ABSTRACT
Occludin, a tight junction protein, has been reported to regulate barrier function – particularly the leak pathway for larger solutes – in epithelia. Therefore, we aimed to precisely define its role in macromolecule passage at single cell–cell junctions. A combination of varying occludin expression by transient and stable knockdown including systematic seeding strategies was employed to achieve a broad and defined pattern of variance in occludin expression over epithelia. This variance model enabled us to examine occludin function in the leak pathway using global and local analysis, i.e. to analyze macromolecule flux across epithelia and macromolecule passage at single-cell level. Macromolecular flux was found not to correlate with occludin expression in intestinal epithelial cells. In fact, by spatially resolving macromolecular permeation sites using a recently developed method we uncovered leaky cell junctions at the edge of Transwells resulting in increased passage. This demonstrates that rare leaks can determine net flux of macromolecules across epithelia while the vast majority of cellular junctions do not contribute significantly. Hence, concomitant local analysis of macromolecule passage across epithelial barriers is indispensable for interpretation of global flux data. By combining this new approach with cell culture models of the leak pathway, we can present evidence that lack of occludin is not sufficient to stimulate the epithelial leak pathway.
Introduction
Epithelial sheets, layers of interconnected cells, separate internal and external compartments in the body. This separation is largely brought about by intercellular junctions (tight and adherence junctions; TJ and AJ) with TJs sealing the space between neighboring cells thereby restricting the paracellular passage pathway. A second transepithelial route is the transcellular pathway absorbing most nutrients as they travel along in the gut. Epithelia have two critical functions: (i) they selectively regulate absorption and secretion of molecules between compartments and (ii) as a barrier they prevent penetration of potentially harmful entities. Tight junctions seal the paracellular space. Hence, they regulate solute/ion absorption and secretion.Citation1,Citation2 This barrier also restricts the passage of larger solutes, which is important in diseases where this discriminative function of epithelia fails.Citation3,Citation4 A basal paracellular passage pathway is assumed to exist for larger solutes (>4 Å),Citation1,Citation5 and this leak pathway is believed to be dysregulated in diseases such as inflammatory bowel disease (IBD) or celiac disease (CD), or possibly even to contribute to their induction.Citation3,Citation6–Citation8 It has been a long-standing issue to get behind the cellular and molecular mechanisms of the leak pathway with occludin, as one of the tight junction proteins, being suggested to regulate it.Citation9–Citation15 Partly on this account, occludin is considered a key marker of epithelial barrier function.
Occludin function in epithelia
Many studies reported changes in barrier function alongside changes in occludin localization, expression and posttranslational modification (e.g. phosphorylation, ubiquitination). Occludin has also been associated with many other aspects of epithelial physiology: recent studies related occludin mutations to brain calcification and renal dysfunction.Citation16,Citation17 Though mice deficient in occludin lack obvious defects in barrier function according to earlier studies,Citation18,Citation19 they suffer from deafness as recently reported.Citation20 With no indication of a direct effect on permeability, occludin was reported to regulate TJs and/or TJ assemblyCitation21 and disassembly of the apical junctional complex,Citation22 as well as lumen formation in 3D-culture.Citation23 Based on studies in different epithelial cells, occludin seems to play a role in epithelial wound healing,Citation24 apoptosisCitation25 and to be important to the interaction with intraepithelial lymphocytes.Citation26 Studies addressing occludin’s role in barrier function have suggested involvement in serine protease-dependent barrier modulation,Citation27 hypoxia-induced,Citation28 ethanol-inducedCitation29 and hydrogen peroxide–induced reduction in barrier function.Citation30,Citation31
Occludin and the epithelial leak pathway
Interestingly, several studies observed no effect of occludin expression on basal flux of solutes across epithelia.Citation11,Citation13 McCarthy and colleagues showed that overexpression of occludin in MDCK cells increased the flux of small solutes,Citation32,Citation33 which was refined by Balda and colleagues. According to their study, deletion of extracellular loops or the transmembrane domain of occludin reduced tracer flux while overexpression of wildtype occludin increased it.Citation34
Considerably fewer studies addressed the question if such changes in occludin are sufficient to directly provoke changes in basal barrier function, particularly towards macromolecular tracers. Al-Sadi and coworkers reported increased passage of dextrans of different sizes through Caco-2 epithelia transiently transfected with occludin-targeting siRNA. These epithelia proved unaffected in terms of transepithelial electrical resistance (TER) and the expression of other tight junction proteins, except for claudin-2 that had gone up massively.Citation14 Buschmann et al. also reported increased passage of different sizes of dextrans in CacoBBe cells stably transfected with occludin-targeting shRNA. In these cells, occludin expression was strongly reduced and TER decreased while expression of other tight junction proteins was only slightly affected. However, contrary to the aforementioned study – claudin-2 expression was found to be reduced in these cells.Citation15 Though Yu et al. and VanItallie et al. found no correlation between occludin deficiency and basal flux of macromolecular tracers in MDCK cells they reported a different response to cytokines or to cell physiology-affecting reagents that scale in line with the occludin level in these cells.Citation11,Citation13 Similar findings were reported in mice showing that cytokine-induced barrier defects depend on occludin.Citation35 The same was reported for the abovementioned CacoBBe cells stably transfected with occludin-targeting shRNA.Citation15 This suggested that occludin is involved in the response of the epithelial barrier to perturbations rather than in regulating basal flux.
To decipher occludin’s contribution to the basal leak pathway, we characterized the barrier function in epithelial layers expressing varying levels of occludin. In the present study, we did not observe an increase in macromolecule flux in occludin-depleted Caco cells as reported by Al-Sadi and colleagues. This was substantiated by similar findings in T84 epithelia. By analyzing the cells generated by Buschmann and colleagues, we reproduced their results; but, using a combination of globally measured tracer flux and locally resolved tracer passage, we can clearly show that the increase in permeability in these cells is independent of occludin.
Results
Transient knockdown of occludin does not increase passage of macromolecules in epithelia
To visualize and localize macromolecule passage in the absence of occludin, CacoBBe cells were transiently transfected with siRNA targeting occludin and were grown on Transwells for 7–8 days. The epithelia were analyzed for apical-to-basal macromolecule passage using FITC-dextran-10 kDa (FD10) and subsequently subjected to the sandwich assayCitation36,Citation37 for analysis of local passage using a 10 kDa tracer dextran (sw-D10). siRNA treatment resulted in a substantial reduction of occludin expression (, Western blot analysis, 29 ± 17% of siScrbl, p < 0.05) and a reduced TER (70 ± 9% of siScrbl controls, p < 0.05). Overall macromolecular passage was not changed significantly (Papp: 100 ± 11% of siScrbl; ). Residual occludin expression was strikingly inhomogeneous in response to siOcln treatment (). This, however, provides the opportunity to directly correlate macromolecular passage with different levels of occludin expression in these epithelia. Analysis of macromolecular tracer passage using a recently developed sandwich assayCitation36 was combined with immunostaining of occludin, which enabled direct comparison of occludin expression and tracer passage at subcellular level (). No correlation between passage of macromolecules (sandwich assay signal) and reduced levels or absence of occludin staining was observed. This was further confirmed when we analyzed our images by increasing contrast and brightness (). Even at sites where occludin expression was reduced to undetectable levels, tracer did not reveal any preferred passage. In fact, passage of macromolecules appeared to occur sporadically. It should be explicitely noted that the number of passage sites overall was very low. To illustrate this, Figure S1A/B shows larger areas (including the same image area as in ) together with additional ZO-1 staining of the tight junction. In these images, leaks may appear “clumpy”. This typical appearance may be caused by (i) the low final resolution of passage sites due to the large image area (up to 3.7 × 0.7 mm) and (ii) because maximum intensity projections of stacks of tile scans were generated to detect as many as possible leaks in these large areas (see Figure S2). Taken together, no correlation between reduced occludin expression and macromolecular passage was observed. Comparable results were obtained in T84 colonic epithelial cells. Transient transfection with siRNA targeting occludin resulted in 53 ± 3% residual occludin expression (data not shown) but again this had no effect on Papp to FD10 and did not correlate to sites of tracer passage (Figure S3). In summary, we have not found any effect of siRNA-mediated occludin reduction on global (Papp to FD10) or local (sandwich assay) passage of macromolecules in two intestinal epithelial cell lines.
Figure 1. CacoBBe cells with transient knockdown of occludin.
Characterization of transient occludin knockdown (siOcln) in Transwell-grown CacoBBe cells at days 7–8 (a) Occludin protein expression by Western blot analysis in CacoBBe cells treated with non-target (siScrbl) or occludin-targeting siRNA (siOcln). The inset shows a representative blot at 58–65 kDa and the diagram depicts relative expression (mean ± SD; n = 5). TER and FITC-dextran-10 kDa (FD10) passage are shown (mean ± SD; from 2 experiments with n = 4). (b) Corresponding occludin immunostaining, nuclei staining and tracer dextran passage (sw-D10). Image display thresholds were set to values that yield a siSrbl/siOcln signal ratio of occludin image signal that is directly comparable to Western blot results from (a). Local expression of occludin is below detectable levels in many cells but passage of tracer dextran (sw-D10) absent. Arrows indicate sites of tracer passage. (c) This is still true when analyzing the low signal range of occludin in the same image (increasing very low signal for display while producing saturated pixels where there is a high signal originally) as shown in red (saturated) and blue (zero) using a HiLo look-up table for image display.

Characterization of CacoBBe cells with stable knockdown of occludin
Given the absence of a correlation between occludin expression and macromolecule passage sites on single-cell level in T84 and CacoBBe cells, we sought to analyze effects of occludin deficiency after longer periods of cultivation. We used CacoBBe cells with stable knockdown of occludin (shOcln) generated by Buschmann and colleagues.Citation15 These occludin knockdown cells were recently reported to provide (i) a >90% reduction in global occludin protein expression, (ii) a reduced TER, and (iii) to have an activated leak pathway, i.e. increased passage of dextrans in the range of 3–40 kDa. In CacoBBe cells stably transfected with occludin-targeting shRNA (shOcln), occludin was substantially reduced compared to control cells (shScrbl; see for Western blot and for immunostaining). In agreement with Buschmann et al., TER of shOcln cells was reduced at any point in time () while permeability to 10 kDa dextran appeared unaffected in the first week of culture but was increased at later stages (). In order to clearly correlate global passage (reflected by the abovementioned measurements) with local macromolecular passage and occludin expression, we analyzed mixed cultures of shOcln and shScrbl cells at different ratios. As expected, Papp to FD10 scaled with the fraction of the shOcln cells in mixed cultures and so did TER reduction (,). But again, local passage was independent of local occludin expression ( and Figure S4), which depicts another experiment of mixed shOcln/shScrbl cell lines using a tracer size of 3 kDa in the local analysis. To test if rescue of occludin deficiency is sufficient to normalize global macromolecule passage, we applied an occludin rescue strategy using CacoBBe shRNAOcln cells with a doxycycline-inducible expression of EGFP-occludin. This variant (shOcln/EGFP-OCLN) was generated from the parental shOcln cell line, as reported by Buschmann and colleagues. Occludin protein expression levels were confirmed by Western blot analysis () and by immunostaining () showing the lowest occludin expression in non-induced shOcln/EGFP-OCLN cells. It should be noted that occludin expression in non-induced shOcln/EGFP-OCLN cells was thus even lower than in the parental shOcln cells. In the absence of doxycycline (i.e. no re-expression of EGFP-occludin), the permeability to FD10 ‒ against all expectations ‒ was substantially decreased compared to the parental occludin knockdown cell line (shOcln) (29% vs. 240% both with regard to shScrbl cells set to 100%, ). TER was unchanged compared to shOcln cells. In the presence of 20 ng/ml doxycycline, substantial occludin re-expression resulted in slightly increased TER but Papp was unaffected. As analyzed with the sandwich assay on shOcln/EGFP-OCLN cells, again macromolecule passage sites did not correlate with occludin expression levels () and did not preferentially localize to junctions lacking occludin.
Figure 2. CacoBBe cells with stable occludin knockdown.
Control (shScrbl) and stable CacoBBE occludin knockdown cells (shOcln) were seeded on Transwells and analyzed for occludin expression using Western blot analysis (a) and immunostaining (b). Transepithelial resistance (c) and macromolecule passage using FITC-Dextran-10 kDa (d) were monitored over 3 weeks (mean ± SD; n = 3 Transwells). Occludin knockdown resulted in a decreased TER (p < 0.05 for all days) and an increased passage of 10 kDa dextran in the occludin-targeting shRNA cell line (p < 0.01 for days 15 and 19).
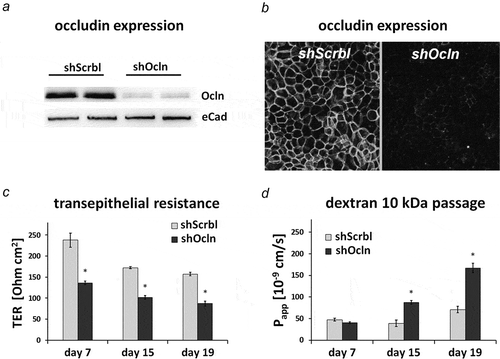
Figure 3. Macromolecule passage does not correlate with local occludin expression in CacoBBe with stable occludin knockdown.
(a) CacoBBe cells stably expressing shOcln-RNA or a control shScrbl-RNA were mixed at different ratios and permeability to FITC-dextran-10 kDa and TER were measured (day 19; mean ± SD; n = 3 Transwells, one out of three similar experiments). All groups differ for Papp and TER (p < 0.05) except for shOcln vs. shScrbl/shOcln 1:3 which exhibited no difference in Papp and shScrbl vs. shScrbl/shOcln 3:1 which showed no difference in TER. (b) Immunostaining for occludin and nuclei illustrating the distribution of occludin in shOcln and shScrbl cells across the epithelial sheet. (c) Higher magnification of an area from (B) with tracer dextran passage (sw-D10 in red) in relation to occludin staining.
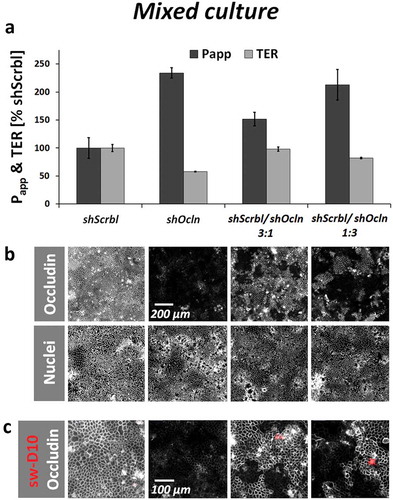
Figure 4. Macromolecule passage in cells with varying occludin expression levels.
Occludin knockdown CacoBBe (shOcln) cells, control CacoBBe (shScrbl) cells and shOcln cells transfected with a doxycycline-inducible re-expression vector encoding EGFP-occludin (shOcln/EGFP-OCLN) were analyzed for FD10 passage and TER.(a) Western blot analyses and (b) Occludin immunostaining (with heat-map coloring) of cell layers were performed after the flux experiments and illustrate the respective occludin expression level. (c) Permeability to FD10 and TER differed between all groups except for shOcln vs. shOcln/EGFP-OCLN (not different in TER) and shOcln/EGFP-OCLN vs. shOcln/EGFP-OCLN+doxy (not different in Papp) (day 21, mean ± SD, n = 3 Transwells, one out of two similar experiments, p < 0.05. (d) Local occludin (and EGFP-occludin) distribution and tracer passage are shown using a maximum intensity projected stack of a 5 × 5 tile scan image of shOcln/EGFP-OCLN cells. (e) Magnified area from (d) with increased brightness of occludin signal showing that knockdown of occludin is not sufficient to allow for macromolecule passage as areas with low or undetectable occludin expression do not correlate with tracer dextran passage (examples of sw-D10 passage indicated by arrows).

Macromolecule passage correlates with shOcln cell line fraction but not with local occludin expression
By running transient and stable knockdown approaches in different cell lines, we were not able to locate sites of macromolecule passage predominantly to cell–cell contacts with reduced or absent occludin expression. Rather, the passage sites appeared sparsely and irregularly. On the other hand, only in cells with stable occludin knockdown (shOcln) Papp was increased compared to control cells (shScrbl), which was somewhat contradictory. Applying the sandwich assay on Transwell filter supports and processing Transwells for microscopy involves excision of membranes from plastic supports. We noticed that cells at the edge area are only rarely preserved and hence usually not analyzed by microscopy. By paying close attention to preserving edges while excising membranes, we indeed observed a stronger signal for macromolecule passage at the edge of shOcln cells compared to shScrbl cells (examples in Figures S5 and S6B). To unequivocally rule out this potential pitfall in interpreting Transwell permeability assays, we seeded shOcln and shScrbl CacoBBe cells by placing one cell line directly at the outer rim of Transwells and the second cell line at the inner membrane surface and vice versa (see for seeding strategy and for corresponding occludin staining).
Figure 5. Passage of macromolecules occurs at Transwell edge in the shOcln cells.
(a) Scheme illustrating the localized seeding strategy for two cell lines on one Transwell. One cell line covers the main central area (inside) whereas the second covers the outer rim of the Transwell growth area (outside). (b) Corresponding images of Transwell membranes stained for occludin and nuclei. (c) Corresponding permeability to FITC-Dextran-10 kDa and TER at day 21 (mean ± SD; n = 3 Transwells, one out of two similar experiments; all groups differed in TER, p < 0.05). Only groups with different cells seeded outside differ in Papp.shScrbl (d–f) and shOcln cells (g–i) were seeded upside-down on 0.33 cm2 Transwells (inverted seeding), analyzed for macromolecule passage using the sandwich assay (sw-D10) and counterstained for nuclei. Transwell membrane edge areas are magnified in F and I.
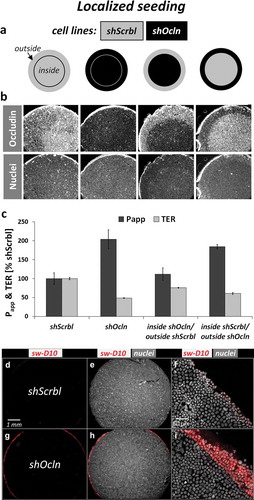
This means, we ran a classical global flux measurement but with defined local occludin expression or rather, with defined cell line location across the Transwell membrane. Independent on how the seeding strategy was set up, only in Transwells with shOcln cells at the outer rim of the growth area, permeability to FD10 was increased (). This finding suggested that the relevant leakage is localized at the outermost rim area of Transwells, being particularly high when shOcln cells are growing in this area. To allow for direct observation without the need to excise Transwell membranes, shOcln and shScrbl cells were seeded on the downside of Transwells (“inverted seeding”) and analyzed for global and local passage. By that seeding strategy the TER for shScrbl cells was 151 ± 3 Ω cm2 and 90 ± 2 Ω cm2 for shOcln cells. In both cell lines FD10 passage was lower compared to classical seeding, but again substantially increased in shOcln cells (4.1 ± 0.4 vs. 0.6 ± 0.1 10−9 cm/s in shScrbl). The sandwich assay applied to inversely seeded shScrbl and shOcln cells confirmed the predominantly permissive area to be a ring at the transition zone from membrane to plastic support (). Edge leakage was readily observable under the microscope (not shown) and now well preserved after excising the membranes. Further analysis revealed that the shOcln/EGFP-OCLN-CacoBBe exhibited lower permeability to FD10 (Figure S6A) and did not show a pronounced passage at the transwell edge (Figure S6B). These cells had even lower occludin expression compared to the parental shOcln cells indicating that the effect of Transwell egde passage is independent of occludin expression but rather is a property of the respective cell line.
To summarize, occludin-deficient cells of the shOcln-CacoBBe cell line at the outermost edges of Transwells proved responsible for passage of FD10 (permeability to FD10; global passage assay) and for sw-D10 labeling (sandwich assay; local passage). This was not observed for occludin-deficient cells of the shOcln/EGFP-OCLN CacoBBe cell line although these cells had even lower occludin expression levels in the absence of doxycycline.
Discussion
In the present study, using intestinal epithelial cells with different expression levels of occludin and employing complementary local and global barrier assays we observed that: (i) siRNA-mediated reduction of occludin in CacoBBe and T84 cells resulted in a large variation of occludin expression across the epithelial layers without affecting the passage of macromolecules, neither when globally measured as fluxes across Transwells nor when assessed by the sandwich assay with single cell resolution. (ii) Global passage was increased in shOcln CacoBBe cells with stable knockdown of occludin expression but this was also independent of occludin expression across the epithelial sheet. In fact, global passage appeared to originate from leaky cell junctions at the Transwell membrane edge instead. (iii) This leaky edge area was detectable by the sandwich assay at 4°C and by global flux measurement at 37°C using a localized seeding approach thus validating this finding. (iv) The leaky edge area was independent of occludin expression and might be a property of this particular shOcln cell line. In shOcln/EGFP-OCLN cells with stable occludin knockdown and non-induced re-expression of EGFP-Occludin no leaky edge area was found and basal permeability to macromolecules was even lower than in shScrbl cells. (v) Re-expression of occludin in these cells had no effect on macromolecular passage.
The cellular and structural basis of the epithelial leak pathway has so far eluded clarification.Citation1,Citation5,Citation38,Citation39 We aimed to characterize the effect of occludin expression on this leak pathway. The tight junction protein occludin was suggested to directly regulate the leak pathway of macromolecules passing across epithelia: reduced occludin expression in Caco-2 and CacoBBe cells was reported to directly result in increased flux of larger solutes,Citation14,Citation15 whereas other studies did not find such effect of occludin expression on the leak pathway in other epithelial cell lines.Citation11,Citation13,Citation32,Citation34 Using a recently developed method allowing for subcellular resolution of macromolecule passage sites within epithelial sheets,Citation36,Citation37 we are able to correlate protein expression with macromolecule passage at the level of single cell junctions. We hypothesized: given that knockdown of occludin indeed results in increased passage of macromolecules as stated by the aforementioned studies,Citation14,Citation15 macromolecule passage should preferentially occur at cellular junctions lacking occludin. Using the sandwich assay that tracks loci of macromolecule passage with subcellular resolution, variation of occludin from expression levels below detection limits to normal expression along cellular junctions should result in a large array of putative passage sites. Any correlation of macromolecule passage and occludin expression would be based on hundreds of thousands of image data points and hence become obvious. In contrast, global flux measurement is sensitive to rare, but possibly unrelated leaks as these will determine net flux in the background of an effective barrier (i.e. very small overall flux). Hence, we applied the recently developed sandwich assay to investigate the passage pathway taken by macromolecules across an epithelial sheet.
Occludin is an ubiquitously expressed tight junction protein, which suggests an essential role in epithelial function. So far, no consistent picture on occludin reduction or deficiency in epithelial barrier function emerged as some studies reported a direct contributionCitation14,Citation15 and others did not.Citation11,Citation13 We can now clarify this apparent contradiction: in Caco-2 cells (as well as in T84 cells) a modulation of occludin expression by different strategies (transient and stable knockdown, inducible rescue) did not result in increased macromolecule passage. Instead, passage between cells grown at the Transwell edge had far greater effect on passage than variation of occludin expression across the entire cell layer in these shOcln cells. The edge passage phenomenon explains the observed increase in dextran fluxes reported by Buschmann and colleagues. Up to now, a permissive area at the edge of Transwells would routinely pass unnoticed because (i) sites of passage at single-cell level would not be detectable even on completely intact membranes because there was no such method available until recently; and (ii) Transwell membranes analyzed by microscopy would not contain cells from the edge region (due to loss by excision). Since this local resolution of macromolecule passage was previously unavailable, respective data used to be interpreted relying on the coincidence of increased global flux and reduced global occludin expression.
A relevant fraction of basal solute flux may run along the edge leak, and this should be checked for in future studies. This fraction might determine the sensitivity of the respective barrier assay with regard to total passage and affects conclusions. The extent of the edge passage may vary between different cell lines and different cell culture settings. Definitively, however, an edge leak-mediated passage route should be taken into account as it will produce a comparably high “offset” on which changes in epithelial transport pathways may go undetected.
In conclusion, we have demonstrated that occludin does not regulate basal permeability to macromolecules in epithelial sheets. Neither lack nor re-expression of occludin had a consistent impact on macromolecule permeability. Therefore, occludin is likely not directly involved in passage of macromolecules across epithelial barriers. Rather it appears to mediate responses to environmental changes in epithelia and thus may have a more regulatory function.Citation13,Citation21,Citation24,Citation35
Further studies will be required to resolve a potential function of occludin in the context of molecule passage. Using in vivo models that avoid the limitations of cell culture would be desirable. Moreover, analysis of epithelial permeability on a global scale in the Transwell assay system is prone to misleading measurements and conclusions, particularly when investigating low capacity transport pathways, since rare but highly conductive leaks will then determine net flux. Upcoming studies on epithelial permeability using the Transwell system may thus benefit from combining global and local analysis of tracer molecule passage.
Material and methods
Cell culture
CacoBBe cells (ATCC-CRL-2102, Rockville, USA) were maintained in Dulbecco’s modified Eagle medium (DMEM) with 10% (v/v) of fetal bovine serum (FBS), and T84 cells in DMEM/HAMs F12 1:1 and 5% (v/v) FBS, each medium supplemented with 100 U/ml of penicillin, 100 μg/ml of streptomycin (Sigma, Taufenkirchen, Germany). Cells were incubated at 37°C in a humidified atmosphere containing 5% of CO2. For the experiments, cells were seeded on Millicell PCF filters (effective area: 0.6 cm2; 0.4 µm pores, Millicell PCF, Millipore, Schwalbach, Germany) or PC Transwell supports (effective area: 0.33 or 1.12 cm2; 0.4 µm pores, Corning Life Sciences, Tewksbury, USA). Experiments were performed with polarized monolayers having reached confluence, thus yielding stable TER on the day of culture as indicated. Seeding density was approximately 2.5–3.5 × 105 cells/cm2 for CacoBBe and 4.5 × 105 cells/cm2 for T84 cells unless otherwise indicated.
To achieve “local resolution” in global assays, we used different seeding strategies in which different CacoBBe cells were mixed (see “Mixed seeding”) or placed at known locations on Transwell membranes (see “Localized seeding”). All combinations of CacoBBe cell lines produced sustained epithelial barriers as evidenced by TER and apparent permeability (Papp).
Mixed seeding
Cells were trypsinized using standard procedures and mixed at indicated ratios to yield a final seeding density of 1.25 × 105 cells/cm2.
Localized seeding
Cells intended to cover the central Transwell membrane area were trypsinized, adjusted to 2 × 106 cells/mL and a 40 µL drop of this cell suspension was placed at the center of 0.6 cm2 Transwells (Millicell PCF) with no basal medium. Cells were allowed to adhere for 6 h, then medium was carefully aspirated, refilled apically and added basally. Thirty hours after initial seeding, the medium was removed and cells intended to cover the circumferential area of Transwell membranes added at a seeding density of 2.0 × 105 cells/cm2. The following day the medium was changed and cells were routinely cultured until used in the experiments.
Inverted seeding
To culture cells on the lower surface of a Transwell membrane, the method introduced by WakabayashiCitation40 was used with slight modifications: a 0.33 cm2 Transwell with a 0.4 µm pore size was placed upside-down inside the chamber of a 12-well culture plate. 1 × 105 cells in a 100 µL medium were seeded on top of the Transwell membrane and the 12-well plate cover was placed against the top of the membrane to prevent evaporation and to evenly distribute the cell suspension across the membrane area. Cells were allowed to adhere for 6 h prior to re-inversion of the Transwell, and subsequently cultured until used in the experiments.
Occludin knockdown and overexpression
For transient silencing of occludin expression, approximately 500000 cells/cm2 were seeded, transfected using 20 nM siRNA with 0.25% (v/v) Lipofectamine 2000 in a 1:1 mixture of Opti-MEM™ and cell culture medium during seeding into Transwells. After 16–20 h, the medium was exchanged and epithelia analyzed at day 7–8. Selected siRNA: ON-TARGETplus Non-targeting Pool, # D-001810–10-05 and ON-TARGETplus Human OCLN siRNA, # L-187897–00-0010 siRNA from GE Dharmacon (Lafayette, USA).
CacoBBe clones stably expressing occludin-targeting shRNA (shOcln-CacoBBe), non-targeting shRNA (shScrbl-CacoBBe) or inducible occludin-EGFP on occludin-targeting shRNA background (shOcln/EGFP-OCLN-CacoBBe) were identical to the ones used in Buschmann et al.Citation15 Briefly, pSUPER (Oligoengine) containing the occludin targeting sequence 5´-GTGAAGAGTACATGGCTGC-3´ was used to transfect CacoBBe cells stably expressing SGLT1.Citation41 For doxycycline-induction studies, piggyBAC-TREtight plasmids were generated by cloning a human EF1α promoter and TetOn3G expression cassette between the inverted terminal repeats of the piggyBAC plasmid (System Biosciences, Mountain View, CA). EGFP-occludin containing siRNA-evading mutationsCitation42 in piggyback-TREtight was stably expressed in these CacoBBe cells. These cells were analyzed about 3 weeks after culturing on Transwells.
Permeability to dextran
Global permeability to dextran 10 kDa was analyzed using Fluorescein-dextran 10 kDa (FD10) from TdBcons (Uppsala, Sweden). For flux experiments, 100 µM FD10 was added to the apical compartment of epithelia grown in Transwells using FluoroBrite™ DMEM (Thermo Fisher Scientific, Bonn, Germany) with 5% (v/v) or 10% (v/v) FBS, 1% (v/v) Glutamax and antibiotics and incubated at 37°C or 4°C as indicated. Apical to basal flux was calculated from a 1 h period. The fluorescence of the basolateral compartment was quantified using a plate reader (M200, TECAN, Austria). The apparent permeability was calculated using Papp = ΔQ/Δt·1/(A·C0), where ΔQ/Δt designates the amount of change in concentration/change in time at steady state, A the growth area and C0 the initial concentration in the donor chamber.
Sandwich assay for localization of transepithelial passage sites
Local analysis of macromolecule passage was performed using the sandwich assay.Citation36,Citation37 This method enables localization of apical-to-basal epithelial passage of tracer macromolecules, based on an avidin–biotin interaction. Biotinylated and fluorescing dextrans bind to basolaterally placed avidin at the site of passage through a junctional barrier. Briefly, epithelia were cooled on ice, washed in PBS (with Ca2+/Mg2+) and incubated with 15 µM of avidin (Merck, Darmstadt, Germany) at the basolateral side. After washing, a solution containing a biotinylated and fluorophore-labeled dextran (10 µM) was added to the apical compartment. Where label control was deemed appropriate, a fluorescing biotinylated dextran (20 µM) was added to the basolateral compartment at this point alternatively. Cells were fixed (2% of paraformaldehyde in PBS, 30 min, room temperature) and further processed for immunofluorescence or mounted for microscopy.
All fluorophore- and biotin-carrying dextrans selected for the sandwich assay (TRITC-biotin-dextran–10 kDa, TRITC-biotin-dextran–3 kDa, FITC-biotin-dextran–10 kDa and FITC-biotin-dextran–3 kDa) were from Invitrogen (Carlsbad, USA). They are referred to as tracer dextran in textual descriptions and sw-D in figures of this paper. The display colors in images are red for apical tracer dextrans and green for basal tracer dextrans irrespective of their original fluorophore. Prior to tracing epithelia at 4°C using the sandwich assay, the barrier defect to be analyzed was verified by running a global FD10 flux at 4°C, i.e. effects of ambient temperature on permeability to FD10 were checked at 37°C and 4°C. As an example, epithelia of shOcln and shScrbl cells underwent an initial flux measurement at 37°C, followed by cooling to 4°C and another flux measurement in cold situation using the same media. Permeability to FD10 was 144.8 ± 15.7 (shOcln) vs. 58.4 ± 6.1 10−9 cm/s (shScrbl) at 37°C and 52.4 ± 4.2 vs. 28.4 ± 6.3 10−9 cm/s at 4°C, respectively (n = 3, p < 0.01), equaling an increase in flux of 2.5-fold at 37°C vs. 1.8-fold at 4°C. This verified that the analyzed difference in barrier function is present also at 4°C, and that this barrier defect can be investigated using the sandwich assay at 4°C.
Western blotting
Total cell lysates were prepared by homogenizing the cells in RIPA buffer (150 mM NaCl, 2 mM of EDTA, 10% (v/v) glycerol, 1% (v/v) Triton-X-100, 0.5% (w/v) Na-deoxycholate, 0.2% (v/v) SDS) supplemented with Complete™ protease inhibitor mix (Roche, Mannheim, Germany) for 10 min on ice. After sonication and centrifugation (20.000 g for 10 min at 4°C), supernatants were diluted 1:2 with 2× SDS-loading buffer. Proteins were separated by SDS-polyacrylamide gel electrophoresis (SDS-PAGE) and Western blotting was performed as described.Citation43 Membranes were incubated overnight at 4°C with anti-Occludin (#331500; Invitrogen, Carlsbad, USA) or anti-E-cadherin antibody (#610181, BD Biosciences, San Jose, CA, USA). Chemiluminescence detection was performed using self-made ECL-solution on a GBOX gel documentation system (Syngene, Cambridge, UK).
Immunostaining and microscopy
To visualize TJs and AJs, PFA-fixed cells were incubated with primary antibodies (anti-ZO-1 #617300, anti-Occludin #331500; both from Invitrogen, Carlsbad, USA) and secondary antibodies (Alexa-488 and −594; −647; Invitrogen, Carlsbad, USA) in PBS with 0.3% (v/v) Triton-X and 0.05% (w/v) BSA for 2 h at room temperature or at 4°C overnight. Images were aquired with an AxioImager with AxioCam MRc (Zeiss, Jena, Germany) or an Axiovert 200 with a Neo camera (Andor Technology Ltd., Belfast, UK) to obtain larger fields of view. Further images, stacks and tile scans were recorded with a confocal laser scanning system TC/SP5 (Leica Microsystems, Wetzlar, Germany) or LSM 710 (Zeiss, Jena, Germany). For z-stack tile scans, after automatic stitching (Zeiss ZEN software) image stacks were mean-filtered, maximum intensity projected to reduce data size and then analyzed for tracer signal using FIJI.Citation44
Electrophysiology
Transepithelial resistance (TER, Ω cm2) of cells in culture supports was determined with a fixed pair electrode STX-2, connected to an ohm meter (EVOM-2, both from World Precision Instruments, Berlin, Germany) at 37°C. Resistance values were corrected for the resistance of an empty filter.
Statistics
The results are presented as mean values ± SD. Data were analyzed using one-way ANOVA. Where ANOVA indicated significant differences, group comparisons with post hoc test (Tukey’s HSD, Bonferroni) were performed. Values identified using Grubbs’ test for outliers were excluded from analysis. Differences with p < 0.05 have been considered significant. For the sake of clarity in diagrams these are given in texts of the legends.
Disclosure of potential conflicts of interest
No potential conflict of interest was reported by the authors.
Supplemental Material
Download MS Word (6.3 MB)Acknowledgments
We wish to thank I. Bernhardt for valuable technical assistance. J.F.R., J.R. and M.S. designed research; J.F.R., M.H.,R.S. and J.R. conducted research; J.T. provided cell lines, J.F.R., M.H., M.S. and J.R. analyzed data; J.F.R., M.S., J.T. and J.R. wrote and revised the paper and J.F.R. had primary responsibility for final content. All authors read and approved the final manuscript.
Supplymental Materials
Supplemental data for this article can be accessed on the publisher’s website.
Additional information
Funding
References
- Anderson JM, Van Itallie CM. Physiology and function of the tight junction. Cold Spring Harb Perspect Biol Aug. 2009;1:2.
- Günzel D, Fromm M. Claudins and other tight junction proteins. Compr Physiol. 2012;3:1819–1852.
- Turner JR. Intestinal mucosal barrier function in health and disease. Nat Rev Immunol. 2009;9(11):799–809. doi:10.1038/nri2653.
- König J, Wells J, Cani PD, García-Ródenas CL, MacDonald T, Mercenier A, Whyte J, Troost F, Brummer RJ. Human intestinal barrier function in health and disease. Clin Transl Gastroenterol. 2016;7(10):e196. doi:10.1038/ctg.2016.54.
- Shen L, Weber CR, Raleigh DR, Yu D, Turner JR. Tight junction pore and leak pathways: a dynamic duo. Annu Rev Physiol. 2011;73:283–309. doi:10.1146/annurev-physiol-012110-142150.
- Martini E, Krug SM, Siegmund B, Neurath MF, Becker C. Mend your Fences: the epithelial barrier and its relationship with mucosal immunity in inflammatory bowel disease. Cell Mol Gastroenterol Hepatol. 2017;4(1):33–46. doi:10.1016/j.jcmgh.2017.03.007.
- Kucharzik T, Maaser C, Lügering A, Kagnoff M, Mayer L, Targan S, Domschke W. Recent understanding of IBD pathogenesis: implications for future therapies. Inflamm Bowel Dis. 2006;11:1068–1083. doi:10.1097/01.mib.0000235827.21778.d5.
- van Elburg RM, Uil JJ, Mulder CJ, Heymans HS. Intestinal permeability in patients with coeliac disease and relatives of patients with coeliac disease. Gut. 1993;34(3):354–357. doi:10.1136/gut.34.3.354.
- Balda MS, Whitney JA, Flores C, González S, Cereijido M, Matter K. Functional dissociation of paracellular permeability and transepithelial electrical resistance and disruption of the apical-basolateral intramembrane diffusion barrier by expression of a mutant tight junction membrane protein. J Cell Biol. 1996;134(4):1031–1049. doi:10.1083/jcb.134.4.1089.
- Li D, Mrsny RJ. Oncogenic Raf-1 disrupts epithelial tight junctions via downregulation of occludin. J Cell Biol. 2000;148(4):791–800. doi:10.1083/jcb.148.4.791.
- Yu AS, McCarthy KM, Francis SA, McCormack JM, Lai J, Rogers RA, Lynch RD, Schneeberger EE. Knockdown of occludin expression leads to diverse phenotypic alterations in epithelial cells. Am. J. Physiol. Cell Physiol. 2005;288:C1231–C1241. doi:10.1152/ajpcell.00343.2004.
- Shen L, Weber CR, Turner JR. The tight junction protein complex undergoes rapid and continuous molecular remodeling at steady state. J Cell Biol. 2008;181(4):683–695. doi:10.1083/jcb.200711165.
- Van Itallie CM, Fanning AS, Holmes J, Anderson JM. Occludin is required for cytokine-induced regulation of tight junction barriers. J Cell Sci. 2010;123(Pt 16):2844–2852. doi:10.1242/jcs.065581.
- Al-Sadi R, Khatib K, Guo S, Ye D, Youssef M, Ma T. Occludin regulates macromolecule flux across the intestinal epithelial tight junction barrier. Am J Physiol Gastrointest Liver Physiol. 2011;300(6):G1054–64. doi:10.1152/ajpgi.00228.2010.
- Buschmann MM, Shen L, Rajapakse H, Raleigh DR, Wang Y, Wang Y, Lingaraju A, Zha J, Abbott E, McAuley EM, et al. Occludin OCEL-domain interactions are required for maintenance and regulation of the tight junction barrier to macromolecular flux. Mol Biol Cell. 2013;24:3056–3068. doi:10.1091/mbc.E13-03-0144.
- O’Driscoll MC, Daly SB, Urquhart JE, Black GC, Pilz DT, Brockmann K, McEntagart M, Abdel-Salam G, Zaki M, Wolf NI, et al. Recessive mutations in the gene encoding the tight junction protein occludin cause band-like calcification with simplified gyration and polymicrogyria. Am J Hum Genet. 2010;87:354–364.
- LeBlanc MA, Penney LS, Gaston D, Shi Y, Aberg E, Nightingale M, Jiang H, Gillett RM, Fahiminiya S, Macgillivray C, et al. A novel rearrangement of occludin causes brain calcification and renal dysfunction. Hum Genet. 2013;132(11):1223–1234. doi:10.1007/s00439-013-1327-y.
- Saitou M, Furuse M, Sasaki H, Schulzke JD, Fromm M, Takano H, Noda T, Tsukita S. Complex phenotype of mice lacking occludin, a component of tight junction strands. Mol Biol Cell. 2000;11(12):4131–4142. doi:10.1091/mbc.11.12.4131.
- Schulzke JD, Gitter AH, Mankertz J, Spiegel S, Seidler U, Amasheh S, Saitou M, Tsukita S, Fromm M. Epithelial transport and barrier function in occludin-deficient mice. Biochim Biophys Acta. 2005;1669(1):34–42. doi:10.1016/j.bbamem.2005.01.008.
- Kitajiri S, Katsuno T, Sasaki H, Ito J, Furuse M, Tsukita S. Deafness in occludin-deficient mice with dislocation of tricellulin and progressive apoptosis of the hair cells. Biol Open. 2014;3:759–766.
- Bolinger MT, Ramshekar A, Waldschmidt HV, Larsen SD, Bewley MC, Flanagan JM, Antonetti DA. Occludin S471 phosphorylation contributes to epithelial monolayer maturation. Mol Cell Biol. 2016;36(15):2051–2066. doi:10.1128/MCB.00053-16.
- Manda B, Mir H, Gangwar R, Meena AS, Amin S, Shukla PK, Dalal K, Suzuki T, Rao R. Phosphorylation hotspot in the C-terminal domain of occludin regulates the dynamics of epithelial junctional complexes. J Cell Sci. 2018;131:7. doi:10.1242/jcs.216507.
- Odenwald MA, Choi W, Buckley A, Shashikanth N, Joseph NE, Wang Y, Warren MH, Buschmann MM, Pavlyuk R, Hildebrand J, et al. ZO-1 interactions with F-actin and occludin direct epithelial polarization and single lumen specification in 3D culture. J Cell Sci. 2017;130(1):243–259. doi:10.1242/jcs.200857.
- Du D, Xu F, Yu L, Zhang C, Lu X, Yuan H, Huang Q, Zhang F, Bao H, Jia L, et al. The tight junction protein, occludin, regulates the directional migration of epithelial cells. Dev Cell. 2010;18(1):52–63. doi:10.1016/j.devcel.2010.01.004.
- Beeman N, Webb PG, Baumgartner HK. Occludin is required for apoptosis when claudin-claudin interactions are disrupted. Cell Death Dis. 2012;23(3):e273. doi:10.1038/cddis.2012.14.
- Edelblum KL, Shen L, Weber CR, Marchiando AM, Clay BS, Wang Y, Prinz I, Malissen B, Sperling AI, Turner JR. Dynamic migration of γδ intraepithelial lymphocytes requires occludin. Proc Natl Acad Sci U S A. 2012;109(18):7097–7102. doi:10.1073/pnas.1112519109.
- Ronaghan NJ, Shang J, Iablokov V, Zaheer R, Colarusso P, Dion S, Désilets A, Leduc R, Turner JR, MacNaughton WK. The serine protease-mediated increase in intestinal epithelial barrier function is dependent on occludin and requires an intact tight junction. Am J Physiol Gastrointest Liver Physiol. 2016;311(3):G466–79. doi:10.1152/ajpgi.00441.2015.
- Cording J, Günther R, Vigolo E, Tscheik C, Winkler L, Schlattner I, Lorenz D, Haseloff RF, Schmidt-Ott KM, Wolburg H, et al. Redox regulation of cell contacts by tricellulin and occludin: redox-sensitive cysteine sites in tricellulin regulate both tri- and bicellular junctions in tissue barriers as shown in Hypoxia and Ischemia. Antioxid Redox Signal. 2015;23(13):1035–1049. doi:10.1089/ars.2014.6162.
- Mir H, Meena AS, Chaudhry KK, Shukla PK, Gangwar R, Manda B, Padala MK, Shen L, Turner JR, Dietrich P, et al. Occludin deficiency promotes ethanol-induced disruption of colonic epithelial junctions, gut barrier dysfunction and liver damage in mice. Biochim Biophys Acta. 2016;1860(4):765–774. doi:10.1016/j.bbagen.2016.04.023.
- Janosevic D, Axis J, Bacallao RL, Amsler K; Occludin Content Modulates Hydrogen. Peroxide-induced increase in renal epithelial paracellular permeability. J Cell Biochem. 2016;117(3):769–779. doi:10.1002/jcb.25508.
- Bilal S, Jaggi S, Janosevic D, Shah N, Teymour S, Voronina A, Watari J, Axis J, Amsler KZO. 1 protein is required for hydrogen peroxide to increase MDCK cell paracellular permeability in an ERK 1/2-dependent manner. Am J Physiol Cell Physiol. 2018 Sep 1;315(3):C422–C431. doi:10.1152/ajpcell.00185.2017.
- McCarthy KM, Skare IB, Stankewich MC, Furuse M, Tsukita S, Rogers RA, Lynch RD, Schneeberger EE. Occludin is a functional component of the tight junction. J Cell Sci. 1996;109:2287–2298.
- McCarthy KM, Francis SA, McCormack JM, Lai J, Rogers RA, Skare IB, Lynch RD, Schneeberger EE. Inducible expression of claudin-1-myc but not occludin-VSV-G results in aberrant tight junction strand formation in MDCK cells. J Cell Sci. 2000;113:3387–3398.
- Balda MS, Flores-Maldonado C, Cereijido M, Matter K. Multiple domains of occludin are involved in the regulation of paracellular permeability. J Cell Biochem. 2000;78(1):85–96. doi:10.1002/(ISSN)1097-4644.
- Marchiando AM, Shen L, Graham WV, Weber CR, Schwarz BT, Austin JR 2nd, Raleigh DR, Guan Y, Watson AJ, Montrose MH, et al. Caveolin-1-dependent occludin endocytosis is required for TNF-induced tight junction regulation in vivo. J Cell Biol. 2010;189(1):111–126. doi:10.1083/jcb.200902153.
- Richter JF, Schmauder R, Krug SM, Gebert A, Schumann M. A novel method for imaging sites of paracellular passage of macromolecules in epithelial sheets. J Control Release. 2016;229:70–79. doi:10.1016/j.jconrel.2016.03.018.
- Reiche J, Schumann M, Richter JF. The sandwich assay: a method for subcellular visualization of paracellular macromolecule passage in epithelial sheets. Curr Protoc Cell Biol. 2018 Mar;78(1):20.
- Weber CR. Dynamic properties of the tight junction barrier. Ann N Y Acad Sci. 2012;1257:77–84.
- Liang GH, Weber CR. Molecular aspects of tight junction barrier function. Curr Opin Pharmacol. 2014;19:84–89. doi:10.1016/j.coph.2014.07.017.
- Wakabayashi Y, Chua J, Larkin JM, Lippincott-Schwartz J, Arias IM. Four-dimensional imaging of filter-grown polarized epithelial cells. Histochem Cell Biol. 2007;127(5):463–472. doi:10.1007/s00418-007-0274-x.
- Turner JR, Rill BK, Carlson SL, Carnes D, Kerner R, Mrsny RJ, Madara JL. Physiological regulation of epithelial tight junctions is associated with myosin light-chain phosphorylation. Am. J. Physiol. 1997;273:C1378–C1385. doi:10.1152/ajpcell.1997.273.4.C1378.
- Raleigh DR, Boe DM, Yu D, Weber CR, Marchiando AM, Bradford EM, Wang Y, Wu L, Schneeberger EE, Shen L, et al. Occludin S408 phosphorylation regulates tight junction protein interactions and barrier function. J Cell Biol. 2011;193(3):565–582. doi:10.1083/jcb.201010065.
- Weiske J, Schöneberg T, Schroder W, Hatzfeld M, Tauber R, Huber O. The fate of desmosomal proteins in apoptotic cells. J. Biol. Chem. 2001;276:41175–41181.
- Schindelin J, Arganda-Carreras I, Frise E, Kaynig V, Longair M, Pietzsch T, Preibisch S, Rueden C, Saalfeld S, Schmid B, et al. Fiji: an open-source platform for biological-image analysis. Nat Methods. 2012;9(7):676–682. doi:10.1038/nmeth.2019.