ABSTRACT
There are many types of biologic compartments in the body. Tight junctions (TJs) function to create a dynamic paracellular barrier to separate these compartments and maintain homeostasis. The TJ component claudin-1 is the major transmembrane protein responsible for forming the paracellular barrier in the epidermis; other claudins in the epidermis include claudin-3, -4, -12, -23 and -25. Accumulating evidence points to a relationship between claudin-1 and skin diseases; for example, a decrease in claudin-1 is reported in human atopic dermatitis. However, how claudin-1 directly or indirectly contributes to disease in the context of the paracellular barrier is poorly understood. We recently established several mouse lines in which the claudin-1 expression was systematically regulated, and showed that claudin-1 time- and dose-dependently regulates epidermis function and disease in vivo. In this commentary, we will discuss recent progress on this topic, including our latest findings, and remaining or newly arisen issues.
Tight junction and claudins
Multicellular organisms, including their organs and tissues, are enclosed by an epithelial cell sheet with distinct inner and outer features. In the cell sheet, tight junctions (TJs), located at the most apical cell-cell adhesion site between epithelial cells, are critical for forming the paracellular barrier that maintains homeostasis.Citation1 TJs also determine the size and charge of molecules that can selectively pass through the barrier.Citation2 Within TJs, members of the claudin protein family are largely responsible for controlling cell-cell adhesion and the characteristics of the paracellular barrier (, ). The claudins, a multigene family with at least 27 members in human and mouse, are polymerized into TJ strandsCitation3 (). Each organ's environment is influenced by the TJ characteristics, which result from each claudin's spatiotemporal behavior at the TJ strands. Different expression patterns of claudins in organs, tissues, and epithelial cells, regulate their variety of functions.
Figure 1. Tight Junctions (TJs) are composed of claudins. (A) Schematic drawing showing the location of TJs between epithelial cells. (B) Electron micrograph of intestinal epithelial cells. TJs are located at the uppermost region of the cell-cell adhering junctional complex. Scale bar, 200 nm. (C) Freeze fracture electron micrograph of TJs. Scale bar, 200 nm. Images of (A) and (B) are modified from Tsukita et al., 2001Citation1. © Nature Reviews Molecular Cell Biology. Reproduced by permission of Nature Publishing Group. Permission to reuse must be obtained from the rightsholder.
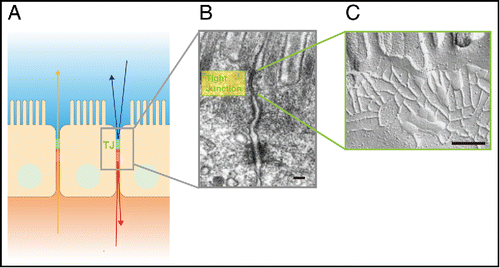
The skin epidermis mainly consists of keratinocytes. Keratinocytes undergo various differentiation steps from the basal cell layers to the stratum corneum (SC).Citation4 During this differentiation journey, the TJs are formed in the second stratum granulosum (SG2 layer) to create the paracellular barrier (). In the epidermis, claudin-1 and claudin-4 are highly expressed, while other claudins, such as claudin-3 and claudin-12, are expressed at low levels (). Claudin-1-deficient mice, which had been previously reported, showed an abnormal skin phenotype and died from water loss within 1 d of birth.Citation5 In contrast, claudin-4-deficient mice have no reported skin phenotypes.Citation6 On the other hand, overexpressing claudin-6 under the involucrin promoter in the epidermis generates the same phenotypes as the claudin-1 deficiency.Citation7 Studies using the other claudin-6 mutated transgenic mouse also highlighted the importance of specific claudins' characteristics to in vivo phenotype.Citation8,9 However, even in the epidermis, how claudin orchestrates its many effects is poorly understood. Recently, the crystal structures of claudin-4, -15, and -19 were reported; these studies suggested the structural basis for the molecules that create the TJ paracellular barriers and channels, and other claudin functions.Citation10-13 Additional structural studies should clarify how various claudins elicit their functions.
Figure 2. Claudin-1 is the main component of the TJs in skin. (A) Schematic drawing of the epidermis. TJs are formed in the SG2 layer. SG (Stratum corneum), SG (Stratum granulosum). (B) Real-time RT-PCR analysis of claudins in keratinocytes.
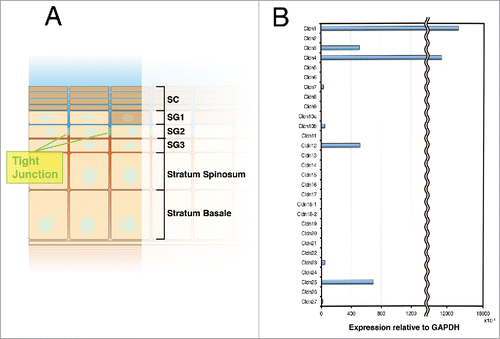
The altered expression of a gene sometimes disturbs a biologic system and leads to disease. The in vivo functions of genes have often been uncovered using a gene knockout (KO) strategy, which compares the “all versus none” situations. However, variations in gene expression levels can also change normal gene function, leading to disease in vivo. For example, claudin-1 is indispensable for the barrier function of skin, and abnormalities in claudin-1 cause human skin diseasesCitation14-17; in particular, a decrease in claudin-1 expression is reported in human atopic dermatitis (AD).Citation17,18 However, claudin-1-deficient mice die within 1 day of birth due to dehydration and abnormal skin differentiation,Citation5 so these mice cannot be used to examine the role of claudin-1 in AD. Therefore, to study the connection between molecular genetics and clinical effects in skin disorders like AD, we examined a set of mice that expressed varying levels of claudin-1.Citation19
Atopic dermatitis
AD, a common chronic inflammatory skin disease in humans, is caused by complex genetic factors, including gene-gene and gene-environmental interactions.Citation20,21 AD appears in approximately 20% of children and 3% of adults, and its symptoms include itching and eczema, which decrease the patient's quality of life.Citation22 Approximately 70% of AD cases start in children under 5 y of age, and the disease often shows spontaneous remission with aging.Citation23,24 While some AD features have been proposed to be due to immunological imbalances, such as increased levels of immunoglobulin E (IgE) and the acquired immune response, recent studies showed that epidermal barrier functions critically influence the pathological features of AD.Citation25,26 However, the cause and development of AD with respect to epidermal barrier functions had not been systematically studied. Therefore, we addressed this issue using our model mice.
Dose-dependent phenotypes of the claudin-1 expression level
In our study, we established mouse lines in which the claudin-1 expression was systematically altered. By generating mice harboring wild-type (WT, +), knockdown (KD, Δ), or knockout (KO, -) alleles in various combinations, we obtained 6 lines of mice with different claudin-1 expression levels: Cldn1+/+, Cldn1+/Δ, Cldn1+/−, Cldn1Δ/Δ, Cldn1Δ/−, and Cldn1−/− mice. To quantify the expression levels of claudin-1, we prepared primary keratinocyte cultures from newborn mice of each genotype. Real-time (RT)-PCR confirmed that the claudin-1 mRNA levels differed according to genotype. We also confirmed that the mRNA and protein levels of claudin-1 showed a strong positive correlation. The protein levels were estimated by the ratio of claudin-1 to β-actin on immunoblots. Next, we examined the paracellular barrier function by the transepithelial electrical resistance (TER) and paracellular flux (FLUX) of 4-kd dextran tracers in primary cultures of keratinocytes, and found that the barrier function in keratinocytes improved exponentially with the claudin-1 mRNA expression level. Previous reports showed that claudin-1 affects the SG, from which the SC is generated, and that Cldn1−/− newborn mice have an abnormal SC.Citation5,27 In our experimental model, H&E staining revealed that, not only Cldn1−/− but also Cldn1Δ/Δ and Cldn1Δ/− newborn mice showed abnormal differentiation of the SC, the severity of which was related to the barrier function.
Time-dependent phenotypes of the reduced claudin-1 expression levels
To understand Claudin-1's role in AD's age-related changes in humans, we examined the phenotypes of the model mice over time, from birth to adulthood. Although the Cldn1−/− mice died within 1 day of birth, the other genotypes survived to adulthood with varying mortality rates. The survival rate at 8 weeks was over 80% for Cldn1+/+, Cldn1+/Δ, Cldn1+/−, and Cldn1Δ/Δ; 4% for Cldn1Δ/−; and 0% for Cldn1−/− mice. These results suggested that the low claudin-1 expression level in Cldn1Δ/− mice was close to the threshold for lethality. In the various claudin-1 mutant mice, the reduction in claudin-1 expression levels altered the epithelial barrier functions and the morphological features of the AD tissue, including hyperkeratosis and acanthosis with aging. In addition, it caused the recruitment of neutrophils and macrophages in Th1 but not Th2 immune responses. These symptoms were apparent in the infant stages, and decreased as the mice became adults, similar to the age-dependent improvement in human AD patients.Citation23 Moreover, the Cldn1Δ/Δ epidermis, which was improved in the adult stage, still differed from the Cldn1+/+ epidermis in hapten-induced contact hypersensitivity, indicating that the recovery of the skin condition in claudin-1 KD is insufficient to defend the skin against percutaneous stimuli.
Considering that claudin-1 reduction affects the paracellular barrier (as shown in our study) and SC barrier function,Citation27,28 it is likely that reduced claudin-1 expression severely down-regulates the epidermal barrier in vivo, leading to abnormal differentiation and inflammation. On the other hand, we found that in human AD patients, the number of macrophages was inversely correlated with the claudin-1 level, although we could not find a correlation between the claudin-1 staining intensity and Th2 markers in AD patients' sera. To further explore the correlation between claudin-1 and AD, experiments performed under a variety of conditions, at a large scale, and over the long-term in mice and humans are required. Nevertheless, our study revealed that claudin-1 plays an important role in the relationship between barrier function and AD in vivo.
Open questions and future perspectives
In this study, we showed how claudin-1 time- and dose-dependently affects a skin phenotype in vivo, highlighting the importance of claudins and their barrier functions (). Not only claudins, but many genes have dose-dependent effects. To understand the time- and dose-dependent effects of genes related to diseases, development, or any other biologic process, it is important to find the quantitative threshold of gene expression that elicits a function. How the roles of genes will be “re-revealed” using such approaches is an important topic for future study.
Figure 3. Summary of our findings. (A) Claudin-1 reduction triggers epidermal barrier dysfunction, including both the paracellular barrier and the SC barrier. These dysfunctions cause abnormal differentiation and inflammation, which are also triggered and/or accelerated by environmental factors. (B) Dose-dependent claudin-1 expression influences the progression of claudin-1 mutant mouse symptoms.
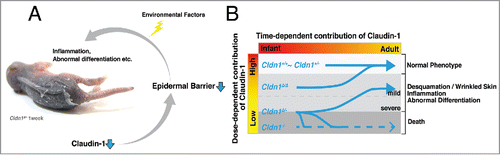
We demonstrated the pattern of effects caused by different expression levels of claudin-1, using a genetic method. On the other hand, a decreased barrier function in an organism could also be induced by environmental factors. Even in our study, which was performed in a specific-pathogen-free mouse room, inflammation was induced by environmental causes. Previous studies reported that the barrier functions of TJs in the epidermis are particularly affected by external stimuli, such as UV radiation and temperature, which can influence the AD symptoms in humans.Citation29,30 The impact of genetic and environmental influences on barrier function merits further study.
Questions also remain about how claudin-1's molecular function in AD changes with aging. Although claudin-1 KD mice mimicked the natural history of human AD cases that improve with age, we did not discover the mechanism by which AD improves with age. As different approaches, in future studies we would like to examine the effect of claudin-1 on AD in different mouse strains (as genetic factors), and using different antigen challenges that activate specific immune responses (as environmental factors).
In our study of TJ function in the skin, we focused on the time- and dose-dependency of claudin-1 and its relationship to AD symptoms. To better understand the TJs' contribution to maintaining homeostasis, we also need to learn more about the interaction between the claudin molecules on adjoining cells, because the barrier and channel functions of TJs depend on the types and expression volumes of claudins. In addition to skin, clinical studies have also shown relationships between claudin expression and cancer.Citation31 Thus, a better understanding of the roles of TJs, claudins, and barriers is important for a broad range of physiologic situations.
Disclosure of potential conflicts of interest
No potential conflicts of interest were disclosed.
Funding
This work was supported in part by Grants-in-Aid for Scientific Research (A) from the Ministry of Education, Culture, Sports, Science and Technology of Japan (MEXT) (to S.T.); by Core Research for Evolutional Science and Technology (CREST) from the Japan Science and Technology Agency (JST) (S.T.); and by Grant-in-Aid for JSPS Fellows from the Japan Society for the Promotion of Science (JSPS) (R.T.).
References
- Tsukita S, Furuse M, Itoh M. Multifunctional strands in tight junctions. Nat Rev Mol Cell Biol 2001; 2(4):285-93; PMID:11283726; https://doi.org/10.1038/35067088
- Tamura A, Tsukita S. Paracellular barrier and channel functions of TJ claudins in organizing biological systems: advances in the field of barriology revealed in knockout mice. Semin Cell Dev Biol 2014; 36:177-85; PMID:25305579; https://doi.org/10.1016/j.semcdb.2014.09.019
- Mineta K, Yamamoto Y, Yamazaki Y, Tanaka H, Tada Y, Saito K, Tamura A, Igarashi M, Endo T, Takeuchi K, et al. Predicted expansion of the claudin multigene family. FEBS Lett 2011; 585(4):606-12; PMID:21276448; https://doi.org/10.1016/j.febslet.2011.01.028
- Simpson CL, Patel DM, Green KJ. Deconstructing the skin: cytoarchitectural determinants of epidermal morphogenesis. Nat Rev Mol Cell Biol 2011; 12(9):565-80; PMID:21860392; https://doi.org/10.1038/nrm3175
- Furuse M, Hata M, Furuse K, Yoshida Y, Haratake A, Sugitani Y, Noda T, Kubo A, Tsukita S. Claudin-based tight junctions are crucial for the mammalian epidermal barrier: a lesson from claudin-1-deficient mice. J Cell Biol 2002; 156(6):1099-111; PMID:11889141; https://doi.org/10.1083/jcb.200110122
- Kage H, Flodby P, Gao D, Kim YH, Marconett CN, DeMaio L, Kim K-J, Crandall ED, Borok Z. Claudin 4 knockout mice: normal physiological phenotype with increased susceptibility to lung injury. Am J Physiol Lung Cell Mol Physiol 2014; 307(7):L524-36; PMID:25106430; https://doi.org/10.1152/ajplung.00077.2014
- Turksen K, Troy TC. Permeability barrier dysfunction in transgenic mice overexpressing claudin 6. Development 2002; 129(7):1775-84; PMID:11923212
- Troy T-C, Arabzadeh A, Larivière NMK, Enikanolaiye A, Turksen K. Dermatitis and aging-related barrier dysfunction in transgenic mice overexpressing an epidermal-targeted claudin 6 tail deletion mutant. PLoS One 2009; 4(11):e7814; PMID:19915705; https://doi.org/10.1371/journal.pone.0007814
- Enikanolaiye A, Larivière N, Troy T-C, Arabzadeh A, Atasoy E, Turksen K. Involucrin-claudin-6 tail deletion mutant (CDelta206) transgenic mice: a model of delayed epidermal permeability barrier formation and repair. Dis Model Mech 2010; 3(3-4):167-80; PMID:20106878; https://doi.org/10.1242/dmm.002634
- Suzuki H, Nishizawa T, Tani K, Yamazaki Y, Tamura A, Ishitani R, Dohmae N, Tsukita S, Nureki O, Fujiyoshi Y. Crystal structure of a claudin provides insight into the architecture of tight junctions. Science 2014; 344(6181):304-7; PMID:24744376; https://doi.org/10.1126/science.1248571
- Saitoh Y, Suzuki H, Tani K, Nishikawa K, Irie K, Ogura Y, Tamura A, Tsukita S, Fujiyoshi Y. Structural insight into tight junction disassembly by Clostridium perfringens enterotoxin. Science 2015; 347(6223):775-8; PMID: 25678664; https://doi.org/10.1126/science.1261833
- Suzuki H, Tani K, Tamura A, Tsukita S, Fujiyoshi Y. Model for the architecture of claudin-based paracellular ion channels through tight junctions. J Mol Biol 2015; 427(2):291-7; PMID:25451028; https://doi.org/10.1016/j.jmb.2014.10.020
- Shinoda T, Shinya N, Ito K, Ohsawa N, Terada T, Hirata K, Kawano Y, Yamamoto M, Kimura-Someya T, Yokoyama S, Shirouzu M. Structural basis for disruption of claudin assembly in tight junctions by an enterotoxin. Sci Rep 2016; 6:33632; https://doi.org/10.1038/srep33632
- Hadj-Rabia S, Baala L, Vabres P, Hamel-Teillac D, Jacquemin E, Fabre M, Lyonnet S, De Prost Y, Munnich A, Hadchouel M, et al. Claudin-1 gene mutations in neonatal sclerosing cholangitis associated with ichthyosis: a tight junction disease. Gastroenterology 2004; 127(5):1386-90; PMID:15521008; https://doi.org/10.1053/j.gastro.2004.07.022
- Kirschner N, Poetzl C, von den Driesch P, Wladykowski E, Moll I, Behne MJ, Brandner JM. Alteration of tight junction proteins is an early event in psoriasis: putative involvement of proinflammatory cytokines. Am J Pathol 2009; 175(3):1095-106; PMID:19661441; https://doi.org/10.2353/ajpath.2009.080973
- Kirchmeier P, Sayar E, Hotz A, Hausser I, Islek A, Yilmaz A, Artan R, Fischer J. Novel mutation in the CLDN1 gene in a Turkish family with Neonatal Ichthyosis Sclerosing Cholangitis (NISCH) syndrome. Br J Dermatol 2014; 170(4):976-8; PMID:24641442; https://doi.org/10.1111/bjd.12724
- De Benedetto A, Rafaels NM, McGirt LY, Ivanov AI, Georas SN, Cheadle C, Berger AE, Zhang K, Vidyasagar S, Yoshida T, et al. Tight junction defects in patients with atopic dermatitis. J Allergy Clin Immunol 2011; 127(3):773-86.e1-7; PMID:21163515; https://doi.org/10.1016/j.jaci.2010.10.018
- Batista DIS, Perez L, Orfali RL, Zaniboni MC, Samorano LP, Pereira N V, Sotto MN, Ishizaki AS, Oliveira LMS, Sato MN, et al. Profile of skin barrier proteins (filaggrin, claudins 1 and 4) and Th1/Th2/Th17 cytokines in adults with atopic dermatitis. J Eur Acad Dermatol Venereol 2015; 29(6):1091-5; PMID:25271795; https://doi.org/10.1111/jdv.12753
- Tokumasu R, Yamaga K, Yamazaki Y, Murota H, Suzuki K, Tamura A, Bando K, Furuta Y, Katayama I, Tsukita S. Dose-dependent role of claudin-1 in vivo in orchestrating features of atopic dermatitis. Proc Natl Acad Sci U S A. 2016; 113:E4061-8; PMID:27342862; https://doi.org/10.1073/pnas.1525474113
- Hanifin JM, Rajka G. Diagnostic features of atopic dermatitis. Acta Derm Venereol Suppl 1980; 92:44-7
- Bieber T. Atopic dermatitis. Ann Dermatol 2010; 22(2):125-37; PMID:20548901; https://doi.org/10.5021/ad.2010.22.2.125
- Kim BE, Leung DY. Epidermal barrier in atopic dermatitis. Allergy Asthma Immunol Res 2012; 4(1):12-6; PMID:22211165; https://doi.org/10.4168/aair.2012.4.1.12
- Beltrani VS. The clinical spectrum of atopic dermatitis. J Allergy Clin Immunol 1999; 104(3 Pt 2):S87-98; PMID:10482859; https://doi.org/10.1016/S0091-6749(99)70050-3
- Thomsen SF. Atopic dermatitis: natural history, diagnosis, and treatment. ISRN Allergy 2014; 2014:354250; PMID:25006501; https://doi.org/10.1155/2014/354250
- Leung DYM. New insights into atopic dermatitis: role of skin barrier and immune dysregulation. Allergol Int 2013; 62(2):151-61; PMID:23712284; https://doi.org/10.2332/allergolint.13-RAI-0564
- Elias PM, Wakefield JS. Mechanisms of abnormal lamellar body secretion and the dysfunctional skin barrier in patients with atopic dermatitis. J Allergy Clin Immunol 2014; 134:781-91.e1; PMID:25131691; https://doi.org/10.1016/j.jaci.2014.05.048
- Sugawara T, Iwamoto N, Akashi M, Kojima T, Hisatsune J, Sugai M, Furuse M. Tight junction dysfunction in the stratum granulosum leads to aberrant stratum corneum barrier function in claudin-1-deficient mice. J Dermatol Sci 2013; 70(1):12-8; PMID:23433550; https://doi.org/10.1016/j.jdermsci.2013.01.002
- Kirschner N, Rosenthal R, Furuse M, Moll I, Fromm M, Brandner JM. Contribution of tight junction proteins to ion, macromolecule, and water barrier in keratinocytes. J Invest Dermatol 2013; 133(5):1161-9; PMID:23407391; https://doi.org/10.1038/jid.2012.507
- Yuki T, Hachiya A, Kusaka A, Sriwiriyanont P, Visscher MO, Morita K, Muto M, Miyachi Y, Sugiyama Y, Inoue S. Characterization of tight junctions and their disruption by UVB in human epidermis and cultured keratinocytes. J Invest Dermatol 2011; 131(3):744-52; PMID:21160495; https://doi.org/10.1038/jid.2010.385
- Sokabe T, Fukumi-Tominaga T, Yonemura S, Mizuno A, Tominaga M. The TRPV4 channel contributes to intercellular junction formation in keratinocytes. J Biol Chem 2010; 285(24):18749-58; PMID:20413591; https://doi.org/10.1074/jbc.M110.103606
- Ding L, Lu Z, Lu Q, Chen Y. The claudin family of proteins in human malignancy: a clinical perspective. Cancer Manag Res 2013; 5:367-75; PMID:24232410