ABSTRACT
Epithelial cells connect with each other by tight junctions (TJs) in several tissues. In epididymides, TJs proteins form the blood-epididymis barrier (BEB), which is crucial for male fertility. However, little is known about BEB morphological and physiological aspects in wild animals. This study examines the region-specific distribution pattern of TJs proteins in D. rotundus’ epididymis, assessing their regulation in rainy and dry season. The expression of zonula occludens-1 (ZO-1), and claudins (Cldn)-1, −3, and −4 were evaluated by confocal immunofluorescence and ELISA analysis. Herein, ZO-1 was strictly expressed in TJs, whereas Cldns were expressed in TJs and basolateral membranes of epithelial cells. Their co-localization and intensity of expression varied in the epididymal regions examined. The effect of season on protein expression was detected mainly in TJ proteins located in the proximal regions. As such, in the initial segment (IS), Cldn-3 and −4 were detected at low levels in basolateral membranes in the rainy season compared to the dry season. Furthermore, in the distal IS, Cldn-1 expression was lower in TJs of epithelial cells during the rainy season than the dry season. ZO-1 expression was higher in the cauda region than the corpus region by ELISA analysis. Additionally, in the corpus region, ZO-1 expression was higher in TJs during dry season compared to the rainy season. Our study sheds light on the understanding of BEB in D. rotundus, improving the knowledge of their reproductive biology.
1. Introduction
The regulation of solute and fluid transport across epithelial barriers is crucial to the survival of multicellular organisms. It promotes the maintenance of body homeostasis in response to the constant changes in the external environment.Citation1,Citation2 Epithelial barriers are formed by tight junctions (TJs), which are composed of membrane proteins, cytoplasmic plaque proteins, and cytoskeletal proteins. However, their structure and the driving forces that regulate their functions differ significantly between organs.Citation3-Citation6 In the epididymis, for instance, TJs between epithelial cells are responsible for the formation of the blood-epididymal barrier (BEB) and represent a key element of epididymal physiology.Citation7,Citation8,Citation9
The BEB consists of a physical, physiological and immunological barrier that regulates the luminal milieu of the epididymal duct, protecting auto-antigenic sperm from the immune system. Moreover, it contributes to the composition of a unique luminal environment required for sperm maturation, besides maintaining apical/basolateral epithelial cell polarity. For all these reasons, it is a critical structure for male fertility.Citation10-Citation12 Transmembrane and peripheral proteins have been described as components of TJs in the epididymis of human, rat and mouse.Citation13-Citation16 Although numerous TJ proteins have been identified, the understanding of their functions in the epididymis remains elusive.
Overall, zonula occludens (ZO) connect transmembrane TJ proteins to the actin cytoskeleton on the cytoplasmic side of its strands. This protein family is composed of ZO-1, ZO-2, and ZO-3,Citation17,Citation18 which have been previously detected in the epididymis.Citation15,Citation19 Other studies have also described the presence of claudin (Cldn)-1, −3, and −4 along the entire epididymal duct.Citation7,Citation20 Claudins are essential organizers of TJs and modulate paracellular permeability to ions and water in tissues.Citation21-Citation22In contrast, to other epithelia where Cldns are restricted to TJs, in the epididymis, they were also detected in basolateral membranes of epithelial cells.Citation4,Citation7,Citation15 Claudins are part of a multigene family with different expression patterns in different organs and their location within an organ. Different Cldns have been described in the same tissue, as well as different interaction patterns between them.Citation11,Citation15,Citation20 Finally, occludins contribute to TJ formation in all epididymal regions, except the initial segment.Citation7,Citation11 This phosphoprotein has been noted as a constituent of TJ strands, being associated with the cytoskeleton through a direct interaction with various proteins.Citation17,Citation23
So far, there is a paucity of information concerning the BEB’s composition and the distribution pattern of TJ proteins in wild animals. In this framework, Desmodus rotundus bats may be an interesting study model, as it is an unthreatened species and the control of its population is necessary for the reduction of rabies disease occurrences in South American countries.Citation24 The reproductive pattern of this vampire bat is apparently influenced by seasonal variations.Citation25 Despite alterations in testis morphometry have been recently described in D. rotundus captured in rainy and dry seasons, no alteration was observed in sperm and testosterone production.Citation26 It is known that testosterone might play an important role in the regulation of TJs, with possible regulation mechanism of Cldn-1 in the initial segment of the rat epididymis.Citation14 Notwithstanding, there is scarce information concerning the influence of environmental variations in the TJ organization within the BEB of wild animals.
In this light, we aimed to describe the distribution pattern of TJ proteins in D. rotundus’ epididymis. To that end, morphological changes in their expression patterns were assessed in different segments of the epididymis, which were compared between rainy and dry seasons. Our data add novelty to the understanding of the expression of the TJs’ proteins in this organ. Moreover, they give a broader view of the BEB in D. rotundus.
2. Methods
2.1. Study area and animals
Sixteen adult males were captured using mist nets in Itamarati de Minas, Minas Gerais State, Brazil (21°23’S and 42°51ʹW; 585 m altitude). Eight animals were captured in November during the rainy season in Brazil, and eight animals were captured in June during the dry season. The means of temperature, rainfall, photoperiod, and relative air humidity during the period of captures were 17.4 °C, 0.07 mm, 7.3 time/light/day and 80.2% in the dry season (April-September), respectively, and 22 °C, 6.77 mm, 8.02 time/light/day and 74.9% in the rainy season (October to March), respectively (Meteorological Station, Department of Agricultural Engineering, Federal University of Viçosa, Minas Gerais, Brazil). The animals were identified according to the identification key for Brazilian bats. After capture, the animals were transported to the Laboratory of Structural Biology at the Universidade Federal de Viçosa (UFV), where they were placed in cages protected from light. Euthanasia was performed by intraperitoneal administration of sodium pentobarbital at a dose of 40 mg/kg of body weight, followed by guillotining.Citation27
Bat capture was authorized by the Chico Mendes Institute for Biodiversity Conservation (ICMBio, license number 40629–1), while the experimental procedures were reviewed and approved by the Committee on the Ethics and Use of Animal Experiments of UFV (CEUA process number 55/2013). This study was carried out in strict accordance with the recommendations in the Guide for the Care and Use of Laboratory Animals of the National Institutes of Health.
2.2. Protein extraction and enzyme-linked immunosorbent assay (ELISA)
For protein extraction, epididymides (n = 6/group) were divided into three regions, proximal portion (including whole initial segments and caput), corpus and cauda. Both initial segment (IS) and caput were processed together due to the thin size of the IS. Each fragment was then immersed in a microtube containing 1% (v/v) Triton X-100 diluted in 0.01 M PBS with protease inhibitors (Protease Inhibitor cocktail P8340, Sigma-Aldrich, USA), followed by homogenization (Pellet pestle, Sigma Aldrich, MO, USA). Thereafter, the microtubes were centrifuged at 700 rpm for 10 min at 4°C. The recovered supernatant was centrifuged again at 14,000 rpm for 10 min at 4°C (2216 MK, Hermle, WI, USA). The resulting supernatant of each fragment was lyophilized for 24 h and stored at room temperature.
Prior to the ELISA measurements, the samples were suspended in 2.8% (w/v) SDS diluted in 0.9% (w/v) NaCl, followed by protein concentration measurement by NanoDropTM2000/2000 c Spectrophotometer [Thermo Scientific; Wilmington, Delaware, USA). Therefore, ELISA was performed according to the Citation28,method with adjustments. Briefly, immunoassay plates were coated with 50 µl/well antigen diluted in coating solution (50 mM Carbonate pH 9.6] and incubated overnight at 4°C. Plates were washed twice with washing buffer (10 mM PBS pH 7.2). Then, it was added in each sample 300 µl/well of blocking solution (0.5% (w/v) nonfat dried milk diluted in 10 mM PBS pH 7.2 for 30 min, followed by two washes with washing buffer. After the washes, it was added 50 µl/well primary antibody of rabbit anti-Cldn-1, −3, and −4 (1:300; Invitrogen, Grand Island, NY, USA), and anti-ZO-1 (1:300; diluted in 10 mM PBS pH 7.2 plus 0.1% (w/v) nonfat dried milk for 2 h at room temperature under gentle shaking. Following the incubation, plates were washed twice, with 10 aspirations each time, with washing buffer in the Wellwash Microplate Washer (Thermo Scientific, Rockford, IL, USA). A 50 µl/well of secondary antibody anti-rabbit IgG-HRP (1:300; Jackson ImmunoResearch Laboratories, West Grove, PA, USA) diluted in PBS solution was incubated for 1 h at room temperature under gentle shaking.
One tablet of 3,3ʹ, 5,5ʹ tetramethylbenzidine dihydrochloride (TMB) substrate was dissolved in 10 ml of 0.05 M phosphate-citrate buffer pH 5.0, followed by the addition of 2 µl of fresh 30% (v/v) hydrogen peroxide. Then, 200 µl/well of this solution was added. After 30 min, the absorbance was determined with a spectrophotometer (Thermo Scientific, Rockford, IL, USA), and the reaction was stopped with 2 M H2SO4. Results were expressed in absorbance at 655 nm.
2.3. Tissue fixation and immunofluorescence
The entire epididymides (n = 10/group) were fixed by immersion in periodate-lysine-paraformaldehyde (PLP) solution containing 4% paraformaldehyde for 4 h at room temperature. They were then rinsed three times in phosphate-buffered saline (PBS). Tissues were cryoprotected by incubating them in a solution of 30% sucrose in PBS overnight, followed by embedding in OCT compound (Tissue-Tek; Sakura Finetek, Torrance, CA, USA). They were mounted on a cutting block and frozen. Tissues were cut at 5 µm thickness using a Leica 3050 cryostat (Leica Microsystems, Bannockburn, IL, USA) and sections were placed onto Fisher Superfrost/Plus microscope slides (Fisher Scientific, Pittsburgh, PA, USA), and stored at 4°C until use.Citation27,Citation29
Sections were hydrated in PBS for 10 min and heated by microwaving in an alkaline buffer (Vector Laboratory, Burlingame, CA) four times for 1 min each time, with 5 min intervals for antigen retrieval. To block nonspecific binding, 1% bovine serum albumin in PBS was applied for 30 min at room temperature. The sections were then incubated with primary rabbit polyclonal antibodies of anti-claudin-1, −3 and −4 (1:200; Invitrogen, Grand Island, NY, USA),Citation15 in a moist chamber overnight at 4°C. Then, the samples were washed in PBS and incubated with secondary antibodies for 60 min at room temperature. Double-immunolabeling was performed with anti-ZO-1 rat monoclonal antibody (1:40; Gift from Dr. Eveline Schneeberger, Department of Pathology, Massachusetts General Hospital, MA, USA to Dr. Sylvie Breton). The secondary antibodies (goat anti-rabbit IgG FITC-conjugated, 1:200; donkey anti-rat CY3-conjugated, 1:800) used in this study were affinity purified and obtained from Jackson ImmunoResearch Laboratories (West Grove, PA, USA). All antibodies were diluted in Dako antibody diluent (Dako).
Four epididymal regions, initial segments, caput, corpus, and cauda, were considered for evaluating the expression pattern of TJs’ proteins along the D. rotundus’ epididymis. Particularly, the initial segments were subdivided into the proximal (pIS), near to the efferent duct, and the distal portion (dIS), located between the pIS and caput region. In order to identify clear cells and basal cells in the epididymal epithelium, chicken V-ATPase B1 subunit antibody was used for clear cell identification,Citation27 and a rabbit polyclonal KRT5 antibody (Abcam, Cambridge, MA, USA) was used for basal cell identification. Citation27,Citation30 The secondary antibodies used in the double immunolabeling were CY3-conjugated donkey anti-chicken and Alexa 488-conjugated goat anti-rabbit IgG, respectively (Jackson ImmunoResearch Laboratories, West Grove, PA, USA).Citation27 Confocal images were acquired on a Nikon A1 R confocal microscope with NIS Elements (Nikon Instruments), followed by analyzes with NIS Elements, Volocity 6 (v.6.3.1, Perkin Elmer), and Adobe Photoshop. Negative controls in which the primary antibody was omitted were included in immunofluorescence studies (Supplementary Figure S1).
The fluorescence intensity of Cldn-1, −3, and −4 labeling in TJs and basolateral membranes was analyzed using the Line Profile tool from Image Pro-Plus (Media Cybernetics, Silver Springs, MD, USA) software. The image mode was first changed to RGB. Then, seven lines were randomly applied along the epithelial cell boundaries in each image (10 images per region) from the apex to the base of the epithelium. Each line covered 60 pixels that were used for measuring the intensity of green pixels (IGP). Thus, IGP corresponded to the intensity of Cldn labeling, which varied from 0 (zero; absence of green color) to 255 (maximum green intensity).
2.4. Transmission electron microscopy (TEM)
Epididymal sections were also incubated with 1% OsO4/0.1 M NaCacodylate on ice for 30 min and histologically processed for embedding in epon resin (Embed 812 kit) to characterize the TJs in the epididymis of D. rotundus.Citation27 Grids were examined using a JEM-1011 transmission electron microscope (JEOL, Tokyo, Japan) at 80 kV, and images acquired using an AMT XR60 digital imaging system (Advance Microscopy Techniques, Danvers, MA) were subsequently imported into Adobe Photoshop.
2.5. Statistical analysis
The normality of the results was evaluated by Shapiro-Wilk test, followed by 2-way ANOVA to compare the absorbance of Cldn-1, −3, −4, and ZO-1 considering regions (proximal portion, corpus, and cauda) and seasons (rainy and dry) as factors. The IGP of Cldns in TJs and basolateral membranes were also assessed by 2-way ANOVA. Then, we performed a post-hoc Tukey’s HSD test to account for the differences between each factor. Differences were considered significant when P < .05. The statistical software GraphPad Prism 6.0 (GraphPad Software Inc., San Diego, CA, USA) was used to perform the test, as well as to design all graphics. Data were expressed as mean ± standard error (SE).
3. Results
3.1. The expression and quantification of TJ proteins by ELISA
The results of ELISA analysis comparing the absorbance of TJ protein markers between regions and seasons are shown in . We did not observe differences among epididymal regions for the magnitude of expression for Cldn-1, Cldn-3, and Cldn-4. By contrast, the magnitude of ZO-1 expression was higher in the cauda epididymis compared to the corpus region. Moreover, seasons did not influence the magnitude of expression of Cldn-1, Cldn-3, and Cldn-4 in the epididymal regions. In fact, we did not observe difference between dry and rainy seasons for the magnitude of Cldns expression. On the other hand, the expression of ZO-1 in the epididymal regions was influenced by seasons. In the corpus region, for instance, the magnitude of ZO-1 expression was higher in the dry season compared to the rainy season. ANOVA p-values are shown in the Supplementary Table 1.
Figure 1. Claudin 1, claudin 3, claudin 4, and zonula occludens 1 (ZO-1) expression in the proximal portion (including whole initial segments and caput), corpus, and cauda regions of Desmodus rotundus’ epididymis measured by ELISA. Asterisks indicate significant differences (P < .05) by 2-way ANOVA and Tukey’s HSD test.
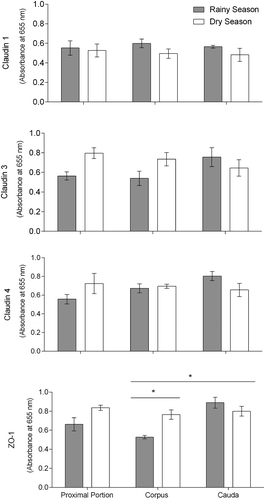
3.2. Identification of epithelial components in the epididymis of vampire bats
By transmission electron microscopy, the epididymis of D. rotundus presented a series of apical TJs between adjacent principal cells (, black arrows) observed in the IS, caput, corpus, and cauda regions (). They were distinguished by the close apposition of the plasma membranes where the membranes of the adjacent cells appear to fuse (, black arrows). Furthermore, desmosomes were observed between epithelial cells along the duct (,, white arrows). In addition to principal cells, clear cells contributed to TJ formation (, black arrows). This cell type was identified here by its irregular-shaped nucleus located in the middle portion of the epithelium. Finally, basal cells were observed in the epididymis by its nucleus located in the basal portion of the epithelium. This cell type did not exhibit slender cytoplasmic extensions (axiopodia) toward the lumen, with no contribution for TJs formation ().
Figure 2. Transmission electron microscopy of the epithelium (Ep) in Desmodus rotundus epididymis. Images show the apical tight junction area (black arrow) in the epithelial cells of initial segment (b), caput (a, c), corpus (d), and cauda (e) regions, specially between principal cells (PC) and clear cells (CC) (a and d). Desmosomes (white arrow) were also observed between epididymal epithelial cells (b and d). L: lumen, BC: basal cell, n: nuclei. Scale bars: (a) 10 µm, rainy season; (b) 500 nm, rainy season; (c) 2 µm, dry season; (d) 500 nm, dry season; (e) 2 µm, rainy season.

In order to identify each epididymal cell type, antibodies against the V-ATPase B1 subunit, a clear cell marker, and antibodies against KRT5, a basal cell marker, were used. The epithelium was double-labeled for V-ATPase and Cldn-1 (), and for KRT5 and ZO-1 (). Several V-ATPase-positive clear cells were detected and showed Cldn-1 labeling in their basolateral membranes (; arrows). Cldn-1 delineated the goblet shape of this cell type, which presented a nucleus located in its apical region. Several principal cells, negative for V-ATPase, were detected and showed their columnar shape with ovoid nucleus located at the middle portion of the cell (, asterisks). Finally, basal cells, positive for KRT5, were observed at the base of the epithelium and were present from the IS to cauda regions (, arrowheads). Cldn-1 was also detected in basal cells (; arrowheads). In D. rotundus, in contrast to the rat and mouse epididymisCitation15,Citation16,Citation31 basal cells did not exhibit luminal-reaching axiopodia and did not cross the TJs, which were delimited by ZO-1 labeling ().
Figure 3. Double-immunolabeling of claudin 1 (Cldn-1; green) and V-ATPase B1 subunit (B1, red), and keratin 5 (KRT5; green) and zonula occludens 1 (ZO-1; red) in the initial segment, caput, corpus, and cauda of the Desmodus rotundus’ epididymis. In all regions, Cldn-1 was observed in the basolateral membrane of the epididymal epithelial cells, whereas V-ATPase B1 subunit (white arrow) was observed only in the apical portion of clear cells. Due to the Cldn-1 labeling, we observed principal cells (*) by its columnar shape, and clear cells by its goblet shape. Basal cells (white arrowhead) was recognized by intense labeling for Cldn-1 and KRT5 at the base of the epithelium in all epididymal regions without axiopodia. ZO-1 was observed strictly in the tight junction portion. L: lumen, Ep: epithelium, In: interduct compartment. Sperm and nuclei are labeled with DAPI (blue). Scale bars: 50 µm.
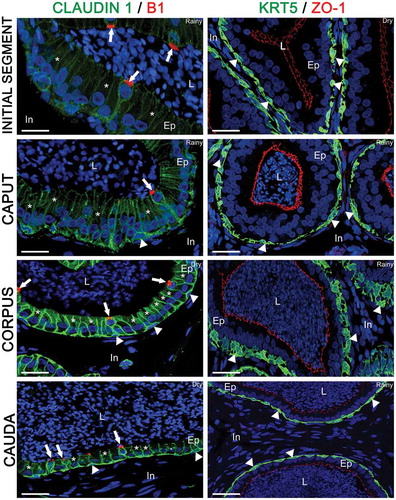
3.3. Immunolocalization of ZO-1 and Cldns, effect of the season
Double-immunofluorescence labeling of epididymal cryosections for ZO-1 and Cldn-1, −3, and −4 was carried out, and high-magnification images were obtained to determine their distribution pattern within the epithelium. Hence, confocal immunofluorescence images showed high expression of these TJ proteins along the entire length of D. rotundus’ epididymis (–). ZO-1 expression was strictly located in the epithelial cell TJs in each epididymal segment analyzed in both rainy and dry seasons (–). The co-localization and intensity of expression of Cldns, in turn, varied between the epididymal regions. This variation was also influenced by the season examined ( and ). ANOVA p-values are shown in the Supplementary Table 2.
Table 1. Expression levels of claudin proteins (IGP) in tight junctions of Desmodus rotundus’s epididymis determined by immunofluorescence analysis.
Table 2. Expression levels of claudin proteins (IGP) in basolateral membranes of Desmodus rotundus’s epididymis determined by immunofluorescence analysis.
Figure 4. Expression of claudin 1 (green) and zonula occludens 1 (ZO-1, red) in the epididymis of Desmodus rotundus in rainy and dry seasons. In the rainy season, claudin 1 was detected in tight junctions and in the basolateral membrane of epithelial cells, from the initial segment (IS) to cauda regions. Claudin 1 labeling was observed in basal cells (arrowheads) in all epididymis regions at rainy and dry season. Sperm and nuclei are labeled with DAPI (blue). Scale bars: 50 µm.
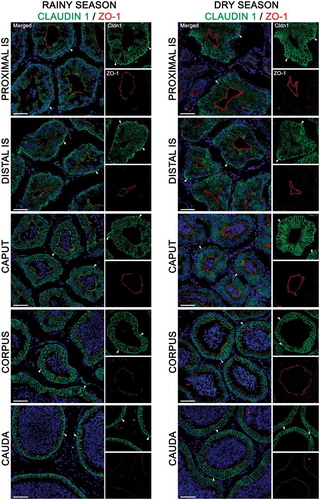
Figure 5. Expression of claudin 3 (green) and zonula occludens 1 (ZO-1, red) in the epididymis of Desmodus rotundus during the rainy and dry season. Higher expression of claudin 3 was detected in the basolateral membrane of initial segment (IS) regions from dry season epididymis compared to rainy season. In contrast, a higher expressed claudin-3 was detected in tight junctions of the corpus and cauda regions from rainy season epididymis compared to dry season. Claudin 3 labeling was observed in basal cells (arrowheads) in the corpus and cauda regions at rainy and dry season. Sperm and nuclei are labeled with DAPI (blue). Scale bars: 50 µm.
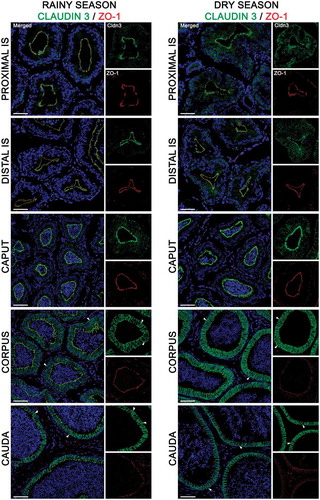
Figure 6. Expression of claudin 4 (green) and zonula occludens 1 (ZO-1, red) in the epididymis of Desmodus rotundus during the rainy and dry season. Higher expression of claudin 4 was detected in the basolateral membrane of initial segment (IS), and caput regions from dry season epididymis compared to rainy season. In contrast, a higher expressed claudin 4 was detected in tight junctions of the corpus and cauda regions from rainy season epididymis compared to dry season. Claudin 4 labeling was observed in basal cells (arrowheads) in the caput, corpus and cauda regions at rainy and dry season. Sperm and nuclei are labeled with DAPI (blue). Scale bars: 50 µm.
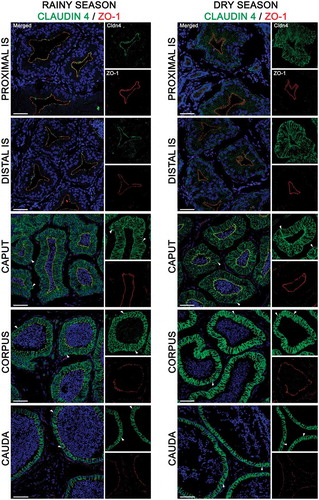
3.4. Localization in TJs
In the rainy season, Cldn-1 was expressed in TJs of the proximal (pIS) and distal initial segments (dIS) and in the caput, showing a strong co-localization with ZO-1 (; left panels). This co-localization became less intense in the corpus and cauda regions (; left panels). The same pattern of Clnd-1/ZO-1 co-localization was observed in the TJs of epididymal regions in the dry season (; right panels). Further, the IGP of Cldn-1 labeling was higher in TJs of the corpus and cauda regions than the caput and pIS during the rainy season (). The dIS region showed the lowest intensity of Cldn-1 labeling (). By contrast, in the dry season, the intensity of Cldn-1 labeling was higher in the cauda region compared to the other regions ().
As shown in , Cldn-3 labeling was moderate in TJs of the pIS, dIS, caput, and corpus in the rainy season, whereas the cauda region showed the strongest IGP (). In the dry season, corpus and cauda regions exhibited higher intensity of Cldn-3 labeling in TJs of epithelial cells than the pIS and dIS regions (; right panels and ).
During the rainy season, Cldn-4 labeling was weak in TJs of pIS and dIS, moderate in the caput and corpus, and intense in the cauda region (; left panels and ). In the dry season, the cauda and corpus regions showed the highest intensity of Cldn-4 labeling, whereas the dIS and pIS showed the lowest intensity of Cldn-4 labeling (; right panels and ).
3.5. Localization in the basolateral membrane
Cldn-1 labeling was present at low levels in the basolateral membrane of epithelial cells in the pIS, dIS and caput, regardless of the season (; ). The highest basolateral membrane expression levels were detected in the cauda region during the rainy season (; left panels and ). By contrast, brighter Cldn-1 basolateral membrane labeling was detected in the cauda and corpus regions of the duct during the dry season (; right panels and ).
While the low detection of Cldn-3 labeling was evident in the basolateral membrane of epithelial cells located in the pIS and dIS during the rainy season, the labeling intensity progressively increased from the caput to the cauda regions (; left panels). In the dry season, Cldn-3 expression was detected in the pIS, dIS, and caput at low levels compared to the other epididymal regions (; right panels). Interestingly, the intensity of Cldn-3 labeling was greater in the pIS, dIS, and caput in the dry season than the rainy season (; ). Finally, the IGP of Cldn-3 labeling was higher in the cauda region compared to the dIS, pIS, and caput regions, regardless of the seasons ().
Cldn-4 labeling was low in the pIS and dIS during the rainy season (; left panels and ). By contrast, Cldn-4 was present in the basolateral membranes at moderate levels in the caput, increasing its intensity in the corpus and cauda regions (; left panels and ). In the dry season, Cldn-4 was expressed at low levels in the pIS and dIS compared to the caput, corpus, and cauda regions (; right panels and ). Similarly to Cldn-3, the intensity of Cldn-4 labeling in the pIS and dIS during the dry season was higher compared to the rainy season (; right panels and ).
With regards to basal cells, Cldn-1 was expressed in their membranes in all segments of D. rotundus’ epididymis during the rainy and dry seasons (, arrowheads). Moreover, in both seasons, this cell type also expressed Cldn-3 and −4 in the caput, corpus, and cauda regions ( and , arrowheads). The most important difference in their expression was observed in the initial segment. While those Cldns were expressed in basal cell membranes in the proximal and distal initial segments in the dry season, no Cldn-3 and −4 labeling were detected in basal cells in both segments during the rainy season.
4. Discussion
This is the first description of the occurrence and distribution of TJ proteins in bat epididymis. TJ structure was first described in this organ by Citation32,using transmission electron and freeze-fracture electron microscopy. Notwithstanding, the use of immunolabeling improved the knowledge concerning the organizational subtleties of TJs, including the identification of its protein components and their interactions.Citation33,Citation34 Herein, these cell-cell adhesion structures were assessed in D. rotundus epididymis using transmission electron microscopy and epifluorescence. Upon both techniques, we observed the BEB presenting TJ mesh networks between principal cells and clear cells, as previously described,Citation14,Citation20,Citation35 with no participation of basal cells. The contribution of basal cells to TJ formation may occur when this cell emits axiopodia, which cross the apical barrier creating new TJs between basal cells and adjacent epithelial cells in a dynamic and temporary way.Citation16,Citation36-Citation38 While basal cell axiopodia have been detected in Sprague-Dawley rats and mice,Citation16,Citation38 to date, this cell extension has not been detected in D. rotundus yet.Citation27,Citation39 Altogether, epithelial cells, connected by TJs, constitute the highly efficient BEB that creates a unique luminal microenvironment critical for sperm maturation,Citation14,Citation40 and controls the unidirectional transport of ions, water, and solutes influencing tissue permeability.Citation8,Citation15
The expression of Cldns and ZO-1 observed in this study has been previously described in human, rat, and mouse BEB using anti-rabbit polyclonal antibodies and an anti-rat monoclonal antibody, respectively.Citation11,Citation14-Citation16,Citation41 Likewise, these antibodies successfully bound to specific proteins in the epididymis of D. rotundus, indicating a possible cross-reactivity of the primary antibodies with proteins in bat tissues. This phenomenon has also been observed in our previous study using other polyclonal antibodies for specific markers of epididymal cells,Citation27 which were also used here. Our negative controls clearly showed that the antibodies are working with specificity in each TJ protein from bat tissue (Supplementary information S1).
The expression pattern of ZO-1 observed in D. rotundus epididymis is also documented in epididymis of rodents.Citation10,Citation15,Citation19 So far, only young rats exhibited this peripheral membrane protein in lateral plasma membranes of epididymal cells.Citation20 ZO-1, as well as ZO-2 and ZO-3, are scaffolding proteins of TJs that link membrane proteins to the actin cytoskeleton in various epithelia.Citation6 Particularly, Citation15,reported that ZO-1 exerts a more fundamental role in epididymal TJ dynamics than ZO-2 and ZO-3, being involved in the targeting of Cldns and occludin to the area of TJs, anchoring these transmembrane proteins at the extracellular seal.Citation19,Citation20 In this study, we have tried to label occludin, ZO-2, and ZO-3 in bat epididymis [according toCitation15] with no success, although the conservation level of those TJ proteins is more than 75% between rodents and bats. We may speculate that, differently to the antibodies used here, anti-rat polyclonal antibodies for those proteins did not show enough cross-reactivity for labeling specific proteins in bat tissue.
Overall, ZO-1 was detected together with Cldns in the TJs along the length of epididymal duct. The presence of Cldn-1, −3, and −4 in TJs, as well as in basolateral membranes, is apparently a conserved feature in rodent and human epididymis,Citation14-Citation16 and now in bats. Collectively, they form the BEB that controls the exchange of molecules between blood and lumen providing an immune-privileged environment adequate for spermatozoa since they can produce an immune response.Citation8,Citation11,Citation12 Moreover, their presence in the apical portion of the epithelium is necessary for cellular polarity and maintenance of cell structure. While the significance of their location outside of the TJs remains unknown, Cldns may serve as reserves of Cldns prior to their incorporation into the TJs.Citation14,Citation20 Moreover, their recruitment to basolateral membranes does not require the participation of ZO-1.Citation15 Changes in expression levels of Cldns and combination of claudin types may alter tissue permeability.Citation7,Citation21,Citation42 Studies have reported at least seven different Cldns in the adult rat epididymis, whose expression levels can vary dramatically between epididymal regions.Citation7,Citation11,Citation20,Citation37 Basically, Cldn-1, −3 and −4 are types related to sealing functions, whereas Cldn-2 and −10 may provide paracellular permeability, and others have ambiguous functions.Citation22
In basal cells, the expression of Cldn-3 and −4, as well as Cldn-1, in their membranes that we observed here in vampire bat epididymis has been previously described in rodents.Citation14,Citation37,Citation40 AlthoughCitation16, proposed that Cldn-1 would provide a “molecular ladder” for supporting basal cell elongation, andCitation15, proposed Cldn-4 as an additional potential mediator of axiopodia extensions of basal cells, in the present study, we may suggest that these Cldns may have a role in cell adhesion and paracellular transportCitation21 rather than supporting basal cell elongation.
Our findings from ELISA assay showed that the effect of season on protein expression was weak. Indeed, the lowest expression magnitude of ZO-1 in corpus region during the dry season determined by this method was not reflected in the histological analysis. Additionally, ZO-1 occurrence was apparently similar in TJs along the epididymal epithelium. Despite the fact that the total protein expression of Cldn measured by ELISA was not affected by seasons in the proximal portion (initial segments and caput), corpus and cauda, their occurrence and distribution in the epithelium of bat epididymis did show variations by confocal microscopy. Precisely, the immunolabeling method showed that initial segments were the main target regions for those changes. Of note, the similarity in Cldn-3 and −4 expression levels in both seasons by ELISA may be explained by the evaluation of the initial segments along with caput tissue in this assay. Indeed, the initial segment is a very small structure, which limited its protein characterization.
To date, the regulation of epididymal TJs and BEB is poorly understood. Authors have suggested that testosterone and other testicular factors might regulate the integrity of BEB, especially Cldn-1 expression.Citation7,Citation14 A recent study reported that serum testosterone levels did not change in D. rotundus captured in both rainy and dry seasons,Citation26 indicating that other factors may be regulating the histological alterations observed here. In mink, low testosterone levels at the end of reproductive season did not modify BEB structure in contrast to the alterations found in its blood-testicular barrier.Citation10,Citation43 Therefore, we may suggest that testosterone regulates the expression of Cldn-1 in basolateral membranes and TJs, but, in the latter, it is not the sole regulator of its expression.
Coincidently, the initial segments are the epididymal regions mostly influenced by testicular luminal fluid factor, so-called lumicrine factors, which are produced by testis and enter the epididymis with testicular luminal fluid. Their identity is not well known, but multiple growth factors, including fibroblast growth factors and epidermal growth factors, as well as high levels of androgens and androgen binding proteins may be involved.Citation15,Citation44 Considering that growth factors were described in the regulation of Cldn expression in other tissues,Citation45-Citation47 we may suggest that lumicrine factors also regulate the expression of Cldn-1, −3, and −4 in the apical portion of epididymal epithelium in the initial segment of bats. Most recentlyCitation15, demonstrated the contribution of the MAPK/ERK pathway to the maintenance of Cldn-1 and Cldn-4 expression, but not Cldn-3 expression.
Furthermore, the maintenance of Cldn-4 and −3 expression in TJs of initial segments, as well as Cldn-4 in basolateral membranes of cells located in other segments may be related to the role of Cldns to provide a luminal environment suitable for sperm maturation.Citation4,Citation20 Specifically, factors associated with the entry of spermatozoa in the epididymal lumen may influence Cldn-4 expression levels.Citation20 In this study, Cldn-3 showed similar patterns of distribution and expression intensity than Cldn-4. So, it is likely that they exert complementary functions in epididymis. The co-localization of different Cldns may indicate interaction with each other, which would determine tissue specific paracellular permeability.Citation22 In other epithelia, Cldn-3 and −4 are heteromerically compatible when expressed in the same cell.Citation4 Nonetheless, more studies are necessary to better understand the Cldn-3 dynamic and function in the epididymis.
Ultimately, the low intensity of Cldn-3 and −4 expressions in basolateral membranes of cells, including basal cells, in the initial segments during the rainy season is intriguing. It may have a relationship with the reproductive behavior in this species. Although D. rotundus bats were not evaluated monthly during rainy season by Citation26, this species exhibited intense activity of seminiferous epithelium in producing mature cells after the dry season that corresponds to their mating season, with consequent increase in sperm numbers in caput/corpus epididymis.Citation26 Thus, it is possible that lumicrine contents may differ between the rainy and dry season. Notably, the profile of luminal fluid content display substantial segment-to-segment variations,Citation44 and these differences may dramatically change during mating season.
In summary, D. rotundus epididymis exhibits ZO-1, Cldn-1, −3, and −4 labeling in TJs and basolateral membranes of epithelial cells. However, their distribution pattern varied between epididymal regions with respect to intensity of expression and co-localization. Moreover, the effect of seasons on protein expression influenced mostly the initial segments, with low expression of Cldn-1 in TJs during the rainy season and Cldn-3 and −4 in basolateral membranes in the rainy season. Lumicrine factors rather than testosterone levels may be regulating such alterations. It is worthy to mention that our data were generated over a single year. Thus, we suggest a more comprehensive sampling (e.g. consecutive months and years) in future studies. Nevertheless, our results are the first pieces of information about the BEB components and the distribution of membrane proteins in the epididymis of D. rotundus, which contributes to a better understanding of its reproductive biology.
Disclosure of potential conflicts of interest
No potential conflicts of interest were disclosed
Author contributions
M.M.C., B.K., S.B., J.E.S., and M.M.-N: Study design and writing of the manuscript. M.M.C, B.K. and E.H.: Immunohistochemistry study. M.M.C., P.D.G., J.E.S.: ELISA assay. C.A.N., M.M.C. and M.M.-N: Morphometry. M.M-N.: Statistical analysis.
Additional information
Competing financial interests: The authors declare no competing financial interests.
Supplemental Material
Download Zip (4 MB)Acknowledgments
We thank Luciano C. H. Puga, Jerusa M. Oliveira, Bruno E. S. Melo and Susana P. Ribeiro for their help during fieldwork and euthanasia. This work was supported by National Institute of Health (NIH grants HD040793 and DK097124, to Sylvie Breton), the Brazilian agency Coordenação de Aperfeiçoamento de Pessoal de Nível Superior (CAPES) (proc. 99999.011031/2013-01 to M.M. Castro), Fundação de Amparo à Pesquisa do Estado de Minas Gerais (FAPEMIG; grant number APQ-02514-16 to M.M-N.), and Conselho Nacional de Desenvolvimento Científico e Tecnológico [grant number 420077/2018-9 to M.M-N.; Fellowship granted to the research in productivity 302088/2018-0 to M.M-N.].
Supplementary material
Supplemental data for this article can be accessed on the publisher’s website.
Additional information
Funding
References
- Parrish AR. The impact of aging on epithelial barriers. Tissue Barriers. 2017;5:1. PMID: 28686506doi:10.1080/21688370.2017.1343172.
- Tokuda S, Yu ASL. Regulation of epithelial cell functions by the osmolality and hydrostatic pressure gradients: a possible role of the tight junction as a sensor. Int J Mol Sci. 2019;20:E3513. PMID: 31319610. doi:10.3390/ijms20143513.
- Al-Asmakh M, Hedin L. Microbiota and the control of blood-tissue barriers. Tissue Barriers. 2015;3(3):e1039691. PMID: 26451344. doi:10.1080/21688370.2015.1039691.
- Angelow S, Ahlstrom R, Yu ASL. Biology of claudins. Am J Physiol Renal Physiol. 2008;295(4):F867–17. PMID: 18480174. doi:10.1152/ajprenal.90264.2008.
- Caceres PS, Benedicto I, Lehmann GL, Rodriguez-Boulan EJ. Directional fluid transport across organ-blood barriers: physiology and cell biology. Cold Spring Harb Perspect Biol. 2017;9:a027847. PMID: 28003183. doi:10.1101/cshperspect.a027847.
- Van Itallie CM, Anderson JM. Architecture of tight junctions and principles of molecular composition. Semi Cell Dev Biol. 2014;36:157–165. PMID: 25171873. doi:10.1016/j.semcdb.2014.08.011.
- Cyr DG, Gregory M, Dubé É, Dufresne J, Chan PTK, Hermo L. Orchestration of occludins, claudins, catenins and cadherins as players involved in maintenance of the blood-epididymal barrier in animals and humans. Asian J Androl. 2007;9:463–475. PMID: 17589783doi:10.1111/j.1745-7262.2007.00308.x.
- Mital P, Hinton BT, Dufour JM. The blood-testis and blood-epididymis barriers are more than just their tight junctions. Biol Reprod. 2011;84:851–858. PMID: 21209417. doi:10.1095/biolreprod.110.087452.
- França LR, Auharek SA, Hess RA, Duffour JM, Hinton BT. Blood-tissue barriers: morphofunctional and immunological aspects of the blood-testis and blood epididymal barriers. Adv Exp Med Biol. 2012;763:237–259. PMID: 23397628. doi:10.1007/978-1-4614-4711-5_12.
- Cyr DG, Robaire B, Hermo L. Structure and turnover of junctional complexes between principal cells of the rat epididymis. Microsc Res Tech. 1995;30:54–66. PMID: 7711320. doi:10.1002/jemt.1070300105.
- Dubé É, Dufresne J, Chan PTK, Hermo L, Cyr DG. Assessing the role of claudins in maintaining the integrity of epididymal tight junctions using novel human epididymal cell lines. Biol Reprod. 2010;82(6):1119–1128. PMID: 20164436. doi:10.1095/biolreprod.109.083196.
- Gregory M, Cyr DG. The blood- epididymis barrier and inflammation. Spermatogenesis. 2014;4:e979619. PMID: 26413391. doi:10.4161/21565562.2014.979619.
- Dubé E, Chan PT, Hermo L, Cyr DG. Gene expression profiling and its relevance to the blood-epididymal barrier in the human epididymis. Biol Reprod. 2007;76(6):1034–1044. PMID: 17287494. doi:10.1095/biolreprod.106.059246.
- Gregory M, Dufresne J, Hermo L, Cyr D. Claudin-1 is not restricted to tight junctions in the rat epididymis. Endocrinology. 2001;142:854–863. PMID: 11159859. doi:10.1210/endo.142.2.7975.
- Kim B, Breton S. The MAPK/ERK-signaling pathway regulates the expression and distribution of tight junction proteins in the mouse proximal epididymis. Biol Reprod. 2016;94:1–12. PMID: 26658708. doi:10.1095/biolreprod.115.134965.
- Shum WWC, Da Silva N, McKee M, Smith PJ, Brown D, Breton S. Transepithelial projections from basal cells are luminal sensors in pseudostratified epithelia. Cell. 2008;135:1108–1117. PMID: 9070580. doi:10.1016/j.cell.2008.10.020.
- Furuse M. Molecular basis of the core structure of tight junctions. Cold Spring Harb Perspect Biol. 2010;2(1):a002907. PMID: 20182608. doi:10.1101/cshperspect.a002907.
- Haskins J, Gu L, Wittchen ES, Hibbard J, Stevenson BR. ZO-3, a novel member of the MAGUK protein family found at the tight junction, interacts with ZO-1 and occludin. J Cell Biol. 1998;141(1):199–208. PMID: 9531559. doi:10.1083/jcb.141.1.199.
- Levy S, Robaire B. Segment-specific changes with age in the expression of junctional proteins and the permeability of the blood-epididymis barrier in rats. Biol Reprod. 1999;60(6):1392–13401. PMID: 10330098. doi:10.1095/biolreprod60.6.1392.
- Gregory M, Cyr DG. Identification of multiple claudins in the rat epididymis. Mol Reprod Dev. 2006;73(5):580–588. PMID: 16489631. doi:10.1002/mrd.20467.
- Lingaraju A, Long TM, Wang Y, Austin JR, Turner JR. Conceptual barriers to understanding physical barriers. Semin Cell Dev Biol. 2015;42:13–21. PMID: 26003050. doi:10.1016/j.semcdb.2015.04.008.
- Markov AG, Aschembach JR, Amasheh S. Claudin clusters as determinants of epithelial barrier function. IUBMB Life. 2015;67:29–35. PMID: 25788154. doi:10.1002/iub.1347.
- Cyr DG, Hermo L, Egenberger N, Mertineit C, Trasler JM, Laird DW. Cellular immunolocalization of occludin during embryonic and postnatal development of the mouse testis and epididymis. Endocrinology. 1999;140:3815–3825. PMID: 10433243. doi:10.1210/endo.140.8.6903.
- Streiker DG, Winternitz JC, Satterfield DA, Condori-Condori RE, Bross A, Tello C, Recuenco S, Velasco Villa A, Altizer S, Walderrama W. Host-pathogen evolutionary signatures reveal dynamics and future invasions of vampire bat rabies. Proc Natl Acad Sci USA. 2016;113:10926–10931. PMID: 27621441. doi:10.1073/pnas.1606587113.
- Delpietro HA, Russo RG, Carter GG, Lord RD, Delpietro GL. Reproductive seasonality, sex ratio and philpatry in Argentina´s common vampire bats. R Soc Open Sci. 2017;4:160959. PMID: 28484615. doi:10.1098/rsos.160959.
- Souza ACF, Santos FC, Bastos DSS, Sertorio MN, Teixeira JPG, Fernandes KM, Machado-Neves M. Reproductive functions in Desmodus rotundus: A comparison between seasons in a morphological context. PLoS One. 2018;13:e0205023. PMID: 30332444. doi:10.1371/journal.pone.0205023.
- Castro MM, Kim B, Hill E, Fialho MCQ, Puga LCHP, Freitas MB, Breton S, Machado-Neves M. The expression. Patterns of aquaporin 9, vacuolar H+-ATPase, and cytokeratin 5 in the epididymis of the common vampire bat. Histochem Cell Biol. 2017a;147:39–48. PMID: 27549752. doi:10.1007/s00418-016-1477-9.
- Guerra A, King JM, Alajajian B, Isgor E, Digicaylioglu M. Occludin and claudin-5 are comparably abundant and co-localized in the rat´s blood brain barrier from late gestation to adulthood. e-J Neonatol Res. 2011;1:31–43.
- Kim B, Roy J, Shum WW, Da Silva N, Breton S. Role of testicular luminal factors on basal cell elongation and proliferation in the mouse epididymis. Biol Reprod. 2015;92(1):1–11. PMID: 25411392. doi:10.1095/biolreprod.114.123943.
- Shum WWC, Hill E, Brown D, et al. Plasticity of basal cells during postnatal development in the rat epididymis. Reproduction 2013; 146:455-469. doi:https://doi.org/10.1530/REP-12-0510.
- Menezes TP, Hill E, Moura AA, Lobo MDP, Monteiro-Moreira ACO, Breton S, Machado-Neves M. Pattern of protein expression in the epididymis of Oligoryzomys nigripes (Cricetidae, Sigmodontinae). Cell Tissue Res. 2018;372:135–147. PMID: 29119327. doi:10.1007/s00441-017-2714-9.
- Friend DS, Gilula NB. Variations in tight and gap junctions in mammalian tissues. J Cell Biol. 1972;53(3):559–571. PMID: 4337577. doi:10.1083/jcb.53.3.758.
- Hermo L, Korah N, Gregory M, Liu LY, Cyr DG, D`Azzo A, Smith CE. Structural alterations of epididymal epithelial cells in cathepsin A-deficient mice affect the blood-epididymal barrier and lead to altered sperm motility. J Androl. 2007;28:784–797. PMID: 17522420. doi:10.2164/jandrol.107.002980.
- Stevenson BR, Siliciano JD, Mooseker MS, Goodenough DA. Identification of ZO-1: a high molecular weight polypeptide associated with the tight junction (zonula occludens) in a variety of epithelia. J Cell Biol. 1986;103:755–766. PMID: 3528172. doi:10.1083/jcb.103.3.755.
- Hinton BT, Palladino MA. Epididymal epithelium: its contribution to the formation of a luminal fluid microenvironment. Micro Res Tech. 1995;30:67–81. doi:10.1002/jemt.1070300106.
- Arrighi S. Are the basal cells of the mammalian epididymis still an enigma? Reprod Fertil Dev. 2014;26(8):1061–1071. PMID: 24138802. doi:10.1071/RD13301.
- Mandon M, Cyr D. Tricellulin and Its Role in the Epididymal Epithelium of the Rat. Biol Reprod. 2015;92:66. PMID: 25568308. doi:10.1095/biolreprod.114.120824.
- Roy J, Kim B, Hill E, Visconti P, Krapf D, Vinegoni C, Weissleder R, Brown D, Breton S. Tyrosine kinase-mediated axial motility of basal cells revealed by intravital imaging. Nat Commun. 2016;7:10666. PMID: 26868824. doi:10.1038/ncomms10666.
- Castro MM, Gonçalves WG, Teixeira SAMV, Fialho MCQ, Santos FC, Oliveira JM, Serrão JE, Machado-Neves M. Ultrastructure and morphometric features of epididymal epithelium in Desmodus rotundus. Micron. 2017b;102:35–43. PMID: 28869875. doi:10.1016/j.micron.2017.08.006.
- Smith TB, Cortez-Retamozo V, Grigoryeva LS, Hill E, Pittel MJ, Da Silva N. Mononuclear phagocytes rapidly clear apoptotic epithelial cells in the proximal epididymis. Andrology. 2014;2:755–762. PMID: 25082073doi:10.1111/j.2047-2927.2014.00251.x.
- Ruan YC, Shum WW, Belleannée C, Da Silva N, Breton S. ATP secretion in the male reproductive tract: essential role of CFTR. J Physiol. 2012;590:4209–4222. PMID: 22711960. doi:10.1113/jphysiol.2012.230581.
- González-Mariscal L, Domínguez-Calderón A, Raya-Sandino A, Ortega-Olvera JM, Vargas-Sierra O, Martínez-Revollar G. Tight junctions and the regulation of gene expression. Semin Cell Dev Biol. 2014;36:213–233. PMID: 25152334. doi:10.1016/j.semcdb.2014.08.009.
- Pelletier R-M. Cyclic modulation of sertoli cell junctional complexes in a seasonal breeder: The mink (Mustela vison). Am J Anat. 1988;183:68–102. doi:10.1002/(ISSN)1553-0795.
- Xu B, Washington AM, Hinton BT. Initial segment differentiation begins during a critical window and is dependent upon lumicrine factors and SRC proto-oncogene (SRC) in the mouse. Biol Reprod. 2016;95(15):1–11. PMID: 27281706. doi:10.1095/biolreprod.116.138388.
- Chakraborty P, William Buaas F, Sharma M, Smith BE, Greenlee AR, Eacker SM, Braun RE. Androgen-dependent Sertoli cell tight junction remodeling is mediated by multiple tight junction components. Mol Endocrinol. 2014;28:1055–1072. PMID: 24825397. doi:10.1210/me.2013-1134.
- Suzuki T. Regulation of intestinal epithelial permeability by tight junctions. Cell Mol Life Sci. 2013;70:631–659. PMID: 22782113. doi:10.1007/s00018-012-1070-x.
- Yang J, Kim WJ, Jun HO, Lee EJ, Lee KW, Jeong JY, Lee SW. Hypoxia-induced fibroblast growth factor 11 stimulates capillary-like endothelial tube formation. Oncol Rep. 2015;34:2745–2751. PMID: 26323829doi:10.3892/or.2015.4223.