ABSTRACT
α-Catenins are actin-filament binding proteins and critical subunits of the cadherin-catenin cell-cell adhesive complex. They are found in nominally-defined epithelial (E), neural (N), and testis (T) forms transcribed from three distinct genes. While most of α-catenin research has focused on the developmentally essential founding member, αE-catenin, this review discusses recent studies on αT-catenin (CTNNA3), a developmentally dispensable isoform that is emerging as relevant to cardiac, allergic and neurological diseases.
Introduction
The cadherin-catenin complex is widely viewed as a linchpin of tissue cohesion and organization. This complex contains a transmembrane cadherin extracellular domain that engages an identical cadherin on adjacent cells. The cadherin cytoplasmic domain associates with catenins that either stabilize cell surface cadherins (e.g., p120ctn) or physically links cadherins to the underlying cytoskeleton (e.g., β-catenin and α-catenin to actin filaments; p120ctn to microtubules) to bring about robust intercellular adhesion.Citation1,Citation2 For historical reasons, the most well studied cadherin-catenin complex comprises cadherin and catenins typically found in epithelia across tissue types—an Epithelial-cadherin (E-cadherin), paired with the more ubiquitously expressed p120ctn, β-catenin and “epithelial” α-catenin (αE-catenin, or αE-cat). This “canonical” cadherin-catenin complex, however, belies known gene complexity at each protein position in the cadherin-catenin complex (). Although fundamental paradigms of cell-cell adhesion have been gleaned from this canonical cadherin-catenin complex, expansion of the cadherin-catenin gene family evolved for a reason— enabling cell and tissue specialization of the basic epithelial adhesive paradigm, which favors organismal fitness. In this review, we focus on one of the more recently evolved catenins, αT-catenin (αT-cat), as a means to understand how modest alterations in the cadherin-catenin adhesion system may be relevant to a range of human diseases.
Figure 1. Schematic representation of cadherin-catenin complexes with distinct α-catenin isoforms αE-cat, αN-cat and αT-cat. Note that there is substantial isoform diversity at the other positions in the cadherin-catenin complex, such as ∼19 classical cadherins (i.e., catenin-binding) encompassing both type I and type II forms,Citation91 three p120ctns as well as the β-catenin homologue, plakoglobin (reviewed inCitation92). For simplicity, these other isoforms are not shown with the exception of αE-cat participating in an E-cadherin complex, and αT-cat with an N-cadherin complex.
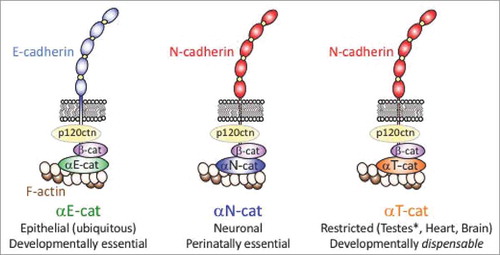
α-Catenins: Knock-out phenotypes reflect tissue distribution
α-Catenins are β-catenin and actin-binding proteins, where binding to both β-catenin and actin is required to directly link the cadherin complex to cortical actin filaments. They are found in nominally-defined epithelial (E), neural (N), and testis (T) forms transcribed from three distinct genes,Citation3 where each is sufficient to rescue cadherin-based adhesion in α-catenin-negative cell lines.Citation3-6 As is often the case with early nomenclature, formal names can be misleading now that greater resolving RNA sequencing technologies are available. In this regard, the human genotype-tissue expression (GTEx) databaseCitation7 clearly shows that αE-cat (CTNNA1) is not epithelial-restricted, but rather ubiquitously expressed (). These data are consistent with evidence that mouse knock-outs targeting Ctnna1 across a range of tissues can lead to penetrant loss of cell-cell adhesion/tissue organization (e.g., whole embryo,Citation8 skin,Citation9 brainCitation10 and heartCitation11). In contrast to the ubiquity of αE-cat, αN-cat (CTNNA2) is largely restricted to brain (), consistent with evidence that Ctnna2 knock-out mice display hypomorphic brains and perinatal lethality.Citation12,Citation13 Remarkably, the more recently evolved αT-cat (CTNNA3), named after its expression in the testis and best known for its role in the heart,Citation14 is also abundantly expressed in the brain, spinal cord, and peripheral nerve (). Although Ctnna3 knock-out mice are viable and fertile,Citation15 this curious tissue distribution of CTNNA3, together with growing linkages between CTNNA3 and diseases compatible with this distribution (see below), raise the intriguing notion that αT-cat/CTNNA3 may be the α-catenin most relevant to a broad range of human diseases.
Figure 2. α-Catenin isoform expression analysis across human tissues. Graphs exported from the human Genotype-Tissue Expression (GTEx) portal using CTNNA1, CTNNA2 and CTNNA3 gene identifiers. Expression values shown as Transcripts Per Million (TPM) calculated from a gene model with isoforms collapsed to a single gene. No other normalization steps were applied. Box plots are shown as median and 25th and 75th percentiles; points are displayed as outliers if they are above or below 1.5 times the interquartile range. Number of human tissue samples range from ∼100-500 per tissue and can be viewed via the portal.
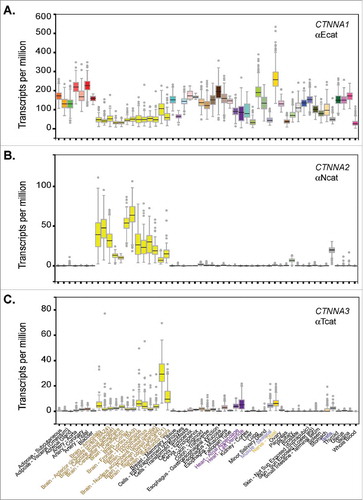
αE-cat: Founding member of the α-catenin family
Due to its ubiquity, molecular and structural analyses are best known for αE-cat, the subject of recent excellent reviews.Citation16-18,Citation19,Citation20 The prevailing view of αE-cat in the cadherin complex is as a mechanosensitive scaffold protein that features a series of six, bundled α-helical domain-regions.Citation21-25 There are two key aspects to its mechanosensitivity. First, the C-terminal F-actin binding domain of αE-cat shows preferential binding to actin filaments under tension in vitro,Citation26 suggesting that αE-cat may preferentially couple the cadherin/β-catenin complex to actin filaments that are under myosin-based cortical tension. Contractile actin structures are typically found at discreet plasma membrane locations (e.g., zonula and focal adhesions), and the precise nature of this force-activated binding event is presently unclear. Second, the middle or M-region of αE-cat undergoes force-dependent unfurling,Citation23,Citation27 exposing a cryptic site that favors recruitment of the related actin-binding protein, vinculin. In epithelia, vinculin recruitment to αE-cat occurs in regions of the plasma membrane that are under elevated forces, such as an apical adhesive zone known as the zonula adherens.Citation23,Citation24 Since a number of proteins interact with αE-cat through its mechanosensitive M-region,Citation17,Citation28 it is possible that some of these partners may be variably recruited to αEcat under distinct force-activated thresholds ().
Figure 3. Mechanosensor model of αE-cat in cell-cell adhesion. The actin-binding domain (ABD) of αE-cat (green) preferentially associates with actin filaments under tension (high tension versus low tension). This leads to unfurling of the M-domain (M1), which allows vinculin binding and adherens junction reinforcement.
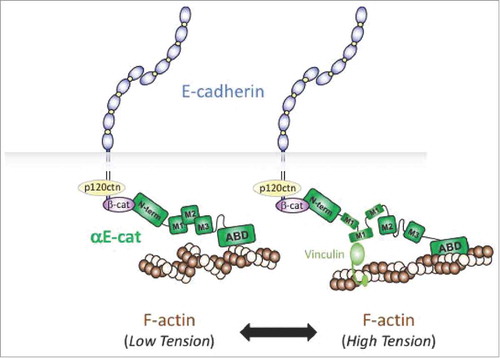
Given the level of amino acid identity/similarity between αE-cat and αT-cat (56.1%/73.7%) or αN-cat (76.5%/83.1%)[3], we may reason that these related α-catenins share an analogous mechanosensitivity. Although biochemical and cellular characterization of αN-cat and αT-cat lags behind αE-cat, recent studies suggest that cadherin complexes containing these α-catenins are indeed different. For example, αE-cat recruits vinculin to adherens junctions more effectively than αN-cat using an α-catenin negative epithelial cell line, possibly due αE-cat's higher affinity for actin filaments in vitro.Citation24 How such differences are leveraged by epithelia (αE-cat) and neurons (αN-cat) to suit their respective junction-coupling needs remains to be clarified. Moreover, in contrast to the established allosteric behavior of αE-cat, where β-catenin binding curiously limits αE-cat's capacity to bind actin filaments in solution,Citation29,Citation30 αT-cat can bind cadherin/β-catenin and actin filaments, simultaneously.Citation31 Thus, while αE-cat within the cadherin/β-catenin complex shows preferential binding to actin filaments under tension,Citation26 αT-cat behaves as a constitutively active, actin-binding protein that can physically couple cadherin/β-catenin to actin in the absence of tension,Citation31 which may be relevant to αT-cat's unique junctional and tissue-specific role (see below). In addition to differences in actin-binding between αE- and αT-cat proteins, recruitment of ligand-binding partners through the M-domain also appears distinct, as loss of αE-cat in heart reduces vinculin recruitment to cardiac cell-cell junctions,Citation11 whereas loss of αT-cat reduces plakophilin-2 (PKP2) recruitmentCitation15,Citation32 (also below). Lastly, it is worth noting that all three α-catenins show a capacity to form homodimers in vitro that are incompatible with cadherin/β-catenin binding, and which allows for robust F-actin binding and bundling activity.Citation30,Citation31,Citation33,Citation34 However, recently measured kinetic parameters suggest that only αE-cat may be able to sustain the homodimeric state at physiological concentration in cells,Citation33 where homodimerization contributes to membrane protrusive activities required for cell migration and nascent contact formation.Citation35,Citation36 Together, these data suggest that mechanosensor, M-domain-binding-partner and homodimerization abilities of αT-cat are distinct from αE-cat, which may be relevant to the tissue-restricted functions of αT-cat.
αT-cat in the heart and cardiomyopathy
αT-cat was named for its localization in peritubular myoid cells of the testis,Citation32 but is currently best known for its role in the heart. This is largely because αT-cat null mice show no obvious fertility defects, but rather develop a dilated cardiomyopathy (DCM) after 3–6 months of age.Citation15 Although mutations in αT-cat have not yet been found associated with DCM in humans,Citation37,Citation38 two mutations (detailed below) have been implicated in the development of arrythmogenic right ventricle cardiomyopathy (ARVC).Citation39 As recent evidence indicates that the left ventricle is often affected in historically defined ARVC patients, this biventricular disease is now referred to as arrhythmogenic cardiomyopathy (ACM).Citation40
ACM disease is typically caused by mutations in proteins that comprise desmosomes, a type of cadherin-based intercellular adhesion that employs plaque proteins (plakoglobin, plakophilins, desmoplakin) to link to the intermediate filament cytoskeleton.Citation41 Desmosomes are particularly important in tissues that withstand substantial mechanical strain, such as heart and skin.Citation42,Citation43 In this regard, αT-cat prominently localizes to a specialized cell-cell junction in cardiomyocytes, known as the intercalated disc (ICD), which contains distinct adherens junction, desmosome and gap junction structures.Citation44,Citation45 In the hearts of higher vertebrates, the ICD largely comprises a hybrid adherens junction/desmosome structure known as the area composita,Citation46,Citation47 where this hybrid junction is considered optimized to withstand the increased mechanical load of the four-chamber mammalian heart.Citation48-50 Although the unique strength and molecular mechanics of this junction type remains poorly understood, αT-cat may be a key integrator of the area composita, as it directly binds the desmosome component plakophilin-2 (PKP2)Citation51 while also participating in the cadherin/β-catenin complex, presumably reinforcing adherens junction and desmosome alignment (). Indeed, while αT-cat knock-out hearts develop normally due to compensation by the related αE-cat, over time, these mice show reduced localization of PKP2 and the Connexin 43 gap junction component at intercalated disks.Citation15 Reduced area composita, hybrid-junction coupling (via PKP2) likely contributes to the decreased cardiac contractility and ejection fraction of αT-cat null mice, whereas reduced gap junction coupling (via Connexin 43) increases sensitivity to ventricular arrhythmia following ischemic injury.Citation15
Figure 4. Model of αT-cat in cardiomyocyte cell-cell adhesion. αT-cat (orange) can interact with β-cat, actin and the desmosomal component, PKP2, via its central M-domain (end of M2 and M3), which allows for the alignment and reinforcement of a hybrid adherens junction-desmosome structure known as the area composita region of the intercalated disk. The intermediate filament-binding protein, desmoplakin (DSP), is also shown.
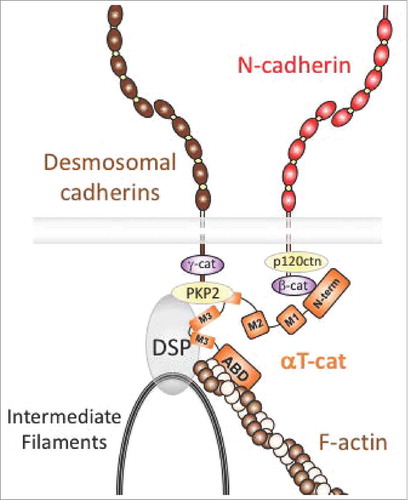
Evidence that PKP2 mutations are also associated with ACM,Citation52 suggest that a particular aspect of αT-cat/PKP2 coupling may be important for normal right ventricle structure and function. For example, PKP2 interacts with αT-cat (but not αE-cat) via the M-domain.Citation39,Citation51 As discussed above, both αE-cat M- and actin-binding domains require force-dependent conformation regulatory events for their respective binding activities, whereas αT-cat appears less mechanosensitive, being more available to its binding-partners.Citation20,Citation31 These or other differences may explain why αT-cat is dispensable for normal heart development (due to compensation by αE-cat), but important for cardiac function with age. Indeed, as the mechanical load on the heart increases after birth and the ICD matures, αT-cat's role as molecular integrator of the area composita appears critical, as evidenced by the earlier onset of cardiomyopathy in αT-cat mutant mice compared to αE-cat conditional KO mice (3 versus 8 months of age, respectively).Citation11,Citation15
Recent biochemical and cell culture studies now rationalize how αT-cat heterozygous mutations may function as dominant inhibitors of cardiomyocyte function in ACM: One mutation (V94D) blocks β-catenin bindingCitation39 and favors αT-cat homodimerzation,Citation31 leading to altered junctional localization in cardiomyocyte junctionsCitation31; the second mutation deletes a leucine in the critical actin-binding domain (L765del) and induces protein dimerization/ aggregation.Citation31,Citation39 Although formal evidence for these mutations causing ACM awaits testing in mouse models, it appears that both αT-cat pathogenic mutations enhance the intrinsic homodimerization and/or aggregation potential of αT-cat, which may prevent normal cadherin/catenin/actin coupling and other possible maladaptation. Lastly, it is worth noting that requirement of α-catenin-based cell-cell adhesion to heart structure and function is not absolute, but contextual, and based on developmental timing or degree of tissue injury. For example, the early loss of both αE- and αT-cat in mice is incompatible with heart development but tolerated when induced perinatally.Citation53 Remarkably, the loss of both catenins appears beneficial when removed in adult hearts subjected to ischemic injury, in part due to elevated YAP signaling that favors proliferation.Citation53 Such complexities raise the counterintuitive possibility that attenuating the function of proteins collectively required for tissue development may be beneficial during adult tissue repair after injury.
αT-cat linkages to allergic disease
One of the more surprising developments in the α-catenin field are the number of independent genetic association studies linking αT-cat (CTNNA3) with asthma and food allergy. Genome-wide association studies have linked several non-coding CTNNA3 polymorphisms with two distinct forms of asthma, occupational asthma induced by chemical exposureCitation54,Citation55 and steroid resistant atopic asthma.Citation56,Citation57 One study identified copy number deletions in CTNNA3 associated with pediatric food allergy.Citation58 The surprise with these associations is that the restricted distribution of αT-cat expression in human tissues (, brain/peripheral nerve, heart, skeletal muscle and testis) suggests that either rare, contextually activated or non-canonical cell-types contribute to allergic disease.
Using the viable and fertile αT-cat knock-out mouse described above, our team has validated these αT-cat linkages to asthma using both chemical and house dust-mite models of asthma.Citation59,Citation60 Curiously, full loss of αT-cat strongly suppresses airway hyperreactivity, a hallmark of asthma, but the αT-cat-expressing cell type that drives allergic airway responses remains to be determined. Remarkably, the only lung cells that obviously express αT-cat are cardiomyocytes that line the pulmonary vasculature.Citation60,Citation61 However, anatomical differences in human versus rodent pulmonary veins, their proximity to airways and relative degree of cardiomyocyte ensheathment has raised doubt that cardiomyocytes are the αT-cat-expressing cell type that drives asthma.Citation61,Citation62 An appealing cell-type to consider for linkages between an adhesion protein and allergic diseases are immune cells. Although low levels of αT-cat RNA have been detected in EBV-transformed peripheral blood cells and lymphoid cancer linesCitation54,Citation56,Citation58 (https://www.proteinatlas.org/ENSG00000183230-CTNNA3/cell), evidence for protein detection is generally lacking, with exception of one study suggesting that αT-cat may contribute to the upregulation of basophil-activation markers, CD203c and CD63.Citation58 Indeed, immune cells generally do not express cadherins or α-catenin adhesion components, but Th2-cytokines can robustly upregulate E-cadherin and αE-cat in dendritic cells and alternatively activated macrophages.Citation63-67 We find no evidence that αT-cat is upregulated under these same conditions (not shown). Thus, future work will be required to further validate and understand these intriguing connections between αT-cat and allergic disease.
αT-cat in the nervous system and disease
Early studies documented αT-cat protein expression in brain,Citation3 but functional significance of this expression has lagged presumably because of difficulties interrogating behavioral defects in mice. Moreover, identification of αT-cat-expressing cell types in the brain has been somewhat limited by the lack of robust tools (e.g., fluorescent membrane-anchored reporter mouse). For example, while an early study suggested that αT-cat protein may be expressed in murine cortical neurons,Citation68 αT-cat is more prominently detected in ependymal cell junctions that line ventricles, as well as cells within the molecular layer of the cerebellum.Citation69 In human tissue, RNA sequencing data reveal that αT-cat expression is highest in brain and spinal cord (), the latter of which is likely due to the presence of a central canal lined by αT-cat-positive ependymal cells. The unique functional role of αT-cat in this specialized epithelium remains unclear, however, as αT-cat knock-out mice show no obvious defect in ventricle structure, possibly due to compensatory upregulation of αE-cat.Citation69
Despite the absence of an obvious neurological phenotype in αT-cat null mice (Frans Van Roy, personal communication), a number of linkage studies raise the possibility that αT-cat may contribute to disease in humans. Specifically, the αT-cat gene, CTNNA3, is located near a common fragile site on chromosome 10,Citation70 and has been linked to late onset Alzheimer's disease in femalesCitation71 (reviewed inCitation72). CTNNA3 is also linked to autism in two large cohorts of European ancestry with replication in two other cohorts,Citation73 and rare deletions in αT-cat were identified in individuals with autism spectrum disorder.Citation74,Citation75 While transcriptomic analysis of WT and αT-cat knock-mouse cerebella suggest alteration of pathways linked to Alzheimer's and autism,Citation69 future work will be required to define the cell type and unique junctional-specialization supported by αT-cat function. Indeed, available online transcriptomic datasets of human and mouse brain cell populations suggest that oligodendrocytes may be a major αT-cat-expressing cell type in brain.Citation76
αT-cat associations with cancer
Among the α-catenin family members, αE-cat is best appreciated for playing a contributing role in tumorigenesis in large part because it plays an integral part in epithelial cell-cell adhesion with E-cadherin (CDH1), a bona fide tumor-suppressor gene.Citation77,Citation78 Since αT-cat mRNA and protein are generally absent from epithelial tissues (; see also Human Atlas), there was an early expectation that it might not contribute to cancer. However, a number of recent studies suggest that we may need to keep an open mind on this front. For example, a recent proximity proteomics study revealed αT-cat as the 9th most abundant protein at E-cadherin contacts in non-transformed Madin Darby Canine Kidney epithelial cells,Citation79 raising the possibility that low levels of αT-cat mRNA may be uncorrelated from its polypeptide abundance. Remarkably, CTNNA3 is one of the largest genes in the genome (i.e., spanning 1.78 Megabases) and proximal to a common fragile site (FRAD10D).Citation80 In this regard, monoallelic or reduced expression of CTNNA3 is associated with urothelial carcinoma of the bladder,Citation81 pancreatic cancer associated with Schwachman-Diamond Syndrome,Citation82 oropharyngeal squamous cell carcinomaCitation83 and hepatocellular carcinoma.Citation84 In addition, deletion, truncation and missense mutations were identified in CTNNA3 in NSCLCsCitation85 and laryngeal carcinoma.Citation86 In some of these studies, αT-cat was knocked-down and phenotypes typically associated with cancer were modestly enhanced (e.g., proliferation, invasion, migration).Citation84,Citation86 Intriguingly, SNPs in CTNNA3 were associated with radiation induced brain cancersCitation87 and focal loss of CTNNA3 was associated with a hybrid neurofibroma/ schwannoma,Citation88 perhaps consistent with the prominent expression of CTNNA3 in brain and peripheral nerve (). While these studies are suggestive, future work that makes use of validated, isoform-specific αT-cat antibodies and αT-cat knock-out/floxed miceCitation15,Citation53 will be required to determine the extent to which αT-cat is a bona fide tumor suppressor protein, particularly since the relationship between cell-cell adhesion and cancer is not universally suppressive.Citation89
Revised evolutionary perspective
Comparison of the three α-catenin genes reveals that αT-cat is the most recently evolved, likely arising from an amniote-specific duplication of the αN-cat gene.Citation90 Evidence that αT-cat emerged with the development of terrestrial vertebrates that have a four-chambered heart, together with it being linked to ACM disease and required for normal cardiac function during murine lifespan, has led to the notion that αT-cat evolved to address the unique mechanical demands of the heart.Citation15,Citation31,Citation39 However, recent transcriptomic studies reveal that αT-cat is also abundantly expressed in the nervous system ().Citation76 This not only strengthens the plausibility of recent genetic linkages between αT-cat and neurological diseases,Citation69-76 but suggests that αT-cat evolved to meet the demands of two very different tissue systems (i.e., brain/peripheral nerves and heart). The mechano-organizational features that αT-cat uniquely brings to adherens junctions across these systems will require further study. Thus, while most of α-catenin research has focused on the developmentally essential founding member, αE-cat, the developmentally dispensable αT-cat may be worthy of greater attention, emerging as a broadly disease-relevant α-catenin.
Disclosure of potential conflicts of interest
No potential conflicts of interest were disclosed.
Acknowledgments
S.E.C. and E.E.R. were supported by the Northwestern University Allergy Immunology Research Program (NUAIR) T32AI083216 and C.J.G. by NIHL134800.
Additional information
Funding
References
- Niessen CM, Leckband D, Yap AS. Tissue organization by cadherin adhesion molecules: Dynamic molecular and cellular mechanisms of morphogenetic regulation. Physiol Rev. 2011; 91(2):691–731. doi:10.1152/physrev.00004.2010. PMID:21527735.
- Zaidel-Bar R. Cadherin adhesome at a glance. J Cell Sci. 2013; 126(Pt 2):373–8. doi:10.1242/jcs.111559. PMID:23547085.
- Janssens B, Goossens S, Staes K, Gilbert B, van Hengel J, Colpaert C, Bruyneel E, Mareel M, van Roy F. Alphat-catenin: A novel tissue-specific beta-catenin-binding protein mediating strong cell-cell adhesion. J Cell Sci. 2001; 114(Pt 17):3177–88. PMID:11590244.
- Herrenknecht K, Ozawa M, Eckerskorn C, Lottspeich F, Lenter M, Kemler R. The uvomorulin-anchorage protein alpha catenin is a vinculin homologue. Proc Natl Acad Sci U S A. 1991; 88(20):9156–60. doi:10.1073/pnas.88.20.9156. PMID:1924379.
- Hirano S, Kimoto N, Shimoyama Y, Hirohashi S, Takeichi M. Identification of a neural alpha-catenin as a key regulator of cadherin function and multicellular organization. Cell. 1992; 70(2):293–301. doi:10.1016/0092-8674(92)90103-J. PMID:1638632.
- Ewing CM, Ru N, Morton RA, Robinson JC, Wheelock MJ, Johnson KR, Barrett JC, Isaacs WB. Chromosome 5 suppresses tumorigenicity of PC3 prostate cancer cells: Correlation with re-expression of alpha-catenin and restoration of E-cadherin function. Cancer Res. 1995; 55(21):4813–7. PMID:7585512.
- Mele M, Ferreira PG, Reverter F, DeLuca DS, Monlong J, Sammeth M, Young TR, Goldmann JM, Pervouchine DD, Sullivan TJ, et al. Human genomics. The human transcriptome across tissues and individuals. Science. 2015; 348(6235):660–5. doi:10.1126/science.aaa0355. PMID:25954002.
- Torres M, Stoykova A, Huber O, Chowdhury K, Bonaldo P, Mansouri A, Butz S, Kemler R, Gruss P. An alpha-E-catenin gene trap mutation defines its function in preimplantation development. Proc Natl Acad Sci U S A. 1997; 94(3):901–6. doi:10.1073/pnas.94.3.901. PMID:9023354.
- Vasioukhin V, Bauer C, Degenstein L, Wise B, Fuchs E. Hyperproliferation and defects in epithelial polarity upon conditional ablation of alpha-catenin in skin. Cell. 2001; 104(4):605–17. doi:10.1016/S0092-8674(01)00246-X. PMID:11239416.
- Lien WH, Klezovitch O, Fernandez TE, Delrow J, Vasioukhin V. Alphae-catenin controls cerebral cortical size by regulating the hedgehog signaling pathway. Science. 2006; 311(5767):1609–12. doi:10.1126/science.1121449. PMID:16543460.
- Sheikh F, Chen Y, Liang X, Hirschy A, Stenbit AE, Gu Y, Dalton ND, Yajima T, Lu Y, Knowlton KU, et al. Alpha-E-catenin inactivation disrupts the cardiomyocyte adherens junction, resulting in cardiomyopathy and susceptibility to wall rupture. Circulation. 2006; 114(10):1046–55. doi:10.1161/CIRCULATIONAHA.106.634469. PMID:16923756.
- Park C, Falls W, Finger JH, Longo-Guess CM, Ackerman SL. Deletion in Catna2, encoding alpha N-catenin, causes cerebellar and hippocampal lamination defects and impaired startle modulation. Nat Genet. 2002; 31(3):279–84. doi:10.1038/ng908. PMID:12089526.
- Uemura M, Takeichi M. Alpha N-catenin deficiency causes defects in axon migration and nuclear organization in restricted regions of the mouse brain. Dev Dyn. 2006; 235(9):2559–66. doi:10.1002/dvdy.20841. PMID:16691566.
- Vite A, Li J, Radice GL. New functions for alpha-catenins in health and disease: From cancer to heart regeneration. Cell Tissue Res. 2015; 360(3):773–83. doi:10.1007/s00441-015-2123-x. PMID:25673211.
- Li J, Goossens S, van Hengel J, Gao E, Cheng L, Tyberghein K, Shang X, De Rycke R, van Roy F, Radice GL. Loss of alphaT-catenin alters the hybrid adhering junctions in the heart and leads to dilated cardiomyopathy and ventricular arrhythmia following acute ischemia. J Cell Sci. 2012; 125(Pt 4):1058–67. doi:10.1242/jcs.098640. PMID:22421363.
- Benjamin JM, Nelson WJ. Bench to bedside and back again: Molecular mechanisms of alpha-catenin function and roles in tumorigenesis. Semin Cancer Biol. 2008; 18(1):53–64. doi:10.1016/j.semcancer.2007.08.003. PMID:17945508.
- Maiden SL, Hardin J. The secret life of alpha-catenin: Moonlighting in morphogenesis. J Cell Biol. 2011; 195(4):543–52. doi:10.1083/jcb.201103106. PMID:22084304.
- Reynolds AB. Epithelial organization: New perspective on alpha-catenin from an ancient source. Curr Biol. 2011; 21(11):R430–2. doi:10.1016/j.cub.2011.04.043. PMID:21640901.
- Ladoux B, Nelson WJ, Yan J, Mège RM. The mechanotransduction machinery at work at adherens junctions. Integr Biol (Camb). 2015; 7(10):1109–19. doi:10.1039/C5IB00070J. PMID:25968913.
- Nelson WJ, Weis WI. 25 Years of tension over actin binding to the cadherin cell adhesion complex: The devil is in the details. Trends Cell Biol. 2016; 26(7):471–3. doi:10.1016/j.tcb.2016.04.010. PMID:27166091.
- le Duc Q, Shi Q, Blonk I, Sonnenberg A, Wang N, Leckband D, de Rooij J. Vinculin potentiates E-cadherin mechanosensing and is recruited to actin-anchored sites within adherens junctions in a myosin II-dependent manner. J Cell Biol. 2010; 189(7):1107–15. doi:10.1083/jcb.201001149. PMID:20584916.
- Barry AK, Tabdili H, Muhamed I, Wu J, Shashikanth N, Gomez GA, Yap AS, Gottardi CJ, de Rooij J, Wang N, et al. Alpha-catenin cytomechanics–role in cadherin-dependent adhesion and mechanotransduction. J Cell Sci. 2014; 127( Pt 8):1779–91. doi:10.1242/jcs.139014. PMID:24522187.
- Yonemura S, Wada Y, Watanabe T, Nagafuchi A, Shibata M. Alpha-Catenin as a tension transducer that induces adherens junction development. Nat Cell Biol. 2010; 12(6):533–42. doi:10.1038/ncb2055. PMID:20453849.
- Ishiyama N, Tanaka N, Abe K, Yang YJ, Abbas YM, Umitsu M, Nagar B, Bueler SA, Rubinstein JL, Takeichi M, et al. An autoinhibited structure of alpha-catenin and its implications for vinculin recruitment to adherens junctions. J Biol Chem. 2013; 288(22):15913–25. doi:10.1074/jbc.M113.453928. PMID:23589308.
- Rangarajan ES, Izard T. Dimer asymmetry defines alpha-catenin interactions. Nat Struct Mol Biol. 2013; 20(2):188–93. doi:10.1038/nsmb.2479. PMID:23292143.
- Buckley CD, Tan J, Anderson KL, Hanein D, Volkmann N, Weis WI, Nelson WJ, Dunn AR. Cell adhesion. The minimal cadherin-catenin complex binds to actin filaments under force. Science. 2014; 346(6209):1254211. doi:10.1126/science.1254211. PMID:25359979.
- Yao M, Qiu W, Liu R, Efremov AK, Cong P, Seddiki R, Payre M, Lim CT, Ladoux B, Mège RM, et al. Force-dependent conformational switch of alpha-catenin controls vinculin binding. Nat Commun. 2014; 5:4525. doi:10.1038/ncomms5525. PMID:25077739.
- Kobielak A, Fuchs E. Alpha-catenin: At the junction of intercellular adhesion and actin dynamics. Nat Rev Mol Cell Biol. 2004; 5(8):614–25. doi:10.1038/nrm1433. PMID:15366705.
- Yamada S, Pokutta S, Drees F, Weis WI, Nelson WJ. Deconstructing the cadherin-catenin-actin complex. Cell. 2005; 123(5):889–901. doi:10.1016/j.cell.2005.09.020. PMID:16325582.
- Drees F, Pokutta S, Yamada S, Nelson WJ, Weis WI. Alpha-catenin is a molecular switch that binds E-cadherin-beta-catenin and regulates actin-filament assembly. Cell. 2005; 123(5):903–15. doi:10.1016/j.cell.2005.09.021. PMID:16325583.
- Wickline ED, Dale IW, Merkel CD, Heier JA, Stolz DB, Kwiatkowski AV. Alphat-catenin is a constitutive actin-binding alpha-catenin that directly couples the cadherin.catenin complex to actin filaments. J Biol Chem. 2016; 291(30):15687–99. doi:10.1074/jbc.M116.735423. PMID:27231342.
- Goossens S, Janssens B, Vanpoucke G, De Rycke R, van Hengel J, van Roy F. Truncated isoform of mouse alphaT-catenin is testis-restricted in expression and function. FASEB J. 2007; 21(3):647–55. doi:10.1096/fj.06-6066com. PMID:17185752.
- Pokutta S, Choi HJ, Ahlsen G, Hansen SD, Weis WI. Structural and thermodynamic characterization of cadherin.beta-catenin.alpha-catenin complex formation. J Biol Chem. 2014; 289(19):13589–601. doi:10.1074/jbc.M114.554709. PMID:24692547.
- Hansen SD, Kwiatkowski AV, Ouyang CY, Liu H, Pokutta S, Watkins SC, Volkmann N, Hanein D, Weis WI, Mullins RD, et al. Alphae-catenin actin-binding domain alters actin filament conformation and regulates binding of nucleation and disassembly factors. Mol Biol Cell. 2013; 24(23):3710–20. doi:10.1091/mbc.E13-07-0388. PMID:24068324.
- Benjamin JM, Kwiatkowski AV, Yang C, Korobova F, Pokutta S, Svitkina T, Weis WI, Nelson WJ. AlphaE-catenin regulates actin dynamics independently of cadherin-mediated cell-cell adhesion. J Cell Biol. 2010; 189(2):339–52. doi:10.1083/jcb.200910041. PMID:20404114.
- Wood MN, Ishiyama N, Singaram I, Chung CM, Flozak AS, Yemelyanov A, Ikura M, Cho W, Gottardi CJ. Alpha-Catenin homodimers are recruited to phosphoinositide-activated membranes to promote adhesion. J Cell Biol. 2017; 216(11):3767–83. doi:10.1083/jcb.201612006. PMID:28874417.
- Janssens B, Mohapatra B, Vatta M, Goossens S, Vanpoucke G, Kools P, Montoye T, van Hengel J, Bowles NE, van Roy F, et al. Assessment of the CTNNA3 gene encoding human alpha T-catenin regarding its involvement in dilated cardiomyopathy. Hum Genet. 2003; 112(3):227–36. PMID:12596047.
- Christensen AH, Benn M, Tybjærg-Hansen A, Haunso S, Svendsen JH. Screening of three novel candidate genes in arrhythmogenic right ventricular cardiomyopathy. Genet Test Mol Biomarkers. 2011; 15(4):267–71. doi:10.1089/gtmb.2010.0151. PMID:21254927.
- van Hengel J, Calore M, Bauce B, Dazzo E, Mazzotti E, De Bortoli M, Lorenzon A, Li Mura IE, Beffagna G, Rigato I, et al. Mutations in the area composita protein alphaT-catenin are associated with arrhythmogenic right ventricular cardiomyopathy. Eur Heart J. 2013; 34(3):201–10. doi:10.1093/eurheartj/ehs373. PMID:23136403.
- Sommariva E, Stadiotti I, Perrucci GL, Tondo C, Pompilio G. Cell models of arrhythmogenic cardiomyopathy: Advances and opportunities. Dis Model Mech. 2017; 10(7):823–35. doi:10.1242/dmm.029363. PMID:28679668.
- Harmon RM, Green KJ. Structural and functional diversity of desmosomes. Cell Commun Adhes. 2013; 20(6):171–87. doi:10.3109/15419061.2013.855204. PMID:24205984.
- Patel DM, Green KJ. Desmosomes in the heart: A review of clinical and mechanistic analyses. Cell Commun Adhes. 2014; 21(3):109–28. doi:10.3109/15419061.2014.906533. PMID:24754498.
- Johnson JL, Najor NA, Green KJ. Desmosomes: Regulators of cellular signaling and adhesion in epidermal health and disease. Cold Spring Harb Perspect Med. 2014; 4(11):a015297. doi:10.1101/cshperspect.a015297. PMID:25368015.
- Forbes MS, Sperelakis N. Intercalated discs of mammalian heart: A review of structure and function. Tissue Cell. 1985; 17(5):605–48. doi:10.1016/0040-8166(85)90001-1. PMID:3904080.
- Vite A, Radice GL. N-cadherin/catenin complex as a master regulator of intercalated disc function. Cell Commun Adhes. 2014; 21(3):169–79. doi:10.3109/15419061.2014.908853. PMID:24766605.
- Franke WW, Borrmann CM, Grund C, Pieperhoff S. The area composita of adhering junctions connecting heart muscle cells of vertebrates. I. Molecular definition in intercalated disks of cardiomyocytes by immunoelectron microscopy of desmosomal proteins. Eur J Cell Biol. 2006; 85(2):69–82. doi:10.1016/j.ejcb.2005.11.003. PMID:16406610.
- Li J, Radice GL. A new perspective on intercalated disc organization: Implications for heart disease. Dermatol Res Pract. 2010; 2010:207835. PMID:20585598.
- Pieperhoff S, Franke WW. The area composita of adhering junctions connecting heart muscle cells of vertebrates – IV: Coalescence and amalgamation of desmosomal and adhaerens junction components – late processes in mammalian heart development. Eur J Cell Biol. 2007; 86(7):377–91. doi:10.1016/j.ejcb.2007.04.001. PMID:17532539.
- Pieperhoff S, Franke WW. The area composita of adhering junctions connecting heart muscle cells of vertebrates. VI. Different precursor structures in non-mammalian species. Eur J Cell Biol. 2008; 87(7):413–30. doi:10.1016/j.ejcb.2008.02.005. PMID:18420304.
- Pieperhoff S, Schumacher H, Franke WW. The area composita of adhering junctions connecting heart muscle cells of vertebrates. V. The importance of plakophilin-2 demonstrated by small interference RNA-mediated knockdown in cultured rat cardiomyocytes. Eur J Cell Biol. 2008; 87(7):399–411. doi:10.1016/j.ejcb.2007.12.002. PMID:18261826.
- Goossens S, Janssens B, Bonné S, De Rycke R, Braet F, van Hengel J, van Roy F. A unique and specific interaction between alphaT-catenin and plakophilin-2 in the area composita, the mixed-type junctional structure of cardiac intercalated discs. J Cell Sci. 2007; 120(Pt 12):2126–36. doi:10.1242/jcs.004713. PMID:17535849.
- Li Mura IE, Bauce B, Nava A, Fanciulli M, Vazza G, Mazzotti E, Rigato I, De Bortoli M, Beffagna G, Lorenzon A, et al. Identification of a PKP2 gene deletion in a family with arrhythmogenic right ventricular cardiomyopathy. Eur J Hum Genet. 2013; 21(11):1226–31. doi:10.1038/ejhg.2013.39. PMID:23486541.
- Li J, Gao E, Vite A, Yi R, Gomez L, Goossens S, van Roy F, Radice GL. Alpha-catenins control cardiomyocyte proliferation by regulating Yap activity. Circ Res. 2015; 116(1):70–9. doi:10.1161/CIRCRESAHA.116.304472. PMID:25305307.
- Kim SH, Cho BY, Park CS, Shin ES, Cho EY, Yang EM, Kim CW, Hong CS, Lee JE, Park HS. Alpha-T-catenin (CTNNA3) gene was identified as a risk variant for toluene diisocyanate-induced asthma by genome-wide association analysis. Clin Exp Allergy. 2009; 39(2):203–12. doi:10.1111/j.1365-2222.2008.03117.x. PMID:19187332.
- Bernstein DI, Kashon M, Lummus ZL, Johnson VJ, Fluharty K, Gautrin D, Malo JL, Cartier A, Boulet LP, Sastre J, et al. CTNNA3 (alpha-catenin) gene variants are associated with diisocyanate asthma: A replication study in a caucasian worker population. Toxicol Sci. 2013; 131(1):242–6. doi:10.1093/toxsci/kfs272. PMID:22977168.
- McGeachie MJ, et al. CTNNA3 and SEMA3D: Promising loci for asthma exacerbation identified through multiple genome-wide association studies. J Allergy Clin Immunol. 2015; 136(6):1503–10. doi:10.1016/j.jaci.2015.04.039. PMID:26073756.
- Perin P, Potocnik U. Polymorphisms in recent GWA identified asthma genes CA10, SGK493, and CTNNA3 are associated with disease severity and treatment response in childhood asthma. Immunogenetics. 2014; 66(3):143–51. doi:10.1007/s00251-013-0755-0. PMID:24407380.
- Li J, Glessner JT, Pandey R, Wei Z, Bakay M, Mentch FD, Pellegrino R, Wang T, Kim C, Hou C, et al. Copy number variations in CTNNA3 and RBFOX1 associate with pediatric food allergy. J Immunol. 2015; 195(4):1599–607. doi:10.4049/jimmunol.1402310. PMID:26188062.
- Folmsbee SS, Budinger GR, Bryce PJ, Gottardi CJ. The cardiomyocyte protein alphaT-catenin contributes to asthma through regulating pulmonary vein inflammation. J Allergy Clin Immunol. 2016; 138(1):123–9e2. doi:10.1016/j.jaci.2015.11.037.
- Folmsbee SS, Morales-Nebreda L, Van Hengel J, Tyberghein K, Van Roy F, Budinger GR, Bryce PJ, Gottardi CJ. The cardiac protein alphaT-catenin contributes to chemical-induced asthma. Am J Physiol Lung Cell Mol Physiol. 2015; 308(3):L253–8. doi:10.1152/ajplung.00331.2014. PMID:25480337.
- Folmsbee SS, Gottardi CJ. Cardiomyocytes of the heart and pulmonary veins: Novel contributors to asthma? Am J Respir Cell Mol Biol. 2017. doi:10.1165/rcmb.2016-0261TR. PMID:28481622.
- Mueller-Hoecker J, Beitinger F, Fernandez B, Bahlmann O, Assmann G, Troidl C, Dimomeletis I, Kääb S, Deindl E. Of rodents and humans: A light microscopic and ultrastructural study on cardiomyocytes in pulmonary veins. Int J Med Sci. 2008; 5(3):152–8. doi:10.7150/ijms.5.152. PMID:18612369.
- Van den Bossche J, Malissen B, Mantovani A, De Baetselier P, Van Ginderachter JA. Regulation and function of the E-cadherin/catenin complex in cells of the monocyte-macrophage lineage and DCs. Blood. 2012; 119(7):1623–33. doi:10.1182/blood-2011-10-384289. PMID:22174153.
- Van den Bossche J, Van Ginderachter JA. E-cadherin: From epithelial glue to immunological regulator. Eur J Immunol. 2013; 43(1):34–7. doi:10.1002/eji.201243168. PMID:23229729.
- Van den Bossche J, Laoui D, Naessens T, Smits HH, Hokke CH, Stijlemans B, Grooten J, De Baetselier P, Van Ginderachter JA. E-cadherin expression in macrophages dampens their inflammatory responsiveness in vitro, but does not modulate M2-regulated pathologies in vivo. Sci Rep. 2015; 5:12599. doi:10.1038/srep12599. PMID:26226941.
- Siddiqui KR, Laffont S, Powrie F. E-cadherin marks a subset of inflammatory dendritic cells that promote T cell-mediated colitis. Immunity. 2010; 32(4):557–67. doi:10.1016/j.immuni.2010.03.017. PMID:20399121.
- Mayumi N, Watanabe E, Norose Y, Watari E, Kawana S, Geijtenbeek TB, Takahashi H. E-cadherin interactions are required for Langerhans cell differentiation. Eur J Immunol. 2013; 43(1):270–80. doi:10.1002/eji.201242654. PMID:23135957.
- Busby V, Goossens S, Nowotny P, Hamilton G, Smemo S, Harold D, Turic D, Jehu L, Myers A, Womick M, et al. Alpha-T-catenin is expressed in human brain and interacts with the Wnt signaling pathway but is not responsible for linkage to chromosome 10 in Alzheimer's disease. Neuromolecular Med. 2004; 5(2):133–46. doi:10.1385/NMM:5:2:133. PMID:15075440.
- Folmsbee SS, Wilcox DR, Tyberghein K, De Bleser P, Tourtellotte WG, van Hengel J, van Roy F, Gottardi CJ. Alphat-catenin in restricted brain cell types and its potential connection to autism. J Mol Psychiatry. 2016; 4:2. doi:10.1186/s40303-016-0017-9. PMID:27330745.
- Smith DI, Zhu Y, McAvoy S, Kuhn R. Common fragile sites, extremely large genes, neural development and cancer. Cancer Lett. 2006; 232(1):48–57. doi:10.1016/j.canlet.2005.06.049. PMID:16221525.
- Miyashita A, Arai H, Asada T, Imagawa M, Matsubara E, Shoji M, Higuchi S, Urakami K, Kakita A, Takahashi H, et al. Genetic association of CTNNA3 with late-onset Alzheimer's disease in females. Hum Mol Genet. 2007; 16(23):2854–69. doi:10.1093/hmg/ddm244. PMID:17761686.
- Smith JD, Meehan MH, Crean J, McCann A. Alpha T-catenin (CTNNA3): A gene in the hand is worth two in the nest. Cell Mol Life Sci. 2011; 68(15):2493–8. doi:10.1007/s00018-011-0728-0. PMID:21598020.
- Wang K, Zhang H, Ma D, Bucan M, Glessner JT, Abrahams BS, Salyakina D, Imielinski M, Bradfield JP, Sleiman PM, et al. Common genetic variants on 5p14.1 associate with autism spectrum disorders. Nature. 2009; 459(7246):528–33. doi:10.1038/nature07999. PMID:19404256.
- O'Roak BJ, Vives L, Girirajan S, Karakoc E, Krumm N, Coe BP, Levy R, Ko A, Lee C, Smith JD, et al. Sporadic autism exomes reveal a highly interconnected protein network of de novo mutations. Nature. 2012; 485(7397):246–50. doi:10.1038/nature10989. PMID:22495309.
- Bacchelli E, Ceroni F, Pinto D, Lomartire S, Giannandrea M, D'Adamo P, Bonora E, Parchi P, Tancredi R, Battaglia A, et al. A CTNNA3 compound heterozygous deletion implicates a role for alphaT-catenin in susceptibility to autism spectrum disorder. J Neurodev Disord. 2014; 6(1):17. doi:10.1186/1866-1955-6-17. PMID:25050139.
- Zhang Y, Chen K, Sloan SA, Bennett ML, Scholze AR, O'Keeffe S, Phatnani HP, Guarnieri P, Caneda C, Ruderisch N, et al. An RNA-sequencing transcriptome and splicing database of glia, neurons, and vascular cells of the cerebral cortex. J Neurosci. 2014; 34(36):11929–47. doi:10.1523/JNEUROSCI.1860-14.2014. PMID:25186741.
- Berx G, van Roy F. Involvement of members of the cadherin superfamily in cancer. Cold Spring Harb Perspect Biol. 2009; 1(6):a003129. doi:10.1101/cshperspect.a003129. PMID:20457567.
- Majewski IJ, Kluijt I, Cats A, Scerri TS, de Jong D, Kluin RJ, Hansford S, Hogervorst FB, Bosma AJ, Hofland I, et al. An alpha-E-catenin (CTNNA1) mutation in hereditary diffuse gastric cancer. J Pathol. 2013; 229(4):621–9. doi:10.1002/path.4152. PMID:23208944.
- Van Itallie CM, Tietgens AJ, Tietgens AJ, Aponte A, Fredriksson K, Fanning AS, Gucek M, Anderson JM. Biotin ligase tagging identifies proteins proximal to E-cadherin, including lipoma preferred partner, a regulator of epithelial cell-cell and cell-substrate adhesion. J Cell Sci. 2014; 127(Pt 4):885–95. doi:10.1242/jcs.140475. PMID:24338363.
- Gao G, Smith DI. Very large common fragile site genes and their potential role in cancer development. Cell Mol Life Sci. 2014; 71(23):4601–15. doi:10.1007/s00018-014-1753-6. PMID:25300511.
- Meehan M, Melvin A, Gallagher E, Smith J, McGoldrick A, Moss C, Goossens S, Harrison M, Kay E, Fitzpatrick J, et al. Alpha-T-catenin (CTNNA3) displays tumour specific monoallelic expression in urothelial carcinoma of the bladder. Genes Chromosomes Cancer. 2007; 46(6):587–93. doi:10.1002/gcc.20443. PMID:17366617.
- Dhanraj S, Manji A, Pinto D, Scherer SW, Favre H, Loh ML, Chetty R, Wei AC, Dror Y. Molecular characteristics of a pancreatic adenocarcinoma associated with Shwachman-Diamond syndrome. Pediatr Blood Cancer. 2013; 60(5):754–60. doi:10.1002/pbc.24453. PMID:23303473.
- Gao G, Kasperbauer JL, Tombers NM, Cornell MD, Smith DI. Prognostic significance of decreased expression of six large common fragile site genes in oropharyngeal squamous cell carcinomas. Transl Oncol. 2014; 7(6):726–31. doi:10.1016/j.tranon.2014.09.009. PMID:25500082.
- He B, Li T, Guan L, Liu FE, Chen XM, Zhao J, Lin S, Liu ZZ, Zhang HQ. CTNNA3 is a tumor suppressor in hepatocellular carcinomas and is inhibited by miR-425. Oncotarget. 2016; 7(7):8078–89. doi:10.18632/oncotarget.6978. PMID:26882563.
- Liu P, Morrison C, Wang L, Xiong D, Vedell P, Cui P, Hua X, Ding F, Lu Y, James M, et al. Identification of somatic mutations in non-small cell lung carcinomas using whole-exome sequencing. Carcinogenesis. 2012; 33(7):1270–6. doi:10.1093/carcin/bgs148. PMID:22510280.
- Fanjul-Fernandez M, Quesada V, Cabanillas R, Cadiñanos J, Fontanil T, Obaya A, Ramsay AJ, Llorente JL, Astudillo A, Cal S, et al. Cell-cell adhesion genes CTNNA2 and CTNNA3 are tumour suppressors frequently mutated in laryngeal carcinomas. Nat Commun. 2013; 4:2531. doi:10.1038/ncomms3531. PMID:24100690.
- Hosking FJ, Feldman D, Bruchim R, Olver B, Lloyd A, Vijayakrishnan J, Flint-Richter P, Broderick P, Houlston RS, Sadetzki S. Search for inherited susceptibility to radiation-associated meningioma by genomewide SNP linkage disequilibrium mapping. Br J Cancer. 2011; 104(6):1049–54. doi:10.1038/bjc.2011.61. PMID:21364586.
- Stahn V, Nagel I, Fischer-Huchzermeyer S, Oyen F, Schneppenheim R, Gesk S, Bohring A, Chikobava L, Young P, Gess B, et al. Molecular Analysis of Hybrid Neurofibroma/Schwannoma Identifies Common Monosomy 22 and alpha-T-Catenin/CTNNA3 as a Novel Candidate Tumor Suppressor. Am J Pathol. 2016; 186(12):3285–96. doi:10.1016/j.ajpath.2016.08.019. PMID:27765635.
- Shibata H, Takano H, Ito M, Shioya H, Hirota M, Matsumoto H, Kakudo Y, Ishioka C, Akiyama T, Kanegae Y, et al. Alpha-catenin is essential in intestinal adenoma formation. Proc Natl Acad Sci U S A. 2007; 104(46):18199–204. doi:10.1073/pnas.0705730104. PMID:17989230.
- Zhao ZM, Reynolds AB, Gaucher EA. The evolutionary history of the catenin gene family during metazoan evolution. BMC Evol Biol. 2011; 11:198. doi:10.1186/1471-2148-11-198. PMID:21740572.
- Shapiro L, Weis WI. Structure and biochemistry of cadherins and catenins. Cold Spring Harb Perspect Biol. 2009; 1(3):a003053. doi:10.1101/cshperspect.a003053. PMID:20066110.
- McCrea PD, Gottardi CJ. Beyond beta-catenin: Prospects for a larger catenin network in the nucleus. Nat Rev Mol Cell Biol. 2016; 17(1):55–64. doi:10.1038/nrm.2015.3. PMID:26580716.