ABSTRACT
The Gastrointestinal (GI) tract is composed of four main barriers: microbiological, chemical, physical and immunological. These barriers play important roles in maintaining GI tract homeostasis. In the crosstalk between these barriers, microbiota and related metabolites have been shown to influence GI tract barrier integrity, and alterations of the gut microbiome might lead to an increase in intestinal permeability. As a consequence, translocation of bacteria and their products into the circulatory system increases, reaching proximal and distal tissues, such as the liver. One of the most prevalent chronic liver diseases, Nonalcoholic Fatty Liver Disease (NAFLD), has been associated with an altered gut microbiota and barrier integrity. However, the causal link between them has not been fully elucidated yet. In this review, we aim to highlight relevant bacterial taxa and their related metabolites affecting the GI tract barriers in the context of NAFLD, discussing their implications in gut homeostasis and in disease.
1. Introduction
The GI tract is responsible for the processing of food and allows the survival of commensal microorganisms while eliminating pathogens.Citation1 The GI tract is a complex structure composed of four main barriers: microbiological, chemical, physical and immunological, which all play key roles in the maintenance of the system homeostasis. The gut microbiota modulates the pool of metabolites present along the GI tract.Citation2 Depending on their origin these metabolites can be classified into three types: (1) metabolites resulting from the transformation by the microbiota of dietary components (e.g. Short-Chain Fatty Acids, SCFAs);Citation3 (2) host secreted metabolites which are modified by the microbiota (e.g. secondary bile acids)Citation4 and (3) metabolites synthesized de novo (e.g. vitamin K).Citation5 All these metabolites can affect different processes in the GI tract of the host.Citation2 For instance, butyrate,Citation6 tryptophan derivativesCitation7 or ethanolCitation8 have been shown to affect the intestinal permeability with different mechanisms. Perturbations in GI barrier functions might lead to increased gut permeability; a condition also termed “leaky gut”, which, in turn, would allow for a higher flow of bacteria and bacterial products to the bloodstream, contributing to liver damage.Citation9–11 Non-alcoholic fatty liver disease (NAFLD) is an umbrella term encompassing several liver disorders. In individuals who consume little or no alcohol, nonalcoholic fatty liver (NAFL) can progress from simple liver steatosis to nonalcoholic steatohepatitis (NASH). Typically, NASH is accompanied by pericellular fibrosis, which may further progress to irreversible cirrhosis and ultimately hepatocellular carcinoma.Citation12–14 Its prevalence has increased worldwide and doubled over the last 20 years, as it is thought to be present in more than 20% of the general population, particularly affecting people suffering from Type 2 Diabetes Mellitus (T2DM).Citation15 Although the mechanisms behind the progression of NAFLD are not well understood yet, several factors contribute to its development and progression. Alterations in the GI microbiota (e.g. changes in microbiota composition, species diversity, community structure and/or function), age, diet and lifestyle, host metabolism, and genetic predisposition are thought to play an important role. These risk factors have been addressed in several reviews.Citation13,Citation16,Citation17
The important role of the gut microbiota in NAFLD has been established both in preclinical and clinical studies.Citation10,Citation18 Qualitative and quantitative alterations in the microbiota impact the GI barrier function and structure, increasing GI tract permeability, contributing to liver damage. In fact, patients with NAFLD show increased intestinal permeability that correlates with the severity of steatosis.Citation11,Citation19,Citation20 Moreover, recent research has shown that the disruption of the GI vascular barrier is also required for NAFLD pathogenesis.Citation19 Most of the NAFLD studies are focused on establishing a correlation between bacterial microbiota composition and the disease state, but the causal mechanisms linking them have not been fully elucidated. For that purpose, in the present review, we aim to highlight relevant bacterial metabolites and taxa affecting the GI tract barriers in NAFLD. Their implications in gut homeostasis and in disease will be discussed. Consequently, this review can contribute to understanding NAFLD pathogenesis, further elucidating the mechanisms that lead to gut barrier dysfunction associated with NAFLD.
2. GI tract structure and function
The GI tract is composed of different segments reflecting their specific physiological functions.Citation21 In this review, we will focus specifically on small and large intestines. The small intestine includes duodenum, jejunum and ileum. Its main functions are the digestion and absorption of nutrients from the diet.Citation22 The large intestine, the last part of the GI tract, is formed by cecum, proximal colon, distal colon and rectum and its primary functions are absorbing water, electrolytes and forming luminal content for elimination.Citation23 The large intestine is also the place where commensal bacteria produce vitamins and Short Chain Fatty Acids (SCFAs).Citation1 The GI tract plays important roles in maintaining the homeostasis between the internal and external environments and it is composed of several barriers: 1) the microbiological barrier composed of the commensal microorganisms;Citation24 2) the chemical barrier represented by secreted molecules that provide a defense against invading microorganisms;Citation25–27 3) the physical barrier regulating nutrient uptake and protecting the intestinal mucosa from pathogen invasion;Citation28–30 and 4) the immunological barrier including a variety of immune cells, which, during pathogenic conditions, are mobilized to fight and clear invading microbes ().Citation31 This review focuses on the structure and function of the microbiological, chemical and, with more emphasis, physical barriers. The role of the immune system in the GI tract as a barrier will be not described in this review. There are several excellent and extensive reviews on the role of the mucosal immune system, which we recommend for further reading, but are outside the scope of this review.Citation31–33
Figure 1. Structure of microbiological, chemical, physical and immunological barriers in the intestine. The microbiological barrier resides in the intestinal lumen, over and inside the mucus. It is composed of intestinal microbiota including bacteria, fungi, archaea and viruses. The mucus layer forms part of the physical barrier (coating with a single layer the small intestine and with a double layer the large intestine), in which AMP, BA and SIgA are secreted and they act as chemical barriers. The mucus covers the intestinal epithelial cells, which are also considered part of the physical barrier. Moreover, these cells are tightly sealed by TJs (claudins, occludin, ZOs), JAM-A and by AJs (E-cadherin) controlling the paracellular permeability. Scattered throughout the epithelium and lamina propria, innate immune cells (i.e. macrophages, dendritic cells and lymphoid cells) and adaptive immune cells (i.e. T and B cells) reside. In the lamina propria of the gut mucosa, endothelial cells tightly sealed by claudin, occludin, JAM-A and VE-cadherin proteins form a gut vascular barrier.; AJs: adherens junctions, AMP: antimicrobial peptides, BA: bile acids, SIgA: Immunoglobulin A, JAM-A: TJs-associated adhesion molecules, TJs: tight junctions, VE-cadherin: vascular-endothelial cadherin, ZOs: zonula occludens
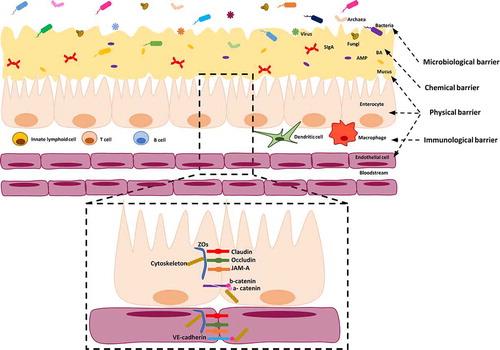
2.1. Microbiological GI tract barrier
The first and outer GI tract barrier is represented by intestinal microbiota, referring to the entire population of microorganisms colonizing the GI tract ().Citation24 It includes not just bacteria, but also fungi, archaea, yeasts and viruses, that have a mutualistic relationship among themselves and with their host.Citation34 The symbiotic relationship between microbiota and host has several effects on the GI tract physiology: 1) it influences the mucosal innate and adaptive immune system;Citation35 2) it provides metabolic functions assisting the digestion and fermentation of food that is important for the production of vitamins and SCFAs;Citation5 and 3) it also provides defense against pathogens competing for food and adhesion sites in the GI tract, some even actively eliminating competitors by secreting antimicrobial peptides (AMPs).Citation36 Qualitative or quantitative alterations in the microbiota can impair this symbiotic relationship, decreasing the GI tract barrier protection.Citation37,Citation38 These alterations have been shown to play a role in NAFLD and will be discussed more in-depth later in this review. It is noteworthy that here terms like “gut microbiota” refer to the totality of the organisms inhabiting our GI tract, leaving a potential higher-than-expected role in gut-related disorders to eukaryotic microorganisms, protozoa, archaea and parasites, all of which have been highly understudied in the past years.Citation39,Citation40 Recent research in the virome highlighted that not only bacterial diversity is reduced in NAFLD, but that the indicators of NAFLD also correlate with significant decreases in viral diversity and in the proportion of bacteriophages.Citation40 On the other hand, You et al.,Citation39 recently reviewed the current knowledge of intestinal fungi in different liver diseases. They reported that an imbalance in fungal populations also occurs in patients with metabolic syndrome and that oral supplementation with Saccharomyces boulardii alleviated hepatic steatosis and inflammation. The under-studied role of viruses and fungi has affected the way we use terms like “gut microbiota” or “microbiome”, which can easily be misused, or misinterpreted to represent only a portion of the total biodiversity of the GI-tract. For example, the microbiome is more than the bacteriome.Citation41 A more precise term should then be used when speaking about the changes in (only) bacterial composition or abundance. Hence, in this review, we will use the term bacterial gut microbiota (BGM) to stress the difference from general statements about the gut microbiota as a whole.
2.2. Chemical GI tract barrier
The chemical barrier in the GI tract is composed of secreted molecules, such as host secreted AMPs,Citation25 bile acids (BAs)Citation26 and secretory Immunoglobulin A (SIgA)Citation27 (). BAs are derivatives of cholesterol metabolism and they are considered to have a direct antimicrobial effect by destroying bacterial membranes because of their detergent-like properties.Citation42 The function of BAs will be further explained later in this review. AMPs are mainly produced by enterocytes and Paneth cells, and also macrophages placed in the gut mucosa can contribute to their production.Citation43–45 The number of Paneth cells changes along the GI tract, with the highest amounts in the ileum.Citation46,Citation47 Paneth cells are lacking in the large intestine, which leads to a different AMPs composition in the large intestine compared to the small intestine.Citation48 The most common secreted AMPs in the GI tract include defensins (α and β), cathelicidins (LL-37/CRAMP) and C-type lectins (RegIII α/γ/β). AMPs target components of the bacterial cell wall, although different AMP families use different mechanisms to exert their antimicrobial activity, including enzymatic and non-enzymatic activities.Citation25 AMPs expression is regulated by diverse mechanisms and some of them require bacterial signals for their expression. For instance, bacteria and bacterial products, but not fungi or protozoa, induce the release of some AMPs, such as αCitation49 and β defensins.Citation50 AMPs also control the access/interaction of the gut microbiota with the intestinal mucosa as shown, for example, in mice lacking RegIIIγ, in which altered mucus distribution has been observed, as well as increased contact of microbiota with intestinal epithelium and inflammation in ileum mucosa.Citation51 Dysfunctions in AMPs activity have also been observed in intestinal bowel diseases. Additionally, AMPs have been shown to play an important role in host-microbiota interactionCitation52 and in promoting barrier integrity.
Inside the mucus, SIgAs are the main immunoglobulins secreted on the intestinal mucosal surface and contribute to maintaining the homeostasis in the intestine. SIgAs are produced by plasma cells inside the mucosa, transported by the polymeric Ig receptor (pIgR) inside IECs and secreted into the lumen. SIgAs are able to neutralize invading microorganisms mainly interfering with the microbial adherence to IECs by forming intraluminal immune complexes. This process, defined as immune exclusion, prevents colonization and damage to IECs. SIgAs can also neutralize the invading pathogens that penetrate inside the lamina propria of the mucosa.Citation53In addition, SIgAs are able to modulate the microbiota composition participating in the maintenance of GI tract homeostasis.Citation54 And, their importance in GI tract has been shown in activation-induced cytidine deaminase (AID) deficient mice in which the SIgAs production is lacking due to the missing switch from Immunoglobulin M (IgM) to IgA. In the intestine of these mice an hyperplasia of lymphoid follicles has been observed suggesting that SIgAs are essential in preventing hyper-stimulation of the mucosal immune system.Citation55 Despite the importance of SIgA in the GI tract, individuals with IgA deficiency do not present specific symptoms. This could be explained as a compensatory mechanism by an increased production of IgM observed in the intestine of these individuals.Citation56 But it has been also reported that individuals with IgA deficiency could suffer from pulmonary infections, allergies and GI tract disorders.Citation57
2.3. Physical GI tract barrier
The physical barrier in the GI tract consists of mucus layers, IECs and endothelial cells (ECs) (). They physically avoid the invasion of microorganisms through the mucosa, preventing the translocation of bacteria and bacterial components to the bloodstream.Citation28–30 However, their features vary between the small and large intestine. The small intestine has a smaller radius than the large intestine, and its epithelium is folded forming villi (evaginations projected toward the intestinal lumen) and crypts (invaginations occurring at the base of the villi). Intestinal stem cells found at the base of the crypts divide, migrate and differentiate continuously into enterocytes, goblet cells, Paneth cells or enteroendocrine cells, whose physiological roles are respectively the absorption, mucus production, antimicrobial production and hormone secretion. Besides, crypts contain mainly stem cells and Paneth cells, while enterocytes are predominantly present in the villi, and enteroendocrine and goblet cells are distributed in the upper crypt and the villi. In contrast, the large intestine lacks Paneth cells and villi, and only crypts are present along it.Citation58 Moreover, the interaction of the different cell types with the microbiota will not be equal, as well as, the concentration of the molecules secreted by the host will differ along the radius of the intestine.
2.3.1. The mucus layer barrier
Mucus represents the first physical barrier of the GI tract mucosa and its distribution varies between the small and large intestine. In the small intestine, a single and easy to remove layer of mucus fills up the space between the villi and covers them. However, in the small intestine, the mucus is not the main and essential mechanism of defense due to the presence of high amounts of AMPs close to the epithelium.Citation28 In fact, the mucus in the small intestine allows bacteria to attach to it as well as to obtain nutrients from it, while it also acts as a viscous medium for the chemical barrier (AMPs and SIgA).Citation59 In contrast, the large intestine is covered by two mucus layers, an inner and an outer layer. The inner layer is dense, directly in contact with the epithelium and only a specific crypt-associated microbiota is able to penetrate the mucus and thrive next to the epithelium,Citation60 while the outer mucus layer has similar properties to the mucus layer found in the small intestine. The outer layer, which derives from the inner layer, forms a less dense net-like structure where most of the microbiota reside.Citation28 Irrespective of its location in the small or large intestine, the mucus is mainly composed by the glycoprotein mucin 2 (MUC2) forming a viscous net. Goblet cells are the producers of MUC2. These cells are able also to produce other minor mucins in the intestine (i.e. MUC1, MUC3, MUC17), the intestinal trefoil factor (ITF), the AMP resistin-like molecule β (RELMβ), and Fc-γ binding protein (Fcgbp).Citation59 The importance of the mucus as a barrier has been shown in murine models, in which the lack of MUC2 leads to direct contact of microbiota with the intestinal mucosa and to severe colitis.Citation61 Interestingly, Bergstrom et al.Citation62 have recently proposed a novel spatial and dynamic organization of mucus in different segments of the large intestine. The proximal colon has been shown as the main producer of MUC2 and responsible for lumen content (microbiota and feces) encapsulation, while the distal colon produces a locally minor amount of MUC2 which adheres with that one received from the proximal colon. Moreover, the microbiota in the proximal colon is able to control the MUC2 production,Citation62 suggesting a new dynamic and continuous mucus system in the colon.
2.3.2. Intestinal epithelial cells barrier
The second physical barrier is composed of IECs, which are organized in a monolayer of columnar epithelial cells. The permeability of this barrier is regulated by both paracellular and transepithelial permeability. Paracellular permeability refers to the passage of molecules through the space between the cells, allowing the passage of small molecules, ions and water, and being impermeable to most other entities.Citation29 Transepithelial permeability regulates the transport of solutes across the IEC.Citation8
The paracellular permeability is mainly regulated by Tight Junctions (TJ) and the TJs-associated junctional adhesion molecules (JAM), residing in the apical surface of IECs (). Relevant members of TJs are occludin, claudins, zonula occludens (ZOs). All of them, with the exclusion of ZOs, are membrane proteins and they extend into the paracellular space between epithelial cells.Citation63 Occludin was the first member identified of the TJ complex.Citation64 Its function is not yet fully understood, but both in vivo and in vitro studies indicate that it might have a role in promoting the integrity of the epithelial barrier in the intestine.Citation65 Claudins, a large family of transmembrane proteins, are responsible for the regulation of paracellular permeability between epithelial cells, mainly promoting intestinal barrier integrity.Citation66,Citation67 While some of the family members are associated with increased paracellular permeability to sodium and water.Citation68,Citation69 A good balance among the various isoforms is needed for maintaining the barrier integrity, as they have differential expression along the GI tract.Citation70 JAMs are glycoproteins of the immunoglobulin superfamily composed of two extracellular immunoglobulin-like domains, one transmembrane domain and one cytoplasmic.Citation71 JAMs are expressed in different cell types, including immune cells, and colocalize with TJs in epithelial and endothelial cells.Citation72 For this reason, they are often wrongly called TJs, although they are TJ-associated proteins. The JAM molecules A and −4 are involved in the maintenance of the barrier integrity. ZOsCitation73,Citation74 are intracellular scaffold proteins and link TJsCitation75 and JAM-ACitation76 to the cytoskeleton. ZOs belong to the family of membrane-associated guanylate kinase (MAGUK)-like proteins and include three different isoforms (ZO-1, 2 and 3).Citation77
The cytoskeleton contraction is regulated by myosin light chain (MLC) and its phosphorylation by kinases such as Myosin light chain kinase (MLCK) results in the opening of the paracellular pathways.Citation78 Therefore, aberrant expression levels of this kinase can reduce the TJs barrier function, since MLCK-mediated MLC phosphorylation leads to TJ loss. Several aspects regarding their function and role in health and pathological conditions remain to be investigated.
Zonulin is a protein that has been shown to be a key player regulating TJs and it is strongly associated with increased intestinal permeability.Citation75 Zonulin is released mainly as a consequence of the intestinal lumen exposure to bacteriaCitation79 and to gliadin.Citation80 Gliadin is a gluten component which has been suggested to bind the chemokine receptor CXCR3 receptor on IECs. This leads to the recruitment of the adapter protein MyD88 and, subsequently, the expression of zonulin.Citation80 Zonulin is mostly present in the jejunum and distal ileum, but absent in the large intestineCitation77 and it is able to disassemble the TJs via polymerization of actin in a protein kinase C (PKC) dependent way.Citation78 Circulating levels of zonulin are considered an important intestinal permeability marker and has been linked to diseases, such as Type 1 DiabetesCitation79 and celiac disease.Citation80
Besides TJs controlling the paracellular permeability, the junctions complex between epithelial cells is also characterized by adherens junctions (AJs) and desmosomes. Both of them have transmembrane domains (cadherins and desmosomal cadherins) supporting the adhesion between epithelial cells and in the cytoplasm respectively connected with the actin cytoskeleton through b and a catenin (AJs) and with intermediate filaments (desmosomes).Citation81 E-cadherin is the most characterized AJ and it plays an important role in maintaining intestinal homeostasisCitation82 and intestinal barrier integrity promoting the assembly of TJs.Citation83
2.3.3. Intestinal endothelial cells barrier
ECs in the lamina propria form a third physical barrier. The gut vascular barrier (GVB) has been first described by Spadoni et al.Citation30 and it is composed of ECs surrounded by pericytes and enteric glial cells. The endothelial barrier shows similarities with the junctions complex observed in IECs. In fact, ECs are characterized by the presence of occludin, ZO-1, JAM-A and vascular endothelial cadherin (VE-cadherin), which control the GI vascular barrier integrity and the trafficking of solutes and fluids into the bloodstream.Citation30,Citation84,Citation85 PV1 is an integral membrane protein of ECs expressed when vascular permeability is increasedCitation85,Citation86 and modulated by Wnt/β-catenin pathway.Citation30 Spadoni et al.Citation30 also demonstrated that bacteria able to cross the IECs do not spread directly to the liver and spleen, due to this vascular GI barrier, thus providing evidence for the existence and importance of the GVB.Citation30 This physical barrier is not so profoundly studied as other physical barriers and its impact on diseases with intestinal mucosa disturbance followed by IECs increased permeability and/or disruption of the mucus layer/s deserves to be further investigated.
3. NAFLD and GI-tract microbiota
3.1. The gut microbiota changes during NAFLD
In NAFLD, it has been observed that several taxa of the BGM are either increased or decreased when comparing patients at different stages of the disease. This notion, as well as, further insights into NAFLD and fibrosis, their diagnostic tools and challenges; and a thorough analysis of the overlap of bacterial components of the gut microbiota between type 2 diabetes, obesity, fibrosis and NAFLD in human patients are further discussed in an outstanding, recent review.Citation87 In the following section, we will discuss these results with respect to BGM alterations and try to provide a link with damage or protection on the gut barrier. Of note, many of the physiological effects of the BGM could be attributed to their metabolites, which will be addressed later in this review.
In human studies (Supplementary Table 1), some concordant results have been reached when comparing patients with different liver conditions, as reported by Aron-Wisnewsky et al.Citation87 In particular, the γ-Proteobacteria phylum, the families Pasteurellaceae and Enterobacteriaceae and the Escherichia and Shigella genera belonging to the latter family, were increased both in NAFLD-fibrosis and in NAFLD. The same increase was observed for Streptococcaceae, Veillonellaceae, Bacteroides and Ruminococcus, but not for the Ruminococcaceae family in general. In contrast, Haemophilus and Eubacterium appeared to be consistently decreased, as well as species of the above mentioned Ruminococcaceae family. Studies on patients with NAFLD-cirrhosis showed some concordant results, such as a decrease in Clostridiales XIV, Lachnospiraceae, Eubacterium and Ruminococcaceae spp; and an increase in Escherichia or Veillonella.Citation88–90 Of note is the increase in Enterobacteriaceae, consistently reported in different liver injuries. This increase contrasts with the low abundance of Proteobacteria sequences (including Escherichia coli) encountered in healthy individuals, where they may represent ~0.1% of the bacteria in the strict anaerobic environment of the colon.Citation91,Citation92 An increase in species belonging to the Streptococcus, Veillonella and Porphyromonas genera, which typically comprise oral species, has also been reported before.Citation93,Citation94 It seems that during the onset and/or progression of NAFLD, oral species are able to migrate and colonize the gut altering the community structure. Interestingly enough, a similar decreased relative abundance of Lachnospiraceae and Ruminococcaceae, paired with increased numbers of Enterobacteriaceae, Streptococcaceae and Porphyromonadaceae, are associated with hepatic encephalopathy, the major clinical manifestation of an altered gut-liver-brain axis.Citation95
3.2. The impact of gut bacteria on intestinal permeability
3.2.1. The influence of lipopolysaccharide
The crosstalk between the GI tract and the liver has been widely addressed in the literature. In short, the GI tract-liver interaction is established by bidirectional communication via the biliary tract and the portal vein. BAs and other mediators are transported from the liver to the gut through the bile ducts, while bacterial metabolites, products and microbe-associated molecular patterns (MAMPs) translocate from the GI tract and reach the liver via the portal vein.Citation96 Therefore, intestinal permeability is a crucial aspect of maintaining homeostasis, and its perturbation could translate into different diseases. It has indeed been suggested that increased systemic inflammation could be associated with increased intestinal permeability, which would pose a higher risk factor for mortality and morbidity in older patients.Citation97 The inflammation-intestinal permeability interaction is likewise bidirectional: an increased intestinal permeability allows for a higher flow of MAMPs, such as lipopolysaccharide (LPS), pathogens and antigens leading to inflammation driven by the production of pro-inflammatory cytokines.Citation98 These cytokines can, in the gut epithelium, increment the intestinal permeability by altering TJs structure and paracellular permeability.Citation48,Citation98,Citation99 In fact, patients with NAFLD have a significantly increased intestinal permeability and alterations in the intestinal TJs in both the IECs and in the ECs, compared to healthy individuals.Citation11 The impairment in the integrity of the intestinal barrier and the GVB by the gut microbiome have been identified as prerequisites for the development of NASH.Citation19
It is therefore not surprising that the association between BGM alterations, lower BGM diversity, NAFLD and increased MAMPs across the studies underlines the role of the BGM in the onset and development of metabolic liver diseases.Citation11,Citation20,Citation100,Citation101 In particular, LPS has a critical role in triggering immune responses that lead to inflammation in different organs and to intestinal permeability, a phenomenon that usually happens during NASH and cirrhosis. Since LPS is one of the major components in the cell wall in gram-negative bacteria, the increased relative abundance of Enterobacteria, Bacteroides, or the invasion of oral gram-negatives could account for increased endotoxemia. However, recent research by d´Hennezel et al.,Citation102 shows how structural modifications of the LPS across members of the order Bacteroidales, the main LPS contributor along the gut, silences TLR4 signaling, facilitating host toleranceCitation102 and alters the proinflammatory paradigm of LPS.Citation103
3.2.2. Intestinal microbiota and MUC2
Although secreted MUC2 is expressed constitutively by goblet cells, its production is upregulated by TLR-signaling to replenish the mucin that is degraded by commensals or removed by peristalsis.Citation104 Bacteria such as Porphyromonas, Ruminococcus gnavus, Ruminococcus torques, Bacteroides or Escherichia coli, with higher relative abundances in NAFLD, as discussed before, can degrade MUC2 through different pathways,Citation105 enabling bacteria to colonize the epithelial surface. Therefore, TLR activation is vastly increased. This leads to an increase in the intracellular molecular pathways in IECs which end up in the activation of NF-κβ and the expression of inflammatory cytokines (TNF-α, IL-1β, IL-6, among others), chemokines, vasoactive factors (like nitric oxide) and reactive oxygen species (ROS).Citation106,Citation107 These different cascades signal for the recruitment of immune cells that perpetuate inflammation.Citation107,Citation108 Forming a vicious cycle of further gut barrier damage via TNF-α, which contributes to TJ dysfunction and malfunction in the synthesis of MUC2. Interestingly, some putative probiotic strains belonging to the Lactobacillus genus have been shown to bind to TLR4 and upregulate MUC2 expression in the HT-29 cell line model.Citation109 In fact, TNF-α, interleukin-4 (IL-4), and IL-13 can induce MUC2 transcription via NF-κβ.Citation110 This could indicate that a certain “basal” level of TLR excitation is required for the proper function of the gut barrier, and leaves open the question of how the gut epithelium is able to discriminate between maleficial and beneficial bacteria.
Intestinal microbiota can upregulate MUC2 transcription by providing the nutrients required for MUC2 syntheses, such as vitamins B, K, and D and amino acids.Citation105 Vitamin D is considered to have good predictive power for identifying NAFLD-cirrhosis, and its insufficiency has been associated with NAFLD.Citation111 Interestingly, a recent reviewCitation112 on long non-coding RNAs (lncRNAs) highlighted the importance of the expression levels of Vitamin D receptors (VDR). The authors speculated that increased levels of lncRNA H19, commonly occurring in various pathological conditions, could be related to the disruption of the epithelial barrier function by increasing the abundance of a microRNA (miR-675). miR-675 then targets VDR mRNA and decreases the levels of ZO-1 and E-cad mRNAs,Citation112,Citation113 contributing to intestinal permeability.
Direct contact of pathogens with the IECs triggers the immune response and affects the TJ complex in several ways. In the following paragraphs, we will describe relationships between the alterations in the TJ protein function and NAFLD.
3.2.3. Alterations in function of zonula occludens, zonulin and TJs-associated adhesion molecules
In the case of ZO-1, it has been reported that adult patients with NAFLD present reduced ZO-1 expression in duodenum compared to healthy patients.Citation11 Moreover, increased small intestine bacterial overgrowth co-occurred in NAFLD patients with intestinal permeability, suggesting a link between intestinal permeability, bacterial overgrowth and hepatic steatosis.Citation11 Mouries et al.,Citation19 reported that ZO-1 expression was reduced as early as after 48 h following feeding mice with a high-fat diet (60%) inducing leakage of the gut, before the emergence of steatosis. Moreover, they reported that the maintenance of the gut leakage occurred throughout the development of NASH and inflammation-driven insulin resistance and obesity.Citation19 In another study, Pacifico et al.,Citation114 found that in children with NAFLD, zonulin levels also correlated with the severity of steatosis,Citation114 and zonulin levels correlate positively with pathological markers of NAFLD (such as body mass index, triglycerides and serum Il-6, among others).Citation115 Assimakopoulos et al.,Citation116 showed how the duodenal expression of occludin and claudin-1 were decreased in patients with cirrhosis, and even further decreased in patients with decompensated cirrhosis. Furthermore, the expression of both occludin and claudin-1 in patients with compensated or decompensated cirrhosis were inversely correlated with endotoxin concentration.Citation116 Unfortunately, none of the previous studies performed a BGM profiling, which precludes to identify BGM alterations that could be compared to those found in other NAFLD studies (section 3.1). It is of note that most of the studies of TJs alterations are performed in the duodenal region, while the microbiota profiling of the gut is based on fecal samples in the majority of the cases, which might hinder specific microbial alterations happening in the duodenal region.
Rahman and colleaguesCitation117 reported reduced protein and transcript levels of JAM-A in the colon mucosa of NAFLD patients, which correlated with increased mucosal inflammation.Citation117 Analysis of the BGM content in JAM-A knockout mice (F11r−/−) fed with high fructose and high cholesterol diet, showed an increase in pro-inflammatory bacteria, mainly belonging to the Proteobacteria and Firmicutes phyla. Besides, they showed that depletion of microbiota protected F11r−/− mice from diet-induced NASH.Citation117 Since JAM-A controls the paracellular permeability and prevents LPS from crossing the epithelium, deficiencies in this protein made mice more susceptible to NASH in the presence of high proinflammatory pathobionts.
3.2.4. Alterations in E-cadherin function
As previously discussed, E-cadherin is a crucial AJ for intercellular associations, and its loss of function has been linked to several pathological processes. In fact, deletion of E-cadherin in mice resulted in severe weight reduction, loss of intestinal epithelial architecture and problems in the differentiation of secretory cell lineages.Citation118 In particular, Paneth cell maturation and placement along the intestine is affected by the loss of E-cadherin. The reduction in Paneth cell count impairs proper bacterial defense, facilitating the approach of pathobionts to the IECs. Interestingly, the enterotoxin fragilysin, produced by Bacteroides fragilis, can interact and cleave the extracellular domains of E-cadherin,Citation119 resulting in intestinal permeability. Bacteroides is a common resident in the gut and conflictive results are reported when assessing its contribution to liver disease (Supplementary Table 1). This bacterium, typically considered a commensal, has also been found to be responsible for a significant increase in the biofilm mass in patients with Crohn’s disease.Citation120 However, the apparent pathological relation with Crohn’s disease is as well challenged by Hsiao et al.Citation121 Treatment of the model maternal immune activation (MIA) mice with B. fragilis rendered an improvement in gut barrier integrity and promoted the restoration of the relative abundance of 6 operational taxonomic units in the absence of B. fragilis colonization. Remarkably, treatment with B. fragilis was able to correct autism spectrum disorder-related behavioral abnormalities in this mice model.Citation121 This highlights how members of the same genus or family contribute differently to the homeostasis of the intestine, blurring their role in health and in disease. How members of the same species account for different effects, possibly depending on the environmental cues (such as diet, or genetic background); as well as, how strain-level identification of the BGM is needed to account for strain-specific traits, are important outstanding questions.
3.2.5. Gut-vascular barrier disruption
Recently, Spadoni et al.Citation30 reported an increase of the GVB disruption in the small and large intestine of mice, after just one week fed with a high-fat diet, and in the colon of NASH patients. The disruption of the GVB, marked by PV1 upregulation, was parallel to increased permeability detected in murine IECs after just 48 hours of high-fat feeding. This suggests that GVB represents yet another barrier of defense that prevents bacteria or specific molecules from progressing to the systemic circulation. In fact, they proposed that disruption of the GVB (indicated by upregulated PV1) is a prerequisite for NASH pathogenesis and is a consequence of diet-induced microbiota changes.Citation19 Pathogens, such as Salmonella Typhimurium, interfere with the WNT/β-catenin pathway allowing them to bypass the GVB, suggesting that the WNT/β-catenin could be an important player in GVB barrier disruption.Citation19,Citation30 More studies, focusing on the mechanism by which the GVB disruption happens and the role of the BGM are needed and represent an interesting approach for studying NAFLD pathogenesis.
4. The link between gut microbiota metabolites and gut barrier function in NAFLD
4.1. Ethanol
Due to the histological similarities between obesity-related fatty liver disease and alcohol-induced liver damage,Citation122 common pathogenic mechanisms have been suggested to be involved in both conditions. Consequently, it has been hypothesized that NAFLD patients may be exposed to endogenous ethanol, as has been shown in human patientsCitation123,Citation124 and miceCitation124 (Supplementary Table 2, ). For instance, pediatric NASH patients present higher serum levels of ethanol than obese and healthy children.Citation123
Figure 2. Alterations in gastrointestinal (GI) tract barriers associated with nonalcoholic fatty liver disease (NAFLD) pathogenesis. An altered GI tract microbiota due to diet, lifestyle, age and genetic predisposition can lead to an altered pool of metabolites along the GI tract. An altered microbiota and metabolite composition can lead to GI tract barriers disruption, with a special focus in this review to the microbiological, chemical and physical barriers. The increase in gut permeability leads to an incremented translocation of bacteria, bacterial components and bacterial metabolites to the portal system; which ultimately cause hepatic inflammation and damage and, hence, contributing to NAFLD pathogenesis. SCFA: Short-chain fatty acid, BA: Bile acid, DCA: Deoxycholic acid, CDCA: Chenodeoxycholic acid; AMP: Antimicrobial peptides
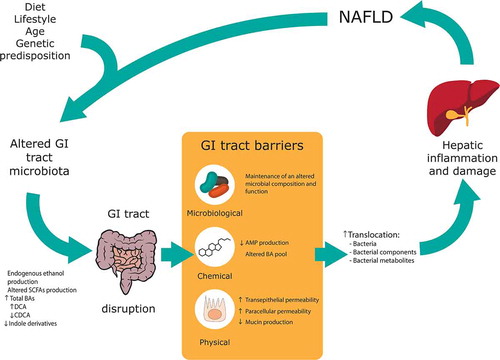
GI tract microbiota has been suggested as the source of this endogenous ethanol, being able to produce it as a result of the fermentation of simple carbohydrates, including sucrose, glucose, and fructose.Citation125–128 An excess of those carbohydrates in the diet and/or a decrease in gut motility (associated with obesity, diabetes, or chronic alcohol abuse) have been shown to enhance this pathway.Citation125 Intestinal stasis has been suggested to permit the overgrowth of colonic bacterial strains in the upper gastrointestinal tract, thus promoting the fermentation of those simple carbohydrates and yielding ethanol as a result.Citation129 Oral administration of the antibiotic neomycin in obese mice reduced breath ethanol excretion, suggesting the role of GI tract microbiota in the production of ethanol.Citation129 Moreover, Zhu et al.Citation123 associated the increase in blood ethanol levels with an increase in abundance of Proteobacteria/Enterobacteriaceae/Escherichia sp. Under anaerobic conditions and in the absence of alternative electron acceptors, Escherichia coli catalyzes the mixed-acid fermentation pathway converting sugars into several products, including ethanol.Citation130 In an interesting recent study, a high alcohol producing Klebsiella pneumoniae strain, but not strains unable to produce ethanol, was shown to induce NAFLD in mice. Its colonization was suggested to affect gut barrier function via decreasing expression of occludin and ZO-1.Citation131 The same study also reported an association between disease severity and high alcohol producing Klebsiella pneumoniae isolates in patients with NAFLD.Citation131 The results of this study point out the importance of studying the role of specific bacterial strains in the development of the disease, showing that the analysis to higher taxons such as phylum, order or genus are insufficient. On the other hand, other intestinal bacterial genera from different phyla have been shown to produce ethanol such as BacteroidesCitation137 and Bifidobacterium.Citation138 Nevertheless, the majority of researchers believe that fungi are the main contributors to the endogenous production of ethanol.Citation8
Ethanol can be metabolized by both the host and the GI tract microbiota. On the one hand, ethanol is typically metabolized in the host through the so-called oxidative conversion. In this pathway, acetaldehyde is produced by the action of the enzyme alcohol dehydrogenase (ADH). Subsequently, the enzyme acetaldehyde dehydrogenase (ALDH) converts acetaldehyde into acetate.Citation8 This process takes place mainly in the hepatocytes, although these enzymes are also present in other tissues including the intestinal mucosa.Citation8 In addition, in the large intestine, the mucosal ALDH activity is lower than ADH activity and, hence, an accumulation of the toxic and reactive metabolite acetaldehyde is expected.Citation132 GI-tract microbiota has also been suggested to metabolize ethanol in an ADH-dependent manner, as it was shown for the Enterobacteriaceae family.Citation133 Other ubiquitous distributed host enzymes involved in ethanol metabolism to acetaldehyde are CYP2E1 and catalase.Citation134 In the intestine, nonoxidative alcohol metabolism via reactions with free fatty acids and membrane phospholipids has been described to occur. The former reaction generates fatty acid ethyl esters (FAEE) and the latter generates abnormal phospholipids affecting cell signaling of intestinal epithelial cells.Citation132
The disruption of gut barrier function by ethanol has been mainly studied in the context of alcoholic fatty liver disease (AFLD) and it can do it through several pathways. First of all, ethanol consumption has been shown to alter GI tract microbiota compositionCitation135,Citation136 and function,Citation137 affecting the microbiological barrier. Regarding the functional alteration of the intestinal microbiota, a decrease in the SCFAs propionate and isobutyrate production has been described, as these metabolites are important for gut barrier integrity.Citation137 Furthermore, ethanol has been associated with the loss of gut-associated lymphoid tissues (GALT) cells in mice, compromising the clearance of potentially pathogenic bacteria such as Salmonella typhimurium.Citation138 Concerning the chemical barrier, the production of antimicrobial molecules by host cells has also been shown to be compromised by alcohol consumption. For instance, alcohol feeding has been associated with mice models with a downregulation of the antimicrobial C-type lectin RegIIIβ in the small intestine.Citation139
However, the most relevant mechanism by which ethanol disrupts gut barrier function is associated with the physical barrier. It has been shown to disrupt the IECs themselves (transepithelial permeability) and the spaces between them (paracellular permeability).Citation8 Transepithelial permeability is mainly caused by: (1) cell death causing loss of epithelium mainly at the duodenum villi tips;Citation140 and (2) reactive oxygen/nitrogen species (ROS/RNS) production resulting from ethanol metabolism, which generates nitroxidative stress and cellular damage.Citation141 Paracellular permeability caused by alcohol involves its action in TJs. Cho et alCitation141 showed a decrease in binge alcohol-exposed rat in the levels of TJs (ZO-1, claudin-1, −4, and occludin), AJs (β-catenin and E-cadherin) and desmosome plakoglobin. The same study found similar results for human subjects who suddenly died from heavy alcohol intoxication. In Caco-2 cells, an in vitro model of intestinal epithelial cell monolayers, alcohol is able to induce a change in expression of ZO-1, claudin-1 and JAM-A, thus increasing intestinal permeability.Citation142,Citation143 Additionally, ethanol can affect gut cell structures via cytoskeleton modification.Citation144 Moreover, alcohol-related metabolites, such as acetaldehyde and FAEEs, have also been shown to cause TJs disruption. Acetaldehyde, the most toxic ethanol-associated metabolite, induces translocation of protein phosphatase 2A (PP2A) to TJs and dephosphorylates occludin threonine residues, preventing its interaction with ZOs, thus disassembling TJs.Citation145–147 This metabolite also inhibits tyrosine phosphatase activity, increasing tyrosine phosphorylation of ZO-1Citation148 and AJs (β-catenin and E-cadherin),Citation149 compromising cell-cell adhesion. FAAEs, such as ethyl oleate and ethyl palmitate, are able to increase permeability dose-dependently through disruption and decrease of ZO-1.Citation150
Despite the histological similarities between both conditions, obesity-related fatty liver disease and alcohol-induced liver damage, the conditions and concentrations under which ethanol exerts its effect in the GI-tract could be potentially different. As a consequence, more studies about endogenous ethanol production under NAFLD conditions are needed in order to uncover its real influence in this pathology and in particular its potential contribution in GI tract barrier dysfunction.
4.2. Short-chain fatty acids (SCFAs)
SCFAs, which include organic acids with 1 to 6 carbons, are the main end products of colonic bacterial metabolism.Citation151 The enzymatic capability of humans is not able to degrade dietary fiber, leaving the complex carbohydrate present in there as substrates for anaerobic bacterial fermentation in the colon (Supplementary Table 2).Citation3,Citation152 This fermentation process involves a variety of enzymatic reactions and metabolic processes, supporting colonic microbial growth and maintenance and yielding metabolic end products, such as SCFAs, that can be used by the host.Citation3 SCFAs produced include acetate, propionate and butyrate. Acetate is the most abundant SCFA, followed by propionate and butyrate.Citation3
Some commensal bacteria have been identified to have an anti-inflammatory effect through the production of SCFAs. Among them, butyrate has been shown to play a key role in maintaining and enhancing gut barrier homeostasis,Citation153 being the entity most extensively studied. In general, this SCFA at a low concentration (2 mM) decreases the permeability to mannitol/inulin and increases transepithelial electrical resistance (TER) in Caco-2 cell monolayer.Citation154,Citation155 However, its effect is considered as paradoxical, since at high concentrations (8 mM) it has the opposite effect, increasing permeability to inulin and reducing TER significantly.Citation154 The ability to produce butyrate has been mainly identified in bacteria classified into different families of the Firmicutes phylum, although some other producers are members from nine other phyla, including Actinobacteria, Bacteroidetes, Proteobacteria or Fusobacteria.Citation156
Mechanistically, butyrate has been shown to promote gut barrier integrity affecting different GI tract barriers. At epithelium level, it directly interacts with IECs since it is preferentially used as intestinal fuel by colonocytes, preferred over other SCFAs, glucose or glutamine.Citation3,Citation157 Metabolism of butyrate, and to a lesser extent acetate´s and propionate´s metabolism, depletes O2 thus resulting in the stabilization of hypoxia-inducible factor (HIF).Citation158 HIF is a transcription factor that targets genes associated with antimicrobial defense,Citation159 mucin production,Citation160 and the ITF implicated in the repair of the intestinal mucosa.Citation161 In addition, butyrate can affect IECs enhancing their base crypt proliferation, an event associated with the prevention of the development of colon cancer.Citation162 However, not all the studies suggest a protective role of butyrate in colon cancer and this lack of agreement has led to the definition of the “butyrate paradox”, suggesting that this discrepancy may be explained by differences in experimental design (excellently reviewed in Lupton, 2004).Citation163 In addition, butyrate promotes goblet cell differentiation by increasing the expression levels of Krüppel-like transcriptional factor 4 (KLF4).Citation164 This SCFA also stimulates the production of mucin via increasing MUC2 expression of goblet cells in in vitro models.Citation165,Citation166 Paracellular permeability has also been shown to be decreased by butyrate, accelerating TJs assembly through activation of AMP-activated protein kinase (AMPK) in Caco-2 cell monolayers.Citation167 The same study reported that 2 mmol/L butyrate did not significantly alter the expression of four different TJs (claudin-1, claudin-4, ZO-1 and occludin). Nonetheless, other studies have found an increase in the expression of occludin, ZO-1 and claudin-2.Citation168,Citation169 With regard to the chemical barrier, butyrate also mediates AMPs production, binding specifically to the G-protein-coupled receptor 109A (GPR109A) in the colonic epithelium. As a consequence, IL-18 is produced,Citation170 a cytokine which has been shown to be involved in mucin production and AMPs production, such as intelectin-1 (ITLN1), RELMβ and members of the angiogenin family.Citation3,Citation171 Nonetheless, IL-18 can control intestinal homeostasis or induce a pro-inflammatory response in a concentration-dependent manner.Citation172 Butyrate also binds to GPR43 in IECs and promotes the production of RegIIIγ and β-defensins.Citation173 Thus, butyrate promotes the expression of other AMPs such as human cathelicidin LL-37/human cationic antimicrobial protein 18 (hCAP18) in IECsCitation174,Citation175 or calprotectin in intestinal macrophages.Citation45 In general, fermentation of SCFAs results in a decreased pH, which influences the microbiological barrier indirectly reducing, for instance, the abundance of potentially pathogenic clostridia.Citation3
A clinical study carried out by Michail et al.Citation176 demonstrated that levels of acetate are decreased in fecal samples of obese children with NAFLD in comparison to healthy children and those obese without evidence of NAFLD. The same study did not report significant differences in butyrate and propionate levels. In contrast, Raman et alCitation177 detected an increase in all three types of SCFAs in fecal samples of obese humans with NAFLD. These latter findings are supported by another clinical study, where butyrate and propionate were enriched in fecal samples of patients with mild NAFLD, whereas acetate was enriched in adults with advanced fibrosis.Citation178
Despite the association of SCFAs with the maintenance and enhancement of gut barrier function, these compounds have been shown to affect other tissues, such as adipose and hepatic tissues, and processes, including lipogenesis and gluconeogenesis (reviewed in Chu et al.Citation26). As a consequence, depending on the mechanisms or signaling pathway that SCFAs activate, they appear to both prevent but also promote the development of NAFLD.
4.3. Bile acids
Bile acids (BAs) are soluble products derived from the catabolism of cholesterol. In mammals, there are two major classes of BAs: primary and secondary BAs.Citation179 Primary BAs, chenodeoxycholic acid (CDCA) and cholic acid (CA) are synthesized in the liver and then conjugated with glycine or, to a lesser extent, taurine.Citation42,Citation179 Upon conjugation and after meal consumption, BAs are released into the duodenum.Citation42 Later in the ileum, BAs are reabsorbed and further re-circulated to the liver in the so-called enterohepatic circulation.Citation179 A small portion of BAs (~5%) escape this resorption and are deconjugated by the microbiota before either being absorbed or converted into secondary BAs.Citation180 The bacterial enzyme catabolizing this deconjugation is the bile salt hydrolase (BSH) and it is present in all major bacterial and archaeal divisions in the human GI tract, including members of the Firmicutes, Bacteroidetes and Actinobacteria phyla.Citation181 In the large intestine, GI tract microbiota further metabolizes deconjugated BAs through 7α-dehydroxylation, converting them into secondary BAs, which can be excreted or passively reabsorbed.Citation42,Citation179 As a consequence, from CDCA lithocholic acid (LCA) is formed and from CA deoxycholic acid (DCA). Clostridium from clusters XIVa and XI and Eubacterium species have been described to be able to produce secondary bile acids.Citation42 In addition, in silico analyses, have shown that bacteria from clades Ruminococcaceae, Lachnospiraceae and Peptreptococcaceae exhibit bile acid-inducible 7α-dehydroxylation genes.Citation182 Some other isomerizations and oxidations can take place further diversifying the pool of secondary BAs.Citation42 Consequently, the presence or absence of bacterial species able to catalyze these transformations will be critical in determining the pool of BAs present in the host.
The BA pool composition is relevant since the hydrophobicity of BA species (UDCA<CA<CDCA<DCA<LCA) is an important determinant of their cytotoxic effect.Citation183 The greater the hydrophobicity, the greater the toxicity, probably via an indirect mechanism that involves oxidative stress induction and the production of ROS species.Citation183 Additionally, BAs can modulate different receptors, each of which shows different affinities and effects depending on the BA species.Citation180 As a consequence, the composition of the pool of BAs is critical in the activation of different signal pathways and, hence, the GI tract microbiota is a critical factor in host physiology. However, not only the GI tract microbiota affects the pool of BAs, but also the latter can shape the bacterial community by inhibiting the growth of sensitive bacteria and promoting those able to metabolize BAs. BAs are considered part of the chemical GI tract barrier, having direct antimicrobial activity due to their detergent properties.Citation42,Citation184 Gram-positive bacteria are generally more sensitive to the antimicrobial effect of BAs than Gram-negative bacteria, although tolerance to BAs has been shown to be a strain-specific feature.Citation185 Nevertheless, BAs also exert indirect effects modulating the activation of the farnesoid X receptor (FXR), which has been shown to induce the transcription of inducible NO synthase (iNOS), IL-18 and angiogenin (ANG1); all of them are involved in antibacterial defense.Citation186
The occurrence of NAFLD has been associated in clinical studies with dysregulation of BA homeostasis (Supplementary Table 2, ). Some studies have described an increase of total BAs in serum levels in NASH patients.Citation187,Citation188 In addition, an increase in conjugated BAs in serum has been associated with NASH.Citation188–191 However, Puri et al.Citation189 reported an increase of serum levels of total primary BAs and a decrease of total secondary BAs in NASH patients, while Jiao et al.Citation187 described the opposite trend. Regarding specific BA species, Jiao et al. described a decrease of CDCA and an increase of ~4 fold of DCA in the serum levels of NASH patients. A similar trend has also been reported by Puri et al,Citation189 although the differences were not statistically significant. This increase in DCA versus CDCA could be relevant for gut barrier function since DCA counteracts and inhibits the agonistic activity of CDCA in FXR,Citation192 the receptor shown to be involved in host antibacterial defense. In addition, DCA has been shown to directly affect IECs in mice models increasing cell proliferation, colonic nuclear damage and colonic tumor incidence.Citation193 However, DCA has also been shown to induce mucin production in colon cancer cells, increasing MUC2 transcription via modulation of NF-κβ and JNK,Citation194 suggesting it as a common mechanism for cytoprotection in vivo. These results manifest the complex interaction of BAs with the host in general and with gut barrier integrity in particular.
In a recent study, worsening fibrosis severity in non-obese NAFLD patients has been correlated with an increase in the synthesis of bile acids. The same study reported a depletion of Ruminococcaceae in non-obese subjects with significant fibrosis and, hence, elevated BA synthesis.Citation195 Ruminococcaceae has been described as a contributor to the bsh gene, implicated in the metabolism of BAs; which was shown to be downregulated in the same subjects.Citation195 The elevated synthesis of BAs and the previously mentioned higher sensitivity of Gram-positive bacteria to them could explain the decrease in the Ruminococcaceae clade. In contrast, Adams et al.Citation196 associated a higher abundance of DCA with the abundance of the Lachnospiraceae family and advanced liver fibrosis. The increase in abundance of the Lachnospiraceae family could explain the increase in DCA levels since this clade has been shown to have bile acid-inducible 7α-dehydroxylation genes.Citation182
With regard to GI tract microbiome signatures in the disease state, Jiao et al. described that two BA-related pathways were elevated in NASH patients: “glycine, serine and threonine metabolism” and “taurine and hypotaurine metabolism”.Citation187 In addition, they described a ~7.2 fold increase in the abundance of bacteria able to metabolize taurine, mainly explained by an increase in the genera Escherichia and Bilophila. It is important to note that taurine has recently been reported to improve GI tract barrier function via enhancing the expression of ZO-1 and occludin in Caco-2 cells.Citation97 Moreover, Ahmadi et al.Citation97 showed how the treatment with a human-origin probiotic cocktail, composed by 5 Lactobacillus and 5 Enterococcus strains, is able to ameliorate leaky gut in older mice via modulating the microbiota-taurine-TJs axis. The probiotic cocktail was shown to enhance BSH activity, releasing a higher quantity of taurine, reducing GI tract leakiness and demonstrating the importance of microbial activity in host physiology.
Nevertheless, it is important to note that the pool of BAs and their effect on the host are different between mice and humans. Mice, besides CDCA and CA, also produce muricholic acids (MCAs) as primary BAs. Tauro-βMCA, not present in humans, is considered an antagonist of FXR and, consequently, deconjugation thus promotes FXR signaling in mice.Citation197 This difference in BA signaling between mice and human models points out the importance of being cautious when extrapolating results from mice to human models.
4.4. Tryptophan
Evidence in mice models and in clinical studies indicate that tryptophan (Trp) metabolism is involved in NAFLD progression (Supplementary Table 2, ).Citation198,Citation199 Trp is an important amino acid present in food and once it is delivered into the GI tract, it is mainly involved in three pathways: 1) the direct transformation of Trp into several different derivatives by the gut microbiota (represents the 4–6% of ingested Trp); 2) the kynurenine (Kyn) pathway (KP) (95% of ingested Trp) via indoleamine 2,3-dioxygenase (IDO1); and 3) the serotonin (5 hydroxytryptamine [5HT]) production pathway (1–2% of ingested Trp) via Trp hydroxylase 1 (TpH1).Citation7
Commensal bacteria are able to metabolize tryptophan into several different derivatives, such as indole pyruvic acid and indole acetaldehyde. The indole pyruvic acid can be transformed into indole propionic acid (IPA); meanwhile, the indole acetaldehyde can be transformed to indole acetic acid (IAA) and then in indole aldehyde (IAld).Citation7 In healthy intestine indoles have been shown to be aryl hydrocarbon receptor (AHR) ligands promoting IL-22 production which controls epithelial cell proliferation, AMPs productionCitation200-202 and are involved in the expression of mucin genes.Citation203 Moreover, indole attenuates inflammation, reducing the levels of TNF-α and IL-8, and increasing the levels of the expression of the anti-inflammatory IL-10 in the human enterocyte cell line. Venkatesh et al.Citation204 have shown in mouse models that IPA promotes intestinal barrier integrity upregulating the expression of ZO-1, occludin and E-cadherin through the activation of pregnane X receptor (PXR).Citation204 Therefore, indoles in NAFLD could be a player for promoting gut barrier function and it deserves more in-depth studies.
Most of the tryptophan ingested (95%) is transformed into Kyn by IDO1 in the gut. Laurans et al.Citation205 have shown increased IDO1 activity in high-fat diet mouse models and decreased indole derivatives and deletion of IDO1 increases intestinal availability of indoles. They also confirmed a shift in Trp metabolism toward more Kyn production and less indole derivatives in feces of subjects with metabolic syndromes.Citation205 Overexpression of the Kyn pathway has also been shown in NAFLD subjects.Citation206 Changes in the balance of Trp metabolism, a decrease of indoles production and an increase of Kyn might be involved in GI barrier functions in NAFLD pathogenesis but no evidence has been shown yet.
While a small amount of ingested Trp is involved in 5HT production, more than 90% of the body’s 5HT available is produced in the GI tract by the enzyme TpH1 in enterochromaffin cells.Citation207 A consistent amount of 5HT production is regulated by the gut microbiota, especially by spore-forming bacteria that stimulate ECs to produce it in the mouse colon but not in the small intestine.Citation208 Moreover, the probiotic Escherichia coli Nissle 1917 has been shown to affect the 5HT production in ex vivo mouse ileum experiments, increasing the 5HT availability.Citation209 Recently, Fung et al.Citation210 have shown that the crosstalk between 5HT and microbiota is bidirectional. In fact, in vitro experiments, the authors have observed that Turicibacter is able to sense, uptake and respond to 5-HT regulating its colonization in the GI tract.Citation210
5HT can exert its functions through a high number of receptors widely expressed in the intestine.Citation207 Interestingly, evidence of a correlation between 5HT and NASH has been recently shown in serum levels of NAFLD patients and in serum levels and duodenum of rat fed with high fat-sucrose diet inducing NASH.Citation211 The inhibition of 5HT in those rats and in in vitro models of NAFLD alleviates hepatic lipid accumulation and inflammation.Citation211 The detrimental role of 5HT in NAFLD has also been shown in Tph1 knockout mice.Citation212,Citation213 where 5HT is able to upregulate lipogenic genes in the liver through hepatic serotonin receptor HTR2A.Citation213 Moreover, the inhibition of the 5-HT3 receptor in mice fed fructose has shown to alleviate hepatic steatosis.Citation214 Whether 5HT is linked with gut barrier permeability observed in NAFLD is still unclear.
Some studies have linked 5HT with the regulation of intestinal permeability. 5HT has been shown to promote the translocation of LPS in the ileum of rats via the 5HT3 receptor.Citation215 In accordance, 5HT reinforced the permeability induced by NaCl perfusion in the duodenum of rats. Moreover, the use of 5HT3 receptor antagonists (granisetron and ondansetron) and 5HT4 receptor antagonist (SB 203186) restored the duodenal mucosal barrier integrity.Citation216 Huab et al.Citation214 have observed with an in vitro model, that 5HT treatment decreased significantly the TJ occludin expression in a dose-dependent way.Citation214 The same group has also shown that leptin-deficient obese mice treated with antagonists (ropisetron and palonosetron) of the 5HT3 receptor have increased expression of occludin, and claudin-1 in the duodenum.Citation217
The mechanisms governing the 5-HT effects in the gut barrier through its receptors are not fully understood yet, as well as its crosstalk with the gut microbiota. 5HT represents a potentially interesting candidate of gut barrier dysfunction observed in NAFLD and deserves more investigation.
5. Conclusions
Based on the currently existing knowledge, we have reviewed 1) how in NAFLD the gut barrier functions are impaired, 2) how bacterial communities differ in NAFLD and NAFLD-related fibrosis and cirrhosis, 3) how specific metabolites vary in NAFLD, and 4) how microbiota and these related metabolites might have an impact in gut barrier damage.
The GI tract contains several barriers playing essential roles in maintaining the GI tract homeostasis. In human clinical studies, GI tract barrier dysfunctions observed in NAFLD are usually assessed by analyzing TJs expression in biopsies from the small intestine. An invasive and challenging method, which, on the other hand, does not take into account the characteristics of the different intestinal barriers and the crosstalk between them along the GI tract. It is, therefore, expected that the gut barrier functions and permeability characteristics may differ in the different segments of the GI tract. It is relevant to point out that gut barrier dysfunction studies are mainly focused on the alteration of the microbiological and physical barriers leaving out the potentially important role of the chemical and immunological barriers and the recently described GVB.
Several metabolites have been shown to modulate gut barrier integrity. Some of the described metabolites, including ethanol, acetaldehyde and some BAs such as DCA, have been associated with impairment of gut permeability. While others, such as the SCFA butyrate and indoles derived from tryptophan metabolism, have been shown to promote gut barrier integrity. However, there is a lack of consensus about the role of some of these metabolites regarding gut permeability modulation, including the mentioned “butyrate paradox”. These contradictory results could be a consequence of differences in study designs, but also of the complexity of the system in which these metabolites exert their action.
Different bacterial taxa have been identified to influence the presence and abundance of the metabolites. Alterations in the GI tract microbiota, as have been shown in NAFLD, will be translated into an altered pool of metabolites and, hence, affect the gut barrier function (). Even more, these alterations in the GI tract microbiota will also shape interactions between different BGM taxa. For instance, increased endogenous ethanol production, primary BA deconjugation or AMP secretion could subject neighboring bacteria to different ecological pressures for which particular taxa might be maladapted and then outcompeted. Indeed, the antimicrobial potential of these bacteria cannot be neglected: they play a major role in shaping the communities and keeping a good balance for the optimal metabolic activity. This topic would need increased attention in future studies on the positive effects some species have to avoid NAFLD disease. However, the most common sampling method for microbiota analysis, fecal sampling, is not able to reflect the community structure of all the different intestinal segments. Therefore, potential relevant processes and ecological transitions are lost. Current technology often fails to detect bacterial taxa at the strain level. Instead, abundance is generally referred to as genus, family or even phylum level, overlooking the impact an individual strain could have. Another essential aspect to consider is that the gut microbiota is composed not only by bacteria but also by fungi, archaea, protists and viruses. However, most of the studies linking gut microbiota alterations with NAFLD pathogenesis refer solely to bacteria. More research focused on unraveling the role of under-represented micro-organisms in the microbiota is of crucial importance.
How the bacterial gut microbiota influences, partly via produced metabolites, the disruption of the gut barrier; what the influence in this process is of other gut microbes; and how the crosstalk between the host and its microbiota intervenes in NAFLD pathogenesis are still unresolved questions. Consequently, more studies reflecting the complexity of the crosstalk between GI tract microbiota, host and metabolites are required to disentangle their causal effect in modulating gut permeability in NAFLD pathogenesis.
Supplemental Material
Download MS Word (33.9 KB)Supplemental Material
Download MS Word (62.9 KB)Acknowledgments
MVFC, DGM, VI, HEN, MK and OPK have received funding from the European Union’s Horizon 2020 research and innovation programme under the Marie Skłodowska-Curie grant agreement No 813781.
Supplementary material
Supplemental data for this article can be accessed on the publisher’s website.
Additional information
Funding
References
- Farré R, Fiorani M, Rahiman SA, Matteoli G. Intestinal permeability, inflammation and the role of nutrients. Nutrients. 2020;12(4):1. doi:https://doi.org/10.3390/nu12041185.
- Sittipo P, Shim J-W, Lee YK. Microbial metabolites determine host health and the status of some diseases. Int J Mol Sci. 2019;20(21):5296. doi:https://doi.org/10.3390/ijms20215296.
- Wong JMW, de Souza R, Kendall CWC, Emam A, Jenkins DJA. Colonic Health: fermentation and Short Chain Fatty Acids. J Clin Gastroenterol. 2006;40(3):235–26. doi:10.1097/00004836–200603000–00015.
- Ridlon JM, Kang D-J, Hylemon PB. Bile salt biotransformations by human intestinal bacteria. J Lipid Res. 2006;47(2):241–259. doi:https://doi.org/10.1194/jlr.R500013-JLR200.
- Rowland I, Gibson G, Heinken A, Scott K, Swann J, Thiele I, Tuohy K. Gut microbiota functions: metabolism of nutrients and other food components. Eur J Nutr. 2018;57(1):1–24. doi:https://doi.org/10.1007/s00394-017-1445-8.
- Nielsen DSG, Jensen BB, Theil PK, Nielsen TS, Knudsen KEB, Purup S. Effect of butyrate and fermentation products on epithelial integrity in a mucus-secreting human colon cell line. J Funct Foods. 2018;40:9–17. doi:https://doi.org/10.1016/j.jff.2017.10.023.
- Taleb S. Tryptophan Dietary Impacts Gut Barrier and Metabolic Diseases. Front Immunol. 2019;10:2113. doi:https://doi.org/10.3389/fimmu.2019.02113.
- Bishehsari F, Magno E, Swanson G, Desai V, Voigt RM, Forsyth CB, KeshavarzianA.Alcohol and Gut-Derived Inflammation. Alcohol Res Curr Rev. 2017;38(2):163–171 [Accessed September 10, 2020]. https://www.ncbi.nlm.nih.gov/pmc/articles/PMC5513683/.
- Chakaroun RM, Massier L, Kovacs P. Gut Microbiome, Intestinal Permeability, and Tissue Bacteria in Metabolic Disease: perpetrators or Bystanders? Nutrients. 2020;12(4):1082. doi:https://doi.org/10.3390/nu12041082.
- Boursier J, Diehl AM. Nonalcoholic fatty liver disease and the gut microbiome. Clin Liver Dis. 2016;20(2):263–275. doi:https://doi.org/10.1016/j.cld.2015.10.012.
- Miele L, Valenza V, La Torre G, Montalto M, Cammarota G, Ricci R, Mascianà R, Forgione A, Gabrieli ML, Perotti G, et al. Increased intestinal permeability and tight junction alterations in nonalcoholic fatty liver disease. Hepatology. 2009;49(6):1877–1887. doi:https://doi.org/10.1002/hep.22848.
- Saadeh S, Younossi ZM. The spectrum of nonalcoholic fatty liver disease: from steatosis to nonalcoholic steatohepatitis. Cleve Clin J Med. 2000;67(2):96–97. doi:https://doi.org/10.3949/ccjm.67.2.96. 101
- Ko JS. New perspectives in pediatric nonalcoholic fatty liver disease: epidemiology, genetics, diagnosis, and natural history. Pediatr Gastroenterol Hepatol Nutr. 2019;22(6):501–510. doi:https://doi.org/10.5223/pghn.2019.22.6.501.
- Sharpton SR, Ajmera V, Loomba R. Emerging Role of the Gut Microbiome in Nonalcoholic Fatty Liver Disease: from Composition to Function. Clin Gastroenterol Hepatol. 2019;17(2):296–306. doi:https://doi.org/10.1016/j.cgh.2018.08.065.
- Sattar N, Forrest E, Preiss D. Non-alcoholic fatty liver disease. BMJ. 2014;349(sep19 15):g4596. doi:https://doi.org/10.1136/bmj.g4596.
- Stols-Gonçalves D, Tristão LS, Henneman P, Nieuwdorp M. Epigenetic Markers and Microbiota/Metabolite-Induced Epigenetic Modifications in the Pathogenesis of Obesity, Metabolic Syndrome, Type 2 Diabetes, and Non-alcoholic Fatty Liver Disease. Curr Diab Rep. 2019;19(6):31. doi:https://doi.org/10.1007/s11892-019-1151-4.
- Buzzetti E, Pinzani M, Tsochatzis EA. The multiple-hit pathogenesis of non-alcoholic fatty liver disease (NAFLD). Metabolism. 2016;65(8):1038–1048. doi:https://doi.org/10.1016/j.metabol.2015.12.012.
- Schnabl B, Brenner DA. Interactions between the intestinal microbiome and liver diseases. Gastroenterology. 2014;146(6):1513–1524. doi:https://doi.org/10.1053/j.gastro.2014.01.020.
- Mouries J, Brescia P, Silvestri A, Spadoni I, Sorribas M, Wiest R, Mileti E, Galbiati M, Invernizzi P, Adorini L, et al. Microbiota-driven gut vascular barrier disruption is a prerequisite for non-alcoholic steatohepatitis development. J Hepatol. 2019;71(6):1216–1228. doi:https://doi.org/10.1016/j.jhep.2019.08.005.
- Giorgio V, Miele L, Principessa L, Ferretti F, Villa MP, Negro V, Grieco A, Alisi A, Nobili V. Intestinal permeability is increased in children with non-alcoholic fatty liver disease, and correlates with liver disease severity. Dig Liver Dis. 2014;46(6):556–560. doi:https://doi.org/10.1016/j.dld.2014.02.010.
- Reed KK, Wickham R. Review of the Gastrointestinal Tract: from Macro to Micro. Semin Oncol Nurs. 2009;25(1):3–143. doi:https://doi.org/10.1016/j.soncn.2008.10.002.
- Elizabeth M. Fish, Bracken Burns. Physiology, Small Bowel. StatPearls. StatPearls Publishing: Treasure Island (FL). 2020. Available online: https://pubmed.ncbi.nlm.nih.gov/30335296/
- Azzouz LL, Sharma S. Physiology, Large Intestine. StatPearls. StatPearls Publishing: Treasure Island (FL). 2020.Available online: https://www.ncbi.nlm.nih.gov/books/NBK507857/.
- Thursby E, Juge N. Introduction to the human gut microbiota. Biochem J. 2017;474(11):1823–1836. doi:https://doi.org/10.1042/BCJ20160510.
- Mukherjee S, Hooper LV. Antimicrobial Defense of the Intestine. Immunity. 2015;42(1):28–39. doi:https://doi.org/10.1016/j.immuni.2014.12.028.
- Chu H, Duan Y, Yang L, Schnabl B. Small metabolites, possible big changes: a microbiota-centered view of non-alcoholic fatty liver disease. Gut. 2019;68(2):359–370. doi:https://doi.org/10.1136/gutjnl-2018-316307.
- Shulzhenko N, Morgun A, Hsiao W, Battle M, Yao M, Gavrilova O, Orandle M, Mayer L, Macpherson AJ, McCoy KD, et al. Crosstalk between B lymphocytes, microbiota and the intestinal epithelium governs immunity versus metabolism in the gut. Nat Med. 2011;17(12):1585–1593. doi:https://doi.org/10.1038/nm.2505.
- Johansson MEV, Sjövall H, Hansson GC. The gastrointestinal mucus system in health and disease. Nat Rev Gastroenterol Hepatol. 2013;10(6):352–361. doi:https://doi.org/10.1038/nrgastro.2013.35.
- Groschwitz KR. Intestinal barrier function: molecular regulation and disease pathogenesis. J Allergy Clin Immunol. 2009;124:3–20. doi:https://doi.org/10.1016/j.jaci.2009.05.038.
- Spadoni I, Zagato E, Bertocchi A, Paolinelli R, Hot E, Di Sabatino A, Caprioli F, Bottiglieri L, Oldani A, Viale G, et al. A gut-vascular barrier controls the systemic dissemination of bacteria. Science. 2015;350(6262):830–834. doi:https://doi.org/10.1126/science.aad0135.
- Perez-Lopez A, Behnsen J, Nuccio S-P, Raffatellu M. Mucosal immunity to pathogenic intestinal bacteria. Nat Rev Immunol. 2016;16(3):135–148. doi:https://doi.org/10.1038/nri.2015.17.
- Mowat A. Anatomical basis of tolerance and immunity to intestinal antigens. Nat Rev Immunol. 2003;3(4):331–341. doi:https://doi.org/10.1038/nri1057.
- Powell N, Walker MM, Talley NJ. The mucosal immune system: master regulator of bidirectional gut–brain communications. Nat Rev Gastroenterol Hepatol. 2017;14(3):143–159. doi:https://doi.org/10.1038/nrgastro.2016.191.
- Clemente JC, Ursell LK, Parfrey,and LW, Knight R. The Impact of the Gut Microbiota on Human Health: an Integrative View. Cell. 2012;148(6):1258–1270. doi:https://doi.org/10.1016/j.cell.2012.01.035.
- Kamada N, Núñez G. Regulation of the immune system by the resident intestinal bacteria. Gastroenterology. 2014;146(6):1477–1488. doi:https://doi.org/10.1053/j.gastro.2014.01.060.
- Pickard JM, Zeng MY, Caruso R, Núñez G. Gut microbiota: role in pathogen colonization, immune responses, and inflammatory disease. Immunol Rev. 2017;279(1):70–89. doi:https://doi.org/10.1111/imr.12567.
- Iacob S, Iacob DG. Infectious Threats, the Intestinal Barrier, and Its Trojan Horse: dysbiosis. Front Microbiol. 2019;10:1676. doi:https://doi.org/10.3389/fmicb.2019.01676.
- McDonald D, Ackermann G, Khailova L, Baird C, Heyland D, Kozar R, Lemieux M, Derenski K, King J, Vis-Kampen C, et al. Extreme Dysbiosis of the Microbiome in Critical Illness. mSphere. 2016;1(4):4. doi:https://doi.org/10.1128/msphere.00199-16.
- You N, Zhuo L, Zhou J, Song Y, Shi J. The Role of Intestinal Fungi and Its Metabolites in Chronic Liver Diseases. Gut Liver. 2020;14(3):291–296. doi:https://doi.org/10.5009/gnl18579.
- Lang S, Demir M, Martin A, Jiang L, Zhang X, Duan Y, Gao B, Wisplinghoff H, Kasper P, Roderburg C, et al. Intestinal Virome Signature Associated With Severity of Nonalcoholic Fatty Liver Disease. Gastroenterology. 2020;159(5):1839–1852. Published online July 9. doi:https://doi.org/10.1053/j.gastro.2020.07.005.
- Shanahan F, Hill C. Language, numeracy and logic in microbiome science. Nat Rev Gastroenterol Hepatol. 2019;16(7):387–388. doi:https://doi.org/10.1038/s41575-019-0163-5.
- Wahlström A, Sayin SI, Marschall H-U, Bäckhed F. Intestinal Crosstalk between Bile Acids and Microbiota and Its Impact on Host Metabolism. Cell Metab. 2016;24(1):41–50. doi:https://doi.org/10.1016/j.cmet.2016.05.005.
- McCormick TS, Weinberg A. Epithelial cell-derived antimicrobial peptides are multi-functional agents that bridge innate and adaptive immunity. Periodontol. 2010;54(1):1. doi:https://doi.org/10.1111/j.1600-0757.2010.00373.x.
- Bevins CL, Salzman NH. Paneth cells, antimicrobial peptides and maintenance of intestinal homeostasis. Nat Rev Microbiol. 2011;9(5):356–368. doi:https://doi.org/10.1038/nrmicro2546.
- Schulthess J, Pandey S, Capitani M, Rue-Albrecht KC, Arnold I, Franchini F, Chomka A, Ilott NE, Johnston DGW, Pires E, et al. The Short Chain Fatty Acid Butyrate Imprints an Antimicrobial Program in Macrophages. Immunity. 2019;50(2):432–445. e7. doi:https://doi.org/10.1016/j.immuni.2018.12.018.
- Karlsson J, Pütsep K, Chu H, Kays RJ, Bevins CL, Andersson M. Regional variations in Paneth cell antimicrobial peptide expression along the mouse intestinal tract. BMC Immunol. 2008;9(1):37. doi:https://doi.org/10.1186/1471-2172-9-37.
- Wehkamp J, Chu H, Shen B, Feathers RW, Kays RJ, Lee SK, Bevins CL. Paneth cell antimicrobial peptides: topographical distribution and quantification in human gastrointestinal tissues. FEBS Lett. 2006;580(22):5344–5350. doi:https://doi.org/10.1016/j.febslet.2006.08.083.
- Wells JM, Brummer RJ, Derrien M, MacDonald TT, Troost F, Cani PD, Theodorou V, Dekker J, Méheust A, de Vos WM, et al. Homeostasis of the gut barrier and potential biomarkers. Am J Physiol-Gastrointest Liver Physiol. 2017;312(3):G171–G193. doi:https://doi.org/10.1152/ajpgi.00048.2015.
- Ayabe T, Satchell DP, Wilson CL, Parks WC, Selsted ME, Ouellette AJ. Secretion of microbicidal alpha-defensins by intestinal Paneth cells in response to bacteria. Nat Immunol. 2000;1(2):113–118. doi:https://doi.org/10.1038/77783.
- O’Neil DA, Porter EM, Elewaut D, Anderson GM, Eckmann L, Ganz T, Kagnoff MF. Expression and regulation of the human beta-defensins hBD-1 and hBD-2 in intestinal epithelium. J Immunol. 1999;163:6718–6724.
- Loonen LMP, Stolte EH, Jaklofsky MTJ, Meijerink M, Dekker J, van Baarlen P, Wells JM. REG3γ-deficient mice have altered mucus distribution and increased mucosal inflammatory responses to the microbiota and enteric pathogens in the ileum. Mucosal Immunol. 2014;7(4):939–947. doi:https://doi.org/10.1038/mi.2013.109.
- Muniz LR, Knosp C, Yeretssian G. Intestinal antimicrobial peptides during homeostasis, infection, and disease. Front Immunol. 2012:3. doi:https://doi.org/10.3389/fimmu.2012.00310.
- Corthésy B. Multi-Faceted Functions of Secretory IgA at Mucosal Surfaces. Front Immunol. 2013:4. doi:https://doi.org/10.3389/fimmu.2013.00185.
- Peterson DA, McNulty NP, Guruge JL, Gordon JI. IgA Response to Symbiotic Bacteria as a Mediator of Gut Homeostasis. Cell Host Microbe. 2007;2(5):328–339. doi:https://doi.org/10.1016/j.chom.2007.09.013.
- Fagarasan S. Critical Roles of Activation-Induced Cytidine Deaminase in the Homeostasis of Gut Flora. Science. 2002;298(5597):1424–1427. doi:https://doi.org/10.1126/science.1077336.
- Klemola T. Immunohistochemical Findings in the Intestine of IgA-Deficient Persons: number of Intraepithelial T Lymphocytes Is Increased. J Pediatr Gastroenterol Nutr. 1988;7(4):537–543. doi:https://doi.org/10.1097/00005176-198807000-00010.
- Yazdani R, Azizi G, Abolhassani H, Aghamohammadi A. Selective IgA Deficiency: epidemiology, Pathogenesis, Clinical Phenotype, Diagnosis, Prognosis and Management. Scand J Immunol. 2017;85(1):3–12. doi:https://doi.org/10.1111/sji.12499.
- Barker N, van de Wetering M, Clevers H. The intestinal stem cell. Genes Dev. 2008;22(14):1856–1864. doi:https://doi.org/10.1101/gad.1674008.
- Schroeder BO. Fight them or feed them: how the intestinal mucus layer manages the gut microbiota. Gastroenterol Rep. 2019;7(1):3–12. doi:https://doi.org/10.1093/gastro/goy052.
- Sekirov I, Russell SL, Antunes LCM, Finlay BB. Gut Microbiota in Health and Disease. Physiol Rev. 2010;90(3):859–904. doi:https://doi.org/10.1152/physrev.00045.2009.
- Malin EV, Johansson. The inner of the two Muc2 mucin-dependent mucus layers in colon is devoid of bacteria. Proc Natl Acad Sci. 2008;105(39):15064–15069. doi:https://doi.org/10.1073/pnas.0803124105.
- Bergstrom K, Shan X, Casero D, Batushansky A, Lagishetty V, Jacobs JP, Hoover C, Kondo Y, Shao B, Gao L, et al. Proximal colon–derived O-glycosylated mucus encapsulates and modulates the microbiota. Science. 2020;370(6515):467–472. doi:https://doi.org/10.1126/science.aay7367.
- Lee SH. Intestinal Permeability Regulation by Tight Junction: implication on Inflammatory Bowel Diseases. Intest Res. 2015;13(1):11. doi:https://doi.org/10.5217/ir.2015.13.1.11.
- Furuse M, Hirase T, Itoh M, Nagafuchi A, Yonemura S, Tsukita S, Tsukita S. Occludin: A novel integral membrane protein localizing at tight junctions. J Cell Biol. 1993;123(6):1777–1788. doi:https://doi.org/10.1083/jcb.123.6.1777.
- Al-Sadi R, Khatib K, Guo S, Dongmei Y, Youssef M, Thomas M. Occludin regulates macromolecule flux across the intestinal epithelial tight junction barrier. Am J Physiol-Gastrointest Liver Physiol. 2011;300(6):G1054–G1064. doi:https://doi.org/10.1152/ajpgi.00055.2011.
- Mrsny RJ, Thomas Brown G, Gerner-Smidt K, Buret AG, Meddings JB, Quan C, Koval M, Nusrat A. A key claudin extracellular loop domain is critical for epithelial barrier integrity. Am J Pathol. 2008;172(4):905–915. doi:https://doi.org/10.2353/ajpath.2008.070698.
- Garcia-Hernandez V, Quiros M, Nusrat A. Intestinal epithelial claudins: expression and regulation in homeostasis and inflammation. Ann N Acad Sci. 2017;1397(1):66–79. doi:https://doi.org/10.1111/nyas.13360.
- Rosenthal R, Milatz S, Krug SM, Oelrich B, Schulzke J-D, Amasheh S, Gunzel D, Fromm M. Claudin-2, a component of the tight junction, forms a paracellular water channel. J Cell Sci. 2010;123(11):1913–1921. doi:https://doi.org/10.1242/jcs.060665.
- Tamura A, Hayashi H, Imasato M, Yamazaki Y, Hagiwara A, Wada M, Noda T, Watanabe M, Suzuki Y, Tsukita S, et al. Loss of claudin-15, but not claudin-2, causes Na+ deficiency and glucose malabsorption in mouse small intestine. Gastroenterology. 2011;140(3):913–923. doi:https://doi.org/10.1053/j.gastro.2010.08.006.
- Lameris AL, Huybers S, Kaukinen K, Mäkelä TH, Bindels RJ, Hoenderop JG, Nevalainen PI. Expression profiling of claudins in the human gastrointestinal tract in health and during inflammatory bowel disease. Scand J Gastroenterol. 2013;48(1):58–69. doi:https://doi.org/10.3109/00365521.2012.741616.
- Williams AF, Barclay AN. The immunoglobulin superfamily domains for cell surface recognition. Annu Rev Immunol. 1988;6(1):381–405. doi:https://doi.org/10.1146/annurev.iy.06.040188.002121.
- Ebnet K. Junctional adhesion molecules (JAMs): more molecules with dual functions? J Cell Sci. 2004;117(1):19–29. doi:https://doi.org/10.1242/jcs.00930.
- Laukoetter MG, Nava P, Lee WY, Severson EA, Capaldo CT, Babbin BA, Williams IR, Koval M, Peatman E, Campbell JA, Dermody TS, Nusrat A, Parkos CA. JAM-A regulates permeability and inflammation in the intestine in vivo. J Exp Med. 2007;204(13):3067–3076. doi:https://doi.org/10.1084/jem.20071416.
- Hirabayashi S, Tajima M, Yao I, Nishimura W, Mori H, Hata Y. JAM4, a Junctional Cell Adhesion Molecule Interacting with a Tight Junction Protein, MAGI-1. Mol Cell Biol. 2003;23(12):4267–4282. doi:https://doi.org/10.1128/MCB.23.12.4267-4282.2003.
- Wittchen ES, Haskins J, Stevenson BR. Protein interactions at the tight junction. Actin has multiple binding partners, and ZO-1 forms independent complexes with ZO-2 and ZO-3. J Biol Chem. 1999;274(49):35179–35185. doi:https://doi.org/10.1074/jbc.274.49.35179.
- Itoh M, Sasaki H, Furuse M, Ozaki H, Kita T, Tsukita S. Junctional adhesion molecule (JAM) binds to PAR-3: a possible mechanism for the recruitment of PAR-3 to tight junctions. J Cell Biol. 2001;154(3):491–498. doi:https://doi.org/10.1083/jcb.200103047.
- Bauer H, Zweimueller-Mayer J, Steinbacher P, Lametschwandtner A, Bauer HC. The Dual Role of Zonula Occludens (ZO) Proteins. J Biomed Biotechnol. 2010;2010:1–11. doi:https://doi.org/10.1155/2010/402593.
- Shen L, Black ED, Witkowski ED, Lencer WI, Guerriero V, EE Schneeberger, Turner JR. Myosin light chain phosphorylation regulates barrier function by remodeling tight junction structure. J Cell Sci. 2006;119(10):2095–2106. doi:https://doi.org/10.1242/jcs.02915.
- Asmar RE, Panigrahi P, Bamford P, Berti I, Not T, Coppa GV, Catassi C, Fasano A. Host-dependent zonulin secretion causes the impairment of the small intestine barrier function after bacterial exposure. Gastroenterology. 2002;123(5):1607–1615. doi:https://doi.org/10.1053/gast.2002.36578.
- Fasano A. Zonulin and Its Regulation of Intestinal Barrier Function: the Biological Door to Inflammation, Autoimmunity, and Cancer. Physiol Rev. 2011;91(1):151–175. doi:https://doi.org/10.1152/physrev.00003.2008.
- Perez-Moreno M, Jamora C, Fuchs E. Sticky business: orchestrating cellular signals at adherens junctions. Cell. 2003;112(4):535–548. doi:https://doi.org/10.1016/s0092-8674(03)00108-9.
- Schneider MR, Dahlhoff M, Horst D, Hirschi B, Trülzsch K, Müller-Höcker J, Vogelmann R, Allgäuer M, Gerhard M, Steininger S, et al. A key role for E-cadherin in intestinal homeostasis and Paneth cell maturation. PLoS One. 2010;5(12):e14325. doi:https://doi.org/10.1371/journal.pone.0014325.
- Nita-Lazar M, Rebustini I, Walker J, Kukuruzinska. MA. Hypoglycosylated E-cadherin promotes the assembly of tight junctions through the recruitment of PP2A to adherens junctions. Exp Cell Res. 2010;316(11):1871–1884. doi:https://doi.org/10.1016/j.yexcr.2010.02.008.
- Spadoni I, Pietrelli A, Pesole G, Rescigno M. Gene expression profile of endothelial cells during perturbation of the gut vascular barrier. Gut Microbes. 2016;7(6):540–548. doi:https://doi.org/10.1080/19490976.2016.1239681.
- Corada M, Mariotti M, Thurston G, Smith K, Kunkel R, Brockhaus M, Lampugnani MG, Martin-Padura I, Stoppacciaro A, Ruco L, et al. Vascular endothelial-cadherin is an important determinant of microvascular integrity in vivo. PNAS. 1999;96(17):9815–9820. doi:https://doi.org/10.1073/pnas.96.17.9815.
- Stan RV, Tkachenko E, Niesman IR; Intestinal permeability is increased in children with non-alcoholic fatty liver disease, and correlates with liver disease severity. PV1 is a key structural component for the formation of the stomatal and fenestral diaphragms. Mol Biol Cell. 2004;15(8):3615–3630. doi:https://doi.org/10.1091/mbc.E03-08-0593.
- Aron-Wisnewsky J, Vigliotti C, Witjes J, Le P, Holleboom AG, Verheij J, Nieuwdorp M, Clément K. Gut microbiota and human NAFLD: disentangling microbial signatures from metabolic disorders. Nat Rev Gastroenterol Hepatol. 2020;17(5):279–297. doi:https://doi.org/10.1038/s41575-020-0269-9.
- Bajaj JS, Heuman DM, Hylemon PB, Sanyal AJ, White MB, Monteith P, Noble NA, Unser AB, Daita K, Fisher AR, et al. Altered profile of human gut microbiome is associated with cirrhosis and its complications. J Hepatol. 2014;60(5):940–947. doi:https://doi.org/10.1016/j.jhep.2013.12.019.
- Bajaj JS, Khoruts A. Microbiota changes and intestinal microbiota transplantation in liver diseases and cirrhosis. J Hepatol. 2020;72(5):1003–1027. doi:https://doi.org/10.1016/j.jhep.2020.01.017.
- Oh TG, Kim SM, Caussy C, Fu T, Guo J, Bassirian S, Singh S, Madamba EV, Bettencourt R, Richards L, et al. A Universal Gut-Microbiome-Derived Signature Predicts Cirrhosis. Cell Metab. 2020;32(5):878–888. e6. doi:https://doi.org/10.1016/j.cmet.2020.06.005.
- Wang X, Heazlewood SP, Krause DO, Florin THJ. Molecular characterization of the microbial species that colonize human ileal and colonic mucosa by using 16S rDNA sequence analysis. J Appl Microbiol. 2003;95(3):508–520. doi:https://doi.org/10.1046/j.1365-2672.2003.02005.x.
- Eckburg PB, Bik EM, Bernstein CN, Purdom E, Dethlefsen L, Sargent M, Gill SR, Nelson KE, Relman DA.Diversity of the Human Intestinal Microbial Flora. Science. 2005;308(5728):1635–1638. doi:https://doi.org/10.1126/science.1110591.
- Nakajima M, Arimatsu K, Kato T, Matsuda Y, Minagawa T, Takahashi N, Ohno H, Yamazaki K. Oral Administration of P. gingivalis Induces Dysbiosis of Gut Microbiota and Impaired Barrier Function Leading to Dissemination of Enterobacteria to the Liver. Plos One. 2015;10(7):e0134234. doi:https://doi.org/10.1371/journal.pone.0134234.
- Kitamoto S, Nagao-Kitamoto H, Jiao Y, Gillilland MG, Hayashi A, Imai J, Sugihara K, Miyoshi M, Brazil JC, Kuffa P, et al. The Intermucosal Connection between the Mouth and Gut in Commensal Pathobiont-Driven Colitis. Cell. 2020;182(2):447–462. e14. doi:https://doi.org/10.1016/j.cell.2020.05.048.
- Bajaj JS, Khoruts A. Microbiota changes and intestinal microbiota transplantation in liver diseases and cirrhosis. J Hepatol. 2020;72(5):1003–1027. doi:https://doi.org/10.1016/j.jhep.2020.01.017.
- Schwenger KJ, Clermont-Dejean N, Allard JP. The role of the gut microbiome in chronic liver disease: the clinical evidence revised. JHEP Rep. 2019;1(3):214–226. doi:https://doi.org/10.1016/j.jhepr.2019.04.004.
- Ahmadi S, Wang S, Nagpal R, Wang B, Jain S, Razazan A, Mishra SP, Zhu X, Wang Z, Kavanagh K, et al. A human-origin probiotic cocktail ameliorates aging-related leaky gut and inflammation via modulating the microbiota/taurine/tight junction axis. JCI Insight 6. 2020;5(9):e132055. doi:https://doi.org/10.1172/jci.insight.132055.
- Ait-Belgnaoui A, Bradesi S, Fioramonti J, Theodorou V, Bueno L. Acute stress-induced hypersensitivity to colonic distension depends upon increase in paracellular permeability: role of myosin light chain kinase. Pain. 2005;113(1):141–147. doi:https://doi.org/10.1016/j.pain.2004.10.002.
- Ait-Belgnaoui A, Han W, Lamine F, H Eutamene, J Fioramonti, L Bueno, V Theodorou.Lactobacillus farciminis treatment suppresses stress induced visceral hypersensitivity: a possible action through interaction with epithelial cell cytoskeleton contraction. Gut. 2006;55(8):1090–1094. doi:https://doi.org/10.1136/gut.2005.084194.
- Wigg AJ, Roberts-Thomson IC, Grose RH, Cummins AG, Dymock RB, McCarthy PJ. The role of small intestinal bacterial overgrowth, intestinal permeability, endotoxaemia, and tumour necrosis factor α in the pathogenesis of non-alcoholic steatohepatitis. Gut. 2001;48(2):206–211. doi:https://doi.org/10.1136/gut.48.2.206. Published online February 1
- Augustyn M, Grys I, Kukla M. Small intestinal bacterial overgrowth and nonalcoholic fatty liver disease. Clin Exp Hepatol. 2019;5(1):1–10. doi:https://doi.org/10.5114/ceh.2019.83151.
- d’Hennezel E, Abubucker S, Murphy LO, Cullen TW. Total Lipopolysaccharide from the Human Gut Microbiome Silences Toll-Like Receptor Signaling. mSystems. 2017;2(6). doi:https://doi.org/10.1128/mSystems.00046-17.
- Di Lorenzo F, De Castro C, Silipo A, Molinaro A. Lipopolysaccharide structures of Gram-negative populations in the gut microbiota and effects on host interactions. FEMS Microbiol Rev. 2019;43(3):257–272. doi:https://doi.org/10.1093/femsre/fuz002.
- Ikeda H, Sasaki M, Ishikawa A, Sato Y, Harada K, Zen Y, Kazumori H, Nakanuma Y. Interaction of Toll-like receptors with bacterial components induces expression of CDX2 and MUC2 in rat biliary epithelium in vivo and in culture. Lab Investig J Tech Methods Pathol. 2007;87(6):559–571. doi:https://doi.org/10.1038/labinvest.3700556.
- Liu Y, Yu X, Zhao J, Zhang H, Zhai Q, Chen W. The role of MUC2 mucin in intestinal homeostasis and the impact of dietary components on MUC2 expression. Int J Biol Macromol. 2020;164:884–891. doi:https://doi.org/10.1016/j.ijbiomac.2020.07.191.
- Seki E, Schnabl B. Role of innate immunity and the microbiota in liver fibrosis: crosstalk between the liver and gut. J Physiol. 2012;590(3):447–458. doi:https://doi.org/10.1113/jphysiol.2011.219691.
- Nicoletti A, Ponziani FR, Biolato M, Valenza V, Marrone G, Sganga G, Gasbarrini A, Miele L, Grieco A. Intestinal permeability in the pathogenesis of liver damage: from non-alcoholic fatty liver disease to liver transplantation. World J Gastroenterol. 2019;25(33):4814–4834. doi:https://doi.org/10.3748/wjg.v25.i33.4814.
- Heymann F, Tacke F. Immunology in the liver–from homeostasis to disease. Nat Rev Gastroenterol Hepatol. 2016;13(2):88–110. doi:https://doi.org/10.1038/nrgastro.2015.200.
- Rokana N, Mallappa RH, Batish VK, Grover S. Interaction between putative probiotic Lactobacillus strains of Indian gut origin and Salmonella: impact on intestinal barrier function. LWT. 2017;84:851–860. doi:https://doi.org/10.1016/j.lwt.2016.08.021.
- Iwashita J, Sato Y, Sugaya H, Takahashi N, Sasaki H, Abe T. mRNA of MUC2 is stimulated by IL-4, IL-13 or TNF-α through a mitogen-activated protein kinase pathway in human colon cancer cells. Immunol Cell Biol. 2003;81(4):275–282. doi:https://doi.org/10.1046/j.1440-1711.2003.t01-1-01163.x.
- Hendrikx T, Schnabl B. Antimicrobial proteins: intestinal guards to protect against liver disease. J Gastroenterol. 2019;54(3):209–217. doi:https://doi.org/10.1007/s00535-018-1521-8.
- Chen J, Wan J, Ye J, Xia L, Lu N. Emerging role of lncRNAs in the normal and diseased intestinal barrier. Inflamm Res Off J Eur Histamine Res Soc Al. 2018;67(9):757–764. doi:https://doi.org/10.1007/s00011-018-1170-7.
- Chen S-W, Wang P-Y, Liu Y-C, Sun L, Zhu J, Zuo S, Ma J, Li T-Y, Zhang J-L, Chen G-W, et al. Effect of Long Noncoding RNA H19 Overexpression on Intestinal Barrier Function and Its Potential Role in the Pathogenesis of Ulcerative Colitis. Inflamm Bowel Dis. 2016;22(11):2582–2592. doi:https://doi.org/10.1097/MIB.0000000000000932.
- Pacifico L, Bonci E, Marandola L, Romaggioli S, Bascetta S, Chiesa C. Increased circulating zonulin in children with biopsy-proven nonalcoholic fatty liver disease. World J Gastroenterol WJG. 2014;20(45):17107–17114. doi:https://doi.org/10.3748/wjg.v20.i45.17107.
- Hendy OM, Elsabaawy MM, Aref MM, Khalaf FM, Oda AMA, Shazly HME. Evaluation of circulating zonulin as a potential marker in the pathogenesis of nonalcoholic fatty liver disease. APMIS. 2017;125(7):607–613. doi:https://doi.org/10.1111/apm.12696.
- Assimakopoulos SF, Tsamandas AC, Tsiaoussis GI, Karatza E, Triantos C, Vagianos CE, Spiliopoulou I, Kaltezioti V, Charonis A, Nikolopoulou VN, et al. Altered intestinal tight junctions’ expression in patients with liver cirrhosis: a pathogenetic mechanism of intestinal hyperpermeability. Eur J Clin Invest. 2012;42(4):439–446. doi:https://doi.org/10.1111/j.1365-2362.2011.02609.x.
- Rahman K, Desai C, Iyer SS, Thorn NE, Kumar P, Liu Y, Smith T, Neish AS, Li H, Tan S, et al. Loss of Junctional Adhesion Molecule A Promotes Severe Steatohepatitis in Mice on a Diet High in Saturated Fat, Fructose, and Cholesterol. Gastroenterology. 2016;151(4):733–746. e12. doi:https://doi.org/10.1053/j.gastro.2016.06.022.
- Schneider MR, Dahlhoff M, Horst D, Hirschi B, Trülzsch K, Müller-Höcker J, Vogelmann R, Allgäuer M, Gerhard M, Steininger S, et al. A key role for E-cadherin in intestinal homeostasis and paneth cell maturation. PLoS ONE. 2010;5(12):e14325. doi:https://doi.org/10.1371/journal.pone.0014325.
- Wu S, Lim K-C, Huang J, Saidi RF, Sears CL. Bacteroides fragilis enterotoxin cleaves the zonula adherens protein, E-cadherin. Proc Natl Acad Sci. 1998;95(25):14979–14984. doi:https://doi.org/10.1073/pnas.95.25.14979.
- Swidsinski A, Weber J, Loening-Baucke V, Hale LP, Spatial Organization LH. Composition of the Mucosal Flora in Patients with Inflammatory Bowel Disease. J Clin Microbiol. 2005;43(7):3380–3389. doi:https://doi.org/10.1128/JCM.43.7.3380-3389.2005.
- Hsiao EY, McBride SW, Hsien S, Sharon G, Hyde E, McCue T, Codelli J, Chow J, Reisman S, Petrosino J, et al. Microbiota modulate behavioral and physiological abnormalities associated with neurodevelopmental disorders. Cell. 2013;155(7):1451–1463. doi:https://doi.org/10.1016/j.cell.2013.11.024.
- J L TV, Db M, Bj O, Oh BJ. Nonalcoholic steatohepatitis: mayo Clinic experiences with a hitherto unnamed disease. Mayo Clin Proc. 1980; 55(7):434–438. [Accessed September 10, 2020]. https://europepmc.org/article/med/7382552.
- Zhu L, Baker SS, Gill C, Liu W, Alkhouri R, Baker RD, Gill SR. Characterization of gut microbiomes in nonalcoholic steatohepatitis (NASH) patients: A connection between endogenous alcohol and NASH. Hepatology. 2013;57(2):601–609. doi:https://doi.org/10.1002/hep.26093.
- Engstler AJ, Aumiller T, Degen C, Dürr M, Weiss E, Maier IB, Schattenberg JM, Jin CJ, Sellmann C, Bergheim I, et al. Insulin resistance alters hepatic ethanol metabolism: studies in mice and children with non-alcoholic fatty liver disease. Gut. 2016;65(9):1564–1571. doi:https://doi.org/10.1136/gutjnl-2014-308379.
- Llorente C, Schnabl B. The Gut Microbiota and Liver Disease. Cell Mol Gastroenterol Hepatol. 2015;1(3):275–284. doi:https://doi.org/10.1016/j.jcmgh.2015.04.003.
- Elshaghabee FMF, Bockelmann W, Meske D, de Vrese M, Walte H-G, Schrezenmeir J, Heller KJ. Ethanol Production by Selected Intestinal Microorganisms and Lactic Acid Bacteria Growing under Different Nutritional Conditions. Front Microbiol. 2016:7. doi:https://doi.org/10.3389/fmicb.2016.00047.
- Frantz JC, McCallum RE. Growth yields and fermentation balance of Bacteroides fragilis cultured in glucose-enriched medium. J Bacteriol. 1979;137(3):1263–1270. doi:https://doi.org/10.1128/JB.137.3.1263-1270.1979.
- Amaretti A, Bernardi T, Tamburini E, Zanoni S, Lomma M, Matteuzzi D, Rossi M. Kinetics and Metabolism of Bifidobacterium adolescentis MB 239 Growing on Glucose, Galactose, Lactose, and Galactooligosaccharides. Appl Environ Microbiol. 2007;73(11):3637–3644. doi:https://doi.org/10.1128/AEM.02914-06.
- Cope K, Risby T, Diehl AM. Increased gastrointestinal ethanol production in obese mice: implications for fatty liver disease pathogenesis. Gastroenterology. 2000;119(5):1340–1347. doi:https://doi.org/10.1053/gast.2000.19267.
- Clark DP. The fermentation pathways of Escherichia coli. FEMS Microbiol Rev. 1989;5(3):223–234. doi:https://doi.org/10.1111/j.1574-6968.1989.tb03398.x.
- Yuan J, Chen C, Cui J, Lu J, Yan C, Wei X, Zhao X, Li N, Li S, Xue G, et al. Fatty Liver Disease Caused by High-Alcohol-Producing Klebsiella pneumoniae. Cell Metab. 2019;30(4):675–688. e7. doi:https://doi.org/10.1016/j.cmet.2019.08.018.
- Elamin EE, Masclee AA, Dekker J, Jonkers DM. Ethanol metabolism and its effects on the intestinal epithelial barrier. Nutr Rev. 2013;71(7):483–499. doi:https://doi.org/10.1111/nure.12027.
- Salaspuro V, Nyfors S, Heine R, Siitonen A, Salaspuro M, Jousimies-Somer H. Ethanol oxidation and acetaldehyde production in vitro by human intestinal strains of Escherichia coli under aerobic, microaerobic, and anaerobic conditions. Scand J Gastroenterol. 1999;34(10):967–973. doi:https://doi.org/10.1080/003655299750025057.
- Samak G, Gangwar R, Meena AS, Rao RG, Shukla PK, Manda B, Narayanan D, Jaggar JH, Rao R. Calcium Channels and Oxidative Stress Mediate a Synergistic Disruption of Tight Junctions by Ethanol and Acetaldehyde in Caco-2 Cell Monolayers. Sci Rep. 2016;6(1):38899. doi:https://doi.org/10.1038/srep38899.
- Engen PA, Green SJ, Voigt RM, Forsyth CB, Keshavarzian A. The Gastrointestinal Microbiome: alcohol Effects on the Composition of Intestinal Microbiota. Alcohol Res Curr Rev. 2015; 37(2):223–236. [Accessed September 10, 2020]. https://www.ncbi.nlm.nih.gov/pmc/articles/PMC4590619/.
- Mutlu EA, Gillevet PM, Rangwala H, Sikaroodi M, Naqvi A, Engen PA, Kwasny M, Lau CK, Keshavarzian A. Colonic microbiome is altered in alcoholism. Am J Physiol-Gastrointest Liver Physiol. 2012;302(9):G966–G978. doi:https://doi.org/10.1152/ajpgi.00380.2011.
- Couch RD, Dailey A, Zaidi F, Navarro K, Forsyth CB, Mutlu E, Engen PA, Keshavarzian A. Alcohol Induced Alterations to the Human Fecal VOC Metabolome. Plos One. 2015;10(3):e0119362. doi:https://doi.org/10.1371/journal.pone.0119362.
- Sibley D, Jerrells TR. Alcohol Consumption by C57BL/6 Mice Is Associated with Depletion of Lymphoid Cells from the Gut-Associated Lymphoid Tissues and Altered Resistance to Oral Infections with Salmonella typhimurium. J Infect Dis. 2000;182(2):482–489. doi:https://doi.org/10.1086/315728.
- Yan AW, Fouts DE, Brandl J, Stärkel P, Torralba M, Schott E, Tsukamoto H, E. Nelson K, A. Brenner D, Schnabl B, et al. Enteric dysbiosis associated with a mouse model of alcoholic liver disease. Hepatology. 2011;53(1):96–105. doi:https://doi.org/10.1002/hep.24018.
- Gottfried EB, Korsten MA, Lieber CS. Alcohol-induced gastric and duodenal lesions in man. Am J Gastroenterol. 1978;70:587–592.
- Cho Y-E, Yu L-R, Abdelmegeed MA, Yoo S-H, Song B-J. Apoptosis of enterocytes and nitration of junctional complex proteins promote alcohol-induced gut leakiness and liver injury. J Hepatol. 2018;69(1):142–153. doi:https://doi.org/10.1016/j.jhep.2018.02.005.
- Wang Y, Tong J, Chang B, Wang B, Zhang D, Wang B. Effects of alcohol on intestinal epithelial barrier permeability and expression of tight junction‑associated proteins. Mol Med Rep. 2014;9(6):2352–2356. doi:https://doi.org/10.3892/mmr.2014.2126.
- Chopyk DM, Kumar P, Raeman R, Liu Y, Smith T, Anania FA. Dysregulation of junctional adhesion molecule-A contributes to ethanol-induced barrier disruption in intestinal epithelial cell monolayers. Physiol Rep. 2017;5(23):e13541. doi:https://doi.org/10.14814/phy2.13541.
- Elamin E, Masclee A, Troost F, Pieters H-J, Keszthelyi D, Aleksa K, Dekker J, Jonkers D. Ethanol Impairs Intestinal Barrier Function in Humans through Mitogen Activated Protein Kinase Signaling: A Combined In Vivo and In Vitro Approach. Plos One. 2014;9(9):e107421. doi:https://doi.org/10.1371/journal.pone.0107421.
- Dunagan M, Chaudhry K, Samak G, Rao RK. Acetaldehyde disrupts tight junctions in Caco-2 cell monolayers by a protein phosphatase 2A-dependent mechanism. Am J Physiol-Gastrointest Liver Physiol. 2012;303(12):G1356–G1364. doi:https://doi.org/10.1152/ajpgi.00526.2011.
- Kale G, Naren AP, Sheth P, Rao RK. Tyrosine phosphorylation of occludin attenuates its interactions with ZO-1, ZO-2, and ZO-3. Biochem Biophys Res Commun. 2003;302(2):324–329. doi:https://doi.org/10.1016/S0006-291X(03)00167-0.
- Elias BC, Suzuki T, Seth A, Giorgianni F, Kale G, Shen L, Turner JR, Naren A, Desiderio DM, Rao R, et al. Phosphorylation of Tyr-398 and Tyr-402 in Occludin Prevents Its Interaction with ZO-1 and Destabilizes Its Assembly at the Tight Junctions. J Biol Chem. 2009;284(3):1559–1569. doi:https://doi.org/10.1074/jbc.M804783200.
- Atkinson KJ, Rao RK. Role of protein tyrosine phosphorylation in acetaldehyde-induced disruption of epithelial tight junctions. Am J Physiol-Gastrointest Liver Physiol. 2001;280(6):G1280–G1288. doi:https://doi.org/10.1152/ajpgi.2001.280.6.G1280.
- Sheth P, Seth A, Atkinson KJ, Gheyi T, Kale G, Giorgianni F, Desiderio D, Li C, Naren A, Rao R, et al. Acetaldehyde dissociates the PTP1B–E-cadherin–β-catenin complex in Caco-2 cell monolayers by a phosphorylation-dependent mechanism. Biochem J. 2007;402(2):291–300. doi:https://doi.org/10.1042/BJ20060665.
- Elamin E, Masclee A, Juuti-Uusitalo K, van IJzendoorn S, Troost F, Pieters H-J, Dekker J, Jonkers D. Fatty Acid Ethyl Esters Induce Intestinal Epithelial Barrier Dysfunction via a Reactive Oxygen Species-Dependent Mechanism in a Three-Dimensional Cell Culture Model. Plos One. 2013;8(3):e58561. doi:https://doi.org/10.1371/journal.pone.0058561.
- Macfarlane S, Macfarlane GT. Regulation of short-chain fatty acid production. Proc Nutr Soc. 2003;62(1):67–72. doi:https://doi.org/10.1079/PNS2002207.
- Fu X, Liu Z, Zhu C, Mou H, Kong Q. Nondigestible carbohydrates, butyrate, and butyrate-producing bacteria. Crit Rev Food Sci Nutr. 2019;59(sup1):S130–S152. doi:https://doi.org/10.1080/10408398.2018.1542587.
- Wang RX, Lee JS, Campbell EL, Colgan SP. Microbiota-derived butyrate dynamically regulates intestinal homeostasis through regulation of actin-associated protein synaptopodin. Proc Natl Acad Sci U S A. 2020;117(21):11648–11657. doi:https://doi.org/10.1073/pnas.1917597117.
- Peng L, He Z, Chen W, Holzman IR, Lin J. Effects of butyrate on intestinal barrier function in a Caco-2 cell monolayer model of intestinal barrier. Pediatr Res. 2007;61(1):37–41. doi:https://doi.org/10.1203/01.pdr.0000250014.92242.f3.
- Mariadason JM, Barkla DH, Gibson PR. Effect of short-chain fatty acids on paracellular permeability in Caco-2 intestinal epithelium model. Am J Physiol. 1997;272(4 Pt 1):G705–712. doi:https://doi.org/10.1152/ajpgi.1997.272.4.G705.
- Vital M, Howe AC, Tiedje JM, Moran MA. Revealing the Bacterial Butyrate Synthesis Pathways by Analyzing (Meta)genomic Data. mBio. 2014;5(2):e00889–14. doi:https://doi.org/10.1128/mBio.00889-14.
- Roediger WEW. Utilization of Nutrients by Isolated Epithelial Cells of the Rat Colon. Gastroenterology. 1982;83(2):424–429. doi:https://doi.org/10.1016/S0016-5085(82)80339-9.
- Kelly CJ, Zheng L, Campbell EL, Saeedi B, Scholz C, Bayless A, Wilson K, Glover L, Kominsky D, Magnuson A, et al. Crosstalk between Microbiota-Derived Short-Chain Fatty Acids and Intestinal Epithelial HIF Augments Tissue Barrier Function. Cell Host Microbe. 2015;17(5):662–671. doi:https://doi.org/10.1016/j.chom.2015.03.005.
- Kelly CJ, Glover LE, Campbell EL, Kominsky DJ, Ehrentraut SF, Bowers BE, Bayless AJ, Saeedi BJ, Colgan SP. Fundamental role for HIF-1α in constitutive expression of human β defensin-1. Mucosal Immunol. 2013;6(6):1110–1118. doi:https://doi.org/10.1038/mi.2013.6.
- Louis NA, Hamilton KE, Canny G, Shekels LL, Ho SB, Colgan SP. Selective induction of mucin-3 by hypoxia in intestinal epithelia. J Cell Biochem. 2006;99(6):1616–1627. doi:https://doi.org/10.1002/jcb.20947.
- Furuta GT, Turner JR, Taylor CT, Hershberg RM, Comerford K, Narravula S, Podolsky DK, Colgan SP. Hypoxia-Inducible Factor 1–Dependent Induction of Intestinal Trefoil Factor Protects Barrier Function during Hypoxia. J Exp Med. 2001;193(9):1027–1034. doi:https://doi.org/10.1084/jem.193.9.1027.
- Velázquez OC, Seto RW, Bain AM, Fisher J, Rombeau JL. Deoxycholate inhibits in vivo butyrate-mediated BrDU labeling of the colonic crypt. J Surg Res. 1997;69(2):344–348. doi:https://doi.org/10.1006/jsre.1997.5075.
- Lupton JR. Microbial Degradation Products Influence Colon Cancer Risk: the Butyrate Controversy. J Nutr. 2004;134(2):479–482. doi:https://doi.org/10.1093/jn/134.2.479.
- Flandez M, Guilmeau S, Blache P, Augenlicht LH. KLF4 regulation in intestinal epithelial cell maturation. Exp Cell Res. 2008;314(20):3712–3723. doi:https://doi.org/10.1016/j.yexcr.2008.10.004.
- Liu Q, Yu Z, Tian F, Zhao J, Zhang H, Zhai Q, Chen W. Surface components and metabolites of probiotics for regulation of intestinal epithelial barrier. Microb Cell Factories. 2020;19(1):23. doi:https://doi.org/10.1186/s12934-020-1289-4.
- Burger-van Paassen N, Vincent A, Puiman PJ, van der Sluis M, Bouma J, Boehm G, van Goudoever J, van Seuningen I, Renes I. The regulation of intestinal mucin MUC2 expression by short-chain fatty acids: implications for epithelial protection. Biochem J. 2009;420(2):211–219. doi:https://doi.org/10.1042/BJ20082222.
- Peng L, Li Z-R, Green RS, Holzman IR, Lin J. Butyrate Enhances the Intestinal Barrier by Facilitating Tight Junction Assembly via Activation of AMP-Activated Protein Kinase in Caco-2 Cell Monolayers. J Nutr. 2009;139(9):1619–1625. doi:https://doi.org/10.3945/jn.109.104638.
- Ma X, Fan PX, Li LS, Qiao SY, Zhang GL, Li DF. Butyrate promotes the recovering of intestinal wound healing through its positive effect on the tight junctions. J Anim Sci. 2012;90(suppl_4):266–268. doi:https://doi.org/10.2527/jas.50965.
- Zheng L, Kelly CJ, Battista KD, Schaefer R, Lanis JM, Alexeev EE, Wang RX, Onyiah JC, Kominsky DJ, Colgan SP. Microbial-Derived Butyrate Promotes Epithelial Barrier Function through IL-10 Receptor–Dependent Repression of Claudin-2 | the Journal of Immunology. J Immunol. 2017;199(8):2976–2984. doi:https://doi.org/10.4049/jimmunol.1700105.
- Singh N, Gurav A, Sivaprakasam S, Brady E, Padia R, Shi H, Thangaraju M, Prasad P, Manicassamy S, Munn D, et al. Activation of Gpr109a, Receptor for Niacin and the Commensal Metabolite Butyrate, Suppresses Colonic Inflammation and Carcinogenesis. Immunity. 2014;40(1):128–139. doi:https://doi.org/10.1016/j.immuni.2013.12.007.
- Levy M, Thaiss CA, Zeevi D, Dohnalová L, Zilberman-Schapira G, Mahdi J, David E, Savidor A, Korem T, Herzig Y, et al. Microbiota-Modulated Metabolites Shape the Intestinal Microenvironment by Regulating NLRP6 Inflammasome Signaling. Cell. 2015;163(6):1428–1443. doi:https://doi.org/10.1016/j.cell.2015.10.048.
- Couto MR, Gonçalves P, Magro F, Martel F. Microbiota-derived butyrate regulates intestinal inflammation: focus on inflammatory bowel disease. Pharmacol Res. 2020;159:104947. doi:https://doi.org/10.1016/j.phrs.2020.104947.
- Zhao Y, Chen F, Wu W, Sun M, Bilotta AJ, Yao S, Xiao Y, Huang X, Eaves-Pyles TD, Golovko G, et al. GPR43 mediates microbiota metabolite SCFA regulation of antimicrobial peptide expression in intestinal epithelial cells via activation of mTOR and STAT3. Mucosal Immunol. 2018;11(3):752–762. doi:https://doi.org/10.1038/mi.2017.118.
- Hase K, Eckmann L, Leopard JD, Varki N, Kagnoff MF. Cell Differentiation Is a Key Determinant of Cathelicidin LL-37/Human Cationic Antimicrobial Protein 18 Expression by Human Colon Epithelium. Infect Immun. 2002;70(2):953–963. doi:https://doi.org/10.1128/IAI.70.2.953-963.2002.
- Schauber J, Svanholm C, Termén S, Iffl K, Menzel T, Scheppach W, Melcher R, Agerberth B, Lührs H, Gudmundsson GH. Expression of the cathelicidin LL-37 is modulated by short chain fatty acids in colonocytes: relevance of signalling pathways | gut. Gut. 2003;52(5):735–741. doi:https://doi.org/10.1136/gut.52.5.735.
- Michail S, Lin M, Frey MR, Fanter R, Paliy O, Hilbush B, Reo NV. Altered gut microbial energy and metabolism in children with non-alcoholic fatty liver disease. FEMS Microbiol Ecol. 2015;91(2):1–9. doi:https://doi.org/10.1093/femsec/fiu002.
- Raman M, Ahmed I, Gillevet PM, Probert CS, Ratcliffe NM, Smith S, Greenwood R, Sikaroodi M, Lam V, Crotty P, et al. Fecal Microbiome and Volatile Organic Compound Metabolome in Obese Humans With Nonalcoholic Fatty Liver Disease. Clin Gastroenterol Hepatol. 2013;11(7):868–875. e3. doi:https://doi.org/10.1016/j.cgh.2013.02.015.
- Loomba R, Ahmed I, Seguritan V, Li W, Long T, Klitgord N, Bhatt A, Dulai PS, Caussy C, Bettencourt R, Highlander SK, et al. Gut Microbiome-Based Metagenomic Signature for Non-invasive Detection of Advanced Fibrosis in Human Nonalcoholic Fatty Liver Disease. Cell Metab. 2017;25(5):1054–1062. e5. doi:https://doi.org/10.1016/j.cgh.2013.02.015.
- Chen ML, Takeda K, Sundrud MS. Emerging roles of bile acids in mucosal immunity and inflammation. Mucosal Immunol. 2019;12(4):851–861. doi:https://doi.org/10.1016/j.cmet.2017.04.001.
- de Aguiar Vallim TQ, Tarling EJ, Edwards PA. Pleiotropic Roles of Bile Acids in Metabolism. Cell Metab. 2013;17(5):657–669. doi:https://doi.org/10.1016/j.cmet.2013.03.013.
- Jones BV, Begley M, Hill C, Gahan CGM, Marchesi JR. Functional and comparative metagenomic analysis of bile salt hydrolase activity in the human gut microbiome. Proc Natl Acad Sci. 2008;105(36):13580–13585. doi:https://doi.org/10.1073/pnas.0804437105.
- Vital M, Rud T, Rath S, Pieper DH, Schlüter D. Diversity of Bacteria Exhibiting Bile Acid-inducible 7α-dehydroxylation Genes in the Human Gut. Comput Struct Biotechnol J. 2019;17:1016–1019. doi:https://doi.org/10.1016/j.csbj.2019.07.012.
- Perez MJ, Briz O. Bile-acid-induced cell injury and protection. World J Gastroenterol WJG. 2009;15(14):1677–1689. doi:https://doi.org/10.3748/wjg.15.1677.
- Kurdi P, Kawanishi K, Mizutani K, Yokota A. Mechanism of Growth Inhibition by Free Bile Acids in Lactobacilli and Bifidobacteria. J Bacteriol. 2006;188(5):1979–1986. doi:https://doi.org/10.1128/JB.188.5.1979-1986.2006.
- Tian Y, Gui W, Koo I, Smith PB, Allman EL, Nichols RG, Rimal B, Cai J, Liu Q, Patterson AD, et al. The microbiome modulating activity of bile acids. Gut Microbes. 2020;11(4):979–996. doi:https://doi.org/10.1080/19490976.2020.1732268.
- Inagaki T, Moschetta A, Lee Y-K, Peng L, Zhao G, Downes M, Yu RT, Shelton JM, Richardson JA, Repa JJ, et al. Regulation of antibacterial defense in the small intestine by the nuclear bile acid receptor. Proc Natl Acad Sci. 2006;103(10):3920–3925. doi:https://doi.org/10.1073/pnas.0509592103.
- Jiao N, Baker SS, Chapa-Rodriguez A, Liu W, Nugent CA, Tsompana M, Mastrandrea L, Buck MJ, Baker RD, Genco RJ, et al. Suppressed hepatic bile acid signalling despite elevated production of primary and secondary bile acids in NAFLD. Gut. 2018;67(10):10. doi:https://doi.org/10.1136/gutjnl-2017-314307.
- Ferslew BC, Xie G, Johnston CK, Su M, Stewart PW, Jia W, Brouwer KLR, Sidney Barritt A. Altered Bile Acid Metabolome in Patients with Nonalcoholic Steatohepatitis. Dig Dis Sci. 2015;60(11):3318–3328. doi:https://doi.org/10.1007/s10620-015-3776-8.
- Puri P, Daita K, Joyce A, Mirshahi F, Santhekadur PK, Cazanave S, Luketic VA, Siddiqui MS, Boyett S, Min H-K, et al. The presence and severity of nonalcoholic steatohepatitis is associated with specific changes in circulating bile acids. Hepatology. 2018;67(2):534–548. doi:https://doi.org/10.1002/hep.29359.
- Kalhan SC, Guo L, Edmison J, Dasarathy S, McCullough AJ, Hanson RW, Milburn M. Plasma metabolomic profile in nonalcoholic fatty liver disease. Metabolism. 2011;60(3):404–413. doi:https://doi.org/10.1016/j.metabol.2010.03.006.
- Rodríguez-Gallego E, Guirro M, Riera-Borrull M, Hernández-Aguilera A, Mariné-Casadó R, Fernández-Arroyo R, Beltrán-Debón R, Sabench F, Hernández F, del Castillo D, Menendez JA , Camps J, Ras R , Arola L , Joven J. Mapping of the circulating metabolome reveals α-ketoglutarate as a predictor of morbid obesity-associated non-alcoholic fatty liver disease. Int J Obes 2005. 2015;39(2):279–287. doi:https://doi.org/10.1038/ijo.2014.53.
- Parks DJ, Blanchard SG, Bledsoe RK, Chandra G, Consler TG , Kliewer SA, Stimmel JB, Willson TM, Zavacki AM, Moore DD, Lehmann JM.Bile Acids: natural Ligands for an Orphan Nuclear Receptor. Science. 1999;284(5418):1365–1368. doi:https://doi.org/10.1126/science.284.5418.1365.
- Bartram H-P, Scheppach W, Schmid H, Hofmann A, Dusel G, Richter F, Richter A, Kasper H.Proliferation of Human Colonic Mucosa as an Intermediate Biomarker of Carcinogenesis: effects of Butyrate, Deoxycholate, Calcium, Ammonia, and pH. Cancer Res. 1993;53(14):3283–3288 [Accessed November 3, 2020]. https://cancerres.aacrjournals.org/content/53/14/3283.
- Lee HY, Crawley S, Hokari R, Kwon S, Kim YS. Bile acid regulates MUC2 transcription in colon cancer cells via positive EGFR/PKC/Ras/ERK/CREB, PI3K/Akt/IκB/NF-κB and p38/MSK1/CREB pathways and negative JNK/c-Jun/AP-1 pathway. Int J Oncol. 2010;36(4):941–953. doi:https://doi.org/10.3892/ijo_00000573.
- Lee G, You HJ, Bajaj JS, Joo SK, Yu J, Park S, Kang H, Park JH, Kim JH, Lee DH, et al. Distinct signatures of gut microbiome and metabolites associated with significant fibrosis in non-obese NAFLD. Nat Commun. 2020;11(1):4982. doi:https://doi.org/10.1038/s41467-020-18754-5.
- Adams LA, Wang Z, Liddle C, Melton PE, Ariff A, Chandraratna H, Tan J, Ching H, Coulter S, Boer B, et al. Bile acids associate with specific gut microbiota, low-level alcohol consumption and liver fibrosis in patients with non-alcoholic fatty liver disease. Liver Int. 2020;40(6):1356–1365. doi:https://doi.org/10.1111/liv.14453.
- Makishima M, Okamoto AY, Repa JJ, Tu H, Learned RM, Luk A, Hull MV, Lustig KD, Mangelsdorf DJ, Shan B. Identification of a Nuclear Receptor for Bile Acids. Science. 1999;284(5418):1362–1365. doi:https://doi.org/10.1126/science.284.5418.1362.
- Ritze Y, Bárdos G, Hubert A, Böhle M, Bischoff SC. Effect of tryptophan supplementation on diet-induced non-alcoholic fatty liver disease in mice. Br J Nutr. 2014;112(1):1–7. doi:https://doi.org/10.1017/S0007114514000440.
- Celinski K, Konturek PC, Slomka M, Cichoz-Lach H, Brzozowski T, Konturek SJ, Korolczuk A. Effects of treatment with melatonin and tryptophan on liver enzymes, parameters of fat metabolism and plasma levels of cytokines in patients with non-alcoholic fatty liver disease–14 months follow up. J Physiol Pharmacol Off J Pol Physiol Soc. 2014;65(1):75–82.
- Beaumont M, Neyrinck AM, Olivares M, Rodriguez J, Rocca Serra A, Roumain M, Bindels LB, Cani PD, Evenepoel P, Muccioli GG, et al. The gut microbiota metabolite indole alleviates liver inflammation in mice. Faseb J. 2018;32(12):6681–6693. doi:https://doi.org/10.1096/fj.201800544.
- Zhao Z-H, Xin F-Z, Xue Y, Hu Z, Han Y, Ma F, Zhou D, Liu X-L, Cui A, Liu Z, et al. Indole-3-propionic acid inhibits gut dysbiosis and endotoxin leakage to attenuate steatohepatitis in rats. Exp Mol Med. 2019;51(9):1–14. doi:https://doi.org/10.1038/s12276-019-0304-5.
- Zelante T, Iannitti RG, Fallarino F, Gargaro M, De Luca A, Moretti S, Bartoli A, Romani L. Tryptophan Feeding of the IDO1-AhR Axis in Host-Microbial Symbiosis. Front Immunol. 2014;5:640. doi:https://doi.org/10.3389/fimmu.2014.00640.
- Sugimoto K, Ogawa A, Mizoguchi E, Shimomura Y, Andoh A, Bhan AK, Blumberg RS, Xavier RJ, Mizoguchi A. IL-22 ameliorates intestinal inflammation in a mouse model of ulcerative colitis. J Clin Invest. 2008 [Published online January 2];118(2):JCI33194. doi:https://doi.org/10.1172/JCI33194.
- Venkatesh M, Mukherjee S, Wang H, Li H, Sun K, Benechet A, Qiu Z, Maher L, Redinbo M, Phillips R, et al. Symbiotic Bacterial Metabolites Regulate Gastrointestinal Barrier Function via the Xenobiotic Sensor PXR and Toll-like Receptor 4. Immunity. 2014;41(2):296–310. doi:https://doi.org/10.1016/j.immuni.2014.06.014.
- Laurans L, Venteclef N, Haddad Y, Chajadine M, Alzaid F, Metghalchi S, Sovran B, Denis RGP, Dairou J, Cardellini M, et al. Genetic deficiency of indoleamine 2,3-dioxygenase promotes gut microbiota-mediated metabolic health. Nat Med. 2018;24(8):1113–1120. doi:https://doi.org/10.1038/s41591-018-0060-4.
- Wolowczuk I, Hennart B, Leloire A, Bessede A, Soichot M, Taront S, Caiazzo R, Raverdy V, Pigeyre M, Guillemin GJ, et al. Tryptophan metabolism activation by indoleamine 2,3-dioxygenase in adipose tissue of obese women: an attempt to maintain immune homeostasis and vascular tone. Am J Physiol-Regul Integr Comp Physiol. 2012;303(2):R135–R143. doi:https://doi.org/10.1152/ajpregu.00373.2011.
- Gershon MD, Tack J. The Serotonin Signaling System: from Basic Understanding To Drug Development for Functional GI Disorders. Gastroenterology. 2007;132(1):397–414. doi:https://doi.org/10.1053/j.gastro.2006.11.002.
- Yano JM, Yu K, Donaldson GP, Shastri G, Ann P, Ma L, Nagler C, Ismagilov R, Mazmanian S, Hsiao E, et al. Indigenous Bacteria from the Gut Microbiota Regulate Host Serotonin Biosynthesis. Cell. 2015;161(2):264–276. doi:https://doi.org/10.1016/j.cell.2015.02.047.
- Nzakizwanayo J, Dedi C, Standen G, Macfarlane WM, Patel BA, Jones BV. Escherichia coli Nissle 1917 enhances bioavailability of serotonin in gut tissues through modulation of synthesis and clearance. Sci Rep. 2015;5(1):17324. doi:https://doi.org/10.1038/srep17324.
- Fung TC, Vuong HE, Luna CDG, Pronovost GN, Aleksandrova AA, Riley NG, Vavilina A, McGinn J, Rendon T, Forrest LR, et al. Intestinal serotonin and fluoxetine exposure modulate bacterial colonization in the gut. Nat Microbiol. 2019;4(12):2064–2073. doi:https://doi.org/10.1038/s41564-019-0540-4.
- Wang L, Fan X, Han J, Cai M, Wang X, Wang Y, Shang J. Gut-Derived Serotonin Contributes to the Progression of Non-Alcoholic Steatohepatitis via the Liver HTR2A/PPARγ2 Pathway. Front Pharmacol. 2020;11:553. doi:https://doi.org/10.3389/fphar.2020.00553.
- Crane JD, Palanivel R, Mottillo EP, Bujak AL, Wang H, Ford RJ, Collins A, Blümer RM, Fullerton MD, Yabut JM, et al. Inhibiting peripheral serotonin synthesis reduces obesity and metabolic dysfunction by promoting brown adipose tissue thermogenesis. Nat Med. 2015;21(2):166–172. doi:https://doi.org/10.1038/nm.3766.
- Choi W, Namkung J, Hwang I, Kim H, Lim A, Park HJ, Lee HW, Han K-H, Park S, Jeong J-S, et al. Serotonin signals through a gut-liver axis to regulate hepatic steatosis. Nat Commun. 2018;9(1):4824. doi:https://doi.org/10.1038/s41467-018-07287-7.
- Haub S, Kanuri G, Volynets V, Brune T, Bischoff SC, Bergheim I. Serotonin reuptake transporter (SERT) plays a critical role in the onset of fructose-induced hepatic steatosis in mice. Am J Physiol-Gastrointest Liver Physiol. 2010;298(3):G335–G344. doi:https://doi.org/10.1152/ajpgi.00088.2009.
- Yamada T, Inui A, Hayashi N, Fujimura M, Fujimiya M. Serotonin stimulates endotoxin translocation via 5-HT3 receptors in the rat ileum. Am J Physiol Gastrointest Liver Physiol. 2003;284(5):G782–G788. doi:https://doi.org/10.1152/ajpgi.00376.2002.
- Nylander O, Pihl L. Luminal hypotonicity increases duodenal mucosal permeability by a mechanism involving 5-hydroxytryptamine. Acta Physiol. 2006;186(1):45–58. doi:https://doi.org/10.1111/j.1748-1716.2005.01507.x.
- Haub S, Ritze Y, Ladel I, Saum K, Hubert A, Spruss A, Trautwein C, Bischoff SC. Serotonin receptor type 3 antagonists improve obesity-associated fatty liver disease in mice. J Pharmacol. 2011;339(3):790–798. doi:https://doi.org/10.1124/jpet.111.181834.