ABSTRACT
Tight junctions (TJs) are sites of cell-cell adhesion, constituted by a cytoplasmic plaque of molecules linked to integral proteins that form a network of strands around epithelial and endothelial cells at the uppermost portion of the lateral membrane. TJs maintain plasma membrane polarity and form channels and barriers that regulate the transit of ions and molecules through the paracellular pathway. This structure that regulates traffic between the external milieu and the organism is affected in numerous pathological conditions and constitutes an important target for therapeutic intervention. Here, we describe how a wide array of G protein-coupled receptors that are activated by diverse stimuli including light, ions, hormones, peptides, lipids, nucleotides and proteases, signal through heterotrimeric G proteins, arrestins and kinases to regulate TJs present in the blood-brain barrier, the blood-retinal barrier, renal tubular cells, keratinocytes, lung and colon, and the slit diaphragm of the glomerulus.
Introduction
In multicellular organisms the exchange of ions and molecules with the external milieu occurs through epithelial cell layers. Epithelial cells have two defining characteristics. The first is the polarization of their plasma membrane in two domains: apical and basolateral, with different biochemical composition, morphology and function [for review see.Citation1] The apical domain is in contact with the external environment or the content present in the lumen of ducts (e.g. intestine and renal tubules) and cavities (e.g. stomach, uterus and prostate), and displays microvilli and cilia, while the basolateral domain faces the underlying parenchyma and the interior of the organism, and exhibits in the basal and lateral regions, cell to substrate and cell to cell adhesion structures, respectively.
The second canonical characteristic of epithelia is the presence of tight junctions (TJs) at the uppermost portion of the lateral membrane. These cell-to-cell adhesion structures block the free diffusion of lipids and proteins within the membrane between the apical and the basolateral domains.Citation2–4 and hence are fundamental to maintain the polarized state of the plasma membrane in epithelial cells that guarantees the vectorial transport of ion and substances across epithelial cells. This notion however has been recently challenged by the observation that complete polarization of epithelial cells has been achieved in single cells that lack junctional cell-cell contacts upon activation of LKB1, a serine/threonine kinase whose gene is homologous to Par-4 polarity gene in C. elegans and D. melanogaster.Citation5
By thin section transmission electron microscopy TJs appear as points of contact between the outer leaflets of the plasma membrane of adjacent cells. At these points originally referred to as “kisses”, the intercellular space disappears blocking the free transit of molecules and ions through the paracellular route.Citation6 By freeze-fracture electron microscopy the TJ is seen as a network of strands in the protoplasmic face of the membrane and of grooves in the E face that surround the cells bellow the apical microvilli.Citation7 [for review see.Citation8] These strands are formed by the polymerization of claudins, a family of tetraspan proteins that interact between neighboring cells forming channels and seals in the paracellular space that define the ionic selectivity and paracellular permeability of epithelia [for reviews see.Citation9,Citation10] Besides claudins, other tetraspan proteins, members of the Marvel family named occludin and marvelD3 are found in TJ strands between two cells,Citation11 while tricellulin concentrates at junctions formed between three cells.Citation12 In addition, integral proteins that belong to the immunoglobulin superfamily like JAMs [for review see.Citation13] and angulins establish cell-cell interactions at TJs and mark the site where these structures are to be established in bicellular and tricellular [for review see.Citation14] junctions, respectively. In the submembranous region of TJs a scaffold of proteins is found that links integral TJ proteins to the actomyosin cytoskeleton. Among these proteins are cingulin and paracingulin, as well as a wide variety of proteins with PDZ domains, including the MAGUK proteins ZO-1, -2, and -3 and Pals1; the inverted MAGUKs also known as MAGI -1, -2 and -3, and proteins containing a single PDZ domain like Par-6 and AF-6 or multiple PDZ domains like MUPP1 and PATJ [for reviews see.Citation15,Citation16]
TJ integrity and barrier function is regulated by several mechanisms including: 1) expression of TJ proteins, 2) integrity and dynamics of TJ-associated actomyosin cytoskeleton, 3) localization of TJ proteins at the plasma membrane and 4) posttranslational modification of TJ proteins that affects protein-protein interactions. These mechanisms in turn, are modulated by various signaling pathways and molecules like kinases, phosphatases, Rho proteins and G protein-coupled receptors (GPCRs) [for review see.Citation17] GPCRs constitute the largest protein family in the human proteome, with more than 800 members that share as common characteristic the presence of seven transmembrane helices. GPCRs are targeted by ≈ 30-40% of drugs in the market highlighting their crucial participation in a wide spectrum of physiological processes and diseases.Citation18 GPCRs are usually located in the plasma membrane, although some concentrate at the membrane of the endoplasmic reticulum. They recognize a wide variety of stimuli including light, ions, hormones, glucocorticoids, peptides, lipids, proteases and neurotransmitters. GPCRs convert these stimuli into intracellular responses through their coupling to intracellular proteins like heterotrimeric guanine-nucleotide-binding proteins (G proteins), arrestins and kinases. Arrestins are adapter proteins that uncouple GPCRs from G proteins and target them to clathrin-mediated endocytosis. Since arrestins form complexes with several kinases, they can recruit them to agonist occupied GPCRs and confer a distinct signaling pathway for these receptors [for review see.Citation19]
GPCR signal transduction through G proteins starts with the coupling of the receptor to a membrane associated heterotrimeric complex constituted by a GTP hydrolysing Gα subunit and a Gβγ dimeric partner. Upon activation by an agonist, GPCRs undergo a conformational change that promotes their guanine-nucleotide-exchange activity. This leads to the release of GDP and subsequent binding of GTP by the Gα subunit, triggering the dissociation of Gα subunit from Gβγ and the subsequent association of different effectors to specific subtypes of Gα subunits (). Around 20 mammalian G protein α subunits have been identified and organized into four families based on their sequence similarity: Gαs, Gαi, Gαq/11, and Gα12/13. When the G protein complex is stimulatory, Gαs activates adenylyl cyclase that converts ATP into cAMP, which can directly activate protein kinase A (PKA). Instead when the complex is inhibitory, Gαi blocks adenylyl cyclase. Pertussis toxin from Bacillus pertussis catalyses the ADP-ribosylation of Gαi leaving it locked in a GDP bound state, leading to an increase in the cellular concentration of cAMP. Gαq/11 subunit activates phospholipase C (PLC), which in turn hydrolyzes phosphatidylinositol 4,5-biphosphate (PIP2) to diacyl glycerol (DAG) and inositol trisphosphate (IP3). The former is a second messenger that activates protein kinase C (PKC), while IP3 induces the release of calcium from the endoplasmic reticulum. Gα12/13 activates guanine nucleotide exchange factors (GEFs) for the small G protein RhoA [for review see.Citation20]. indicates the type of G protein α subunit involved in signaling by GPCRs regulating TJ barrier function.
Figure 1. Schematic representation of GPCR signal transduction through G proteins. Signal transduction starts with the association of the receptor to a G protein heterotrimeric complex formed by a Gα subunit and a Gβγ dimeric partner. GPCR activation leads to the release of GDP by the Gα subunit and subsequent binding of GTP, triggering the dissociation of Gβγ subunit from Gα. The latter then interacts with different effectors. Gαs activates adenylyl cyclase that converts ATP into cAMP, which can directly activate cAMP-dependent protein kinase (PKA) and through the conversion of cAMP to AMP activates AMP dependent kinase (AMPK). Instead, Gαi blocks adenylyl cyclase. Gαq/11 subunit activates activates phospholipase C (PLC) that hydrolyzes phosphatidylinositol 4,5-biphosphate (PIP2) to diacyl glycerol (DAG) and inositol trisphosphate (IP3). DAG activates protein kinase C (PKC) and IP3 induces the release of calcium from the endoplasmic reticulum. While Gα12/13 activates guanine nucleotide exchange factors (GEFs) for RhoA.
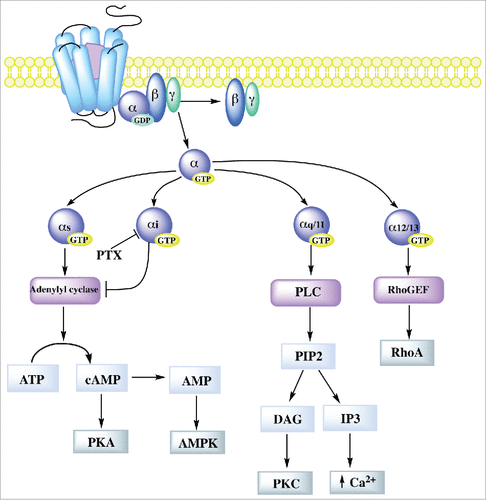
Table 1. G protein-coupled receptors regulating tight junction barriers.
Since 1991, Balda demonstrated that G proteins are implicated in the regulation of TJ assembly and sealing,Citation21 and afterwards, Gα subunits were found to localize at epithelial TJs. Thus, constitutive activation of Gα12/13, which binds to the SH3 domain of ZO-1, increased the paracellular permeability of epithelial cells.Citation22 TJs were disrupted since Gα12/13 stimulated Src-mediated phosphorylation of ZO-1 and ZO-2, which in turn caused dissociation of occludin and claudin-1 from the TJ complex. In addition, recruitment of heat shock protein 90 was necessary for Src activation by Gα12/13. Instead, endogenous Gα12/13 was activated upon TJ assembly triggered by switching the monolayers from low to normal calcium.Citation23 Gαi2 subunit also localizes at TJs and immunoprecipitates with ZO-1, and its activation accelerates TJ biogenesis in epithelia.Citation24,Citation25
According to sequence similarities within their seven-transmembrane region, GPCRs are classified into six major families: class A also known as rhodopsin receptor family, that includes receptors for odorants and small ligands; class B or secretin receptor family with ligands that include high molecular weight hormones; class C or metabotropic glutamate receptor family that includes GABA, taste, olfactory and pheromone receptors; class D or fungus pheromone receptor family; class E or cAMP receptors found only in Dictyostelium discoideum; and class F or frizzled/smoothened receptor family involved in cell polarity and segmentation [for review see.Citation26]
In this review we focus on GPCRs that influence TJ assembly and sealing evaluated by changes in transepithelial electrical resistance (TER) and paracellular permeability, as well as alterations in the expression of TJ proteins. In the case of claudins, we also report modifications in the isoforms of claudins being expressed. However, in many cases these changes in isoforms have not been correlated to variations in permeability and moreover, in the case of cancers, differences in claudin expression could be the result of a dedifferentiation of cells to a more stem-cell-like state.
shows that of the 52 GPCR receptors that regulate the TJ barrier, 43 belong to class A and 5 to class B, while only 2 GPCRs belong to classes C and F, respectively. The two GPCRs of class C promote TJ formation and sealing, whereas the rest of the classes have some GPCRs that induce TJ sealing and others that open the barrier. These effects also vary according to the receptor ligand and tissue.
Here, for clarity purposes, we have organized the information based on the effect of GPCRs on TJs, and have subdivided the data according to the type of agonists that activate the receptors. Schematic summaries of the effect of different GPCRs in TJs of the intestine, kidney, blood-brain barrier (BBB), blood-retinal barrier (BRB) and other endothelia are presented in figs –.
Figure 2. Regulation of TJs in intestinal epithelia by different G protein coupled receptors. Left, schematic representation of colon epithelia, showing a list of GPCRs the stimulate TJ formation (blue arrow) or favor TJ disassembly (red arrow). Right, signaling pathways known to be activated in the colon by GPCRs to promote TJ opening or closure. References for these studies are shown in . Receptors: A2B, adenosine receptor B; BLT2/LTB4R2, leukotriene B4 receptor type 2; BR2/BKR2/BDKRB2, bradykinin receptor B2; Calcrl, calcitonin receptor-like receptor; CaSR, calcium sensing receptor; CBR, cannabinoid receptor; CRHR, corticotropin releasing hormone receptor; CXCR, C-X-C motif chemokine receptor; EP, E-type prostanoid receptor; GPR, G protein-coupled receptor; OGR1, ovarian cancer G protein-coupled receptor 1; PAR-2, protease-activating receptor 2; SSTR, somatostatin receptor; S1PR, sphingosine-1 phosphate receptor. Other abbreviations: AMPK, AMP-activated protein kinase; cAMP, cyclic adenosine monophosphate; ERK, extracellular signal-regulated protein kinase; IP3, inositol triphosphate; MEK, MAPK/ERK kinase; MLC, Myosin light-chain; MLCK, myosin light-chain kinase; MMP2, matrix metalloproteinase 2; mTOR, target of rapamycin; NFκB, nuclear factor kappa B; PKA, protein kinase A; PKC, protein kinase C; PLC, Phospolipase C; SRF, serum response factor; STAT, Signal transducer and activator of transcription; TNFα, tumor necrosis factor α; ZO-2, zonula occludens 2.
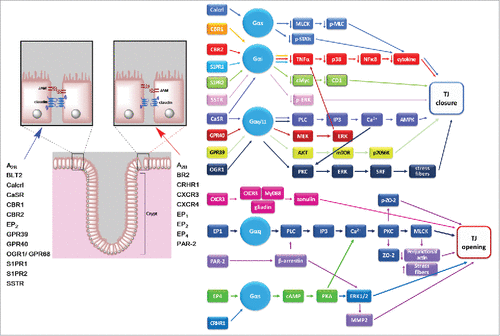
Figure 3. GPCR regulation of TJs in the thick ascending limb of Henle, and of the slit diaphragm in the glomerulus. A) Left, schematic representation of a nephron and the slit diaphragm between podocytes in the glomerulus. The GPCRs that open (red arrow) or tighten (blue arrow) the podocytes slit diaphragms are indicated. Right, signaling pathways activated by GPCRs that regulate the slit diaphragm B) Schematic representation of epithelial cells lining the thick ascending limb of Henle illustrating how activation of CaSR favors claudin-14 expression, blocking in consequence cation reabsorption through the claudin-16/claudin-19 paracellular heteromeric channel. CaSR promotes claudin-14 expression blocking the transcription of miR-9 and miR-374 genes that induce the decay of claudin-14 mRNA. Receptors: AT1, angiotensin II receptor 1; BR2/BKR2/BDKRB2, bradykinin receptor B2; CaSR, calcium sensing receptor; CBR, cannabinoid receptor. Other abbreviations: ADAM, disintegrin and metalloenzyme; EGFR; epidermal growth factor receptor; ERK, extracellular signal-regulated protein kinase; miR, microRNA; PIP2, Phosphatidylinositol 4,5-bisphosphate; PLC, Phospolipase C; Src, protein-tyrosine kinase.
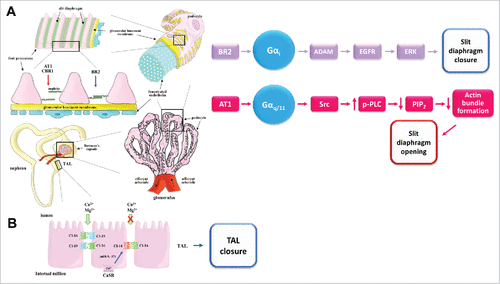
Figure 4. BBB regulation by different G protein coupled receptors. Left, schematic representation of the BBB, showing a list of GPCRs the stimulate TJ formation (blue arrow) or favor TJ disassembly (red arrow). Right, signaling pathways activated in the BBB by GPCRs to promote BBB opening or closure. References for these studies are shown in . Receptors: AT1, angiotensin II receptor 1; A1/ADOR, adenosine receptor; BLT2/LTB4R2, leukotriene B4 receptor type 2; BR2/BKR2/BDKRB2, bradykinin receptor B2; Calcrl, calcitonin receptor-like receptor; CBR, cannabinoid receptor; CCR, C-C motif chemokine receptor; CXCR, C-X-C motif chemokine receptor; C5aR, complement C5a receptor; EP, E-type prostanoid receptor; ETB, endothelin receptor; FZD, frizzled class receptor; GLPR1, glucagon like peptide 1 receptor; GPR, G protein-coupled receptor; PAR, protease-activating receptor; SSTR, somatostatin receptor; S1PR, sphingosine-1 phosphate receptor. Other abbreviations: cAMP, cyclic adenosine monophosphate; CREB, cAMP response element-binding; eNOS, endothelial nitric oxide synthase; ERK, extracellular signal-regulated protein kinase; iNOS, inducible nitric oxide synthase; LRP, low density lipoprotein receptor-related protein; MLC, Myosin light-chain; MMP, Matrix metalloproteinase; NFκB, nuclear factor kappa B; p, phosphorylated; PKA, protein kinase A; PLC, Phospolipase C; ROCK; Rho-associated protein kinase; Sox-17, SRY-Box 17 transcription factor; ZONAB, ZO-1-associated nucleic acid-binding protein.
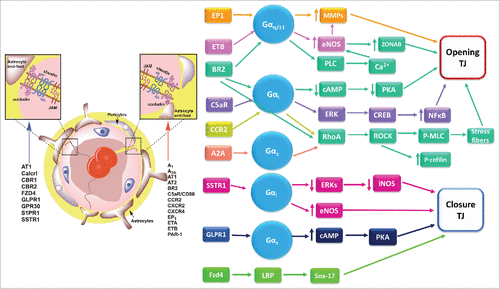
Figure 5. Schematic representation of the retina showing GPCRs that regulate the retinal barriers and the known mechanisms involved. Left, schematic representation of the retina showing a list of GPCRs the stimulate TJ formation (blue arrow) or favor TJ disassembly (red arrow) in the iBRB, oBRB and the TJ-like barrier between the photoreceptors and Müller glial cells. Right, signaling pathways activated by GPCRs to promote iBRB closure. Receptors: Calcrl, calcitonin receptor-like receptor; GLPR1, glucagon like peptide 1 receptor; MC, melanocortin receptor; S1PR, sphingosine-1 phosphate receptor. Other abbreviations: AKT, protein kinase B; ERK, extracellular signal-regulated protein kinase; iNOS, inducible nitric oxide synthase; NFκB, nuclear factor kappa B; p, phosphorylated; STAT3, Signal transducer and activator of transcription 3; TNFα, tumor necrosis factor α.
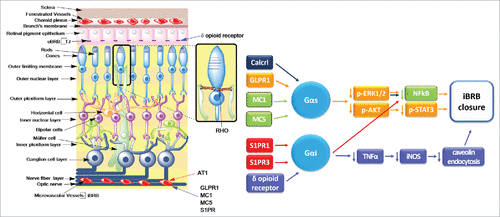
Figure 6. Regulation of endothelial TJs present in tissues different to the retina or the brain by different G protein coupled receptors. Left, schematic representation of TJs between endothelial cells with a list of GPCRs the stimulate TJ formation (blue arrow) or favor TJ disassembly (red arrow). Right, signaling pathways activated in endothelial cells by GPCRs to promote TJ opening or closure. References for these studies are shown in . Receptors: Calcrl, calcitonin receptor-like receptor; CCR, C-C motif chemokine receptor; H1, histamine receptor 1; PAR, protease-activating receptor; S1PR, sphingosine-1 phosphate receptor.Other abbreviations: AKT, protein kinase B; Ang, angiopoietin; cAMP, cyclic adenosine monophosphate; ERK, extracellular signal-regulated protein kinase; IP3, inositol triphosphate; MLC, Myosin light-chain; NFκB, nuclear factor kappa B; p, phosphorylated; PKA, protein kinase A; PLC, Phospolipase C; Rac1, Ras-related C3 botulinum toxin substrate 1; Rap1, Ras-related protein 1; RhoA, Ras homolog gene family, member A; ROCK, Rho-associated Coiled-coil Kinase; STAT3, Signal transducer and activator of transcription 3; Tie2, endothelial receptor tyrosine kinase 2.
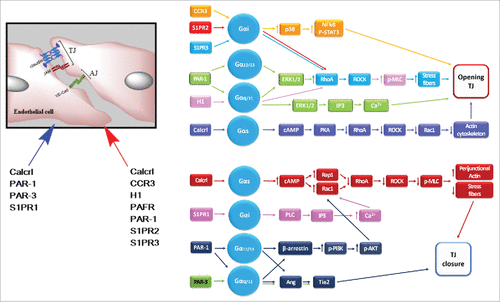
G protein-coupled receptors that induce TJ formation
Ion sensing receptors
Proton-sensing receptor OGR1/GPR68
The ovarian cancer G protein-coupled receptor OGR1/GPR68 also known as a receptor for sphingosylphosphorylcholine is a proton-sensor receptor of mild to moderate extracellular acidification below the physiological set point of pH 7.4. The receptor signals via Gαq/11 and activates PKC, ERK and gene promoters that depend on the transcription factor serum response factor (SRF), which is a master regulator of actin cytoskeleton.Citation27 OGR1/GPR68 is expressed in osteoblasts,Citation28 kidney and the intestinal epithelium where it is up-regulated during inflammation. In intestinal Caco-2 cells stably over-expressing OGR1/GPR68, an acidic pH shift from pH 7.8 to 6.6 leads to an acute TJ barrier enhancement explained by augmented expression of occludin and ZO-1 and a decrease of claudin-2. This change is accompanied by increased formation of actin stress fibers and reduced cell mobility.Citation29 Hence, OGR1/GPR68 could become a target for innovative therapies dealing with the pathogenesis and progression of inflammatory bowel disease, which is commonly associated to a local pH decrease, TJ barrier disfunction and claudin-2 over-expression. However, chronic activation of OGR1/GPR68 may also exacerbate tissue damage since it also induces genes associated with tissue inflammation like those for interleukin 8 (IL-8),Citation30 chemokines CXCL1 and CXCL2, and prostaglandin-endoperoxide synthase,Citation29 explaining why OGR1/GPR68 deficiency protects from inflammation in the IL-10 knock-out (KO) mice model.Citation30
Zinc-sensing receptor GPR39
Zinc is an essential micronutrient important for human health. Zinc deficiency alters the epidermal, digestive, immune, reproductive and neuronal systems [for review see.Citation31] Zinc is a cofactor of several enzymes and binds to zinc fingers domains in transcription factors and other proteins including transporters, ion channels and membrane receptors. G protein-coupled receptor GPR39 that senses extracellular zinc and signals through Gαq/11, is present in neurons in the hippocampus,Citation32 the thyroidCitation33 and the gastrointestinal system, including the stomach, small intestine, colon and pancreas.Citation34,Citation33
GPR39 belongs to a family of receptors that includes those for gherlin, motilin and neurotensin.Citation35 Gherlin is the ligand of growth hormone secretagogue receptor and is known to stimulate appetite. Both gherlin and motilin, stimulate gastric emptying and interdigestive motility. Obestatin, a peptide derived from gherlin precursor peptide is the natural ligand of GPR39 and opposes gherlin's effect on food intake.Citation36 GPR39 KO mice shows symptoms of zinc deficiency like accelerated gastric emptying and increased fecal secretion,Citation33 accompanied by a decreased expression of ZO-1 and occludin in the colon.Citation37
Activation in colon of zinc/GPR39 signaling regulates proliferation and differentiation of the epithelia and induces TJ formation.Citation37 Hence, GPR39 silencing attenuated the activation of ERK1/2, AKT and mTOR/p70S6K pathways that promote proliferation, but at the same time inhibited alkaline phosphatase activity, a marker of colon cell differentiation. These changes were accompanied by a decrease in TER and a reduced expression of the apical junctional complex proteins occludin, ZO-1 and E-cadherin. Therefore, it is not surprising to observe that in the dextran sulfate sodium (DSS) model of ulcerative colitis, the loss of GPR39 increased inflammation susceptibility due to a lower expression of occludin.Citation38 and that zinc supplementation through GPR39 activation enhanced the amount of ZO-1 and occludin and improved epithelial integrity in Salmonella typhimurium infected colonic cells.Citation39
Zinc activation of GPR39 also leads to epithelial repair. Thus, in keratinocytes zinc/GPR39 signaling up-regulates the activity of the sodium proton exchanger NHE1 and enhances scratches closure.Citation40 Interestingly, extracellular zinc can derive from the injured cells in the tissue, revealing a mechanism through which the damaged cells induce the repair of the wound.
Calcium-sensing receptor CaSR
In the kidney, claudins -14, -16 and -19 regulate paracellular reabsorption of calcium. In the thick ascending limb of Henle (TAL), where a major percentage of Ca2+ and Mg2+ is reabsorbed via the paracellular route [for review see,Citation41] claudins -16 and -19 form a paracellular heteromeric cationic channel that permeates Ca2+ and Mg2+.Citation42,Citation43 Claudin-14 interacts with claudin-16, diminishing the permeability of the paracellular claudin-16/claudin-19 cation channel.Citation44
Calcium sensing receptor (CaSR) which is critical for the homeostasis of divalent ions and is upregulated by extracellular Ca2+, decreases the phosphorylation in serine residues of claudin-16 and triggers its dissociation from ZO-1 and translocation to the lysosome.Citation45 CaSR also favors claudin-14 expression, blocking in consequence Ca2+ reabsorption through the claudin-16/claudin-19 channel.Citation44 Accordingly, a deficiency of CaSR, down-regulates in kidney the expression of claudin-14, up-regulates claudin-16 and lowers Ca2+ urinary excretionCitation46 ().
CaSR promotes claudin-14 expression blocking binding of the nuclear factor of activated T cells to the proximal promoter region of miR-9 and miR-374 genes.Citation47 These micro RNAs target claudin-14 mRNA and induce its decay and translational repression.Citation44,Citation47 Inhibitors of histone deacetylase stimulate the transcription of miR-9 and miR-374 and in consequence increased paracellular cation conductance in the TAL and rescued the phenotype of cells and animal models of autosomal dominant hypocalcemia, characterized by a gain of function mutation in CaSR.Citation48 Altogether, these observations highlight the importance of CaSR as a novel therapeutic target to treat renal calcium handling pathologies.
CaSR promotes TJ assembly and sealing in diverse tissues. Hence, the over-expression of CaSR in the basal cells of mice epidermis accelerates the differentiation of embryonic epidermal cells and the formation of the epidermal permeability barrier by claudins.Citation49 In MDCK cells, transfection of a CaSR gain of function mutant increased TER, and the activation of CaSR, relocated ZO-1 and occludin to the cell borders in cells cultured in low Ca2+ media, in a process that promoted the interaction of ZO-1 with I-afadin mediated by AMP-activated kinase (AMPK).Citation50 This effect seems surprising since CaSR signals through Gαi that inhibits adenylyl cyclase and lowers AMPK activation. However, CaSR also transmits information through Gαq/11 that via PLC and IP3 releases calcium from the endoplasmic reticulum and activates AMPK. This signaling cascade was demonstrated in intestinal cells where chitosan, an oligosaccharide derived from chitin found in the cytoskeleton of shrimp, crab and insects, activated CaSR and induced TJ assembly.Citation51
Receptors activated by hormones
Adrenomedullin and intermedin receptor Calcrl
Adrenomedullin and intermedin also known as adrenomedullin2, are peptide hormones of the same family that bind to the G protein-coupled calcitonin receptor-like receptor (Calcrl) when the latter is associated with a receptor activity modifying protein (RAMP). The latter constitutes a family of three members: RAMP-1, -2 and -3, which translocate the Calcrl to the plasma membrane. Adrenomedullin binds to Calcrl/RAMP-2 and Calcrl/RAMP-3 and with less affinity than calcitonin gene-related peptide, to Calcrl/RAMP-1. Intermedin also binds Calcrl/RAMP-1 to -3 but with lower affinity than adrenomedullin [for review see.Citation52]
Adrenomedullin and intermedin are secreted from a variety of organs and tissues including endothelial cells, and plasma levels of adrenomedullin are elevated in patients with hypertension, congestive heart failure and chronic kidney disease.
Endothelial cell specific KO mice of RAMP-2 die perinatally and the surviving adults are afflicted with spontaneous vasculitis. Moreover, in drug inducible adult KO mice, the deletion of endothelial RAMP-2 provoked pronounced edema and vascular leakage. These deleterious effects on endothelial cells barrier function were triggered by a decrease in Rac1-GTP accompanied by an increase in RhoA-GTP that produced fragmentation of the cortical actin ring.Citation53 Adrenomedullin counteracts actomyosin contractility by activating Rap1, a small GTPase similar in structure to Ras, which inhibits RhoA. Accordingly, loss of the actin binding protein cortactin, causes endothelial barrier dysfunction due to actomyosin contractility mediated by a reduced adrenomedullin secretion.Citation54
In human umbilical vein endothelial cells (HUVEC), adrenomedullin and intermedin decreased the paracellular hyperpermeability induced by thrombin, through a mechanism involving cAMP accumulation. Adrenomedullin and intermedin reduce stress fibers formation.Citation55,Citation56 and in the case of adrenomedullin this was found to be accompanied by a decreased phosphorylation of myosin light chain, while the intermedin study reported an increase in Rac1 activation together with a reduced RhoA activity and a consequential diminished actomyosin contraction. Intermedin however, was less potent than adrenomedullin. It is however noteworthy, that in rat coronary microvascular endothelial cells, intermedin increased permeability.Citation57 This effect, opposite to that observed in HUVEC is due to the fact that although intermedin inactivated the RhoA/ROCK pathway in both cell types, it inactivated Rac1 in coronary microvascular endothelial cells but not in HUVEC, highlighting the importance of the Rac1-GTP/RhoA-GTP ratio to preserve the endothelial barrier stability.
Intermedin increased TER of human pulmonary microvascular endothelial cells and attenuated ventilator-induced lung hyperpermeability in mice. These results highlight the potential therapeutical use of intermedin to prevent or ameliorate ventilator induced lung injury in patients receiving mechanical ventilation due to respiratory failure, surgery or trauma.Citation58
In lymphatic cells, adrenomedullin tightened the endothelial barrier by concentrating ZO-1 and VE-cadherin at the plasma membrane.Citation59 while the temporal deletion of Calcrl in adult mice induced a disorganized expression of ZO-1 and VE-cadherin at lymphatic junctions triggering a pathologic dilation of lymph vessels known as lymphangiectasia.Citation60
In the BBB, adrenomedullin increased TER and decreased paracellular permeability, and these changes were accompanied by enhanced claudin-5 expression,Citation61 although others reported that the improval of BBB function did not affect the expression of claudin-1, occludin and ZO-1.Citation62,Citation63
In Kimba mice that over-express vascular endothelial growth factor (VEGF) in the retina and display characteristics of diabetic retinopathy, the administration of adrenomedullin ameliorated the capillary dropout and vascular leakage, and in retinal capillary endothelial cells in culture treated with VEGF, adrenomedullin suppressed the enhanced permeability and reduced TER by reducing the level of molecules related to NFκB signaling and inflammation like MCP-1, IL-1β, VCAM-1, ICAM-1 and TNF-α, and by inducing TJ formation.Citation64 A somewhat similar effect was observed in HUVEC cells, where intermedin reduced the irregular and over sprouted vasculature caused by VEGF by preventing junctional VE-cadherin dissociation.Citation65
Adrenomedullin has a potential therapeutic value for the treatment of inflammatory bowel disease, as it can maintain the intestinal epithelial barrier function in rodent models of colitis induced by 2,4,6-trinitro-benzene-sulfonic acid or DSS. The protective effect that allowed the maintenance of TJ proteins was exerted via down-regulation of myosin light chain kinase (MLCK) and phosphorylated myosin light chain, and suppressed phosphorylation of STAT1 and STAT3 that triggered the decreased expression of inflammatory cytokines TNF-α, IL-6 and IFN-ϒ.Citation66,Citation67 Adrenomedullin also reduced intestinal permeability in rats with Staphylococcus aureus α-toxin induced septic shock and in Caco-2 cells treated with the α-toxin or H2O2 .Citation68
Somatostatin receptor SSTR
Somatostatin, also known as growth hormone is a peptide hormone that inhibits insulin and glucagon secretion. Somatostatin interacts with five receptors named SSTR 1 to 5 that are coupled to inhibitory, pertussis toxin sensitive G proteins.
Somatostatin maintains the integrity of the BBB and restores the organization of ZO-1 in human brain endothelial cells treated with cytokines and lipopolysaccharide (LPS) to disrupt TJs. Hence, the decreased level of somatostatin found in the cerebrospinal fluid of patients with multiple sclerosis appears to contribute to the leaky BBB present in this disease.Citation69
In intestinal Caco-2 cells, somatostatin ameliorates LPS induced TJ damage, by decreasing ERK1/2 phosphorylation and increasing the expression of occludin and ZO-1 and inhibiting their redistribution.Citation70 Interestingly, SSTR3 interacts with MUPP1 TJ protein, and as a result of this SSTR3 is targeted to TJs. The interaction with MUPP1 gives the receptor the capacity to increase TER in a pertussis sensitive manner.Citation71 and in cultured human keratinocytes, treatment with somatostatin increased the expression of claudin-4.Citation72 that functions as a cationic barrier,Citation73 hence explaining the increase in TER observed.
Glucagon-like peptide receptor GLPR1
Glucagon-like peptide (GLP-1) is a 30-amino acid long peptide hormone secreted by intestinal enteroendocrine L-cells and certain neurons upon food consumption that signals through GLP-1 receptor (GLPR1). GLP-1 decreases blood sugar level by enhancing the secretion of insulin, and hence has the potential of being used for the treatment of type-2 diabetes.
Treatment of brain capillary endothelial cells with GLP-1 increased TER and the amount of occludin and claudin-5, and decreased paracellular permeability via cAMP/PKA signaling.Citation74 An agonist of GLP-1 named exendin-4, protects the inner endothelial blood-retinal barrier (iBRB) from vascular leakage induced by diabetic insult, by up-regulating the expression of GLPR1, claudin-5 and occludin.Citation75
Melanocortin receptors MC1 and MC5
Melanocortins are peptide hormones with a wide range of biological activities. Melanocortin receptors MC1, MC3, MC4 and MC5 are ubiquitously expressed, while MC2 is localized at adrenal glands. Intravitreal injection of MC1 and MC5 agonist in streptozotocin-induced diabetic mice augmented the expression of occludin in the retina, attenuating diabetic retinal damage. The mechanism involved is not yet clear, but appears to be related to a decrease to nondiabetic values, of the main mediators of the inflammatory response including cytokines IL-1α, IL-1β and IL-6, and chemokines MIP-1α, MIP-2α and MIP-3α, and of the main markers of vessel proliferation such as VEGF and ki-67.Citation76 The ability of melanocortins to reduce production of proinflammatory cytokines relies on their capacity to inhibit NFκB translocation to the nucleus.Citation77
G protein-coupled receptors activated by lipids
Receptors activated by metabolites of arachidonic acid
BLT2 a leukotriene B4 receptor type 2 activated by 12-HHT
12-Hydroxyheptadecatrenoic acid (12-HHT) is a 17-carbon metabolite of arachidonic acid that for many years was thought be an inactive byproduct of prostaglandin synthesis. However, recent research demonstrates that it protects epithelial barriers through the activation of G protein-coupled leukotriene B4 (LTB4) receptor type 2 (BLT2), for which it has even a higher affinity than LTB4. In mice lacking BLT2 an increased susceptibility to DSS-induced colitis was found, while transfection of BLT2 into MDCK cells decreased paracellular permeability.Citation78 and induced a faster recovery of TER after a calcium switch.Citation79 12-HHT treatment of human keratinocytes activated BLT2 and enhanced TJ sealing by inducing claudin-4 expression through a process mediated by Gαi and MAPK activation.Citation79 12-HHT increased TER even in cell grown under low calcium condition that mimic the basal layer of cells in the epidermis.Citation80 This suggests that BLT2 agonist could be employed for the treatment of barrier-disrupted skin diseases.
ALX/FPR2 receptor activated by lipoxin A4
Lipoxins are arachidonic acid metabolites with potent anti-inflammatory and immunoregulatory properties. Lipoxin A4 that binds to ALX/FPR2 G protein-coupled receptor plays an anti-inflammatory role in human airways and its production is decreased in cystic fibrosis and severe asthma. Lipoxin A4 increased TER and stimulated ZO-1, claudin-1 and occludin expression in human bronchial epithelia,Citation81 and delayed Pseudomonas aeruginosa invasion and transepithelial migration in cystic fibrosis and normal bronchial epithelial cell cultures by reducing TJ disruption.Citation82
Cannabinoid receptors
Cannabinoids include endocannabinoids that are produced naturally in the body of animals; phytocannabinoids found in plants like marijuana [e.g the psychoactive compound Δ9-tetrahydrocannabinol (THC) and cannabidiol (CBD)], and synthetic cannabinoids. Endocannabinoids are lipids derived from arachidonic acid that undergo rapid degradation by fatty acid amide hydrolase. There are two cannabinoid receptors: CBR1, which is associated with the psychoactive effect of cannabinoids, and CBR2 that mediates anti-inflammatory actions.
Activation of cannabinoid receptors produces potent neuroprotective and anti-inflammatory effects. Thus, CBR1 synthetic agonist CP55940 inhibited HIV-1 glycoprotein 120-induced increase of permeability of human brain microvascular endothelial cells in co-culture with astrocytes, and prevented the down-regulation of ZO-1, claudin-5 and JAM-1, and in vivo blocked BBB permeability and the transmigration of human monocytes into the brain.Citation83 Agonists of CBR2 protect the blood-spinal cord barrier from ischemia reperfusion injury,Citation84 and the BBB dysfunction after LPS-induced encephalitis.Citation85 or subarachnoid hemorrhage.Citation86 by increasing TJ protein expression and reducing barrier leakiness. The mechanism of action has been described on the blood-spinal cord barrier during ischemia reperfusion injury, where CBR2 agonist JWH-015 down-regulates the expression of caveolin-1 and up-regulates in consequence TJ protein expression, and in an in vitro BBB model where this agonist increased TER of brain microvascular endothelial cells by inducing the phosphorylation of phosphoinositide-3 kinase (PI3K) and of transcription factor FoxO1 that binds to the promoter region of caveolin-1 gene and in turn decreased the expression of caveolin-1 protein.Citation84
In intestinal and pulmonary epithelia cannabinoids also exert an anti-inflammatory effect coupled with reinforcement of the TJ barrier. So, in mice with DSS-induced colitis, WIN55-212-2, an agonist of CBR1 and CBR2, through the inhibition of p38MAPK, decreased the plasma levels of TNF-α and IL-6, and enhanced the expression of claudin-1.Citation87 Interestingly, apical or basolateral treatment of intestinal Caco-2 cells with THC or CBD enhanced through CBR1 the speed of recovery of EDTA-induced permeability, while endocannabinoids exerted this effect only when applied basolaterally. All cannabinoids augmented the mRNA of ZO-1, but endocanabinoids also decreased the mRNA of claudin-1.Citation88 In rats with cirrhosis and ascites, activation of CBR2 decreased intestinal oxidative stress, inflammatory cytokines, intestinal mucosal damage, bacterial translocation and spontaneous bacterial peritonitis. These changes respond to a down-regulation by CBR2 agonist JWH133 of systemic TNF-α/NFκB/cytokine signaling cascade that increases epithelial permeability by decreasing TJ proteins.Citation89 Similarly, in airway epithelia, THC through CBR2 activation reversed TNF-α induced decrease in TER and increase in permeability due to a decreased expression of occludin and ZO-1,Citation90 and in pulmonary edema induced after subarachnoid hemorrhage, JWH133 an agonist of CBR2 inhibit the infiltration of neutrophils reducing pulmonary edema Citation91
In kidney in contrast, antagonizing cannabinoids signaling reinforces the slit diaphragm barrier. Thus, AM251, the antagonist of CBR1 prevented diabetes-induced down-regulation of nephrin, podocin and ZO-1 in podocytes, ameliorating albuminuria.Citation92
Receptor GPR40 activated by a gut microbial metabolite of polyunsaturated fatty acids
A gut microbial metabolite of linoleic acid named 10-hydroxy-cis-12-octadecenoic acid (HYA) ameliorated in mice the TNF-α and DSS-induced colitis, suppressing the reduction in intestinal occludin and the increased expression of MLCK. HYA blocked the expression of TNF receptor 2 in intestinal epithelial cells through the activation of GPR40 G-coupled free fatty acid receptor 1 and MEK/ERK signaling pathway.Citation93
Sphingosine-1 phosphate receptors S1PR
Sphingosine-1 phosphate (S1P) is a bioactive lipid generated from sphingomyelin by sphingosine kinases (SphK). S1P is the natural ligand of five different GPCRs named S1PR1-5. S1PR1 is also transactivated as a result of the interaction of the protease activated protein C (APC) with its receptor endothelial protein C receptor (EPCR), resulting in activation of protease activated receptor 1 (PAR-1) and Rac and endothelial barrier enhancement.Citation94,Citation95 (see also section 3.1.2). S1P analogues have potent barrier protective effects in lung endothelia.Citation96 and the BRB in diabetic rats.Citation97 and increased expression of SphK1 induced by the anesthetic isoflurane, suppresses BBB disruption after subarachnoid hemorrhage.Citation98 In addition, SEW2871, a S1PR1 agonist, improves intestinal barrier function, protecting IL-10 deficient mice from experimental colitis.Citation99
In endothelia, a physiological concentration of S1P enhances endothelial integrity through activation of S1PR1-PLC-IP3R-Ca2+-Rac pathway, while excessive S1P causes barrier dysfunction by activating S1PR2-calcium influx-RhoA/ROCK Citation100 Instead, S1P-mediated activation of S1PR2 up-regulates the expression of E-cadherin and ZO-1 in intestinal epithelial cells.Citation101
Delta opioid receptors activated by flavonoids
Flavonoids are plant or fungus secondary metabolites. Epicatechin, the predominant flavonoid present in dark chocolate, through the activation of δ opioid receptor diminishes the detrimental effect of high glucose, on TJs of the retinal pigment epithelia. Exposure to high glucose that mimics the milieu found under a diabetic condition increases the levels of TNF-α and inducible nitric oxide synthase (iNOS), creating nitrosative stress that results in S-nitrosylation and increased endocytosis of caveolin-1. This condition increases the interaction of caveolin-1 with claudin-1 and occludin and decreases their expression. This cascade is inhibited by treatment with high–polyphenol cocoa or epicatechin that through activation of δ opioid receptor, decrease TNF-α-induced iNOS upregulation and caveolin-1 endocytosis and restore TER in ARPE-19 monolayers.Citation102
Glucocorticoid-stimulated receptor GPR158
Trabecular meshwork cells are located in the eye at the base of the cornea and form a spongy tissue that allows draining of the aqueous humor from the anterior chamber of the eye into the tubes of the Schlemm´s canal that flows into the blood system. GPR158 is a glucocorticoid stimulated G protein-coupled receptor, whose over-expression enhances the barrier function of cultured trabecular meshwork cells, by increasing the expression of ZO-1 and occludin. This mechanism is responsible for glucocorticoid induced ocular hypertension that leads to glaucoma.Citation103
CCR6 receptor activated by β-defensins
β-defensins are antimicrobial peptides produced by epithelial cells lining various organs. Human β-defensins increased TER and reduced paracellular permeability of keratinocytes increasing the expression and localization of claudins. These effects were reversed with the blockade of G protein-coupled chemokine receptor CCR6.Citation104
Activation of frizzled-4 receptor by norrin
Norrin is a protein unrelated to Wnt ligands that signals through activation of frizzled-4 G protein-coupled receptor and LRP co-receptor (see also chapter 2.7). The NDP gene, whose mutations in humans cause Norrie disease, an X-linked disorder characterized by retinal hypovascularization and a severe loss of visual function, encodes Norrin. This protein promotes angiogenesis in the retina and is essential for BBB formation. Thus, after subarachnoid hemorrhage, norrin through frizzled-4 activation, protected BBB integrity enhancing the expression of occludin, VE-cadherin and ZO-1.Citation105
Precocious norrin production in engineered mice leads to premature retinal vascular invasion and delayed norrin production leads to defects in intra-retinal vascular architecture. In the adult retina and cerebellum, gain or loss of norrin/Fzd4 signaling resulted in gain or loss, respectively, of both the BRB and the BBB function,Citation106,Citation107 while the administration of a monoclonal antibody that neutralized frizzled-4 function in vivo inhibited physiological and pathologic sprouting angiogenesis within the retina and allowed the extravasation of molecules through the BRB.Citation108 Transcription factor Sox17, up-regulated by norrin/frizzled-4 signaling, appears to plays a central role in the angiogenesis induced by norrin Citation107).
Rhodopsin, a G protein-coupled receptor activated by light
Rhodopsin is a light-sensitive G protein-coupled receptor found in the eye in the rods of the retina that enables vision in low-light conditions. Retinitis pigmentosa is an inherited disease characterized by primary degeneration of rod photoreceptors and secondary degeneration of cones in the retina. In a mouse model of retinitis pigmentosa, induced by the targeted disruption of rhodopsin gene, the expression of ZO-1, β-catenin and p120-catenin diminishes at the outer limiting membrane of the retina.Citation109 The latter forms a TJ-like barrier between the photoreceptor cells and Müller glial cells, containing occludin, JAM and ZO-1, at the apical site of the outer nuclear layer of the photoreceptors.Citation110
G protein-coupled receptors that open TJs
Hormone activated receptors
Calcitonin activated receptor
Calcitonin (CT) is a 32-amino acid linear polypeptide hormone that in humans is produced by the parafollicular cells of the thyroid. It reduces blood calcium, in opposition to the effect of parathyroid hormone. Calcitonin is formed by the proteolytic cleavage of the product of CALC1 gene. Calcitonin receptor (CTR) signals through Gαs, adenylyl cylase and cAMP in target cells.
Expression of CT and CTR is frequently elevated in prostate cancers and activation of CT-CTR axis in non-invasive prostate cancer cells induces an invasive phenotype, while their silencing in highly metastasic prostate cancer cells reduces their tumorigenicity and ability to form distant metastases.Citation111 The activation of CTR induces the phosphorylation and inactivation of glycogen synthase kinase-3β (GSK-3β), a key enzyme needed for the cytosolic degradation of β-catenin in the Wnt signaling pathway. This results in β-catenin translocation into the nucleus and transcription of Wnt target genes involved in epithelial to mesenchymal transformation.Citation112 These changes are accompanied by increased secretion of matrix metalloproteinase (MMP) a switch from E-cadherin to N-cadherin, ZO-1 delocalization and loss of cell-cell adhesion.Citation112 The PDZ binding motif present at the carboxy-terminus of CTR binds to the PDZ3 domain of ZO-1,Citation113 bringing CTR-activated PKA in close proximity to TJs facilitating the phosphorylation of ZO-1 and claudin-3 that is responsible for TJ disassembly Citation114 These results hence explain why CTR-ZO-1 interaction is required for CTR to destabilize TJs and form metastases of prostate cancer.Citation113
Neurotransmitter activated receptors
Serotonin activated receptor 5-HT7
Serotonin is a monoamine neurotransmitter derived from tryptophan. In esophageal squamous epithelial cells, serotonin disrupts the barrier function through serotonin receptor 5-HT7 that activated p38MAPK and decreased the expression of ZO-1, and by activation of ERK that decreased occludin level. Serotonin also reduced claudin-1 expression but through a mechanism independent of ERK or MAPK activation.Citation115 In mammary epithelium, treatment with 5-HT7 receptor antagonists.Citation116 or a low concentration of serotonin increased TER, while at higher serotonin concentration and later time points, TER decreased and the TJs were disrupted through 5-HT7 activation of Gαs/cAMP and p38, while inhibition of PKA prevented the increase in TER in response to the low serotonin dose.Citation117 Serotonin opening of TJs could be a negative-feedback mechanism in lactation that might serve to dissipate the transepithelial gradients necessary for milk secretion.Citation116
Acetylcholine receptor mAChR
Acetylcholine (ACh) is an ester of acetic acid and choline that functions as a neurotransmitter at the neuromuscular junction. Activation of muscarinic Ach receptors (mAChRs) by carbachol and cevimeline reduced the TER and increased the permeability of rat salivary epithelial cells through a mechanism involving phosphorylation of claudin-4 by ERK1/2, which facilitated the interaction of claudin-4 with β-arrestin2, triggered claudin-4 internalization via clathrin and its subsequent ubiquitin-mediated degradation.Citation118
Histamine receptors
Histamine is an amine formed by the removal of a carboxyl group of the amino acid histidine. Histamine regulates a variety of physiological functions in the gut and acts as a neurotransmitter for the brain, spinal cord and uterus. It participates in the inflammatory response and plays a central role as a mediator of itching. For this purpose, histamine is produced by basophils and mast cells present in the connective tissue. Histamine signals via four G protein-coupled receptors named H1 to H4.
In cultured human nasal epithelial cells, histamine down-regulated ZO-1 mRNA through a process mediated by histamine receptor H1.Citation119 This receptor signals through Gαq/11 and promotes the expression of transcription factor NFκB that regulates the inflammatory process [for review see.Citation120]
In epithelial cells, histamine increased paracellular permeability and stimulated stress fiber formation, myosin light chain phosphorylation and occludin phosphorylation. However, only the cytoskeletal events were mediated by RhoA/ROCK activation.Citation121 Similarly, in endothelial HUVEC cells and in microlymphatic endothelial cells of dermal origin, histamine decreased TER through a process independent of myosin light chain kinase.Citation122
In a microfluidic model of microcirculation containing both blood and lymphatic vessels, treatment with histamine decreased the junctional localization of claudin-5 and VE-cadherin and increased the permeability of both type of vessels,Citation123 and in a blood-tumor barrier model, histamine increased paracellular permeability, decreased TER, disrupted the integrity of TJs and lowered the expression of ZO-1, occludin and claudin-5.Citation124
In endothelial cells histamine also alters the adherens junctions and their link to vimentin. Thus, in HUVEC cells histamine caused a transient and reversible disruption of binding between β-catenin and VE-cadherin,Citation125 induced serine/threonine phosphorylation of catenin p120 and its splice variant p100,Citation126 and decreased the amount of vimentin that immunoprecipitates with VE-cadherin.Citation127
MOR an opioid activated receptor
The activation of μ opioid receptor (MOR) with opioids in human non-small cell lung cancer cells increased the expression of snail, slug and vimentin and decreased the protein level of ZO-1 and claudin-1, consistent with an epithelial to mesenchymal transition.Citation128 This observation might explain why epidemiological studies have recently shown a diminished incidence of cancer recurrences following regional anesthesia with lower doses of opioids following surgeries for prostate and colon cancer.Citation129,Citation130
Chemokines activated receptors
Chemokines are chemotactic cytokines that control the migration and localization of immune cells. There are approximately 50 endogenous chemokines that bind to 20 different G protein-coupled receptors.
Receptors for CXC chemokines
CXC chemokines or α-chemokines have two N-terminal cysteines separated by one amino acid. In the central nervous system, the microglia functions as the immune system and accordingly, in brains of Alzheimer's disease microglia accumulates and surrounds the senile plaques of beta-amyloid. Since bone marrow-derived microglia originates from monocytes, interest has arisen in discovering how these monocytes cross the BBB. In this respect, it has been found that monocytes that over-express chemokine CXCL1, like those derived from Alzheimer's disease patients, decrease TER and increase the permeability of brain microvascular endothelial cells altering the distribution of ZO-1 and occludin, through a mechanism involving chemokine receptor CXCR2 and ROCK.Citation131 Moreover, in mice an antagonist to chemokine receptor CXCR4 named AMD3100, protects BBB integrity and reduces the inflammatory response after ischemia induced by middle cerebral artery occlusion,Citation132 and protects intestinal barrier function from experimental colitis preventing the decreased expression of claudins -1, -3, -5, -7 and -8 and the increase in claudin-2 expression triggered by DSS.Citation133 Interestingly, gliadin the toxic component of gluten that triggers celiac disease, binds to chemokine receptor CXCR3 in intestinal epithelial cells, inducing the recruitment of the adaptor protein MyD88 and zonulin release. The latter is an endogenous regulator of TJs that increases intestinal permeability enabling the paracellular passage of gliadin and other antigens from the lumen to the gut mucosa leading to the pathology associated with celiac disease.Citation134
Receptors for CC chemokines
CC chemokines or β-chemokines have two adjacent cysteines near their amino terminal. The monocyte chemoattractant protein-1 (MCP-1) also known as chemokine CCL2 besides its main function of recruiting leukocytes at sites of inflammation opens the BBB. Thus, studies performed in vitro with a co-culture of brain endothelial cells and astrocytes, and under in vivo conditions, demonstrate that MCP-1/CCL2 through the CCR2 receptor present in endothelial cells and signaling via RhoA and ROCK, increases BBB permeability altering the distribution and expression of occludin, claudin-5, ZO-1 and ZO-2,Citation135,Citation136 Accordingly, during ischemia-reperfusion injury, BBB permeability increased together with MCP-1/CCL2 secretion from both astrocytes and brain endothelial cells, while CCL2 blockade or the absence of CCR2 from endothelial cells decreased BBB permeability.Citation137
Another chemokine named eotaxin or CCL11 increased the permeability of coronary artery endothelial cells through receptor CCR3, down-regulating the expression of ZO-1, occludin and claudin-1, producing oxidative stress and the activation of p38MAPK, STAT3 and NFκB pathways.Citation138
Receptors activated by complement peptides
Anaphylatoxins or complement peptides C3a, C4a and C5a are peptide fragments produced by the activation of the complement system. C3, C4 and C5 are large complement glycoproteins, which are proteolytically activated by cleavage at specific sites, forming a- and b-fragments. Anaphylatoxins are synthesized in the liver and found in the blood and are known to cause muscle contraction, histamine release from mast cells and enhanced vascular permeability.
Exposure of an in vitro model of BBB to C5a or to serum from patients with systemic lupus, where complement activation occurs, decreased TER and the expression of claudin-5 and ZO-1, through the activation of G protein-coupled receptor C5aR/CD88.Citation139 The mechanism through which C5a disrupts BBB integrity involves NFκB signaling, since treatment with a C5aR/CD88 antagonist that preserved ZO-1 expression in mouse brain endothelial cells treated with lupus serum, reduced the translocation of NFκB into the nucleus.Citation140 These results help to explain why impaired BBB integrity and neurological complications are frequently associated to systemic lupus.
In epithelial cells as well, C5aR destabilizes cell-cell adhesion. Thus, in hepatocellular carcinoma cells, activation of C5aR/CD88 by C5a promoted cell invasion and migration through down-regulation of E-cadherin and claudin-1 and up-regulation of snail accompanied by the activation of ERK1/2 pathway.Citation141
Endothelin receptors activated by vasoconstricting peptides
Endothelins are 21-amino acid peptides that constrict blood vessels and raise blood pressure. In rat, status epilepticus induced the activation of endothelin B receptor, leading to nitric oxide synthase (eNOS) mediated activation of MMP-9 and degradation of claudin-2, occludin and ZO-1 in endothelia from brain cortex.Citation142 Moreover, transgenic mice with endothelial endothelin-1 over-expression show more severe vascular permeability and BBB breakdown after transient middle cerebral artery occlusion. The brain of these animals displayed oxidative stress, edema, increased MMP-2 expression, BBB leakage and a decreased occludin level. Interestingly, endothelin-1 antagonist A-147627 partially normalized the infarct volume and neurological deficit in these mice, highlighting the contribution of endothelin receptor activation to BBB breakdown under ischemia.Citation143
Frizzled receptors activated by Wnt ligands
The canonical Wnt pathway that promotes epithelial to mesenchymal transition and is involved in the development and progression of a wide variety of carcinomas, is activated by Wnt ligands that bind to the G protein-coupled receptor frizzled and the co-receptor LRP5/6. Frizzled receptors constitute a family that in humans has 11 members.Citation144 In the absence of a Wnt ligand, a complex of scaffold proteins APC and axin, and the kinases GSK3β, and casein kinase 1, is located in the cytosol. β-catenin is phosphorylated by these kinases and is then recognized and ubiquitinated by a multiprotein complex containing β-TrCP, leading to the degradation of β-catenin in the proteosome. However when Wnt ligands activate the pathway, LRP5/6 is phosphorylated by casein kinase 1 and GSK3β, triggering the translocation of the complex from the cytosol to the plasma membrane and the recruitment of dishevelled, another scaffold protein. In this membrane complex, where dishevelled binds to frizzled and axin binds to phosphorylated LRP5/6, β-catenin ubiquitination is suppressed, leading to saturation by accumulation of phosphorylated β-catenin.Citation145 As a result, newly synthesized β-catenin accumulates in the cytoplasm and enters into the nucleus where it binds to LEF/TCF transcription factor, promoting as a result the expression of a vast group of genes involved in EMT [for review see.Citation146]
Snail transcription factor that represses the transcription of E-cadherin, claudins and occludin, promoting TJ and adherens junctions disassembly and EMT,Citation147 is a target of GSK3β-dependent phosphorylation and β-TrCP-directed ubiquitination and proteosomal degradation.Citation148 Wnt signaling inhibits snail phosphorylation and increases in consequence snail level and activity.Citation149 This process is mediated by axin2. Thus, β-catenin at the nucleus upon association to LEF/TCF induces the expression of axin2,Citation150 which functions as a chaperone that promotes the exportation of GSK3β from the nucleus into the cytoplasm, inhibiting in consequence the turnover of snail.Citation151 Therefore activation of frizzled receptors by Wnt ligands disrupts TJ stability.
The placenta regulates the transfer of materials between the mother and the developing fetus. One of the earliest steps of placental development is branching of the sheet of trophoblast cells. These cells are present at the outer layer of the blastocyst, and branching increases the surface of the epithelium of the chorionic villi that exchanges nutrients, gases and wastes between the fetus and the mother's blood present in the intervillous space. Wnt ligands via frizzled-5 receptor and the activation of the Wnt signaling pathway, regulate chorionic branching. Thus, the up-regulation of frizzled 5 triggered by transcription factor Gcm 1 induces the dissociation of cell junctions needed for branching initiation through the down-regulation of ZO-1, claudin-4 and claudin-7 in trophoblast cells, at the base of the chorion.Citation152
Receptors activated by lipids
Receptors activated by the phospholipid platelet-activating factor
Platelet-activating factor (PAF) is a phospholipid involved in inflammation and thrombosis that activates a G protein-coupled receptor. Treatment with PAF increased endothelial permeability and triggered the formation of intercellular filopodia and lamellipodia through a process involving Rac1 activation and the association of Rac1 and its GEF, Tiam1, with PAF receptor (PAFR). This process is independent of myosin light chain phosphorylation and MLCK activation,Citation153,Citation154 Accordingly, serum from patients with severe dengue virus infection with evidence of vascular leakage, displayed high PAF levels and reduced TER and ZO-1 expression when tested on an endothelial cell line.Citation155 In caecum and distal colon PAF has also been reported to increase paracellular permeability.Citation156
Takeda G protein-coupled receptor 5 activated by bile acids
The transformation of normal esophageal squamous epithelium to reflux esophagitis, followed by Barrett's esophagus and dysplasia, can finally lead to esophageal adenocarcinoma. Chronic exposure to bile salts in gastro esophageal reflux disease induces inflammation mediated through the bile acid receptors, including the Takeda G protein-coupled receptor 5 (TGR5). Interestingly, in esophageal carcinoma and precancerous lesion, expression of TGR5 is associated with high expression of claudin-2,Citation157 which is a pore forming claudin whose overexpression has also been reported in colorectal and esophagus carcinomas.Citation158–160 and inflammatory bowel disease.Citation161
Receptors activated by extracellular nucleotides and nucleosides
Nucleotides are organic molecules constituted by three distinctive chemical units: a five-carbon sugar molecule and a nitrogenous base, which together are called a nucleoside, and one phosphate group. Therefore a nucleotide is also named a nucleoside monophosphate. However common usage has extended the definition in order to include as nucleotides the molecules with two or three phosphates also known respectively as nucleoside diphosphate and nucleoside triphosphate [for review see.Citation162]
Nucleotides contain either a purine (adenine or guanine) or a pyrimidine (thymine, uracil or cytosine) nitrogenous base, and are termed ribonucleotides if the sugar is ribose or deoxyribonucleotides if the sugar is deoxyribose. Nucleotides have several functions: 1) as monomer units for forming the nucleic acid polymers DNA and RNA, 2) as packets of chemical energy in the form of the nucleoside triphosphates ATP, GTP, CTP and UTP, 3) as signaling molecules in the form of cyclic nucleotides cGMP and cAMP, and 4) as cofactors of enzymatic reactions.
P2Y2 receptor activated by nucleotides
The P2Y family of G protein-coupled receptors, is activated by a wide range of extracellular mono and dinucleotides. P2Y2 receptor, which is activated by ATP, promotes cell invasion and metastasis in prostate cancer cells, triggering the expression of snail and inhibiting E-cadherin and claudin-1 expression.Citation163 The ability of P2Y2 receptor to disrupt epithelial TJ has been employed to improve ocular drug delivery. Thus, in human corneal epithelial cells, treatment with the dinucleotide P1,P4-Di (adenosine-5’) tetraphosphate (Ap4A) activated ERK and reduced TER and TJ protein levels through a process dependent on P2Y2 receptor. In addition, the topical application of Ap4A to rabbit eyes, disrupted ZO-1 membrane distribution in the cornea and improved the delivery of a hypotensive compound that reduces intraocular pressure, into the aqueous humour.Citation164
Adenosine activated receptors A1, A2A and A2B
Adenosine is a purine nucleoside that besides its role in the metabolism exerts physiological functions by interacting with four receptors: A1, A2A, A2B and A3. Adenosine is produced inside and outside of cells. Extracellular adenosine is generated by the conversion of adenosine triphosphate (ATP) to adenosine diphosphate (ADP) and adenosine monophosphate (AMP) by the extracellular enzyme CD39. AMP it then converted to adenosine by CD73, another extracellular enzyme.
Activation of adenosine receptors A1 and A2A increases BBB permeability, facilitating the entry of intravenously administered macromolecules into the brain, including compounds of therapeutic value like chemotherapeutic drugs and antibodies against β-amyloid. Opening of the BBB is reversible and mediated by a reorganization of the actin cytoskeleton induced by RhoA, and involves actomyosin stress fibers formation and a diminished expression of ZO-1, occludin, claudin-5 and VE-cadherin,Citation165,Citation166 These observations have led to the development of adenosine receptor agonists that have a longer circulation lifetime and hence exert a broader BBB opening time window that can be allowed to match with the pharmacokinetics of the therapeutic agent.Citation167
Adenosine receptor signaling exerts conflicting effects on the intestinal barrier. Thus, while some reported that inhibition of A2B adenosine receptors attenuated the decrease in TER and diminished claudin-1, occludin and ZO-1 expression, induced by ischemia/reperfusion injury or acute hypoxia,Citation168 others showed that adenosine receptor signaling induced by AMP cleavage, had a protective role against Clostridium difficile toxins TcdA and TcdB, reversing the reduced TER and increased paracellular permeability of intestinal cells.Citation169
G protein-coupled receptors with dual effect on TJs
Protease-activated receptors
PAR-2
Proteinase-activated receptor-2 (PAR-2) is a G protein-coupled receptor activated by a proteolytic cleavage on the N-terminal extracellular region that unmasks amino terminal residues that serve as tethered ligands that activate the receptor. PAR-2 is activated by trypsin, chymase and mast cell tryptase, which are highly expressed in the intestine. The colonic administration of PAR-2 agonist up-regulates PAR-2 expression and induces an inflammatory reaction that decreases transepithelial resistance.Citation170 and increases paracellular permeability,Citation171 and that is accompanied by the redistribution of perijunctional F-actin, ZO-1 and occludin.Citation172 and the reduction of claudin-5 expression.Citation170 The mechanism through which mast cells induce an inflammatory reaction in the colon following degranulation and the activation of PAR-2 involves association of the receptor to the multiadaptor protein β-arrestin that mediates activation of kinases ERK1/ERK2 which in turn re-organize the perijunctional ring of F-actin to increase epithelial permeability.Citation172 In Caco-2 cells, PAR-2 activation with chymase also involves MMP-2 expression and activation.
PAR-2 activation explains why infiltration of mast cells that are replete with proteases including tryptase, delocalizes TJ proteins and increases the permeability of the intestine that is inflamed due to chronic stress, cytokines, allergens and bacterial products. Moreover, the role of PAR-2 is critical to understand TJ disruption in patients with inflammatory bowel disease where luminal trypsin and tryptase are elevated,Citation173,Citation174 In this respect, it was found that mucosal application in mice of faecal supernatants with increased serine protease activity from diarrhea-predominant irritable bowel syndrome patients, increased colonic paracellular permeability in a manner dependent of PAR-2 expression.Citation175
Activation of PAR-2 by specific peptides also increases colon permeability. Hence, PAR-2 activation with the peptide SLIGRL increases colonic permeability and alters ZO-1 localization even without causing inflammation, via calmodulin that binds and activates MLCK.Citation176 In addition, the amino terminal portion of Vibrio cholerae-derived Zonula occludens toxin, has a PAR-2 activating motif (FCIGRL), that augments the phosphorylation by PKCα of ZO-1 and myosin. These modifications induce the dissociation of ZO-1 from occludin, claudin and myosin and open the TJ.Citation177 Nitric oxide and capsaicin-sensitive afferent neurons are also involved in PAR-2 mediated colonic inflammation and paracellular permeability increase.Citation178
Activation of PAR-2 by mast cell tryptase is also causative of stress-induced epithelial barrier dysfunction in the esophagus.Citation179
In epithelial MDCK cells, PAR-2 localizes at the lateral surface explaining, why the application of a PAR-2 activating peptide to the apical side of monolayers exerts no effect on TER (Citation180). In these cells PAR-2 leads to TJ disruption through the activation of p38MAPK.
In mice placenta instead, the expression of PAR-2 is critical for placental barrier function. Thus, mice double deficient in the serine protease matriptase and PAR-2 are embryonic lethal and die at midgestation despite normal development. The defect lies in the sealing of the placental epithelium in direct contact with the maternal blood, named the laberynth and composed of an external layer of syncytiotrophoblast and an underlying layer of cytotrophoblast that surround the mesenchyma with the fetal vessels. Thus, in matriptase and PAR-2 double deficient embryos, the placental epithelium is leaky to the paracellular marker inulin and has a significantly reduced expression of claudin-1.Citation181
PAR-1 and PAR-3
PAR-1 is the major thrombin activated receptor and plays a major role in platelet aggregation, inflammatory responses and neurodegeneration [for review see.Citation182] Thrombin promotes coupling of PAR-1 to Gα12/13 and Gαq and activation of RhoA.Citation183
Upon breakdown of the BBB thrombin enters the brain and activates PAR-1 receptors. Therefore, intracerebral injection of autologous blood produce damage to the brain parenchyma that is significantly reduced in PAR-1 KO mice or by the addition of the thrombin inhibitor, hirudin.Citation184 Similarly, intracerebral injection of thrombin in mice causes massive brain lesions and brain hemorrhages by BBB disruption, that are markedly reduced in PAR-1 KO mice,Citation185 and hypothermia, reduces brain water content in thrombin–induced brain edema by lowering the expression of PAR-1.Citation186 In addition, in an experimental model of multiple sclerosis known as experimental autoimmune encephalomyelitis, inactivation of PAR-1 with KC-A0590 preserved the expression of occludin and ZO-1 in brain endothelial cells and down-regulated the secretion of MMP-9 in astrocytes,Citation187 and under oscillatory sheer stress that accelerates atherogenesis, endothelial TJ are impaired through a mechanism that involves activation of PAR-1 followed by increased expression of Dickkopf 1, a protein that inhibits the Wnt signaling pathway.
These observations in principle indicate that inhibition of PAR-1 could be employed as a therapeutic strategy for decreasing BBB breakdown in a wide variety of pathological conditions. However, activation of PARs by other serine proteases like APC or factor Xa (FXa), can have a protective effect on endothelial barrier function. APC is an anticoagulant also known as blood coagulation factor XIV, that through association to its receptor EPCR allows activation of PAR-1.Citation188 Signaling of APC-activated PAR-1 is not mediated by heterotrimeric G proteins signaling, but through β-arrestin and dishevelled-2 scaffolds and Rac1 activation.Citation189 While thrombin activation of PAR-1 involves a canonical cleavage at Arg41, activation of PAR-1 by APC generates a non-canonical proteolysis at Arg46.Citation190 FXa, a protease critical in the blood coagulation cascade, can cleave PAR-3 at non-canonical Arg41, similar to APC.Citation191
Activation of PAR-1 or PAR-3 by APC or FXa requires the presence of EPCR and involves activation of Tie2, a transmembrane tyrosine kinase receptor for angiopoietin, and results in up-regulation and translocation of ZO-1 to cell borders and stabilization of endothelial TJs.Citation191,Citation192 This mechanism explains why recombinant human APC has been approved for patients with severe sepsis, where increased mortality is associated with bleeding risk.Citation193
In epithelial cells a similar mechanism promotes TJ barrier integrity. Thus in keratinocytes APC activates Tie2 through a mechanism that requires the sequential activation of EPCR, PAR-1 and EGFR that in turn enhances PI3K/AKT signaling, increases the expression of ZO-1 and claudin-1 and reduces paracellular permeability.Citation194
Hormone receptors
Angiotensin II activated receptor AT1
Angiotensin II (Ang II) is a peptide hormone that causes vasoconstriction and subsequently increases blood pressure. It also stimulates the release of aldosterone from the adrenal cortex, which promotes sodium retention and augments blood pressure. These effects of Ang II are mediated by G-coupled receptor AT1. In the kidney, podocytes are the cells responsible of the glomerular filtration barrier. Podocytes instead of exhibiting TJ between their neighboring foot processes, form a structure named slit diaphragm that allows the passage of molecules up to 70 oA, in contrast to TJ that prevent the passage of molecules >8oA. Slit diaphragms are constituted by some proteins common to TJs like JAM-A, occludin, cingulin and ZO-1 and other unique membrane proteins like nephrin and podocinCitation195 ().
In podocytes in culture, Ang II changes the pattern of organization of F-actin and ZO-1. Thus, the transcytoplasmic F-actin filaments disappear and ZO-1 distribution at cell-cell contacts becomes fragmented through a process mediated by the activation of Src kinase and phospholipase C.Citation196 Accordingly, in male MWF rats that develop spontaneous proteinuria with age, treatment with lisinopril, an inhibitor of angiotensin converting enzyme, preserves the distribution of ZO-1 at the slit diaphragm in the glomeruli, and in consequence prevents proteinuria and increases glomerular ultrafiltration coefficient.Citation197 Moreover, blockade of AT1 with ZD7155 attenuates the reduced expression and cytoplasmic redistribution of ZO-1, observed in rat glomerular epithelial cells exposed to high glucose and in diabetic rats.Citation198 Interestingly, a combined therapy with an AT1 blocker and a vitamin D analog that suppresses renin biosynthesis, which hydrolyses angiotensinogen to angiotensin I, prevents renal injury in diabetic nephropathy by reversing the decline of slit diaphragm proteins nephrin-1, ZO-1 and α-actinin-4.Citation199
Ang II also regulates endothelial TJs. Thus, Ang II promotes diabetic retinopathy by inducing VEGF expression and loss of TJ proteins in retinal endothelial cells. In accordance, blockade of Ang II with an inhibitor of angiotensin converting enzyme blocked these effects and attenuated the increased vascular permeability of diabetic retina.Citation200 The effect of Ang II on the BBB remains controversial. Thus, some have reported that in brain microvessel endothelial cells Ang II induced through AT1 activation an increase in albumin permeability and a decrease in TER that is not accompanied by changes in the expression of occludin and claudin-5.Citation201 Others instead, observed that angiotensin KO animals have a disorganized pattern of occludin at the BBB, and that activation of AT1 diminishes the permeability of BBB endothelial cells and mobilizes occludin to lipid rafts membrane microdomains.Citation202 Accordingly, multiple sclerosis lesions with a leaky BBB have a reduced number of perivascular astrocytes positive for angiotensinogen.Citation202
Corticotropin-releasing hormone receptors CRHR1 and CRHR2
Corticotropin-releasing hormone (CRH) is a 41-amino acid peptide secreted by the hypothalamus in response to stress, whose main function is the stimulation of the synthesis of adrenocorticotropic hormone by the anterior pituitary gland. In breast cancer cells, CRH inhibits TGFβ1-mediated transformation mainly via G-coupled CRH receptor 2 (CRHR2), inducing the expression of E-cadherin and occludin and down-regulating that of snail1 and twist1.Citation203 Instead, activation of CRHR1 exerts a negative effect in TJs of the intestine. Thus, in the Wistar Kyoto rat model of irritable bowel syndrome, the administration of anti IL-6 and CRHR1 antagonist antalarmin, normalized stress-induced defecation and visceral pain sensitivity through a mechanism that involves a reduction in the overexpression of claudin-2.Citation204 In a similar sense, fish oil enhanced intestinal barrier function by increasing the expression of claudin-1, via inhibition of CRHR1 signaling in weaned pigs after lipopolysaccharide challenge.Citation205
Membrane bound G protein-coupled estrogen receptor GPR30
Estrogen is the primary female sex hormone responsible for the development and regulation of the female reproductive system and secondary sex characteristics. In women, estrone, estradiol and estriol constitute the major naturally occurring forms of estrogen, being estradiol the most potent and prevalent estrogen. The canonical mechanism of estrogen action is through nuclear receptors ERα and ERβ, which stimulate gene expression by acting as transcription factors. The non-classical mechanism instead, involves action through GPR30, a G protein-coupled estrogen receptor found in the membrane of the endoplasmic reticulum that promotes signaling through Zn2+-dependent matrix metalloproteinases, EGFR, PI3K, Ca2+ mobilization and nitric oxide production. In human hepatoma cells, activation of GPR30 leads to cleavage of occludin by MMP-9. Since occludin is a receptor for hepatitis C virus, these results contribute to explain why hepatocellular carcinoma and cirrhosis, in response to hepatitis C virus, is more associated with men and postmenopausal women than with premenopausal women and women receiving hormone replacement therapy.Citation206 Likewise, 2,2’,4,4’-tetrabromodiphenyl ether (BDE-47) increases migration and invasion of neuroblastoma cells, by down-regulating E-cadherin and ZO-1 expression and upregulating MMP-9 via the GPR30/PI3K/AKT signaling pathway.Citation207 In contrast, in the brain GPR30 activation reduces occludin and claudin-5 disruption in the BBB, via inhibition of VEGF expression after ischemic injury.Citation208
Prostaglandin E2 receptors
Prostaglandins PGE2, PGF2α and PGI2 are lipid autacoids, meaning that these prostanoids, product of cyclooxygenase mediated metabolism of arachidonic acid, are locally produced and metabolized and usually act near their site of synthesis.
During the differentiation of Caco-2 intestinal epithelial cells, a decrease in cyclooxygenase-2 expression and PGE2 synthesis was observed, accompanied by a reduction in paracellular permeability, while the addition of PGE2 to differentiated cells disrupted the epithelial barrier function,Citation209 through activation of EP1 and EP4 G-coupled PGE2 receptors. This process that involved redistribution of occludin, ZO-1 and perijunctional actin, and increased MLCK activity, was mediated through the PLC/IP3/ Ca2+ /PKC and cAMP-PKA signaling pathways.Citation210 Another study revealed that PGE2 receptor EP2 was also involved in TJ disassembly in Caco-2 cells by PGE2 through a mechanism mediated by PKC and not PKA.Citation211 However, the presence of PGE2 receptor EP2 appears to be crucial for maintaining TJ barrier integrity, since EP2 receptor antagonism or siRNA based silencing decreased claudin-4 expression by proteosomal degradation decreasing in consequence the TER of Caco-2 cells.Citation212
In rat brain, post-ischemic treatment with EP1 antagonist, SC-51089 or EP1 genetic ablation, reduces MMP-9/3 activity and preserves ZO-1 and occludin at the BBB, highlighting the possibility of targeting EP1 receptor to diminish stroke induced neurovascular damage.Citation213
Bradykinin activated receptor BR2
Bradykinin is a 9-amino acid peptide generated by the proteolytic cleavage of high-molecular weight kininogen by the enzymes kallikreins, and is degraded by kininases including angiotensin-converting enzyme. Bradykinin dilates blood vessels causing a fall in blood pressure. Bradykinin and its long-lasting analogue labradimil/RPM-7, selective for the G-coupled bradykinin receptor 2 (BR2), induce a higher increase in permeability in the blood-brain tumor barrier than in the BBB. This feature is of utmost therapeutic importance as it allows the delivery of hydrophilic chemotherapeutics,Citation214,Citation215 and virus vectors with killing-genes.Citation216 to brain tumors and not to healthy brain tissue. In brain microvascular endothelial cells BR2 through Gαi inhibits adenylyl cyclase decreasing the expression of cAMP and PKA and through Gαq stimulates PLC to increase intracellular calcium. These changes alter the perijunctional actin cytoskeleton and down-regulates the expression of ZO-1, occludin and claudin-5.Citation217 The increase in blood-brain tumor barrier permeability is also mediated by ROCK activation that induces TJ protein relocalization, stress fibers formation, and MLC and cofilin phosphorylation,Citation218,Citation219 and by a NOS dependent mechanism that induces the translocation to the nucleus of ZONAB transcription factor, which inhibits the promoters of claudin-5 and occludin genes, resulting in a reduced expression of the corresponding TJ proteins.Citation220
In BR1 KO mice, the associated compensatory over-expression of BR2 in the intestine exacerbates the DSS-induced colitis, decreasing occludin gene expression.Citation221 In contrast, bradykinin decreased podocyte permeability and increased ZO-1 staining at the cell borders, through activation of BR2 that transactivates EGFR by the disintegrin and metalloenzyme ADAM-17.Citation222
Regulation of colonic, renal, endothelial, blood-retinal and blood-brain barriers by different G protein-coupled receptors
In this section we summarize the interplay between different G protein-coupled receptors in epithelial and endothelial barriers, highlighting the studies where a clear mechanistic link to TJs has been found.
Colon
shows that opening and closure of TJ in colon epithelia is regulated by a wide variety of G protein-coupled receptors. Signaling through Gαs and Gαq/11 modulates both TJ closure and opening, whereas signaling via Gαi promotes TJ closure.
TJ formation and closure is obtained upon activation of Calcrl receptor that leads to a decreased phosphorylation of MLC and the STAT transcription factors,Citation66,Citation67; the activation of CBR1 and CBR2 receptors that lower the expression of NFκB and cytokinesCitation88,Citation89 and upon activation of AMPKCitation51 and p70S6KCitation37 by receptors CaSR and GPR39, respectively. A decrease in TNF-α that leads to TJ closure appears to be a common step employed by the activation of several GPCRs including CBR1,Citation66 CBR2,Citation67 and S1PR1Citation99 receptors.
The impact of ERK on intestinal TJs is difficult to understand due to contradictory observations. Thus for example, TJ closure has been reported in colon due to a decrease in ERK phosphorylation induced by SSTR,Citation70 while others have reported the same effect owed to ERK activation by receptors GPR40Citation93 or OGR1.Citation29 Moreover, receptor CRHR1 activation of Gαs promotes ERK1/2 activation that opens intestinal TJs.Citation204
TJ opening in intestine is also achieved by activation of EP1 receptor through Gαq/11 that activates PKC and MLCK, inducing the contraction of prerijunctional actin and the formation of stress fibers.Citation210 In colon, G-protein coupled receptors also open TJs signaling through routes independent of Gα. Thus, receptor CXCR3 forms a complex with the adaptor protein MyD88 and gliadin, the toxic compound of gluten, which increases the expression zonulin that opens TJs,Citation134 and PAR-2 through β-arrestin activates ERK1/2 and induces TJ opening.Citation172
Slit diaphragm in the glomerulus
Closure of the slit diaphragm between podocytes in the glomerulus, is induced by BR2 receptor via Gαi through activation of ERK,Citation222 while the opening is triggered by AT1 receptor via Gαq/11 and a decrease in actin bundle formation.Citation196 ().
Blood brain barrier
A wide diversity of GPCRs regulate BBB opening and closure () and while signaling through Gαs and Gαi is implicated in both processes, only Gαq/11 is involved in BBB opening. The mechanisms triggered by GPCRs that promote BBB closure include the participation of Sox-17 transcription factor induced by the activation of frizzled-4 receptor by norrin protein, a non-Wnt ligand,Citation107 and a decrease in iNOS accompanied by an augmentation of eNOS due to SSTR1 receptor activation.Citation69 The latter point appears to be controversial since other studies have shown that an increase in eNOS by activation of receptors ETBCitation142 and BR2Citation220 lead to BBB opening.
The BBB appears to be very sensitive to PKA levels, as activation of PKA by receptor GLPR1 tightens the barrier,Citation74 while reducing cAMP and PKA levels through BR2 activation opens the BBB Citation217 In addition, activation of MMPs by EP1 receptor,Citation213 of NFκB by C5aRCitation140 or RhoA by BR2 receptorCitation218 also promote BBB opening.
Inner blood-retinal barrier
In the retina it is surprising to observe that both Gαs and Gαi, which exert opposite effects on adenyly cyclase, mediate closure of the iBRB (). However, upon closer examination it becomes clear that activation of MC1, MC5Citation76 and CalcrlCitation64 receptors that signal through Gαs, and S1PR1 and S1PR3 Citation97 receptors that signal via Gαi, leads to a decrease in NFκB that induces closure of the iBRB. Other mechanisms that lead to iBRB closure include activation of Gαs by GLPR1 that induces a decrease in AKT phosphorylation, which diminishes the phosphorylation and activity of STAT3,Citation75 as well as the activation of Gαi by δ opioid receptor that triggers a decrease in TNF-α, iNOS and caveolin endocytosis.Citation102
Endothelia in tissues different to the retina or the brain
In endothelia present in tissues different to the retina or the brain, the four Gα subunits are involved in both TJ opening and closure (). It is noteworthy that the outcome of Calcrl activation varies depending on the kind of endothelia. Thus, in rat coronary microvascular endothelial cells (RCECs) signaling through Gαs leads to an increase in cAMP and PKA activation that blocks RhoA and Rac1 activation and in consequence produces barrier failure.Citation57 Instead, in HUVEC Gαs activation by Calcrl decreases RhoA activity as in RCEC but increases Rac1 activity leading to endothelial barrier enhancement,Citation56,Citation57 The reason why Calcrl exerts opposites effects on Rac1 in these endothelia remains unknown.
The appearance of stress fibers is deleterious for endothelial TJ closure. Hence, an increase in these fibers triggered by S1PR3 debilitates the barrier,Citation223 while a decrease in stress fibers induced by Calcrl promotes TJ closure.Citation53–57 In endothelia, stimulation of Gαi by CCR3 augments the expression of NFκB and promotes TJ opening,Citation138 in agreement to what has been observed in colonCitation93 and retina.Citation97 Other GPCRs that induce TJ closure include PAR-1 activation of Gα12/13 that through β-arrestin stimulates PI3K and AKTCitation190; and PAR-3 that through Gαq/11 leads to the activation of angiopoietin receptor Tie2.Citation191
Concluding remarks
GPCRs activated by diverse stimuli like ions, hormones, lipids, chemokines, nucleotides, proteases and light, signal through heterotrimeric G proteins, arrestins and kinases, to modulate the degree of sealing of TJs in epithelial and endothelial cells throughout the organism. Activation of these receptors regulates TJ proteins by changing their level of expression, localization at the cell borders or degree of phosphorylation. Another mechanism triggered by some GPCRs to induce TJ opening is contraction of the cortical ring of actomyosin. In the BBB, BRB, keratinocytes, colon epithelia, renal tubular cells and the glomerulus, numerous studies have been done revealing the participation of a wide variety of GPCRs in TJ modulation. Nevertheless, the relationship between GPCRs and TJs has not always been studied in depth and deeper knowledge of the mechanisms involved is needed. In addition, it would be helpful if future studies provide insight on the interplay between different GPCRs that act on the same epithelial and endothelial barriers.
Disclosure of potential conflicts of interest
No potential conflicts of interest were disclosed.
Additional information
Funding
References
- Cereijido M, Valdes J, Shoshani L, Contreras RG. Role of tight junctions in establishing and maintaining cell polarity. Annual Rev Physiol. 1998;60:161–77. doi:10.1146/annurev.physiol.60.1.161.
- van Meer G, Simons K. The function of tight junctions in maintaining differences in lipid composition between the apical and the basolateral cell surface domains of MDCK cells. EMBO J. 1986;5(7):1455–64.
- Dragsten PR, Blumenthal R, Handler JS. Membrane asymmetry in epithelia: Is the tight junction a barrier to diffusion in the plasma membrane? Nature. 1981;294(5843):718–22. doi:10.1038/294718a0.
- Nava P, Lopez S, Arias CF, Islas S, Gonzalez-Mariscal L. The rotavirus surface protein VP8 modulates the gate and fence function of tight junctions in epithelial cells. J Cell Sci. 2004;117(Pt 23):5509–19. Epub 2004/10/21. doi:10.1242/jcs.01425.
- Baas AF, Kuipers J, van der Wel NN, Batlle E, Koerten HK, Peters PJ, Clevers HC. Complete polarization of single intestinal epithelial cells upon activation of LKB1 by STRAD. Cell. 2004;116(3):457–66. doi:10.1016/S0092-8674(04)00114-X.
- Farquhar MG, Palade GE. Junctional complexes in various epithelia. J Cell Biol 1963;17:375–412. Epub 1963/05/01. doi:10.1083/jcb.17.2.375.
- Staehelin LA. Further observations on the fine structure of freeze-cleaved tight junctions. J Cell Sci. 1973;13(3):763–86.
- Staehelin LA. Structure and function of intercellular junctions. Int Rev Cytol. 1974;39:191–283. doi:10.1016/S0074-7696(08)60940-7.
- Furuse M. Molecular basis of the core structure of tight junctions. Cold Spring Harbor Perspectives Biol. 2010;2(1):a002907. doi:10.1101/cshperspect.a002907.
- Gunzel D, Yu AS. Claudins and the modulation of tight junction permeability. Physiological reviews. 2013;93(2):525–69. Epub 2013/04/17. doi:10.1152/physrev.00019.2012.
- Raleigh DR, Marchiando AM, Zhang Y, Shen L, Sasaki H, Wang Y, Long M, Turner JR. Tight junction-associated MARVEL proteins marveld3, tricellulin, and occludin have distinct but overlapping functions. Mol Biol Cell. 2010;21(7):1200–13. doi:10.1091/mbc.E09-08-0734.
- Ikenouchi J, Furuse M, Furuse K, Sasaki H, Tsukita S, Tsukita S. Tricellulin constitutes a novel barrier at tricellular contacts of epithelial cells. J Cell Biol. 2005;171(6):939–45. doi:10.1083/jcb.200510043.
- Garrido-Urbani S, Bradfield PF, Imhof BA. Tight junction dynamics: The role of junctional adhesion molecules (JAMs). Cell Tissue Res. 2014;355(3):701–15. doi:10.1007/s00441-014-1820-1.
- Furuse M, Izumi Y, Oda Y, Higashi T, Iwamoto N. Molecular organization of tricellular tight junctions. Tissue barriers. 2014;2:e28960. doi:10.4161/tisb.28960.
- Gonzalez-Mariscal LM, Ortega-Olvera JM, Gallego-Guitierrez H, Raya-Sandino A, Vargas-Sierra O. Involvement of tight junction plaque proteins in cancer. Curr Pathobiol Reports. 2016;4:117. doi:10.1007/s40139-016-0108-4.
- Gonzalez-Mariscal LM, Ortega-Olvera JM, Gallego-Guitierrez H, Raya-Sandino A, Vargas-Sierra O. Zonula occludens proteins in cancer. Curr Pathobiol Reports. 2016;4:107. doi:10.1007/s40139-016-0109-3.
- Gonzalez-Mariscal L, Tapia R, Chamorro D. Crosstalk of tight junction components with signaling pathways. Biochim Et Biophys Acta. 2008;1778(3):729–56. Epub 2007/10/24. doi:10.1016/j.bbamem.2007.08.018.
- Stevens RC, Cherezov V, Katritch V, Abagyan R, Kuhn P, Rosen H, Wüthrich K. The GPCR Network: A large-scale collaboration to determine human GPCR structure and function. Nat Rev Drug Discovery. 2013;12(1):25–34. doi:10.1038/nrd3859.
- Luttrell LM, Lefkowitz RJ. The role of beta-arrestins in the termination and transduction of G-protein-coupled receptor signals. J Cell Sci. 2002;115(Pt 3):455–65.
- Wu J, Xie N, Zhao X, Nice EC, Huang C. Dissection of aberrant GPCR signaling in tumorigenesis–a systems biology approach. Cancer genomics & proteomics. 2012;9(1):37–50.
- Balda MS, Gonzalez-Mariscal L, Contreras RG, Macias-Silva M, Torres-Marquez ME, Garcia-Sainz JA, Cereijido M. Assembly and sealing of tight junctions: possible participation of G-proteins, phospholipase C, protein kinase C and calmodulin. J Membrane Biol. 1991;122(3):193–202. doi:10.1007/BF01871420.
- Meyer TN, Schwesinger C, Denker BM. Zonula occludens-1 is a scaffolding protein for signaling molecules. Galpha(12) directly binds to the Src homology 3 domain and regulates paracellular permeability in epithelial cells. J Biol Chem. 2002;277(28):24855–8. doi:10.1074/jbc.C200240200.
- Sabath E, Negoro H, Beaudry S, Paniagua M, Angelow S, Shah J, Grammatikakis N, Yu AS, Denker BM. Galpha12 regulates protein interactions within the MDCK cell tight junction and inhibits tight-junction assembly. J Cell Sci. 2008;121(Pt 6):814–24. doi:10.1242/jcs.014878.
- Denker BM, Saha C, Khawaja S, Nigam SK. Involvement of a heterotrimeric G protein alpha subunit in tight junction biogenesis. J Biol Chem. 1996;271(42):25750–3. doi:10.1074/jbc.271.42.25750.
- Saha C, Nigam SK, Denker BM. Involvement of Galphai2 in the maintenance and biogenesis of epithelial cell tight junctions. J Biol Chem. 1998;273(34):21629–33. doi:10.1074/jbc.273.34.21629.
- Tuteja N. Signaling through G protein coupled receptors. Plant Signal Behav. 2009;4(10):942–7. doi:10.4161/psb.4.10.9530.
- Mohebbi N, Benabbas C, Vidal S, Daryadel A, Bourgeois S, Velic A, Ludwig MG, Seuwen K, Wagner CA. The proton-activated G protein coupled receptor OGR1 acutely regulates the activity of epithelial proton transport proteins. Cell Physiol Biochem. 2012;29(3-4):313–24. doi:10.1159/000338486.
- Ludwig MG, Vanek M, Guerini D, Gasser JA, Jones CE, Junker U, Hofstetter H, Wolf RM, Seuwen K. Proton-sensing G-protein-coupled receptors. Nature. 2003;425(6953):93–8. doi:10.1038/nature01905.
- de Valliere C, Vidal S, Clay I, Jurisic G, Tcymbarevich I, Lang S, Ludwig MG, Okoniewski M, Eloranta JJ, Kullak-Ublick GA, et al. The pH-sensing receptor OGR1 improves barrier function of epithelial cells and inhibits migration in an acidic environment. Am J Physiol Gastrointestinal Liver Physiol. 2015;309(6):G475–90. doi:10.1152/ajpgi.00408.2014.
- de Valliere C Wang Y, Eloranta JJ, Vidal S, Clay I, Spalinger MR, Tcymbarevich I, Terhalle A, Ludwig MG, Suply T, et al. G Protein-coupled pH-sensing Receptor OGR1 Is a Regulator of Intestinal Inflammation. Inflammatory Bowel Dis. 2015;21(6):1269–81.
- Roohani N, Hurrell R, Kelishadi R, Schulin R. Zinc and its importance for human health: An integrative review. J Res Med Sci. 2013;18(2):144–57.
- Besser L, Chorin E, Sekler I, Silverman WF, Atkin S, Russell JT, Hershfinkel M. Synaptically released zinc triggers metabotropic signaling via a zinc-sensing receptor in the hippocampus. J Neurosci Official J Soc Neurosci. 2009;29(9):2890–901. doi:10.1523/JNEUROSCI.5093-08.2009.
- Moechars D, Depoortere I, Moreaux B, de Smet B, Goris I, Hoskens L, Daneels G, Kass S, Ver Donck L, Peeters T, et al. Altered gastrointestinal and metabolic function in the GPR39-obestatin receptor-knockout mouse. Gastroenterology. 2006;131(4):1131–41. doi:10.1053/j.gastro.2006.07.009.
- Hershfinkel M, Moran A, Grossman N, Sekler I. A zinc-sensing receptor triggers the release of intracellular Ca2+ and regulates ion transport. Proc Natl Acad Sci U S A. 2001;98(20):11749–54. doi:10.1073/pnas.201193398.
- McKee KK, Tan CP, Palyha OC, Liu J, Feighner SD, Hreniuk DL, Smith RG, Howard AD, Van der Ploeg LH. Cloning and characterization of two human G protein-coupled receptor genes (GPR38 and GPR39) related to the growth hormone secretagogue and neurotensin receptors. Genomics. 1997;46(3):426–34. doi:10.1006/geno.1997.5069.
- Zhang JV, Ren PG, Avsian-Kretchmer O, Luo CW, Rauch R, Klein C, Hsueh AJ. Obestatin, a peptide encoded by the ghrelin gene, opposes ghrelin's effects on food intake. Science. 2005;310(5750):996–9. doi:10.1126/science.1117255.
- Cohen L, Sekler I, Hershfinkel M. The zinc sensing receptor, ZnR/GPR39, controls proliferation and differentiation of colonocytes and thereby tight junction formation in the colon. Cell Death Dis. 2014;5:e1307. doi:10.1038/cddis.2014.262.
- Sunuwar L, Medini M, Cohen L, Sekler I, Hershfinkel M. The zinc sensing receptor, ZnR/GPR39, triggers metabotropic calcium signalling in colonocytes and regulates occludin recovery in experimental colitis. Philos Trans R Soc Lond B Biol Sci. 2016;371(1700):20150420. doi:10.1098/rstb.2015.0420.
- Shao YX, Lei Z, Wolf PG, Gao Y, Guo YM, Zhang BK. Zinc Supplementation, via GPR39, Upregulates PKCzeta to Protect Intestinal Barrier Integrity in Caco-2 Cells Challenged by Salmonella enterica Serovar Typhimurium. J Nutr. 2017;147:1282. doi:10.3945/jn.116.243238.
- Sharir H, Zinger A, Nevo A, Sekler I, Hershfinkel M. Zinc released from injured cells is acting via the Zn2+-sensing receptor, ZnR, to trigger signaling leading to epithelial repair. J Biol Chem. 2010;285(34):26097–106. doi:10.1074/jbc.M110.107490.
- Greger R. Ion transport mechanisms in thick ascending limb of Henle's loop of mammalian nephron. Physiol Rev. 1985;65(3):760–97.
- Hou J, Renigunta A, Gomes AS, Hou M, Paul DL, Waldegger S, Goodenough DA. Claudin-16 and claudin-19 interaction is required for their assembly into tight junctions and for renal reabsorption of magnesium. Proc Natl Acad Sci U S A. 2009;106(36):15350–5. doi:10.1073/pnas.0907724106.
- Hou J, Renigunta A, Konrad M, Gomes AS, Schneeberger EE, Paul DL, Waldegger S, Goodenough DA. Claudin-16 and claudin-19 interact and form a cation-selective tight junction complex. J Clin Invest. 2008;118(2):619–28.
- Gong Y, Renigunta V, Himmerkus N, Zhang J, Renigunta A, Bleich M, Hou J. Claudin-14 regulates renal Ca(+)(+) transport in response to CaSR signalling via a novel microRNA pathway. EMBO J. 2012;31(8):1999–2012. doi:10.1038/emboj.2012.49.
- Ikari A, Okude C, Sawada H, Sasaki Y, Yamazaki Y, Sugatani J, Degawa M, Miwa M. Activation of a polyvalent cation-sensing receptor decreases magnesium transport via claudin-16. Biochim Et Biophys Acta. 2008;1778(1):283–90. doi:10.1016/j.bbamem.2007.10.002.
- Toka HR, Al-Romaih K, Koshy JM, DiBartolo S, 3rd Kos CH, Quinn SJ, Curhan GC, Mount DB, Brown EM, Pollak MR. Deficiency of the calcium-sensing receptor in the kidney causes parathyroid hormone-independent hypocalciuria. J Am Soc Nephrol. 2012;23(11):1879–90. doi:10.1681/ASN.2012030323.
- Gong Y, Hou J. Claudin-14 underlies Ca(+)(+)-sensing receptor-mediated Ca(+)(+) metabolism via NFAT-microRNA-based mechanisms. J Am Soc Nephrol. 2014;25(4):745–60. doi:10.1681/ASN.2013050553.
- Gong Y, Himmerkus N, Plain A, Bleich M, Hou J. Epigenetic regulation of microRNAs controlling CLDN14 expression as a mechanism for renal calcium handling. J Am Soc Nephrol. 2015;26(3):663–76. doi:10.1681/ASN.2014020129.
- Troy TC, Li Y, O'Malley L, Turksen K. The temporal and spatial expression of Claudins in epidermal development and the accelerated program of epidermal differentiation in K14-CaSR transgenic mice. Gene Expression Patterns: GEP. 2007;7(4):423–30. doi:10.1016/j.modgep.2006.11.006.
- Jouret F, Wu J, Hull M, Rajendran V, Mayr B, Schofl C, Geibel J, Caplan MJ. Activation of the Ca(2)+-sensing receptor induces deposition of tight junction components to the epithelial cell plasma membrane. J Cell Sci. 2013;126(Pt 22):5132–42. doi:10.1242/jcs.127555.
- Muanprasat C, Wongkrasant P, Satitsri S, Moonwiriyakit A, Pongkorpsakol P, Mattaveewong T, Pichyangkura R, Chatsudthipong V. Activation of AMPK by chitosan oligosaccharide in intestinal epithelial cells: Mechanism of action and potential applications in intestinal disorders. Biochem Pharmacol. 2015;96(3):225–36. doi:10.1016/j.bcp.2015.05.016.
- Garcia-Ponce A, Chanez Paredes S, Castro Ochoa KF, Schnoor M. Regulation of endothelial and epithelial barrier functions by peptide hormones of the adrenomedullin family. Tissue Barriers. 2016;4(4):e1228439. doi:10.1080/21688370.2016.1228439.
- Koyama T, Ochoa-Callejero L, Sakurai T, Kamiyoshi A, Ichikawa-Shindo Y, Iinuma N, Arai T, Yoshizawa T, Iesato Y, Lei Y, et al. Vascular endothelial adrenomedullin-RAMP2 system is essential for vascular integrity and organ homeostasis. Circulation. 2013;127(7):842–53. doi:10.1161/CIRCULATIONAHA.112.000756.
- Garcia Ponce A, Citalan Madrid AF, Vargas Robles H, Chanez Paredes S, Nava P, Betanzos A, Zarbock A, Rottner K, Vestweber D, Schnoor M. Loss of cortactin causes endothelial barrier dysfunction via disturbed adrenomedullin secretion and actomyosin contractility. Scientific Reports. 2016;6:29003. doi:10.1038/srep29003.
- Hippenstiel S, Witzenrath M, Schmeck B, Hocke A, Krisp M, Krull M, Seybold J, Seeger W, Rascher W, Schütte H, et al. Adrenomedullin reduces endothelial hyperpermeability. Circulation Res. 2002;91(7):618–25. doi:10.1161/01.RES.0000036603.61868.F9.
- Aslam M, Pfeil U, Gunduz D, Rafiq A, Kummer W, Piper HM, Noll T. Intermedin (adrenomedullin2) stabilizes the endothelial barrier and antagonizes thrombin-induced barrier failure in endothelial cell monolayers. Br J Pharmacol. 2012;165(1):208–22. doi:10.1111/j.1476-5381.2011.01540.x.
- Aslam M, Gunduz D, Schuler D, Li L, Sharifpanah F, Sedding D, Piper HM, Noll T. Intermedin induces loss of coronary microvascular endothelial barrier via derangement of actin cytoskeleton: role of RhoA and Rac1. Cardiovascular Res. 2011;92(2):276–86. doi:10.1093/cvr/cvr213.
- Muller-Redetzky HC, Kummer W, Pfeil U, Hellwig K, Will D, Paddenberg R, Tabeling C, Hippenstiel S, Suttorp N, Witzenrath M. Intermedin stabilized endothelial barrier function and attenuated ventilator-induced lung injury in mice. PloS One. 2012;7(5):e35832. doi:10.1371/journal.pone.0035832.
- Dunworth WP, Fritz-Six KL, Caron KM. Adrenomedullin stabilizes the lymphatic endothelial barrier in vitro and in vivo. Peptides. 2008;29(12):2243–9. doi:10.1016/j.peptides.2008.09.009.
- Hoopes SL, Willcockson HH, Caron KM. Characteristics of multi-organ lymphangiectasia resulting from temporal deletion of calcitonin receptor-like receptor in adult mice. PloS One. 2012;7(9):e45261. doi:10.1371/journal.pone.0045261.
- Honda M, Nakagawa S, Hayashi K, Kitagawa N, Tsutsumi K, Nagata I, Niwa M. Adrenomedullin improves the blood-brain barrier function through the expression of claudin-5. Cell Mol Neurobiol. 2006;26(2):109–18. doi:10.1007/s10571-006-9028-x.
- Kis B, Deli MA, Kobayashi H, Abraham CS, Yanagita T, Kaiya H, Isse T, Nishi R, Gotoh S, Kangawa K, et al. Adrenomedullin regulates blood-brain barrier functions in vitro. Neuroreport. 2001;12(18):4139–42. doi:10.1097/00001756-200112210-00055.
- Kis B, Snipes JA, Deli MA, Abraham CS, Yamashita H, Ueta Y, Busija DW. Chronic adrenomedullin treatment improves blood-brain barrier function but has no effects on expression of tight junction proteins. Acta Neurochirurgica Supplement. 2003;86:565–8.
- Imai A, Toriyama Y, Iesato Y, Hirabayashi K, Sakurai T, Kamiyoshi A, Ichikawa-Shindo Y, Kawate H, Tanaka M, Liu T, et al. Adrenomedullin suppresses vascular endothelial growth factor-induced vascular Hyperpermeability and inflammation in retinopathy. Am J Pathol. 2017;187(5):999–1015. doi:10.1016/j.ajpath.2017.01.014.
- Xiao F, Wang LJ, Zhao H, Tan C, Wang DN, Zhang H, Wei YG, Liu J, Zhang W. Intermedin restricts vessel sprouting by inhibiting the loosening of endothelial junction. Biochem Biophys Res Commun. 2015;458(1):174–9. doi:10.1016/j.bbrc.2015.01.090.
- Ashizuka S, Inagaki-Ohara K, Kuwasako K, Kato J, Inatsu H, Kitamura K. Adrenomedullin treatment reduces intestinal inflammation and maintains epithelial barrier function in mice administered dextran sulphate sodium. Microbiol Immunol. 2009;53(10):573–81. doi:10.1111/j.1348-0421.2009.00159.x.
- Yi Z, Fan H, Liu X, Tang Q, Zuo D, Yang J. Adrenomedullin improves intestinal epithelial barrier function by downregulating myosin light chain phosphorylation in ulcerative colitis rats. Mol Med Reports. 2015;12(3):3615–20. doi:10.3892/mmr.2015.3887.
- Temmesfeld-Wollbruck B, Brell B, zu Dohna C, Dorenberg M, Hocke AC, Martens H, Klar J, Suttorp N, Hippenstiel S. Adrenomedullin reduces intestinal epithelial permeability in vivo and in vitro. Am J Physiol Gastrointestinal Liver Physiol. 2009;297(1):G43–51. doi:10.1152/ajpgi.90532.2008.
- Basivireddy J, Somvanshi RK, Romero IA, Weksler BB, Couraud PO, Oger J, Kumar U. Somatostatin preserved blood brain barrier against cytokine induced alterations: possible role in multiple sclerosis. Biochem Pharmacol. 2013;86(4):497–507. doi:10.1016/j.bcp.2013.06.001.
- Lei S, Cheng T, Guo Y, Li C, Zhang W, Zhi F. Somatostatin ameliorates lipopolysaccharide-induced tight junction damage via the ERK-MAPK pathway in Caco2 cells. Eur J Cell Biol. 2014;93(7):299–307. doi:10.1016/j.ejcb.2014.05.003.
- Liew CW, Vockel M, Glassmeier G, Brandner JM, Fernandez-Ballester GJ, Schwarz JR, Schulz S, Buck F, Serrano L, Richter D, et al. Interaction of the human somatostatin receptor 3 with the multiple PDZ domain protein MUPP1 enables somatostatin to control permeability of epithelial tight junctions. FEBS Letters. 2009;583(1):49–54. doi:10.1016/j.febslet.2008.11.048.
- Vockel M, Breitenbach U, Kreienkamp HJ, Brandner JM. Somatostatin regulates tight junction function and composition in human keratinocytes. Exp Dermatol. 2010;19(10):888–94. doi:10.1111/j.1600-0625.2010.01101.x.
- Van Itallie C, Rahner C, Anderson JM. Regulated expression of claudin-4 decreases paracellular conductance through a selective decrease in sodium permeability. J Clin Invest. 2001;107(10):1319–27. doi:10.1172/JCI12464.
- Fukuda S, Nakagawa S, Tatsumi R, Morofuji Y, Takeshita T, Hayashi K, Tanaka K, Matsuo T, Niwa M. Glucagon-Like Peptide-1 strengthens the barrier integrity in primary cultures of rat brain endothelial cells under basal and hyperglycemia conditions. J Mol Neurosci. 2016;59(2):211–9. doi:10.1007/s12031-015-0696-1.
- Fan Y, Liu K, Wang Q, Ruan Y, Ye W, Zhang Y. Exendin-4 alleviates retinal vascular leakage by protecting the blood-retinal barrier and reducing retinal vascular permeability in diabetic Goto-Kakizaki rats. Exp Eye Res. 2014;127:104–16. doi:10.1016/j.exer.2014.05.004.
- Rossi S, Maisto R, Gesualdo C, Trotta MC, Ferraraccio F, Kaneva MK, Getting SJ, Surace E, Testa F, Simonelli F, et al. Activation of melanocortin receptors MC 1 and MC 5 attenuates retinal damage in experimental diabetic retinopathy. Mediators Inflammation. 2016;2016:7368389. doi:10.1155/2016/7368389.
- Manna SK, Aggarwal BB. Alpha-melanocyte-stimulating hormone inhibits the nuclear transcription factor NF-kappa B activation induced by various inflammatory agents. J Immunol. 1998;161(6):2873–80.
- Iizuka Y, Okuno T, Saeki K, Uozaki H, Okada S, Misaka T, Sato T, Toh H, Fukayama M, Takeda N, et al. Protective role of the leukotriene B4 receptor BLT2 in murine inflammatory colitis. FASEB J Official Publication Federation Am Soc Exp Biol. 2010;24(12):4678–90. doi:10.1096/fj.10-165050.
- Ishii Y, Saeki K, Liu M, Sasaki F, Koga T, Kitajima K, Meno C, Okuno T, Yokomizo T. Leukotriene B4 receptor type 2 (BLT2) enhances skin barrier function by regulating tight junction proteins. FASEB J Official Publication Federation Am Soc Exp Biol. 2016;30(2):933–47. doi:10.1096/fj.15-279653.
- Chiba T, Nakahara T, Hashimoto-Hachiya A, Yokomizo T, Uchi H, Furue M. The leukotriene B4 receptor BLT2 protects barrier function via actin polymerization with phosphorylation of myosin phosphatase target subunit 1 in human keratinocytes. Exp Dermatol. 2016;25(7):532–6. doi:10.1111/exd.12976.
- Grumbach Y, Quynh NV, Chiron R, Urbach V. LXA4 stimulates ZO-1 expression and transepithelial electrical resistance in human airway epithelial (16HBE14o-) cells. Am J Physiol Lung Cell Mol Physiol. 2009;296(1):L101–8. doi:10.1152/ajplung.00018.2008.
- Higgins G, Fustero Torre C, Tyrrell J, McNally P, Harvey BJ, Urbach V. Lipoxin A4 prevents tight junction disruption and delays the colonization of cystic fibrosis bronchial epithelial cells by Pseudomonas aeruginosa. Am J Physiol Lung Cell Mol Physiol. 2016;310(11):L1053–61. doi:10.1152/ajplung.00368.2015.
- Lu TS, Avraham HK, Seng S, Tachado SD, Koziel H, Makriyannis A, Avraham S. Cannabinoids inhibit HIV-1 Gp120-mediated insults in brain microvascular endothelial cells. J Immunol. 2008;181(9):6406–16. doi:10.4049/jimmunol.181.9.6406.
- Yang MC, Zhang HZ, Wang Z, You FL, Wang YF. The molecular mechanism and effect of cannabinoid-2 receptor agonist on the blood-spinal cord barrier permeability induced by ischemia-reperfusion injury. Brain Res. 2016;1636:81–92. doi:10.1016/j.brainres.2016.01.047.
- Ramirez SH, Hasko J, Skuba A, Fan S, Dykstra H, McCormick R, Reichenbach N, Krizbai I, Mahadevan A, Zhang M, et al. Activation of cannabinoid receptor 2 attenuates leukocyte-endothelial cell interactions and blood-brain barrier dysfunction under inflammatory conditions. J Neurosci Official J Soc Neurosci. 2012;32(12):4004–16. doi:10.1523/JNEUROSCI.4628-11.2012.
- Fujii M, Sherchan P, Krafft PR, Rolland WB, Soejima Y, Zhang JH. Cannabinoid type 2 receptor stimulation attenuates brain edema by reducing cerebral leukocyte infiltration following subarachnoid hemorrhage in rats. J Neurol Sci. 2014;342(1-2):101–6. doi:10.1016/j.jns.2014.04.034.
- Feng YJ, Li YY, Lin XH, Li K, Cao MH. Anti-inflammatory effect of cannabinoid agonist WIN55, 212 on mouse experimental colitis is related to inhibition of p38MAPK. World J Gastroenterol. 2016;22(43):9515–24. doi:10.3748/wjg.v22.i43.9515.
- Alhamoruni A, Lee AC, Wright KL, Larvin M, O'Sullivan SE. Pharmacological effects of cannabinoids on the Caco-2 cell culture model of intestinal permeability. J Pharmacol Exp Therapeutics. 2010;335(1):92–102. doi:10.1124/jpet.110.168237.
- Yang YY, Hsieh SL, Lee PC, Yeh YC, Lee KC, Hsieh YC, Wang YW, Lee TY, Huang YH, Chan CC, et al. Long-term cannabinoid type 2 receptor agonist therapy decreases bacterial translocation in rats with cirrhosis and ascites. J Hepatol. 2014;61(5):1004–13. doi:10.1016/j.jhep.2014.05.049.
- Shang VC, Kendall DA, Roberts RE. Delta9-Tetrahydrocannabinol reverses TNFalpha-induced increase in airway epithelial cell permeability through CB2 receptors. Biochem Pharmacol. 2016;120:63–71. doi:10.1016/j.bcp.2016.09.008.
- Fujii M, Sherchan P, Soejima Y, Doycheva D, Zhao D, Zhang JH. Cannabinoid Receptor Type 2 Agonist Attenuates Acute Neurogenic Pulmonary Edema by Preventing Neutrophil Migration after Subarachnoid Hemorrhage in Rats. Acta Neurochirurgica Supplement. 2016;121:135–9. doi:10.1007/978-3-319-18497-5_24.
- Barutta F, Corbelli A, Mastrocola R, Gambino R, Di Marzo V, Pinach S, Rastaldi MP, Perin PC, Gruden G. Cannabinoid receptor 1 blockade ameliorates albuminuria in experimental diabetic nephropathy. Diabetes. 2010;59(4):1046–54. doi:10.2337/db09-1336.
- Miyamoto J, Mizukure T, Park SB, Kishino S, Kimura I, Hirano K, Bergamo P, Rossi M, Suzuki T, Arita, et al. A gut microbial metabolite of linoleic acid, 10-hydroxy-cis-12-octadecenoic acid, ameliorates intestinal epithelial barrier impairment partially via GPR40-MEK-ERK pathway. J Biol Chem. 2015;290(5):2902–18. doi:10.1074/jbc.M114.610733.
- Finigan JH, Dudek SM, Singleton PA, Chiang ET, Jacobson JR, Camp SM, Ye SQ, Garcia JG. Activated protein C mediates novel lung endothelial barrier enhancement: role of sphingosine 1-phosphate receptor transactivation. J Biol Chem. 2005;280(17):17286–93. doi:10.1074/jbc.M412427200.
- Feistritzer C, Riewald M. Endothelial barrier protection by activated protein C through PAR1-dependent sphingosine 1-phosphate receptor-1 crossactivation. Blood. 2005;105(8):3178–84. doi:10.1182/blood-2004-10-3985.
- Wang L, Bittman R, Garcia JG, Dudek SM. Junctional complex and focal adhesion rearrangement mediates pulmonary endothelial barrier enhancement by FTY720 S-phosphonate. Microvasc Res. 2015;99:102–9. doi:10.1016/j.mvr.2015.03.007.
- Fan L, Yan H. FTY720 attenuates retinal inflammation and protects blood-retinal barrier in diabetic rats. Invest Ophthalmol Visual Sci. 2016;57(3):1254–63. doi:10.1167/iovs.15-18658.
- Altay O, Suzuki H, Hasegawa Y, Caner B, Krafft PR, Fujii M, Tang J, Zhang JH. Isoflurane attenuates blood-brain barrier disruption in ipsilateral hemisphere after subarachnoid hemorrhage in mice. Stroke J Cerebral Circulation. 2012;43(9):2513–6. doi:10.1161/STROKEAHA.112.661728.
- Dong J, Wang H, Zhao J, Sun J, Zhang T, Zuo L, Zhu W, Gong J, Li Y, Gu L, Li J. SEW2871 protects from experimental colitis through reduced epithelial cell apoptosis and improved barrier function in interleukin-10 gene-deficient mice. Immunol Res. 2015;61(3):303–11. doi:10.1007/s12026-015-8625-5.
- Li Q, Chen B, Zeng C, Fan A, Yuan Y, Guo X, Huang X, Huang Q. Differential activation of receptors and signal pathways upon stimulation by different doses of sphingosine-1-phosphate in endothelial cells. Exp Physiol. 2015;100(1):95–107. doi:10.1113/expphysiol.2014.082149.
- Chen T, Huang Z, Liu R, Yang J, Hylemon PB, Zhou H. Sphingosine-1 phosphate promotes intestinal epithelial cell proliferation via S1PR2. Front Biosci (Landmark Ed). 2017;22:596–608. doi:10.2741/4505.
- Rosales MA, Silva KC, Duarte DA, Rossato FA, Lopes de Faria JB, Lopes de Faria JM. Endocytosis of tight junctions caveolin nitrosylation dependent is improved by cocoa via opioid receptor on RPE cells in diabetic conditions. Invest Ophthalmol Visual Sci. 2014;55(9):6090–100. doi:10.1167/iovs.14-14234.
- Patel N, Itakura T, Gonzalez JM, Jr, Schwartz SG, Fini ME. GPR158, an orphan member of G protein-coupled receptor Family C: glucocorticoid-stimulated expression and novel nuclear role. PloS one. 2013;8(2):e57843. doi:10.1371/journal.pone.0057843.
- Kiatsurayanon C, Niyonsaba F, Smithrithee R, Akiyama T, Ushio H, Hara M, Okumura K, Ikeda S, Ogawa H. Host defense (Antimicrobial) peptide, human beta-defensin-3, improves the function of the epithelial tight-junction barrier in human keratinocytes. J Invest Dermatol. 2014;134(8):2163–73. doi:10.1038/jid.2014.143.
- Chen Y, Zhang Y, Tang J, Liu F, Hu Q, Luo C, Tang J, Feng H, Zhang JH. Norrin protected blood-brain barrier via frizzled-4/beta-catenin pathway after subarachnoid hemorrhage in rats. Stroke J Cerebral Circulation. 2015;46(2):529–36. doi:10.1161/STROKEAHA.114.007265.
- Wang Y, Rattner A, Zhou Y, Williams J, Smallwood PM, Nathans J. Norrin/Frizzled4 signaling in retinal vascular development and blood brain barrier plasticity. Cell. 2012;151(6):1332–44. doi:10.1016/j.cell.2012.10.042.
- Ye X, Wang Y, Cahill H, Yu M, Badea TC, Smallwood PM, Peachey NS, Nathans J. Norrin, frizzled-4, and Lrp5 signaling in endothelial cells controls a genetic program for retinal vascularization. Cell. 2009;139(2):285–98. doi:10.1016/j.cell.2009.07.047.
- Paes KT, Wang E, Henze K, Vogel P, Read R, Suwanichkul A, Kirkpatrick LL, Potter D, Newhouse MM, Rice DS. Frizzled 4 is required for retinal angiogenesis and maintenance of the blood-retina barrier. Invest Ophthalmol Visual Sci. 2011;52(9):6452–61. doi:10.1167/iovs.10-7146.
- Campbell M, Humphries M, Kennan A, Kenna P, Humphries P, Brankin B. Aberrant retinal tight junction and adherens junction protein expression in an animal model of autosomal dominant Retinitis pigmentosa: the Rho(-/-) mouse. Exp Eye Res. 2006;83(3):484–92. doi:10.1016/j.exer.2006.01.032.
- Omri S, Omri B, Savoldelli M, Jonet L, Thillaye-Goldenberg B, Thuret G, Gain P, Jeanny JC, Crisanti P, Behar-Cohen F. The outer limiting membrane (OLM) revisited: clinical implications. Clin Ophthalmol. 2010;4:183–95.
- Shah GV, Thomas S, Muralidharan A, Liu Y, Hermonat PL, Williams J, Chaudhary J. Calcitonin promotes in vivo metastasis of prostate cancer cells by altering cell signaling, adhesion, and inflammatory pathways. Endocr Relat Cancer. 2008;15(4):953–64. doi:10.1677/ERC-08-0136.
- Shah GV, Muralidharan A, Gokulgandhi M, Soan K, Thomas S. Cadherin switching and activation of beta-catenin signaling underlie proinvasive actions of calcitonin-calcitonin receptor axis in prostate cancer. J Biol Chem. 2009;284(2):1018–30. doi:10.1074/jbc.M807823200.
- Aljameeli A, Thakkar A, Thomas S, Lakshmikanthan V, Iczkowski KA, Shah GV. Calcitonin Receptor-Zonula Occludens-1 Interaction Is Critical for Calcitonin-Stimulated Prostate Cancer Metastasis. PloS One. 2016;11(3):e0150090. doi:10.1371/journal.pone.0150090.
- Aljameeli A, Thakkar A, Shah G. Calcitonin receptor increases invasion of prostate cancer cells by recruiting zonula occludens-1 and promoting PKA-mediated TJ disassembly. Cell Signal. 2017;36:1–13. doi:10.1016/j.cellsig.2017.04.008.
- Wu L, Oshima T, Tomita T, Ohda Y, Fukui H, Watari J, Miwa H. Serotonin disrupts esophageal mucosal integrity: an investigation using a stratified squamous epithelial model. J Gastroenterol. 2016;51(11):1040–9. doi:10.1007/s00535-016-1195-z.
- Stull MA, Pai V, Vomachka AJ, Marshall AM, Jacob GA, Horseman ND. Mammary gland homeostasis employs serotonergic regulation of epithelial tight junctions. Proc Natl Acad Sci U S A. 2007;104(42):16708–13. doi:10.1073/pnas.0708136104.
- Pai VP, Horseman ND. Biphasic regulation of mammary epithelial resistance by serotonin through activation of multiple pathways. J Biol Chem. 2008;283(45):30901–10. doi:10.1074/jbc.M802476200.
- Cong X, Zhang Y, Li J, Mei M, Ding C, Xiang RL, Zhang LW, Wang Y, Wu LL, Yu GY. Claudin-4 is required for modulation of paracellular permeability by muscarinic acetylcholine receptor in epithelial cells. J Cell Sci. 2015;128(12):2271–86. doi:10.1242/jcs.165878.
- Takeuchi K, Kishioka C, Ishinaga H, Sakakura Y, Majima Y. Histamine alters gene expression in cultured human nasal epithelial cells. J Allergy Clin Immunol. 2001;107(2):310–4. doi:10.1067/mai.2001.112127.
- Canonica GW, Blaiss M. Antihistaminic, anti-inflammatory, and antiallergic properties of the nonsedating second-generation antihistamine desloratadine: a review of the evidence. World Allergy Organ J. 2011;4(2):47–53. doi:10.1097/WOX.0b013e3182093e19.
- Hirase T, Kawashima S, Wong EY, Ueyama T, Rikitake Y, Tsukita S, Yokoyama M, Staddon JM. Regulation of tight junction permeability and occludin phosphorylation by Rhoa-p160ROCK-dependent and -independent mechanisms. J Biol Chem. 2001;276(13):10423–31. doi:10.1074/jbc.M007136200.
- Breslin JW. ROCK and cAMP promote lymphatic endothelial cell barrier integrity and modulate histamine and thrombin-induced barrier dysfunction. Lymphatic Res Biol. 2011;9(1):3–11. doi:10.1089/lrb.2010.0016.
- Sato M, Sasaki N, Ato M, Hirakawa S, Sato K, Sato K. Microcirculation-on-a-Chip: A Microfluidic Platform for Assaying Blood- and Lymphatic-Vessel Permeability. PloS one. 2015;10(9):e0137301. doi:10.1371/journal.pone.0137301.
- Wang Z, Cai XJ, Qin J, Xie FJ, Han N, Lu HY. The role of histamine in opening blood-tumor barrier. Oncotarget. 2016;7(21):31299–310. doi:10.18632/oncotarget.8896.
- Guo M, Breslin JW, Wu MH, Gottardi CJ, Yuan SY. VE-cadherin and beta-catenin binding dynamics during histamine-induced endothelial hyperpermeability. Am J Physiol Cell Physiol. 2008;294(4):C977–84. doi:10.1152/ajpcell.90607.2007.
- Ratcliffe MJ, Smales C, Staddon JM. Dephosphorylation of the catenins p120 and p100 in endothelial cells in response to inflammatory stimuli. Biochem J. 1999;338 (Pt 2):471–8. doi:10.1042/bj3380471.
- Shasby DM, Ries DR, Shasby SS, Winter MC. Histamine stimulates phosphorylation of adherens junction proteins and alters their link to vimentin. Am J Physiol Lung Cell Mol Physiol 2002;282(6):L1330–8. doi:10.1152/ajplung.00329.2001.
- Lennon FE, Mirzapoiazova T, Mambetsariev B, Poroyko VA, Salgia R, Moss J, Singleton PA. The Mu opioid receptor promotes opioid and growth factor-induced proliferation, migration and Epithelial Mesenchymal Transition (EMT) in human lung cancer. PloS One. 2014;9(3):e91577. doi:10.1371/journal.pone.0091577.
- Biki B, Mascha E, Moriarty DC, Fitzpatrick JM, Sessler DI, Buggy DJ. Anesthetic technique for radical prostatectomy surgery affects cancer recurrence: a retrospective analysis. Anesthesiology. 2008;109(2):180–7. doi:10.1097/ALN.0b013e31817f5b73.
- Christopherson R, James KE, Tableman M, Marshall P, Johnson FE. Long-term survival after colon cancer surgery: a variation associated with choice of anesthesia. Anesthesia Analgesia. 2008;107(1):325–32. doi:10.1213/ane.0b013e3181770f55.
- Zhang K, Tian L, Liu L, Feng Y, Dong YB, Li B, Shang DS, Fang WG, Cao YP, Chen YH. CXCL1 contributes to beta-amyloid-induced transendothelial migration of monocytes in Alzheimer's disease. PloS one. 2013;8(8):e72744. doi:10.1371/journal.pone.0072744.
- Huang J, Li Y, Tang Y, Tang G, Yang GY, Wang Y. CXCR4 antagonist AMD3100 protects blood-brain barrier integrity and reduces inflammatory response after focal ischemia in mice. Stroke J Cerebral Circulation. 2013;44(1):190–7. doi:10.1161/STROKEAHA.112.670299.
- Xia XM, Wang FY, Zhou J, Hu KF, Li SW, Zou BB. CXCR4 antagonist AMD3100 modulates claudin expression and intestinal barrier function in experimental colitis. PloS one. 2011;6(11):e27282. doi:10.1371/journal.pone.0027282.
- Lammers KM, Lu R, Brownley J, Lu B, Gerard C, Thomas K, Rallabhandi P, Shea-Donohue T, Tamiz A, Alkan S, et al. Gliadin induces an increase in intestinal permeability and zonulin release by binding to the chemokine receptor CXCR3. Gastroenterology. 2008;135(1):194–204 e3. doi:10.1053/j.gastro.2008.03.023.
- Stamatovic SM, Shakui P, Keep RF, Moore BB, Kunkel SL, Van Rooijen N Andjelkovic AV. Monocyte chemoattractant protein-1 regulation of blood-brain barrier permeability. J Cereb Blood Flow Metab. 2005;25(5):593–606. doi:10.1038/sj.jcbfm.9600055.
- Stamatovic SM, Keep RF, Kunkel SL, Andjelkovic AV. Potential role of MCP-1 in endothelial cell tight junction 'opening': Signaling via Rho and Rho kinase. Journal of cell science. 2003;116(Pt 22):4615–28. doi:10.1242/jcs.00755.
- Dimitrijevic OB, Stamatovic SM, Keep RF, Andjelkovic AV. Effects of the chemokine CCL2 on blood-brain barrier permeability during ischemia-reperfusion injury. J Cereb Blood Flow Metab. 2006;26(6):797–810. doi:10.1038/sj.jcbfm.9600229.
- Jamaluddin MS, Wang X, Wang H, Rafael C, Yao Q, Chen C. Eotaxin increases monolayer permeability of human coronary artery endothelial cells. Arterioscler Thromb Vasc Biol. 2009;29(12):2146–52. doi:10.1161/ATVBAHA.109.194134.
- Mahajan SD, Parikh NU, Woodruff TM, Jarvis JN, Lopez M, Hennon T, Cunningham P, Quigg RJ, Schwartz SA, Alexander JJ. C5a alters blood-brain barrier integrity in a human in vitro model of systemic lupus erythematosus. Immunology. 2015;146(1):130–43. doi:10.1111/imm.12489.
- Jacob A, Hack B, Chen P, Quigg RJ, Alexander JJ. C5a/CD88 signaling alters blood-brain barrier integrity in lupus through nuclear factor-kappaB. Journal of neurochemistry. 2011;119(5):1041–51. doi:10.1111/j.1471-4159.2011.07490.x.
- Hu WH, Hu Z, Shen X, Dong LY, Zhou WZ, Yu XX. C5a receptor enhances hepatocellular carcinoma cell invasiveness via activating ERK1/2-mediated epithelial-mesenchymal transition. Exp Mol Pathol. 2016;100(1):101–8. doi:10.1016/j.yexmp.2015.10.001.
- Kim JY, Ko AR, Hyun HW, Kang TC. ETB receptor-mediated MMP-9 activation induces vasogenic edema via ZO-1 protein degradation following status epilepticus. Neuroscience. 2015;304:355–67. doi:10.1016/j.neuroscience.2015.07.065.
- Leung JW, Chung SS, Chung SK. Endothelial endothelin-1 over-expression using receptor tyrosine kinase tie-1 promoter leads to more severe vascular permeability and blood brain barrier breakdown after transient middle cerebral artery occlusion. Brain research. 2009;1266:121–9. doi:10.1016/j.brainres.2009.01.070.
- Gene Family. G protein-coupled receptors, Class F frizzled (FZD). Available from: www.genenames.org/cgi-bin/genefamilies/set/286.
- Li VS, Ng SS, Boersema PJ, Low TY, Karthaus WR, Gerlach JP, Mohammed S, Heck AJ, Maurice MM, Mahmoudi T, et al. Wnt signaling through inhibition of beta-catenin degradation in an intact Axin1 complex. Cell. 2012;149(6):1245–56. Epub 2012/06/12. doi:10.1016/j.cell.2012.05.002.
- Clevers H, Nusse R. Wnt/beta-catenin signaling and disease. Cell. 2012;149(6):1192–205. doi:10.1016/j.cell.2012.05.012.
- Ikenouchi J, Matsuda M, Furuse M, Tsukita S. Regulation of tight junctions during the epithelium-mesenchyme transition: Direct repression of the gene expression of claudins/occludin by Snail. Journal of cell science. 2003;116(Pt 10):1959–67. doi:10.1242/jcs.00389.
- Zhou BP, Deng J, Xia W, Xu J, Li YM, Gunduz M, Hung MC. Dual regulation of Snail by GSK-3beta-mediated phosphorylation in control of epithelial-mesenchymal transition. Nat Cell Biol. 2004;6(10):931–40. doi:10.1038/ncb1173.
- Yook JI, Li XY, Ota I, Fearon ER, Weiss SJ. Wnt-dependent regulation of the E-cadherin repressor snail. Journal of biological chemistry. 2005;280(12):11740–8. doi:10.1074/jbc.M413878200.
- Jho EH, Zhang T, Domon C, Joo CK, Freund JN, Costantini F. Wnt/beta-catenin/Tcf signaling induces the transcription of Axin2, a negative regulator of the signaling pathway. Molecular and cellular biology. 2002;22(4):1172–83. doi:10.1128/MCB.22.4.1172-1183.2002.
- Yook JI, Li XY, Ota I, Hu C, Kim HS, Kim NH, Fearon ER, Weiss SJ. A Wnt-Axin2-GSK3beta cascade regulates Snail1 activity in breast cancer cells. Nat Cell Biol. 2006;8(12):1398–406. doi:10.1038/ncb1508.
- Lu J, Zhang S, Nakano H, Simmons DG, Wang S, Kong S, Wang Q, Shen L, Tu Z, Wang W, et al. A positive feedback loop involving Gcm1 and Fzd5 directs chorionic branching morphogenesis in the placenta. PLoS biology. 2013;11(4):e1001536. doi:10.1371/journal.pbio.1001536.
- Knezevic II, Predescu SA, Neamu RF, Gorovoy MS, Knezevic NM, Easington C, Malik AB, Predescu DN. Tiam1 and Rac1 are required for platelet-activating factor-induced endothelial junctional disassembly and increase in vascular permeability. Journal of biological chemistry. 2009;284(8):5381–94. doi:10.1074/jbc.M808958200.
- Adamson RH, Zeng M, Adamson GN, Lenz JF, Curry FE. PAF- and bradykinin-induced hyperpermeability of rat venules is independent of actin-myosin contraction. Am J Physiol Heart Circ Physiol. 2003;285(1):H406–17. doi:10.1152/ajpheart.00021.2003.
- Jeewandara C, Gomes L, Wickramasinghe N, Gutowska-Owsiak D, Waithe D, Paranavitane SA, Shyamali NL, Ogg GS, Malavige GN. Platelet activating factor contributes to vascular leak in acute dengue infection. PLoS Negl Trop Dis. 2015;9(2):e0003459. doi:10.1371/journal.pntd.0003459.
- Travis SP, Jewell DP. Regional differences in the response to platelet-activating factor in rabbit colon. Clin Sci (Lond). 1992;82(6):673–80. doi:10.1042/cs0820673.
- Abu-Farsakh S, Wu T, Lalonde A, Sun J, Zhou Z. High expression of Claudin-2 in esophageal carcinoma and precancerous lesions is significantly associated with the bile salt receptors VDR and TGR5. BMC Gastroenterol. 2017;17(1):33. doi:10.1186/s12876-017-0590-0.
- Aung PP, Mitani Y, Sanada Y, Nakayama H, Matsusaki K, Yasui W. Differential expression of claudin-2 in normal human tissues and gastrointestinal carcinomas. Virchows Arch. 2006;448(4):428–34. Epub 2005/12/06. doi:10.1007/s00428-005-0120-2.
- Gyorffy H. [Study of claudins and prognostic factors in some gastrointestinal diseases]. Magyar onkologia. 2009;53(4):377–83. Epub 2010/01/15.
- Gyorffy H, Holczbauer A, Nagy P, Szabo Z, Kupcsulik P, Paska C, Papp J, Schaff Z, Kiss A. Claudin expression in Barrett's esophagus and adenocarcinoma. Virchows Arch. 2005;447(6):961–8. Epub 2005/09/01. doi:10.1007/s00428-005-0045-9.
- Weber CR, Nalle SC, Tretiakova M, Rubin DT, Turner JR. Claudin-1 and claudin-2 expression is elevated in inflammatory bowel disease and may contribute to early neoplastic transformation. Lab Invest. 2008;88(10):1110–20. Epub 2008/08/20. doi:10.1038/labinvest.2008.78.
- Ulbricht TLV. Purines, Pyrimidines and Nucleotides and the Chemistry of Nucleic Acids. NEW YORK: THE MACMILLAN COMPANY;1964.
- Li WH, Qiu Y, Zhang HQ, Liu Y, You JF, Tian XX, Fang WG. P2Y2 receptor promotes cell invasion and metastasis in prostate cancer cells. Br J Cancer. 2013;109(6):1666–75. doi:10.1038/bjc.2013.484.
- Loma P, Guzman-Aranguez A, Perez de Lara MJ, Pintor J. Diadenosine tetraphosphate induces tight junction disassembly thus increasing corneal epithelial permeability. British journal of pharmacology. 2015;172(4):1045–58. doi:10.1111/bph.12972.
- Carman AJ, Mills JH, Krenz A, Kim DG, Bynoe MS. Adenosine receptor signaling modulates permeability of the blood-brain barrier. J Neurosci. 2011;31(37):13272–80. doi:10.1523/JNEUROSCI.3337-11.2011.
- Kim DG, Bynoe MS. A2A Adenosine Receptor Regulates the Human Blood-Brain Barrier Permeability. Mol Neurobiol. 2015;52(1):664–78. doi:10.1007/s12035-014-8879-2.
- Gao X, Qian J, Zheng S, Changyi Y, Zhang J, Ju S, Zhu J, Li C. Overcoming the blood-brain barrier for delivering drugs into the brain by using adenosine receptor nanoagonist. ACS nano. 2014;8(4):3678–89. doi:10.1021/nn5003375.
- Yang Y, Qiu Y, Wang W, Xiao W, Liang H, Zhang C, Yang H, Teitelbaum DH, Sun LH, Yang H. Adenosine A2B receptor modulates intestinal barrier function under hypoxic and ischemia/reperfusion conditions. Int J Clin Exp Pathol. 2014;7(5):2006–18.
- Schenck LP, Hirota SA, Hirota CL, Boasquevisque P, Tulk SE, Li Y, Wadhwani A, Doktorchik CT, Macnaughton WK, Beck PL, et al. Attenuation of Clostridium difficile toxin-induced damage to epithelial barrier by ecto-5'-nucleotidase (CD73) and adenosine receptor signaling. Neurogastroenterol Motil. 2013;25(6):e441–53. doi:10.1111/nmo.12139.
- Groschwitz KR, Wu D, Osterfeld H, Ahrens R, Hogan SP. Chymase-mediated intestinal epithelial permeability is regulated by a protease-activating receptor/matrix metalloproteinase-2-dependent mechanism. Am J Physiol Gastrointest Liver Physiol. 2013;304(5):G479–89. doi:10.1152/ajpgi.00186.2012.
- Cenac N, Coelho AM, Nguyen C, Compton S, Andrade-Gordon P, MacNaughton WK, Wallace JL, Hollenberg MD, Bunnett NW, Garcia-Villar R, et al. Induction of intestinal inflammation in mouse by activation of proteinase-activated receptor-2. American journal of pathology. 2002;161(5):1903–15. doi:10.1016/S0002-9440(10)64466-5.
- Jacob C, Yang PC, Darmoul D, Amadesi S, Saito T, Cottrell GS, Coelho AM, Singh P, Grady EF, Perdue M, et al. Mast cell tryptase controls paracellular permeability of the intestine. J Biol Chem. 2005;280(36):31936–48. doi:10.1074/jbc.M506338200.
- Bustos D, Negri G, De Paula JA Di Carlo M, Yapur V, Facente A, De Paula A. Colonic proteinases: Increased activity in patients with ulcerative colitis. Medicina (B Aires). 1998;58(3):262–4.
- Raithel M, Winterkamp S, Pacurar A, Ulrich P, Hochberger J, Hahn EG. Release of mast cell tryptase from human colorectal mucosa in inflammatory bowel disease. Scand J Gastroenterol. 2001;36(2):174–9. doi:10.1080/003655201750065933.
- Gecse K, Roka R, Ferrier L, Leveque M, Eutamene H, Cartier C, Ait-Belgnaoui A, Rosztóczy A, Izbéki F, Fioramonti J, et al. Increased faecal serine protease activity in diarrhoeic IBS patients: a colonic lumenal factor impairing colonic permeability and sensitivity. Gut. 2008;57(5):591–9. doi:10.1136/gut.2007.140210.
- Cenac N, Chin AC, Garcia-Villar R, Salvador-Cartier C, Ferrier L, Vergnolle N, Buret AG, Fioramonti J, Bueno L. PAR2 activation alters colonic paracellular permeability in mice via IFN-gamma-dependent and -independent pathways. J Physiol. 2004;558(Pt 3):913–25. doi:10.1113/jphysiol.2004.061721.
- Goldblum SE, Rai U, Tripathi A, Thakar M, De Leo L Di Toro N, Not T, Ramachandran R, Puche AC, Hollenberg MD, et al. The active Zot domain (aa 288-293) increases ZO-1 and myosin 1C serine/threonine phosphorylation, alters interaction between ZO-1 and its binding partners, and induces tight junction disassembly through proteinase activated receptor 2 activation. FASEB J. 2011;25(1):144–58. doi:10.1096/fj.10-158972.
- Cenac N, Garcia-Villar R, Ferrier L, Larauche M, Vergnolle N, Bunnett NW, Coelho AM, Fioramonti J, Bueno L. Proteinase-activated receptor-2-induced colonic inflammation in mice: Possible involvement of afferent neurons, nitric oxide, and paracellular permeability. J Immunol. 2003;170(8):4296–300. doi:10.4049/jimmunol.170.8.4296.
- Zhong CJ, Wang K, Zhang L, Yang CQ, Zhang K, Zhou SP, Duan LP. Mast cell activation is involved in stress-induced epithelial barrier dysfunction in the esophagus. J Dig Dis. 2015;16(4):186–96. doi:10.1111/1751-2980.12226.
- Enjoji S, Ohama T, Sato K. Regulation of epithelial cell tight junctions by protease-activated receptor 2. J Vet Med Sci. 2014;76(9):1225–9. doi:10.1292/jvms.14-0191.
- Szabo R, Peters DE, Kosa P, Camerer E, Bugge TH. Regulation of feto-maternal barrier by matriptase- and PAR-2-mediated signaling is required for placental morphogenesis and mouse embryonic survival. PLoS Genet. 2014;10(7):e1004470. doi:10.1371/journal.pgen.1004470.
- Cirino G, Severino B. Thrombin receptors and their antagonists: an update on the patent literature. Expert Opin Ther Pat. 2010;20(7):875–84. doi:10.1517/13543776.2010.487864.
- McLaughlin JN, Shen L, Holinstat M, Brooks JD, Dibenedetto E, Hamm HE. Functional selectivity of G protein signaling by agonist peptides and thrombin for the protease-activated receptor-1. Journal of biological chemistry. 2005;280(26):25048–59. doi:10.1074/jbc.M414090200.
- Xue M, Hollenberg MD, Demchuk A, Yong VW. Relative importance of proteinase-activated receptor-1 versus matrix metalloproteinases in intracerebral hemorrhage-mediated neurotoxicity in mice. Stroke. 2009;40(6):2199–204. doi:10.1161/STROKEAHA.108.540393.
- Cheng Y, Xi G, Jin H, Keep RF, Feng J, Hua Y. Thrombin-induced cerebral hemorrhage: role of protease-activated receptor-1. Transl Stroke Res. 2014;5(4):472–5. doi:10.1007/s12975-013-0288-8.
- Gao D, Ding F, Lei G, Luan G, Zhang S, Li K, Wang D, Zhang L, Dai D. Effects of focal mild hypothermia on thrombin-induced brain edema formation and the expression of protease activated receptor-1, matrix metalloproteinase-9 and aquaporin 4 in rats. Molecular medicine reports. 2015;11(4):3009–14. doi:10.3892/mmr.2014.3111.
- Kim HN, Kim YR, Ahn SM, Lee SK, Shin HK, Choi BT. Protease activated receptor-1 antagonist ameliorates the clinical symptoms of experimental autoimmune encephalomyelitis via inhibiting breakdown of blood-brain barrier. Journal of neurochemistry. 2015;135(3):577–88. doi:10.1111/jnc.13285.
- Riewald M, Petrovan RJ, Donner A, Mueller BM, Ruf W. Activation of endothelial cell protease activated receptor 1 by the protein C pathway. Science. 2002;296(5574):1880–2. doi:10.1126/science.1071699.
- Soh UJ, Trejo J. Activated protein C promotes protease-activated receptor-1 cytoprotective signaling through beta-arrestin and dishevelled-2 scaffolds. Proc Natl Acad Sci U S A. 2011;108(50):E1372–80. doi:10.1073/pnas.1112482108.
- Mosnier LO, Sinha RK, Burnier L, Bouwens EA, Griffin JH. Biased agonism of protease-activated receptor 1 by activated protein C caused by noncanonical cleavage at Arg46. Blood. 2012;120(26):5237–46. doi:10.1182/blood-2012-08-452169.
- Stavenuiter F, Mosnier LO. Noncanonical PAR3 activation by factor Xa identifies a novel pathway for Tie2 activation and stabilization of vascular integrity. Blood. 2014;124(23):3480–9. doi:10.1182/blood-2014-06-582775.
- Minhas N, Xue M, Fukudome K, Jackson CJ. Activated protein C utilizes the angiopoietin/Tie2 axis to promote endothelial barrier function. FASEB J. 2010;24(3):873–81. doi:10.1096/fj.09-134445.
- Bernard GR, Vincent JL, Laterre PF, LaRosa SP, Dhainaut JF, Lopez-Rodriguez A, Steingrub JS, Garber GE, Helterbrand JD, Ely EW, et al. Efficacy and safety of recombinant human activated protein C for severe sepsis. New England journal of medicine. 2001;344(10):699–709. doi:10.1056/NEJM200103083441001.
- Xue M, Chow SO, Dervish S, Chan YK, Julovi SM, Jackson CJ. Activated protein C enhances human keratinocyte barrier integrity via sequential activation of epidermal growth factor receptor and Tie2. Journal of biological chemistry. 2011;286(8):6742–50. doi:10.1074/jbc.M110.181388.
- Fukasawa H, Bornheimer S, Kudlicka K, Farquhar MG. Slit diaphragms contain tight junction proteins. J Am Soc Nephrol. 2009;20(7):1491–503. doi:10.1681/ASN.2008101117.
- Macconi D, Abbate M, Morigi M, Angioletti S, Mister M, Buelli S, Bonomelli M, Mundel P, Endlich K, Remuzzi G, et al. Permselective dysfunction of podocyte-podocyte contact upon angiotensin II unravels the molecular target for renoprotective intervention. American journal of pathology. 2006;168(4):1073–85. doi:10.2353/ajpath.2006.050701.
- Macconi D, Ghilardi M, Bonassi ME, Mohamed EI, Abbate M, Colombi F, Remuzzi G, Remuzzi A. Effect of angiotensin-converting enzyme inhibition on glomerular basement membrane permeability and distribution of zonula occludens-1 in MWF rats. J Am Soc Nephrol. 2000;11(3):477–89.
- Rincon-Choles H, Vasylyeva TL, Pergola PE, Bhandari B, Bhandari K, Zhang JH, Wang W, Gorin Y, Barnes JL, Abboud HE. ZO-1 expression and phosphorylation in diabetic nephropathy. Diabetes. 2006;55(4):894–900. doi:10.2337/diabetes.55.04.06.db05-0355.
- Zhang Z, Zhang Y, Ning G, Deb DK, Kong J, Li YC. Combination therapy with AT1 blocker and vitamin D analog markedly ameliorates diabetic nephropathy: Blockade of compensatory renin increase. Proc Natl Acad Sci U S A. 2008;105(41):15896–901. doi:10.1073/pnas.0803751105.
- Kim JH, Kim JH, Yu YS, Cho CS, Kim KW. Blockade of angiotensin II attenuates VEGF-mediated blood-retinal barrier breakdown in diabetic retinopathy. J Cereb Blood Flow Metab. 2009;29(3):621–8. doi:10.1038/jcbfm.2008.154.
- Fleegal-DeMotta MA, Doghu S, Banks WA. Angiotensin II modulates BBB permeability via activation of the AT(1) receptor in brain endothelial cells. J Cereb Blood Flow Metab. 2009;29(3):640–7. doi:10.1038/jcbfm.2008.158.
- Wosik K, Cayrol R, Dodelet-Devillers A, Berthelet F, Bernard M, Moumdjian R, Bouthillier A, Reudelhuber TL, Prat A. Angiotensin II controls occludin function and is required for blood brain barrier maintenance: relevance to multiple sclerosis. J Neurosci. 2007;27(34):9032–42. doi:10.1523/JNEUROSCI.2088-07.2007.
- Jin L, Chen J, Li L, Li C, Chen C, Li S. CRH suppressed TGFbeta1-induced Epithelial-Mesenchymal Transition via induction of E-cadherin in breast cancer cells. Cellular signalling. 2014;26(4):757–65. doi:10.1016/j.cellsig.2013.12.017.
- Buckley MM, O'Halloran KD, Rae MG, Dinan TG, O'Malley D. Modulation of enteric neurons by interleukin-6 and corticotropin-releasing factor contributes to visceral hypersensitivity and altered colonic motility in a rat model of irritable bowel syndrome. Journal of physiology. 2014;592(23):5235–50. doi:10.1113/jphysiol.2014.279968.
- Zhu H, Liu Y, Chen S, Wang X, Pi D, Leng W, Chen F, Zhang J, Kang P. Fish oil enhances intestinal barrier function and inhibits corticotropin-releasing hormone/corticotropin-releasing hormone receptor 1 signalling pathway in weaned pigs after lipopolysaccharide challenge. British journal of nutrition. 2016;115(11):1947–57. doi:10.1017/S0007114516001100.
- Ulitzky L, Lafer MM, KuKuruga MA, Silberstein E, Cehan N, Taylor DR. A New Signaling Pathway for HCV Inhibition by Estrogen: GPR30 Activation Leads to Cleavage of Occludin by MMP-9. PloS one. 2016;11(1):e0145212. doi:10.1371/journal.pone.0145212.
- Tian PC, Wang HL, Chen GH, Luo Q, Chen Z, Wang Y, Liu YF. 2,2',4,4'-Tetrabromodiphenyl ether promotes human neuroblastoma SH-SY5Y cells migration via the GPER/PI3K/Akt signal pathway. Hum Exp Toxicol. 2016;35(2):124–34. doi:10.1177/0960327115578974.
- Lu D, Qu Y, Shi F, Feng D, Tao K, Gao G, He S, Zhao T. Activation of G protein-coupled estrogen receptor 1 (GPER-1) ameliorates blood-brain barrier permeability after global cerebral ischemia in ovariectomized rats. Biochem Biophys Res Commun. 2016;477(2):209–14. doi:10.1016/j.bbrc.2016.06.044.
- Martin-Venegas R, Roig-Perez S, Ferrer R, Moreno JJ. Arachidonic acid cascade and epithelial barrier function during Caco-2 cell differentiation. Journal of lipid research. 2006;47(7):1416–23. doi:10.1194/jlr.M500564-JLR200.
- Rodriguez-Lagunas MJ, Martin-Venegas R, Moreno JJ, Ferrer R. PGE2 promotes Ca2+-mediated epithelial barrier disruption through EP1 and EP4 receptors in Caco-2 cell monolayers. American journal of physiology Cell physiology. 2010;299(2):C324–34. doi:10.1152/ajpcell.00397.2009.
- Tanaka MN, Diaz BL, de Souza W, Morgado-Diaz JA. Prostaglandin E2-EP1 and EP2 receptor signaling promotes apical junctional complex disassembly of Caco-2 human colorectal cancer cells. BMC cell biology. 2008;9:63. doi:10.1186/1471-2121-9-63.
- Lejeune M, Moreau F, Chadee K. Loss of EP2 receptor subtype in colonic cells compromise epithelial barrier integrity by altering claudin-4. PloS one. 2014;9(11):e113270. doi:10.1371/journal.pone.0113270.
- Frankowski JC, DeMars KM, Ahmad AS, Hawkins KE, Yang C, Leclerc JL, Doré S, Candelario-Jalil E. Detrimental role of the EP1 prostanoid receptor in blood-brain barrier damage following experimental ischemic stroke. Scientific reports. 2015;5:17956. doi:10.1038/srep17956.
- Elliott PJ, Hayward NJ, Huff MR, Nagle TL, Black KL, Bartus RT. Unlocking the blood-brain barrier: A role for RMP-7 in brain tumor therapy. Experimental neurology. 1996;141(2):214–24. doi:10.1006/exnr.1996.0156.
- Inamura T, Nomura T, Bartus RT, Black KL. Intracarotid infusion of RMP-7, a bradykinin analog: A method for selective drug delivery to brain tumors. Journal of neurosurgery. 1994;81(5):752–8. doi:10.3171/jns.1994.81.5.0752.
- Barnett FH, Rainov NG, Ikeda K, Schuback DE, Elliott P, Kramm CM, Chase M, Qureshi NH, Harsh G 4th, Chiocca EA, et al. Selective delivery of herpes virus vectors to experimental brain tumors using RMP-7. Cancer gene therapy. 1999;6(1):14–20. doi:10.1038/sj.cgt.7700003.
- Liu LB, Xue YX, Liu YH, Wang YB. Bradykinin increases blood-tumor barrier permeability by down-regulating the expression levels of ZO-1, occludin, and claudin-5 and rearranging actin cytoskeleton. Journal of neuroscience research. 2008;86(5):1153–68. doi:10.1002/jnr.21558.
- Ma T, Xue Y. RhoA-mediated potential regulation of blood-tumor barrier permeability by bradykinin. J Mol Neurosci. 2010;42(1):67–73. doi:10.1007/s12031-010-9345-x.
- Ma T, Liu L, Wang P, Xue Y. Evidence for involvement of ROCK signaling in bradykinin-induced increase in murine blood-tumor barrier permeability. Journal of neuro-oncology. 2012;106(2):291–301. doi:10.1007/s11060-011-0685-3.
- Liu LB, Liu XB, Ma J, Liu YH, Li ZQ, Ma T, Zhao XH, Xi Z, Xue YX. Bradykinin increased the permeability of BTB via NOS/NO/ZONAB-mediating down-regulation of claudin-5 and occludin. Biochem Biophys Res Commun. 2015;464(1):118–25. doi:10.1016/j.bbrc.2015.06.082.
- Marcon R, Claudino RF, Dutra RC, Bento AF, Schmidt EC, Bouzon ZL, Sordi R, Morais RL, Pesquero JB, Calixto JB. Exacerbation of DSS-induced colitis in mice lacking kinin B(1) receptors through compensatory up-regulation of kinin B(2) receptors: The role of tight junctions and intestinal homeostasis. British journal of pharmacology. 2013;168(2):389–402. doi:10.1111/j.1476-5381.2012.02136.x.
- Dey M, Baldys A, Sumter DB, Gooz P, Luttrell LM, Raymond JR, Göoz M. Bradykinin decreases podocyte permeability through ADAM17-dependent epidermal growth factor receptor activation and zonula occludens-1 rearrangement. Journal of pharmacology and experimental therapeutics. 2010;334(3):775–83. doi:10.1124/jpet.110.168054.
- Yu M, He P, Liu Y, He Y, Du Y, Wu M, Zhang G, Yang C, Gao F. Hyaluroan-regulated lymphatic permeability through S1P receptors is crucial for cancer metastasis. Medical Oncol. 2015;32(1):381. doi:10.1007/s12032-014-0381-1.