ABSTRACT
Tight junctions play key roles in the regulation of airway epithelial barrier function and promotion of tight junction integrity is beneficial to lung health. G-protein coupled receptor (GPR) 40 has been identified as a receptor of polyunsaturated fatty acids. This study aimed to investigate the function of GPR40 in regulating tight junction assembly in human airway epithelial cells (Calu-3 cells) using GW9508, a GPR40 agonist. Immunoblotting and immunofluorescence analyses showed that Calu-3 cells expressed both types of polyunsaturated fatty acid receptors including GPR40 and GPR120. Intracellular Ca2+ measurements confirmed that GW9508 stimulated GPR40, but not GPR120. In Ca2+ switch assays, GW9508 promoted the recovery of transepithelial electrical resistance and re-localization of zonula occludens (ZO)-1 to intercellular areas. These effects were suppressed by inhibitors of GPR40 and phospholipase C (PLC). Interestingly, GW9508 enhanced tight junction assembly in an AMP-activated protein kinase (AMPK)-dependent manner. The effect of GW9508 on inducing tight junction assembly was also confirmed in 16HBE14o- cells. Our results indicate that GPR40 stimulation by GW9508 leads to AMPK activation via calcium/calmodulin-dependent protein kinase kinase β (CaMKKβ). Collectively, this study reveals an unprecedented role of GPR40 in facilitating airway epithelial tight junction assembly via PLC-CaMKKβ-AMPK pathways. GPR40 represents a novel regulator of airway epithelial integrity and its stimulation may be beneficial in the treatment of airway diseases.
Introduction
Joining of adjacent airway epithelial cells by tight junctions serves as a physical barrier preventing entry of pathogens and noxious substances into lung tissues.Citation1 Tight junctions maintain polarity and integrity of airway epithelial cells, in which zonula occludens (ZO)-1, a membrane-anchoring component, plays crucial roles in tight junction formation and regulation of paracellular permeability.Citation2 Dysregulation of ZO-1 and tight junction complex is involved in the pathogenesis of airway disorders. Indeed, dislocalization of ZO-1, reduced expression of ZO-1 and increased paracellular permeability are correlated with the severity of chronic obstructive pulmonary disease (COPD).Citation3 Furthermore, several cytokines involved in allergic inflammation in asthma including interleukin (IL)-4, IL-13, interferon (IFN)-γ and tumor necrosis factor (TNF)-α suppress ZO-1 expression in airway epithelial cells.Citation4 Therefore, stabilization of tight junction complex is considered as a promising therapeutic approach to the treatment of airway diseases.Citation5
Recently, several studies have revealed the beneficial roles of polyunsaturated fatty acids (PUFA) ω–3 in modulating airway epithelial barrier integrity. For instance, plasma levels of ω–3 fatty acids in COPD and asthmatic patients are less than that in healthy individuals.Citation6,Citation7 Supplement of ω–3 prevents an increase in lung permeability and disruption of lung epithelial barrier both in vitro and in vivo models of acute lung injury through mechanisms involving inhibition of lipopolysaccharide-induced ZO-1 downregulation by ω–3 metabolites resolvin D1 and maresin 1.Citation8,Citation9 In contrast, some reports showed that PUFA induce incorporation of fatty acids into plasma membrane phospholipids, leading to a change in membrane properties, ZO-1 alteration and increased paracellular permeability in human airway epithelial (Calu-3) cells.Citation10 This controversy emphasizes the need to explore various mechanisms by which ω–3 modulates airway epithelial integrity.
GPR40 has recently been discovered as a receptor for PUFA including ω–3 fatty acids.Citation11,Citation12 GPR40 is expressed in several tissues including brain,Citation11,Citation13 intestine,Citation14 bone,Citation15 islet cells,Citation11 and lung.Citation16 Stimulation of GPR40 suppresses inflammation and inflammation-associated downregulation of tight junction proteins in intestinal epithelial cells.Citation17 GPR40 is coupled with Gq/11 and its stimulation leads to elevation in intracellular Ca2+ 11, 12, which can in turn activate AMPK through CaMKKβ.Citation18 AMPK activation is known to promote epithelial tight junction assembly.Citation19 Likewise, AMPK activation protects against inflammation-associated disruption of airway epithelial barrier.Citation20,Citation21 Intriguingly, stimulation of GPR40 activates AMPK in hepatocytes.Citation22 However, roles of GPR40 in regulating airway epithelial barrier integrity is largely unknown. We hypothesized that activation of GPR40 may promote tight junction assembly via AMPK activation in airway epithelial cells. Therefore, this study was performed to investigate the effect of GPR40 stimulation by GPR40 agonist GW9508 on tight junction assembly and its underlying mechanisms in human airway epithelial cells.
Results
Expression of GPR40 and specificity of GW9508 in Calu-3 cells
We first analyzed the specificity of GW9508 in stimulating GPR40 in Calu-3 cells, since GW9508 has been reported to stimulate GPR120 at higher concentrations.Citation23 As analyzed by western blotting and immunofluorescence staining, both GPR40 and GPR120 were expressed in Calu-3 cells (). Since both GPR40 and GPR120 were coupled to Gq/11-phospholipase C (PLC)-Ca2+ signaling, we investigated the target specificity of GW9508 in Calu-3 cells by determining the effect of GPR40 and GPR120 antagonists on GW9508-induced elevation of intracellular Ca2+ concentration ([Ca2+]i) using a ratiometric Ca2+ indicator indo-1. The ratio of emitted fluorescence at 405 nm (from Ca2+-bound indo-1) to 490 nm (from Ca2+-free indo-1) (indo-1 ratio) was used as an indicator of [Ca2+]i. As shown in , an addition of GW9508 (5 μM) caused an abrupt increase in [Ca2+]i compared to a vehicle-treated group. Pretreatment with DC260126 (3 μM), a GPR40 antagonist, completely abolished the effect of GW9508 on [Ca2+]i, whereas AH7614 (100 μM), a GPR120 antagonist, had no effect. These results indicate that GW9508 at 5 μM specifically activates GPR40 without stimulating GPR120 in Calu-3 cells. In addition, the GW9508-induced elevation of [Ca2+]i was inhibited by pretreatment with a PLC inhibitor U73122 (20 μM), confirming that GW9508 stimulated GPR40-PLC-Ca2+ pathway in Calu-3 cells.
Figure 1. Expression of GPR40 and GPR120 in airway epithelial Calu-3 cells. Western blot analysis and immunofluorescence staining of GPR40 and GPR120 in Calu-3 cells are shown (n = 3–4). GPR40 and GPR120 in green (Alexa 488), nuclear content in blue (Hoechst), BF is brightfield.
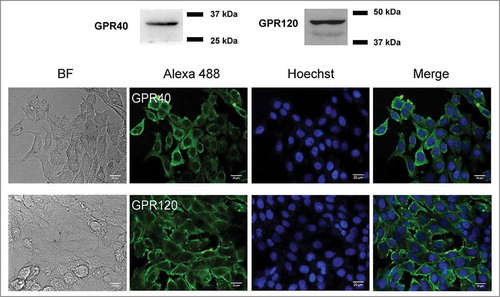
Figure 2. Effect of GPR40 on [Ca2+]i. Calu-3 cells were loaded with indo-1 and suspended in Ca2+-free buffers. After the indicated pretreatments (DC260126 (5 μM; GPR40 antagonist), AH7614 (100 μM; GPR120 antagonist), and U73122 (10 μM; PLC inhibitor) for an hour), GW9508 (5 μM) was added into bathing solutions during continual recording of indo-1 dual fluorescence (emitted at 405 nm and 490 nm). Representative tracings of indo-1 fluorescence ratio (F405/F490) and summary of the data are shown. Data are expressed as means of indo-1 fluorescence ratio (F405/F490) ±S.E.M. (n = 3–6). *** p < 0.001 compared with vehicle-treated group. ## p < 0.01; ### p < 0.001 compared with GW9508-treated group (one-way ANOVA).
![Figure 2. Effect of GPR40 on [Ca2+]i. Calu-3 cells were loaded with indo-1 and suspended in Ca2+-free buffers. After the indicated pretreatments (DC260126 (5 μM; GPR40 antagonist), AH7614 (100 μM; GPR120 antagonist), and U73122 (10 μM; PLC inhibitor) for an hour), GW9508 (5 μM) was added into bathing solutions during continual recording of indo-1 dual fluorescence (emitted at 405 nm and 490 nm). Representative tracings of indo-1 fluorescence ratio (F405/F490) and summary of the data are shown. Data are expressed as means of indo-1 fluorescence ratio (F405/F490) ±S.E.M. (n = 3–6). *** p < 0.001 compared with vehicle-treated group. ## p < 0.01; ### p < 0.001 compared with GW9508-treated group (one-way ANOVA).](/cms/asset/e2ed864c-cdd4-4dc5-9494-8eae11e6d468/ktib_a_1480741_f0002_b.gif)
Effect of GPR40 stimulation on tight junction assembly
To investigate the roles of GPR40 on facilitating tight junction assembly in airway epithelial cells, we induced disassembly of tight junctions by removing extracellular Ca2+ and investigated the effect of GW9508 on the rate of tight junction assembly induced by re-addition of Ca2+ (Ca2+ switch). Tight junction assembly was analyzed by both immunofluorescence staining of a tight junction protein ZO-1 and TER measurements. As shown in , after 16-h incubation in Ca2+-free media, ZO-1 was located in perinuclear regions in Calu-3 cells. In the presence of Ca2+, GW9508 (5 μM) enhanced re-assembly of ZO-1 to intercellular areas in a time-dependent manner. TER analysis demonstrated that culture in Ca2+-free media led to 50% decrease in TER of Calu-3 cell monolayers (). Treatment with GW9508 at 5 μM significantly promoted the recovery of TER at 24 h, whereas it had no effect on TER of intact monolayers (inset). Moreover, we found that GW9508 produced the similar effect on enhancing tight junction assembly in non-tumorigenic airway epithelial (16HBE14o-) cells, which also expressed both GPR40 and GPR120 (). Taken together, these results indicate that GW9508 enhances tight junction assembly in airway epithelial cells.
Figure 3. Effect of GW9508 on tight junction assembly. Cells were cultured for 16 h in Ca2+-free media. TER of Calu-3 and 16HBE14o- cell monolayers was then measured after replacement of Ca2+-free media with media containing Ca2+ plus GW9508 at the indicated concentrations. (A) Effect of GW9508 on localization of ZO-1 in Calu-3 cells. After the indicated durations of incubation, cells were fixed and immunostained for ZO-1 (green) and nuclear content was stained with Hoechst (blue) (n = 4). (B) Effect of GW9508 on TER in Calu-3 cells. (Inset) Effect of GW9508 on TER in intact monolayers. (C) Expression of GPR40 and effect of GW9508 on TER in 16HBE14o- cells. After the indicated durations of incubation, TER was measured. Data are expressed as means of % of baseline TER ± S.E.M (n = 4–6). ** p < 0.01; *** p < 0.001 compared with vehicle-treated group (two-way ANOVA).
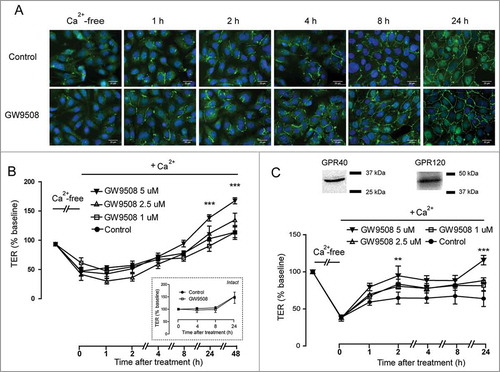
Involvement of GPR40 and PLC in GW9508-induced tight junction assembly
To confirm that the effect of GW9508 on promoting tight junction assembly was via GPR40, Calu-3 cells were pretreated for an hour with specific GPR40 antagonists including DC260126 and GW1100 before addition of GW9508. As shown in , both DC260126 and GW1100 (3 μM) pretreatment significantly prevented the effect of GW9508 on promoting tight junction assembly. In addition, we investigated an involvement of PLC in mediating the effect of GW9508 on tight junction assembly using U73122, a PLC inhibitor. showed that the effect of GW9508 on tight junction assembly was completely abolished by U73122 pretreatment. These results indicate that GPR40 stimulation by GW9508 induces tight junction assembly in Calu-3 cells via a PLC-dependent pathway.
Figure 4. Roles of GPR40 and PLC in mediating GW9508-induced tight junction assembly. Cells were cultured for 16 h in Ca2+-free media. TER of Calu-3 cell monolayers was then measured after replacement of Ca2+-free media with the media containing Ca2+ plus vehicle or GW9508 (5 μM). (A) Role of GPR40. Cells were pretreated with GPR40 antagonists DC260126 (3 μM) or GW1100 (3 μM). (B) Role of PLC. Cells were pretreated with PLC inhibitor U73122 (20 μM). Data are expressed as means of % baseline TER ± S.E.M (n = 6). *** p < 0.001 compared with vehicle-treated group. # p < 0.05; ### p < 0.001 compared with GW9508-treated group. § p < 0.05; §§§ p < 0.001 compared between GW9508-treated and GW9508 + GW1100-treated groups (two-way ANOVA).
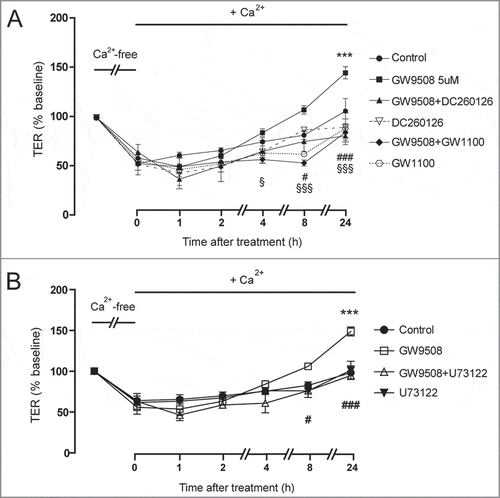
AMPK mediates the effect of GPR40 stimulation on tight junction assembly
AMPK has been shown to promote tight junction assembly in epithelial cells.Citation19 Next, we investigated an involvement of AMPK in mediating the effect of GPR40 stimulation by GW9508 on tight junction assembly. We first compared the effect of GW9508 to metformin, a known AMPK activator. As shown in , TER changes induced by metformin (1.5 mM) was comparable to that induced by GW9508 at 0–8 h. Pretreatment with compound C (40 μM), an AMPK inhibitor, completely abolished the effect of GW9508 on tight junction assembly at 8 h and 24 h post-treatment in Calu-3 cells ( and ) and in 16HBE14o- cells (). These results indicate that GPR40 stimulation by GW9508 induces tight junction assembly via an AMPK-dependent mechanism.
Figure 5. Involvement of AMPK in GPR40 stimulation-induced tight junction assembly. (A) Effect of metformin on tight junction assembly in Calu-3 cells. Cells were cultured for 16 hours in Ca2+-free media. TER of Calu-3 cells monolayers was then measured after replacement of Ca2+-free media with media containing Ca2+ plus vehicle, GW9508 (5 μM), or metformin (1.5 mM). Data are expressed as means of % baseline TER ± S.E.M (n = 4). ** p < 0.01; *** p < 0.001 compared between vehicle-treated and GW9508-treated groups. # p < 0.05 compared between vehicle-treated and metformin-treated groups (two-way ANOVA). (B) Involvement of AMPK in GW9508-induced tight junction assembly in Calu-3 cells. In the GW9508-treated group, cells were pretreated with vehicle or compound C (40 μM). Data are expressed as means of % baseline TER±S.E.M (n = 6). * p < 0.05; *** p < 0.001 compared with vehicle-treated group. ### p < 0.001 compared with GW9508-treated group (two-way ANOVA). (C) Summary of data at 8 h and 24 h. ** p < 0.05; *** p < 0.001 (one-way ANOVA). (D) Involvement of AMPK in GW9508-induced tight junction assembly in 16HBE14o- cells. * p < 0.05; ** p < 0.01 compared with vehicle-treated group. # p < 0.05; ## p < 0.01; ### p<0.001 compared with GW9508-treated group (two-way ANOVA).
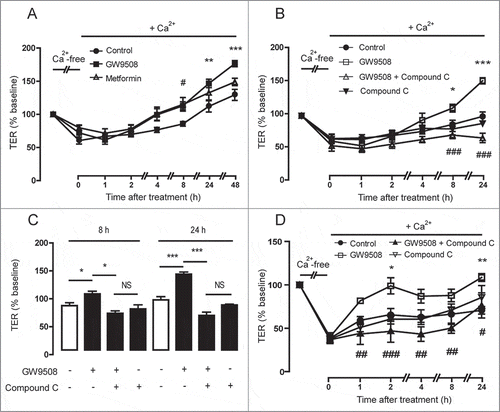
AMPK activation by GPR40 agonist
To test whether GPR40 stimulated AMPK, western blot analyses of AMPK phosphorylation at Thr-172 in Calu-3 cells were performed. We found that GW9508 treatment induced AMPK phosphorylation in time and dose-dependent manners ( and ). Since treatment with GW9508 at 5 μM produced significant AMPK phosphorylation at 24 h post-treatment (), this treatment condition was used in subsequent experiments exploring mechanisms of GW9508-induced AMPK activation. Interestingly, AMPK phosphorylation induced by GW9508 was significantly inhibited by pretreatment with GPR40 antagonist DC2600126 (), but not GPR120 antagonist AH-7614 (). These data indicate that GW9508 activates AMPK via a GPR40-dependent mechanism.
Figure 6. GPR40 stimulation by GW9508 leads to AMPK activation. Western blot analyses of p-AMPK, AMPKα, and β-actin after treatment of Calu-3 cells with GW9508 at various times (A) and doses (B). Calu-3 cells were treated with GW9508 (5 μM) without or with DC260126 (3 μM) (C) and AH7614 (100 μM) (D) for 24 h before sample collection for western blot analysis. Data are expressed as mean of ratio of control (vehicle-treated group) ± S.E.M. (n = 4–5). * p < 0.05 compared with vehicle-treated group. ## p < 0.01 compared with GW9508-treated group (one-way ANOVA).
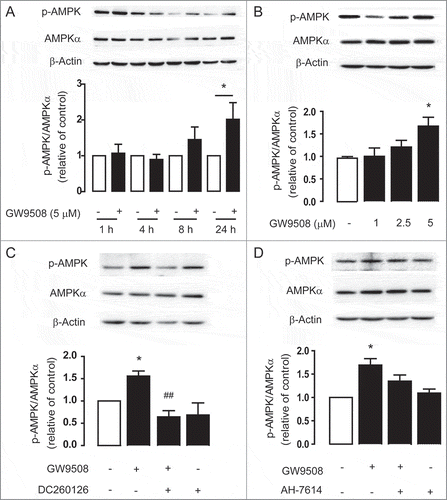
Because GW9508 induced an increase in [Ca2+]i, which is known to stimulate AMPK via CaMKKβ, we hypothesized that GPR40 stimulation by GW9508 may activate AMPK and tight junction assembly via CaMKKβ. demonstrated that pretreatment with CaMKKβ inhibitor STO-609 completely abolished the effect of GW9508 on AMPK phosphorylation. Furthermore, TER measurements showed that pretreatment with STO-609 significantly prevented the effect of GW9508 on tight junction assembly (). These results indicate that CaMKKβ mediates the effect of GPR40 stimulation by GW9508 on both AMPK activation and tight junction assembly in Calu-3 cells.
Figure 7. Involvement of CaMKKβ in AMPK activation following GPR40 stimulation. (A) Role of CaMKKβ in AMPK activation. Calu-3 cells were treated for 24 h with vehicle or GW9508 (5 μM) without or with pretreatment with CaMKKβ inhibitor STO-609 (5 μM) before sample collection for western blot analysis. Data are expressed as ratio of control (vehicle-treated group) ± S.E.M. (n = 4–5). * p < 0.05 compared with vehicle-treated group. # p < 0.05 compared with GW9508-treated group (one-way ANOVA). (B) Role of CaMKKβ in GPR40 stimulation-induced tight junction assembly. Cells were cultured for 16 h in Ca2+-free media. TER of Calu-3 cell monolayers was then measured after replacement of Ca2+-free media with media containing Ca2+ plus vehicle or GW9508 (5 μM) with or without pretreatment with STO-609 (5 μM). Data are expressed as means of % baseline TER ± S.E.M (n = 6). * p < 0.05; ** p < 0.01; *** p < 0.001 compared with vehicle-treated group. ## p<0.01 compared with GW9508-treated group (two-way ANOVA).
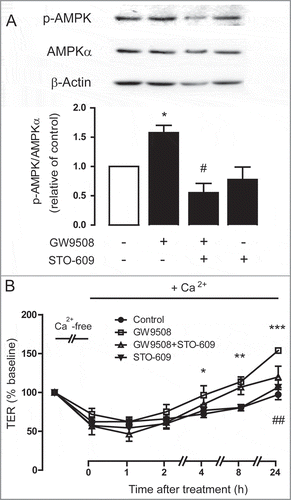
Discussion
In addition to their beneficial roles in alleviating abnormal metabolism and inflammation in various cell types,Citation12,Citation14,Citation15,Citation22,Citation24 GPR40 was demonstrated for the first time in this study to play an important role in regulation of epithelial barrier in airway epithelial cells. The mechanism by which GPR40 stimulation induced tight junction assembly involved AMPK activation via PLC-Ca2+- CaMKKβ pathway.
Dysregulation of tight junction has been implicated in the pathogenesis of airway diseases.Citation4,Citation5 In the present study, we demonstrated functional expression of GPR40 and found that GPR40 activation led to enhanced barrier function in human airway epithelial cells. Interestingly, AMPK is activated in response to GPR40 stimulation, resulting in the assembly of tight junction protein ZO-1. AMPK is well known to promote tight junction assembly following the exposure to extracellular Ca2+. For example, in MDCK cells, phosphorylated AMPK level was corresponded with the Ca2+-switch condition and led to the recovery of TER and reassembly of ZO-1. Further, the rate of tight junction recovery could be accelerated by an AMPK activator AICAR.Citation25 Similar effects have also been observed in intestinal epithelial cells. Treatment with dietary compounds that activate AMPK including butyrateCitation26 and chitosan oligosaccharideCitation27 led to the enhancement of tight junction assembly in Caco-2 and T84 cells, respectively. These effects were blunted by pretreatment with compound C, an AMPK inhibitor.Citation26,Citation27 In the present study, an AMPK activator metformin promoted tight junction assembly in Calu-3 cells. Indeed, metformin has previously been shown to increase tight junction integrity in Calu-3 cells and reduce permeability of glucose across airway epithelial cells resulting in the limitation of Staphylococcus aureus growth in murine tracheas.Citation20,Citation21 However, it should be aware that metformin may exert these effects via AMPK-independent mechanisms. Importantly, we found that the time course of GPR40 stimulation-induced AMPK phosphorylation was corresponded with that of ZO-1 localization and a change in TER. Taken together, these results indicate that GPR40 stimulation leads to AMPK activation and, consequently, tight junction assembly in airway epithelial cells.
In this study, a mechanism of GPR40 stimulation-induced tight junction assembly was also explored using both Ca2+-switch assays and immunoblotting analyses. GPR40 has previously been reported as a Gq/11-coupled receptor, whose activation results in increased [Ca2+]i and PLC activation.Citation28,Citation29 Herein, GPR40 stimulation by GW9508 was verified by measuring [Ca2+]i. In addition, a PLC inhibitor and GPR40 antagonists reversed the effects of GW9508 on tight junction assembly. These results suggest that GPR40 stimulation transduce through Gq/11 –PLC-Ca2+ pathways. Nevertheless, accumulated lines of evidence have revealed that engagement of Gq/11-coupled receptors leads to AMPK activation via CaMKKβ.Citation30 Indeed, we have previously found that stimulation of Ca2+-sensing receptor, a Gq/11-coupling receptor, leads to AMPK activation via a PLC-Ca2+-CaMKKβ-dependent mechanism in intestinal epithelial cells. Similarly, it has been reported that GW9508 induces AMPK phosphorylation in hepatocytes via a GPR40-Gq-CaMKKβ pathway.Citation22 These lines of evidence support our findings that GPR40 stimulation results in AMPK activation via Gq-PLC-Ca2+-CaMKKβ pathways.
Even though previous studies have reported that GW9508 stimulates both GPR40 and GPR120.Citation23 Using a GPR40 antagonist and a GPR120 antagonist we showed that GW9508 stimulated an increase in [Ca2+]i and AMPK activity via GPR40 but not GPR120 in Calu-3 cells, which express both types of receptors. This functional bias of GW9508 toward the agonism of GPR40 has previously been reported in other studies.Citation31,Citation32 Moreover, the sensitivity of GPR40 for GW9508 (pEC50 ≈ 7.3) is about 50-fold higher than that of GPR120 (pEC50 ≈ 5.4) in GPR40 or GPR120 transfected cells.Citation23 Further studies using a gene silencing approach are required to confirm the contribution of GPR40 to the tight junction-enhancing effect of GW9508.
In contrast to our results, previous studies have identified physiological roles of PUFA ω–3 fatty acids, endogenous agonists of GPR40, in increasing permeability of epithelial cells. Administration of PUFA led to an increase in paracellular permeability, disruption in ZO-1 localization and a decline in TER in Calu-3 cells.Citation10 Likewise, ω–3 fatty acids enhanced sulfonic acid permeability and lowered TER in Caco-2 cells via undefined mechanisms.Citation33,Citation34 These contradictory results of endogenous and exogenous agonists of GPR40 may be because ω–3 fatty acids induce additional signaling cascades independent of GPR40.Citation35 For example, ω–3 fatty acids can be incorporated into plasma membrane, leading to a change in plasma membrane biophysical properties and signal transduction in lipid rafts, where tight junctions are embedded.Citation35,Citation36
In conclusion, we identified an unprecedented effect of GPR40 stimulation on tight junction assembly in airway epithelial cells. Our results indicate that the mechanism of GPR40 stimulation-induced tight junction assembly is via PLC-Ca2+-CaMKKβ-AMPK pathways. These findings suggest that GPR40 represents an important regulator of airway barrier integrity and may be a promising therapeutic target for the treatment of airway diseases. Future studies should be performed to investigate the functional role and expression of GPR40 in airway diseases including COPD.
Material and methods
Material
Calu-3 cells were obtained from the American Type Culture Collection (Manassas, VA, USA). 16HBE14o- cells were kindly provided by Dr. Nawiya Huipao (Prince of Songkla University, Hat Yai, Thailand). GW9508, DC260126, GW1100, U73122, STO-609, compound C and metformin hydrochloride were purchased from Sigma-Aldrich (Saint Louis, MO, USA). AH7614 was form Tochis Bioscience (Bristol, UK).
Cell culture and Ca2+-switch assay
Calu-3 cells were cultured in an 1:1 mixture of Dulbecco's modified Eagle's medium (DMEM) and Ham's F-12 medium. 16HBE14o- cells were cultured in a minimum essential medium (MEM). Both types of medium were supplemented with 1% non-essential amino acid, 10% fetal bovine serum (FBS), 100 U/mL penicillin and 100 mg/mL streptomycin (Life Technologies, Carlsbad, CA, USA). Cells were maintained in a humidified 95% O2/5% CO2 atmosphere at 37°C.
Calu-3 and 16HBE14o- cells from passage 25–35 were seeded on Transwell permeable supports (2.5 × 105 cells/well) (Corning Life Sciences, Tewksbury, MA, USA) and cultured for 14 days (TER ≥ 900 Ω.cmCitation2). After measuring baseline TER, culture media were replaced with the Minimum Essential Medium Eagle, Spinner Modification (S-MEM) Ca2+-free culture media to induce tight junction disassembly. Sixteen h later, the S-MEM medium was replaced with FBS-free culture medium containing Ca2+supplemented with vehicle, GW9508, or GW9508 plus pharmacological inhibitors. TER was measured before and after treatment (at 1 h, 2 h, 4 h, 8 h, 24 h and 48 h) using an EVOM2 volt/ohm meter (World Precision Instruments, Inc., Sarasota, FL, USA).
Immunocytochemistry
Calu-3 cells were seeded in 96-well plates (5 × 104 cells/well) and cultured for 5 days. After Ca2+ switch, cells were washed with phosphate-buffered saline (PBS). Fixation was performed by 20-min incubation with –20°C absolute ethanol followed by 30-min incubation with 5% bovine serum albumin (BSA). Then, cells were stained overnight with anti-GPR40 (catalog number ab211049; lot GR252665-1) or anti-GPR120 (catalog number ab118757; lot GR26398-33) primary antibodies (Abcam, Cambridge, MA, USA) and conjugated with anti-rabbit IgG Alexa fluor 488 (Thermo Fisher Scientific Inc, MA, USA; catalog number A11034; lot 1670152). For ZO-1 staining, mouse anti-ZO-1 antibody (Invitrogen, California, United States; catalog number 339100; lot 1100420A) and anti-mouse IgG Alexa fluor 488 (Thermo Fisher Scientific Inc, MA, USA; catalog number A11001; lot 1834337) were used. Images were captured using Operetta High-Content Imaging System (PerkinElmer, MA, USA).
Western blot analysis
Cells were plated on 6-well plates at a density of 5 × 105 cells/mL (Corning Life Sciences, Tewksbury, MA, USA). Protein extraction and immunoblotting protocols were performed as previously described.Citation27 Briefly, after treatments, cell lysates were harvested using RIPA lysis buffer. Proteins were separated using sodium dodecyl sulfate polyacrylamide gel electrophoresis (SDS-PAGE) before transferring to a nitrocellulose membrane. The membrane was blocked for an hour with 5% non-fat dried milk (Bio-Rad, Hercules, CA, USA), and incubated overnight with rabbit antibodies to phospho-AMPK (Thr-172) (catalog number 2531S; lot 15), AMPKα (catalog number 2532S; lot 19), or β-actin (catalog number 4970S; lot 14) (Cell Signaling Technology, Boston, MA, USA). The membrane was then washed for 4 times with Tris-Buffered Saline Tween-20 (TBST) and incubated for an hour at room temperature with 2.5% non-fat dried milk plus horseradish peroxidase conjugated goat antibody to rabbit immunoglobulin G (Abcam, Cambridge, MA, USA; catalog number ab6721; lot GR194011-1). The signal was detected using Luminata Forte' Western HRP Substrate (Merck Millipore, Billerica, MA, USA) captured by Omega Lum G Imaging System (Aplegen, San Francisco, CA). Densitometry analysis was performed using Image J software (version 1.51).
Intracellular calcium measurement
Suspended Calu-3 cells were harvested and incubated for an hour at 37°C with 10 μM indo-1 (Life Technologies, Carlsbad, CA, USA). Cells were then washed with fresh buffer containing 0.441 mM KH2PO4, 5.33 mM KCl, 4.17 mM NaHCO3, 5.56 mM D-glucose, 137.93 mM NaCl, 0.338 mM Na2HPO4, 1 mM CaCl2 and 1% (w/v) BSA. An hour prior to the experiment, Calu-3 cells were pretreated with or without pharmacological inhibitors. The mean fluorescence intensity ratio between Ca2+-bound indo-1 (excitation wavelength of 338 nm, emission wavelength of 405 nm) and Ca2+-free indo-1 (excitation wavelength of 338 nm, emission wavelength of 490 nm) was detected by an FP-6200 spectrofluorometer (JASCO, Essex, UK).
Statistical analysis
Results are presented as means ±S.E.M. One-way ANOVA with tukey's post test was performed for multiple comparison. Two-way ANOVA followed by Bonferroni's post hoc test was used for multiple comparison data in time series. p value <0.05 was considered statistically significant.
Disclosure of potential conflicts of interest
No potential conflicts of interest were disclosed.
Additional information
Funding
References
- Ganesan S, Comstock AT, Sajjan US. Barrier function of airway tract epithelium. Tissue Barriers. 2013;1:e24997. doi:10.4161/tisb.24997.
- Shen L, Weber CR, Raleigh DR, Yu D, Turner JR. Tight junction pore and leak pathways: a dynamic duo. Annual Rev Physiol. 2011;73:283–309. doi:10.1146/annurev-physiol-012110-142150.
- Heijink IH, Noordhoek JA, Timens W, van Oosterhout AJ, Postma DS. Abnormalities in airway epithelial junction formation in chronic obstructive pulmonary disease. Am J Respiratory Critical Care Med. 2014;189:1439–42. doi:10.1164/rccm.201311-1982LE.
- Georas SN, Rezaee F. Epithelial barrier function: at the front line of asthma immunology and allergic airway inflammation. J Allergy Clin Immunol. 2014;134:509–20. doi:10.1016/j.jaci.2014.05.049.
- Wittekindt OH. Tight junctions in pulmonary epithelia during lung inflammation. Pflugers Archiv: Eur J Physiol. 2017;469:135–47. doi:10.1007/s00424-016-1917-3.
- Shahar E, Boland LL, Folsom AR, Tockman MS, McGovern PG, Eckfeldt JH. Docosahexaenoic acid and smoking-related chronic obstructive pulmonary disease. The atherosclerosis risk in communities study investigators. Am J Respir Critical Care Med. 1999;159:1780–5. doi:10.1164/ajrccm.159.6.9810068.
- Nagakura T, Matsuda S, Shichijyo K, Sugimoto H, Hata K. Dietary supplementation with fish oil rich in omega-3 polyunsaturated fatty acids in children with bronchial asthma. Eur Respir J. 2000;16:861–5. doi:10.1183/09031936.00.16586100.
- Chen L, Liu H, Wang Y, Xia H, Gong J, Li B, Yao S, Shang Y. Maresin 1 maintains the permeability of lung epithelial cells in vitro and in vivo. Inflammation. 2016;39:1981–9. doi:10.1007/s10753-016-0433-0.
- Xie W, Wang H, Wang L, Yao C, Yuan R, Wu Q. Resolvin D1 reduces deterioration of tight junction proteins by upregulating HO-1 in LPS-induced mice. Lab Invest J Technical Methods Pathol. 2013;93:991–1000. doi:10.1038/labinvest.2013.80.
- Ghadiri M, Mamlouk M, Spicer P, Jarolimek W, Grau GE, Young PM, Traini D. Effect of polyunsaturated fatty acids (PUFAs) on airway epithelial cells' tight junction. Pulmonary Pharmacol Therapeutics. 2016;40:30–8. doi:10.1016/j.pupt.2016.07.004.
- Briscoe CP, Tadayyon M, Andrews JL, Benson WG, Chambers JK, Eilert MM, Ellis C, Elshourbagy NA, Goetz AS, Minnick DT, et al. The orphan G protein-coupled receptor GPR40 is activated by medium and long chain fatty acids. J Biol Chem. 2003;278:11303–11. doi:10.1074/jbc.M211495200.
- Itoh Y, Kawamata Y, Harada M, Kobayashi M, Fujii R, Fukusumi S, Ogi K, Hosoya M, Tanaka Y, Uejima H, et al. Free fatty acids regulate insulin secretion from pancreatic beta cells through GPR40. Nature. 2003;422:173–6. doi:10.1038/nature01478.
- Nakamoto K, Nishinaka T, Sato N, Mankura M, Koyama Y, Kasuya F, Tokuyama S. Hypothalamic GPR40 signaling activated by free long chain fatty acids suppresses CFA-induced inflammatory chronic pain. Plos One. 2013;8:e81563. doi:10.1371/journal.pone.0081563.
- Edfalk S, Steneberg P, Edlund H. Gpr40 is expressed in enteroendocrine cells and mediates free fatty acid stimulation of incretin secretion. Diabetes. 2008;57:2280–7. doi:10.2337/db08-0307.
- Wauquier F, Philippe C, Leotoing L, Mercier S, Davicco MJ, Lebecque P, Guicheux J, Pilet P, Miot-Noirault E, Poitout V, et al. The free fatty acid receptor G protein-coupled receptor 40 (GPR40) protects from bone loss through inhibition of osteoclast differentiation. J Biol Chem. 2013;288:6542–51. doi:10.1074/jbc.M112.429084.
- Gras D, Chanez P, Urbach V, Vachier I, Godard P, Bonnans C. Thiazolidinediones induce proliferation of human bronchial epithelial cells through the GPR40 receptor. Am J Physiol Lung Cell Mol Physiol. 2009;296:L970–8. doi:10.1152/ajplung.90219.2008.
- Miyamoto J, Mizukure T, Park SB, Kishino S, Kimura I, Hirano K, Bergamo P, Rossi M, Suzuki T, Arita M, et al. A gut microbial metabolite of linoleic acid, 10-hydroxy-cis-12-octadecenoic acid, ameliorates intestinal epithelial barrier impairment partially via GPR40-MEK-ERK pathway. J Biol Chem. 2015;290:2902–18. doi:10.1074/jbc.M114.610733.
- Woods A, Dickerson K, Heath R, Hong SP, Momcilovic M, Johnstone SR, Carlson M, Carling D. Ca2+/calmodulin-dependent protein kinase kinase-beta acts upstream of AMP-activated protein kinase in mammalian cells. Cell Metab. 2005;2:21–33. doi:10.1016/j.cmet.2005.06.005.
- Zhang L, Li J, Young LH, Caplan MJ. AMP-activated protein kinase regulates the assembly of epithelial tight junctions. Proc Natl Acad Sci U S A. 2006;103:17272–7. doi:10.1073/pnas.0608531103.
- Garnett JP, Baker EH, Naik S, Lindsay JA, Knight GM, Gill S, Tregoning JS, Baines DL. Metformin reduces airway glucose permeability and hyperglycaemia-induced Staphylococcus aureus load independently of effects on blood glucose. Thorax. 2013;68:835–45. doi:10.1136/thoraxjnl-2012-203178.
- Patkee WR, Carr G, Baker EH, Baines DL, Garnett JP. Metformin prevents the effects of Pseudomonas aeruginosa on airway epithelial tight junctions and restricts hyperglycaemia-induced bacterial growth. J Cell Mol Med. 2016;20:758–64. doi:10.1111/jcmm.12784.
- Li M, Meng X, Xu J, Huang X, Li H, Li G, Wang S, Man Y, Tang W, Li J. GPR40 agonist ameliorates liver X receptor-induced lipid accumulation in liver by activating AMPK pathway. Scientific Reports. 2016;6:25237. doi:10.1038/srep25237.
- Briscoe CP, Peat AJ, McKeown SC, Corbett DF, Goetz AS, Littleton TR, McCoy DC, Kenakin TP, Andrews JL, Ammala C, et al. Pharmacological regulation of insulin secretion in MIN6 cells through the fatty acid receptor GPR40: identification of agonist and antagonist small molecules. Br J Pharmacol. 2006;148:619–28. doi:10.1038/sj.bjp.0706770.
- Syed I, Lee J, Moraes-Vieira PM, Donaldson CJ, Sontheimer A, Aryal P, Wellenstein K, Kolar MJ, Nelson AT, Siegel D, et al. Palmitic acid hydroxystearic acids activate GPR40, which is involved in their beneficial effects on glucose homeostasis. Cell Metab. 2018;27:419-27 e4. doi:10.1016/j.cmet.2018.01.001.
- Zheng B, Cantley LC. Regulation of epithelial tight junction assembly and disassembly by AMP-activated protein kinase. Proc Natl Acad Sci U S A. 2007;104:819–22. doi:10.1073/pnas.0610157104.
- Peng L, Li ZR, Green RS, Holzman IR, Lin J. Butyrate enhances the intestinal barrier by facilitating tight junction assembly via activation of AMP-activated protein kinase in Caco-2 cell monolayers. J Nutr. 2009;139:1619–25. doi:10.3945/jn.109.104638.
- Muanprasat C, Wongkrasant P, Satitsri S, Moonwiriyakit A, Pongkorpsakol P, Mattaveewong T, Pichyangkura R, Chatsudthipong V. Activation of AMPK by chitosan oligosaccharide in intestinal epithelial cells: Mechanism of action and potential applications in intestinal disorders. Biochem Pharmacol. 2015;96:225–36. doi:10.1016/j.bcp.2015.05.016.
- Suh HN, Huong HT, Song CH, Lee JH, Han HJ. Linoleic acid stimulates gluconeogenesis via Ca2+/PLC, cPLA2, and PPAR pathways through GPR40 in primary cultured chicken hepatocytes. Am J Physiol Cell Physiol. 2008;295:C1518–27. doi:10.1152/ajpcell.00368.2008.
- Fujiwara K, Maekawa F, Yada T. Oleic acid interacts with GPR40 to induce Ca2+ signaling in rat islet beta-cells: mediation by PLC and L-type Ca2+ channel and link to insulin release. Am J Physiol Endocrinol Metab. 2005;289:E670–7. doi:10.1152/ajpendo.00035.2005.
- Hutchinson DS, Summers RJ, Bengtsson T. Regulation of AMP-activated protein kinase activity by G-protein coupled receptors: potential utility in treatment of diabetes and heart disease. Pharmacol Ther. 2008;119:291–310. doi:10.1016/j.pharmthera.2008.05.008.
- Mizuta K, Zhang Y, Mizuta F, Hoshijima H, Shiga T, Masaki E, Emala CW Sr. Novel identification of the free fatty acid receptor FFAR1 that promotes contraction in airway smooth muscle. Am J Physiol Lung Cell Mol Physiol. 2015;309:L970–82. doi:10.1152/ajplung.00041.2015.
- Puebla C, Cisterna BA, Salas DP, Delgado-Lopez F, Lampe PD, Saez JC. Linoleic acid permeabilizes gastric epithelial cells by increasing connexin 43 levels in the cell membrane via a GPR40- and Akt-dependent mechanism. Biochim Biophys Acta. 2016;1861:439–48. doi:10.1016/j.bbalip.2016.02.002.
- Beguin P, Errachid A, Larondelle Y, Schneider YJ. Effect of polyunsaturated fatty acids on tight junctions in a model of the human intestinal epithelium under normal and inflammatory conditions. Food Funct. 2013;4:923–31. doi:10.1039/c3fo60036j.
- Usami M, Komurasaki T, Hanada A, Kinoshita K, Ohata A. Effect of gamma-linolenic acid or docosahexaenoic acid on tight junction permeability in intestinal monolayer cells and their mechanism by protein kinase C activation and/or eicosanoid formation. Nutrition. 2003;19:150–6. doi:10.1016/S0899-9007(02)00927-9.
- Calder PC. Mechanisms of action of (n-3) fatty acids. J Nutr. 2012;142:592S–9S. doi:10.3945/jn.111.155259.
- Nusrat A, Parkos CA, Verkade P, Foley CS, Liang TW, Innis-Whitehouse W, Eastburn KK, Madara JL. Tight junctions are membrane microdomains. J Cell Sci. 2000;113(Pt 10):1771–81.