a preliminary report. Contributions to Canadian Biology and Fisheries 1929; 4:257-64; http://dx.doi.org/10.1139/f29-019
- Harrison FC. The discoloration of halibut. Can J Res 1929; 1:214-39; http://dx.doi.org/10.1139/cjr29-013
- Littman DR, Pamer EG. Role of the commensal microbiota in normal and pathogenic host immune responses. Cell Host Microbe 2011; 10:311-23; PMID:22018232; http://dx.doi.org/10.1016/j.chom.2011.10.004
- Musharrafieh R, Tacchi L, Trujeque J, LaPatra S, Salinas I. Staphylococcus warneri, a resident skin commensal of rainbow trout (Oncorhynchus mykiss) with pathobiont characteristics. Vet Microbiol 2014; 169:80-8; PMID:24438987; http://dx.doi.org/10.1016/j.vetmic.2013.12.012
- Clements KD, Angert ER, Montgomery WL, Choat JH. Intestinal microbiota in fishes: what's known and what's not. Mol Ecol 2014; 23:1891-8; PMID:24612310; http://dx.doi.org/10.1111/mec.12699
- Larsen A, Tao Z, Bullard SA, Arias CR. Diversity of the skin microbiota of fishes: evidence for host species specificity. FEMS Microbiol Ecol 2013; 85:483-94; PMID:23607777; http://dx.doi.org/10.1111/1574-6941.12136
- Larsen AM, Mohammed HH, Arias CR. Characterization of the gut microbiota of three commercially valuable warmwater fish species. J Appl Microbiol 2014; 116:1396-404; PMID:24529218; http://dx.doi.org/10.1111/jam.12475
- Danzeisen JL, Kim HB, Isaacson RE, Tu ZJ, Johnson TJ. Modulations of the chicken cecal microbiome and metagenome in response to anticoccidial and growth promoter Treatment. PLoS ONE 2011; 6:e27949; PMID:22114729; http://dx.doi.org/10.1371/journal.pone.0027949
- Kesarcodi-Watson A, Kaspar H, Lategan MJ, Gibson L. Probiotics in aquaculture: the need, principles and mechanisms of action and screening processes. Aquaculture 2008; 274:1-14; http://dx.doi.org/10.1016/j.aquaculture.2007.11.019
- Fuller R. Probiotics in man and animals. J Appl Bacteriol 1989; 66:365-78; PMID:2666378; http://dx.doi.org/10.1111/j.1365-2672.1989.tb05105.x
- Declercq AM, Haesebrouck F, Van den Broeck W, Bossier P, Decostere A. Columnaris disease in fish: a review with emphasis on bacterium-host interactions. Vet Res 2013; 44:27; PMID:23617544; http://dx.doi.org/10.1186/1297-9716-44-27
- Farmer BD, Mitchell AJ, Straus DL. The effect of high total ammonia concentration on the survival of channel catfish experimentally infected with Flavobacterium columnare. J Aquat Anim Health 2011; 23:162-8; PMID:22216715; http://dx.doi.org/10.1080/08997659.2011.616836
- Hawke JP, Thune RL. Systemic isolation and antimicrobial susceptibility of Cytophaga columnaris from commercially reared channel catfish. J Aquat Anim Hwalth 1992; 4:109-13; http://dx.doi.org/10.1577/1548-8667(1992)004%3c0109:SIAASO%3e2.3.CO;2
- Chen K, Xu W, Wilson M, He B, Miller NW, Bengtén E, Edholm ES, Santini PA, Rath P, Chiu A, et al. Immunoglobulin D enhances immune surveillance by activating antimicrobial, proinflammatory and B cell-stimulating programs in basophils. Nat Immunol. 2009;10:889-98; PMID:19561614; http://dx.doi.org/10.1038/ni.1748
- Hawke NA, Yoder JA, Haire RN, Mueller MG, Litman RT, Miracle AL, Stuge T, Shen L, Miller N, Litman GW. Extraordinary variation in a diversified family of immune-type receptor genes. Proc Natl Acad Sci U S A. 2001;98:13832-7; PMID:11698645; http://dx.doi.org/10.1073/pnas.231418598
- Wise DJ, Greenway TE, Byars TS, Griffin MJ, Khoo LH. Oral vaccination of channel catfish against enteric septicemia of catfish using a live attenuated Edwardsiella ictaluri Isolate. J Aquat Anim Health. 2015;27:135-43; PMID:26030354; http://dx.doi.org/10.1080/08997659.2015.1032440