ABSTRACT
Background
Rivaroxaban is one of the direct factor Xa inhibitors. Its function in the inactivated coagulation cascade is unclear. The aim of the study was to assess the effect of rivaroxaban on the endothelial integrity and inflammatory properties of endothelial cells stimulated by 25-hydroxycholesterol (25-OHC).
Methods
HUVECs were stimulated with 25-OHC, rivaroxaban and 25-OHC+ rivaroxaban. HUVEC integrity and permeability were measured using the xCELLigence system and paracellular flux assay. The mRNA expression of tissue factor, ICAM-1, VEGF, IL-33, MCP-1, TNF-α was analyzed in the real-time PCR. Apoptosis and viability were measured by flow cytometry. The VEGF protein concentration was assessed by ELISA. The confocal microscope was used to evaluate the expression of VE-cadherin in endothelial cells.
Results
25-OHC decreased endothelial cell integrity and increased the mRNA expression of IL-33, tissue factor, ICAM-1, MCP-1, VEGF, TNF-α as compared to unstimulated controls. Following the stimulation with rivaroxaban, HUVEC restored integrity disrupted by 25-OHC (p < .01). In HUVECs pre-stimulated with oxysterol, rivaroxaban decreased mRNA expression of IL-33, TNF-α, chemokines MCP-1, ICAM-1, VEGF and tissue factor (p < .01). Rivaroxaban 100 mg/ml+25-OHC increased the VE-cadherin expression in endothelium as compared to 25-OHC (p < .05).
Conclusion
Our finding suggests that rivaroxaban may restore the endothelial barrier and inhibit the inflammatory activation caused by oxysterol in vitro.
Graphical abstract The effect of stimulation: 25-hydroksycholesterol (10µg/ml)+ rivaroxaban (100, 500ng/ml) on the barrier function and immune profile of human umbilical vascular endothelial cells (HUVECs)
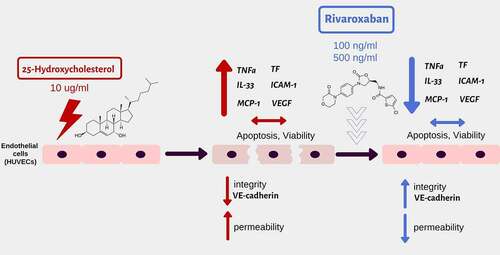
Introduction
Rivaroxaban is a non-VKA oral anticoagulant (NOAC) that directly inhibits factor Xa, thereby suppressing thrombin generation.1 Results of clinical studies have shown that NOACs may reduce the incidence of cardiovascular events, including coronary and peripheral artery disease (PAD), cerebral ischemia, thromboembolic events, and atherosclerosis.Citation1,Citation2 The COMPASS trial (Cardiovascular Outcomes for People Using Anticoagulation Strategies) demonstrated that in patients with chronic coronary syndromes and/or PAD, the combination of rivaroxaban, administered at a dose of 2.5 mg twice daily and acetylsalicylic acid at a dose of 100 mg per day not only prevented cardiovascular death, myocardial infarction, and stroke but even reduced all-cause mortality by around 18% after a mean 23-month follow-up.Citation2
Dysfunction of the vascular endothelium is an important contributor to the pathobiology of atherosclerotic cardiovascular disease.Citation3 Molecular studies have shown that both factor Xa (FXa) and thrombin are involved in inflammatory processes and endothelial activities, including inflammation, vascular remodeling, and atherosclerosis, presumably mediated through protease activated receptors (PARs). Thrombin is an activator of PAR1, whereas FXa activates PAR1, −2, and −3 alone or in complex with TF-FVIIa.Citation4,Citation5 It should be noted that Factor X is not converted to FXa constitutively but is only activated by intrinsic clotting factors and during a cellular injury. The function of rivaroxaban in the inactivated coagulation cascade is unclear. Seki et al. suggest that rivaroxaban per se may not influence gene modulation in the inactivated coagulation state, while rivaroxaban may suppress the expression of pro-inflammatory genes such as MCP-1, ICAM-1, and IL-8, but only in endothelial cells activated by FXa. Interestingly, Daci et al. imply that pre-treatment with rivaroxaban attenuates LPS-induced acute vascular inflammation by a significant reduction of IL-6, MCP-1 and VCAM-1 levels in rat plasma. These anti-inflammatory effects might perhaps have an impact on vascular functions and prevent the development of atherosclerosis.Citation6
Oxysterols are bioactive lipids that act as regulators of inflammation and cell viability. Formation of oxysterols in atherotic lesions is confirmed. 25-hydroxycholesterol (25-OHC) is a type of oxidized cholesterol, which participates in chronic inflammation, vascular proliferation, and development of atherosclerosis.Citation7 It is known that the control of coagulation environment is essential in the treatment of coronary artery disease. Mechanisms by which the inhibition of FXa interrupts atherosclerotic plaque development are complex.Citation8 Histological analyses demonstrated that rivaroxaban significantly decreased lipid deposition, collagen loss, macrophage accumulation, and matrix metallopeptidase-9 (MMP-9) expression in atherosclerotic plaques in the aortic root of ApoE(-/-) mice. Moreover, rivaroxaban significantly reduced mRNA expression of inflammatory molecules, such as MMP-9 and TNF-α.Citation9 In our previous study on HUVECs, we showed that rivaroxaban was able to reduce the level of oxidative damage induced by 25-OHC.Citation10
However, truly little is currently known about the effect of rivaroxaban on endothelial cell damage by atherogenic oxysterols. Firstly, we investigated the effect of rivaroxaban on the integrity and permeability of endothelial cells damaged by 25-hydroxycholesterol. Additionally, we analyzed the rivaroxaban effect on molecular adhesion, cytokine, chemokine, and growth factor expression. Our results suggest that rivaroxaban may restore endothelial integrity and significantly reduce pro-inflammatory profile.
Materials and methods
Chemicals
Human umbilical vascular endothelial cells (HUVECs), trypsin with EDTA, trypsin neutralizing solution, endothelial cell growth medium-2 (EGM-2) with hydrocortisone, hFGF-B, vascular endothelial growth factor (VEGF), R3-IGF-1, ascorbic acid, hEGF, GA-1000, heparin, and fetal bovine serum (FBS) were purchased in Lonza (Basel, Switzerland). Dabigatran, rivaroxaban, and 25-hydroxycholesterol (25-OHC) were bought from Sigma-Aldrich (St. Louis, MO, USA).
Cells
Human umbilical vascular endothelial cells (HUVECs) (Lonza C2517A) were expanded in endothelial basal medium-2 (EGM-2) (Lonza, Clonetics, CC-3162) containing 10% fetal bovine serum (FBS), hydrocortisone, hFGF-B, vascular endothelial growth factor (VEGF), R3-IGF-1, ascorbic acid, hEGF, GA-1000, heparin and penicillin (100 U/mL), and streptomycin (100 µg/mL) at 37°C, 5% CO2. After reaching 80%–90% confluence, the HUVECs were removed by treatment with 0.05% trypsin with 0.02% EDTA for 3 minutes and then neutralized by trypsin neutralizing solution.
Study protocol.
Concentrations of rivaroxaban 100, 500 ng/ml mL (159 nM, 796 nM) were selected on the basis of pilot experiment and previous studies on pharmacokinetics/pharmacodynamics dependence in blood serum. The plasma concentration of rivaroxaban is reported to peak about 141–318 ng/mL (224–506 nM) following a dose of 20 mg.Citation11 The optimal concentrations of the 25-hydroxycholesterol use in this study were determined in pilot experiments and compared with data from publications.Citation12
Cell culture in the real-time cell electric impedance sensing system (RTCA-DP, xCELLigence)
The RTCA-DP xCELLigence system (Roche Applied Science) allows cell growth status to be monitored in real time on microelectrode-coated plates by tracking electrical impedance signals. The impedance readout is expressed in arbitrary units such as cell index (CI); the values reflect changes in barrier properties, monolayer permeability, cell number, viability and adhesion and morphology. The normalized cell index (nCI) is calculated by dividing CI at the normalized time by the original CI value. The rate of cell growth was determined by calculating the slope of the line between two given time points. The integrated software allows data to be collected each minute for any period of time.
In the present study, the impedance measurement system was used for the purpose of dynamic and qualitative analysis of HUVEC cells. The trypsinized HUVEC cells were separately seeded on E-16 plates at a density of 10,000 cells per well in proper media, and CI changes were observed. After reaching the plateau phase, the cells were preincubated with 10 µg/ml 25-hydroxycholesterol for 4 hours, and then incubated with 100 ng/mL, and 500 ng/mL of rivaroxaban (Cayman Chemical, No. 16043) for 40 hours. Cells cultured with medium and 10 µg/ml25-hydroxycholesterol were used as controls.
Six wells were used for each file. Data for cell adherence were normalized (nCl) after 4 hours of preincubation with 25-hydroxycholesterol and before stimulation with rivaroxaban. The HUVEC cultures were analyzed 4, 6, 9, 12, 24, 30, and 40 hours after induction, as the most significant changes could be seen at these time points.
Cell culture in the paracellular flux system
The permeability of HUVEC cells was assessed by paracellular flux with the use of fluorometer. Fluorometry is a direct method for measuring the quantity of light emitted by the sample. HUVECs were seeded into 24-well, PET, 6.5 mm transwell Culture Inserts (Millipore, PIRP12R48) with 1.0 μm pore size in EGM-2 medium. After reaching confluence, HUVECs were pre-stimulated with 10 µg/ml 25-hydroxycholesterol for 4 hours and then stimulated with selected concentration (100 ng/ml) of rivaroxaban. After 12 and 24 hours of incubation, fluorescein (FITC)-coupled dextran with molecular masses of 40-kD (FITC-dextran, Sigma, FD40-100 mg) was added to the upper compartment. After 30 minutes of incubation, the fluorescence of the dextran-FITC in the lower compartment was measured by a Wallac 1420 Victor 2 Microplate Reader. Obtained fluorescence values were subtracted from the background fluorescence of EGM2 medium. Due to the fact that fluorescent techniques are based on direct measurement of light, they possess 1000 to 10000 times greater sensitivity than spectrophotometry; in some cases they can measure concentrations as small as one particle per trillion.
Cell culture in monolayers
HUVECs were seeded on 12-well plates at a density of 50,000 cells per well in 300 µl proper media. After reaching 80–90% confluence, the cells were pre-induced with 10 µg/ml 25-hydroxycholesterol for 4 hours and then induced with two selected concentrations (100, 500 ng/ml) of rivaroxaban for 24 hours. The cultures were then subjected to real-time PCR to determine the expression of selected cytokine mRNAs.
Assessment of apoptosis and viability of HUVEC cells by flow cytometry
After 24 hours, the HUVECs were trypsinized. Apoptosis and viability were assessed in a Beckman Coulter FC500 flow cytometer (Wladyslaw Bieganski Memorial Hospital Laboratory, Lodz, Poland) using Annexin V-FITC and propidium iodide (PI) co-staining (FITC annexin V Apoptosis detection kit, BD Pharmingen, 556547). Annexin-V (-) and PI (-) cells were regarded as living cells, Annexin-V (+) and PI (-) as early apoptotic cells, Annexin-V (+) and PI (+) as late-apoptotic cells, and Annexin-V (-) and PI (+) as necrotic cells. Percentage changes of the Mean Fluorescence Intensity (MFI) were used for an analysis.
mRNA extraction, complementary DNA (cDNA) preparation, real-time polymerase chain reaction (RT-PCR)
mRNA was isolated using the RNeasy Mini Kit (Qiagen) kit, following the manufacturer’s instructions. Potential genomic DNA contamination was removed by on-column DNase I digestion, and 10 μg of mRNA was reverse transcribed with a High-Capacity cDNA Kit (Applied Biosystems, Foster City, CA, US). PCR was then carried out using an Applied Biosystems 9700HT Fast Real-Time PCR System (Applied Biosystems). The PCR mixture consisted of cDNA solution, SYBR-Green PCR Mastermix (Applied Biosystems), and both sense and antisense primers. The reaction was conducted as follows: 4 minutes at 95°C, followed by 40 cycles of 15 s at 95°C and 60 s at 60°C. EF1-α was amplified as a housekeeping gene. IL-33, MCP-1, TNF-α, ICAM-1, VEGF, tissue factor mRNA expression was normalized to EF1-α using ΔΔCt calculation ().
Table 1. Primer sequences used for qPCR
Enzyme-linked immunosorbent assay (ELISA) for VEGF
The presence of VEGF levels in the HUVECs supernatants was assessed in the enzyme-linked immunosorbent assay (ELISA) using an ELISA ST-360 microplate reader (450 nm and 630 nm) according to the manufacturer’s protocol (Diaclone, 873.020.048). The range of VEGF ELISA was 15.6–1000 pg/ml.
Confocal microscopy
HUVEC monolayers were fixed in 4% paraformaldehyde (PFA) for 15 min at room temperature and then incubated with anti-VE-cadherin antibodies (1:200) (MA1-198, Thermo Fisher Scientific, Waltham, MA, USA) overnight at 4°C. After that cells were washed three times in PBS and incubated with a fluorescently tagged goat anti-mouse secondary antibody (1:400) for 2 hours at room temperature. Subsequently, the cells were washed three times in PBS, and the cell nuclei were stained with DAPI (4′,6-diamidino-2-phenylindole) (1:2000, 15 minutes at room temperature) (D1306, Invitrogen, Carlsbad, CA, USA). Mouse IgG2a kappa Isotype Control (eBM2a) and FITC (11–4724-42, eBioscience, Santa Clara, CA, USA) were used as an isotype control. Leica TCS SP8 confocal microscope (Leica-Microsystems, Wetzlar, Germany) featuring 63x/1.40 lens (HC PL APO CS2 Oil Immersion) was used to evaluate the state of intercellular junctional proteins. For visualization of cells supercontinuum laser with 495 nm excitations and emission at 510–580 nm was used. For nuclei stained with DAPI, excitation and emission parameters were 405 nm and 460–480 nm, respectively. Leica software (Wetzlar, Germany) was used to analyze the data.
Statistical analysis
The results are presented as mean ± SEM for variables with normal distribution. The distribution of particular variables was verified by the Shapiro-Wilk W-test, and homogeneity of variance was determined with the Brown–Fisher test. The Tukey’s test was used as a post hoc test. p-value < 0.05 was considered statistically significant. An individual analysis was performed in four independent experiments, with each experiment being repeated twice or three times depending on the method. All analyses were conducted with the STATISTICA v 12.5 software (StatSoft, Inc., Kraków, Poland). The investigations were approved by the Bioethics Committee of the Medical University of Lodz No RNN/236/19/KE.
Results
HUVEC cells were treated with 25-hydroxycholesterol (25-OHC) (10 µg/ml) for 4 hours and then cultured alone or treated with rivaroxaban (100 ng/ml and 500 ng/ml). A control group was set up with cells cultured with control medium and 25-OHC (10 µg/ml).
Rivaroxaban restored the endothelial integrity disturbed by 25-hydroxycholesterol
Endothelial monolayers were stimulated with 25-hydroxycholesterol (10 µg/ml), rivaroxaban (100 or 500 ng/ml) and 25-hydroxycholesterol+ rivaroxaban (100 or 500 ng/ml). Changes in the barrier properties of the HUVEC were monitored dynamically with an RTCA-DP device. Normalized Cell Index (nCI) value were recorded in 4, 6, 9, 12, 24, 30, and 40 hours of culture.
The HUVECs after 24, 30, and 40 hours of culture with rivaroxaban (100 ng/ml) +25-hydroxycholesterol (10 µg/ml) demonstrated higher nCI then those stimulated with 25-hydroxycholesterol alone (p < .01). Rivaroxaban in concentration of 500 ng/ml did not significantly increase nCl in HUVECs pretreated with 25-OHC. There was also no difference in the nCI between HUVEC stimulated by rivaroxaban (100 ng/ml, 500 ng/ml) and unstimulated control () ()).
Table 2. Summary of the normalized cell index (nCl) HUVECs stimulated with 10 µg/ml 25-hydroxycholesterol, as well as with 100 ng/ml or 500 ng/ml rivaroxabanor, 25-hydroxycholesterol + rivaroxaban (100 ng/ml, 500 ng/ml). Results were obtained with the real-time cell electric impedance sensing system
Figure 1. The effect of rivaroxaban 100 or 500 ng/ml on human vein umbilical endothelial cell (HUVEC) barrier functions preincubated with 25-hydroxycholesterol(25-OHC) 10 µg/ml; data collected by real-time cell electric impedance sensing system. (a) The effect of rivaroxaban 100 ng/ml on HUVEC permeability (12 hours or 24 hours) preincubated with 10 µg/ml 25-OHC according to the paracellular flux system (b) n = 9, mean ± SEM of 3 independent experiments; *p < .05, **p < .01, ***p < .001 nCI normalized cell index, these are significant differences between rivaroxaban 100 ng/ml+25-OHC vs 25-OHC after 24 hours of stimulation (one-way ANOVA with post hoc Tukey’s test)
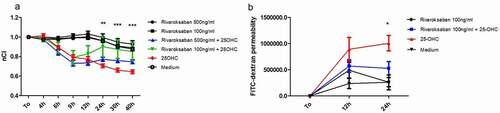
To confirm observations from the RTCA-DP, a paracellular flux assay was used to analyze the effect of rivaroxaban + 25-hydroxycholesterol on HUVEC permeability after 12 and 24 hours of culture. We chose rivaroxaban in concentration of 100 ng/ml for cell stimulation. Treatment with rivaroxaban (100 ng/ml) + 25-hydroxycholesterol (10 µg/ml) significantly lowered HUVEC permeability as compared to 25-hydroxycholesterol alone after 24-h stimulation (528257 ± 287803 vs 1007345 ± 331623) (p < .05). There was no significant effect of rivaroxaban on HUVECs pretreated with 25-OHC after 12 h of culture (571754 ± 271525 vs 891237 ± 505014) ()).
Rivaroxaban and 25-hydroxycholesterol did not affect endothelial viability
The viability and apoptosis of the HUVEC cells were assessed after 24 hours of culture with 25-hydroxycholesterol (10 µg/ml), rivaroxaban (100 and 500 ng/ml) and rivaroxaban (100 and 500 ng/ml) + 25-hydroxycholesterol (10 µg/ml) in flow cytometer.
No significant difference (p > .05) was observed between HUVECs incubated with 25-hydroxycholesterol (10 µg/ml), rivaroxaban (100 and 500 ng/ml) and rivaroxaban (100 and 500 ng/ml) + 25-hydroxycholesterol (10 µg/ml) (p > .05) with regard to the percentage of apoptotic HUVEC cells (Ax+Pi-plus Ax+Pi+) ().
Figure 2. The effect of 25-hydroxycholesterol 10 µg/ml, rivaroxaban (Riv) 100 ng/ml or 500 ng/ml and rivaroxaban 100 ng/ml or 500 ng/ml +10 µg/ml 25-hydroxycholesterol on human endothelial cell (HUVECs) apoptosis and viability (24 h) assessed by flow cytometry, n = 6, mean ± SEM of 3 independent experiments; Ax-Pi- Annexin-V-negative, propidium iodide-negative cells; Ax+Pi- Ax+Pi+ Annexin-V-positive, propidium iodide-negative cells plus Annexin-V-positive, propidium iodide-positive cells, (one-way ANOVA and post hoc Tukey’s test)
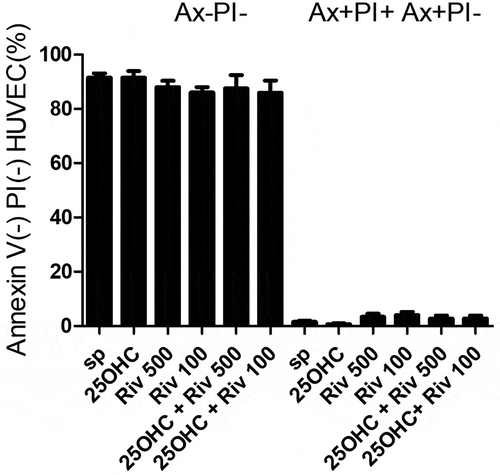
Rivaroxaban decreased mRNA expression of tissue factor in endothelial cells
The next step investigated the effect of 25-hydroxycholesterol 10 µg/ml, rivaroxaban 100 ng/ml and 500 ng/ml and rivaroxaban + 25-hydroxycholesterol on 10 µg/ml the expression of tissue factor (TF) mRNA in HUVECs. Real-time PCR was used to assess the mRNA expression in endothelial cells after 24 hours of culture.
25-hydroxycholesterol treatment significantly increased mRNA expression of tissue factor in HUVECs (p < .001); rivaroxaban 100 ng/ml and 500 ng/ml significantly decreased mRNA expression of TF in HUVECs 4hpreincubatedwith 25-OHC (p < .01) ().
Figure 3. The effect of 25-hydroxycholesterol 10 µg/ml, rivaroxaban 100 ng/ml or 500 ng/ml and rivaroxaban 100mg/ml, 500 ng/ml + 25-hydroxycholesterol 10 µg/ml on mRNA expression Tissue factor on endothelial cells after 24 hours of culture assessed by real-time PCR, n = 6, mean ± SEM of 3 independent experiments; * p < .05, ** p < .01, *** p < .001, (one-way ANOVA with post hoc Tukey’s test)
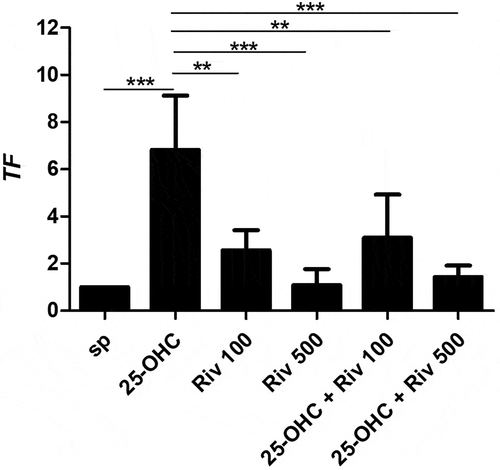
Rivaroxaban decreased expression of ICAM-1 and VEGF in endothelial cells
The effect of 25-hydroxycholesterol 10 µg/ml, rivaroxaban 100 ng/ml and 500 ng/ml, and rivaroxaban 100 ng/ml or 500 ng/ml + 25-hydroxycholesterol 10 µg/ml on mRNA expression of ICAM-1 and VEGF was determined by real-time PCR in endothelial cells after 24 hours of culture. In addition, ELISA was performed to determine the VEGF level in the HUVECs supernatants.
25-OHC increased mRNA expression of ICAM-1 and VEGF in endothelial cells (p < .001). Rivaroxaban 100 ng/ml and 500 ng/ml significantly decreased the mRNA expression of ICAM-1 and VEGFin HUVECs pre-inducted with 25-OHC (, b)) (p < .01). In addition, 100 ng/ml rivaroxaban decreased VEGF level in endothelial cells supernatants activated by 25-OHC (592.65625 ± 77.34375 pg/ml vs 347.5 ± 8.4375 pg/ml; p < .01) ()).
Figure 4. The effect of 25-hydroxycholesterol 10 µg/ml, rivaroxaban 100mg/ml and 500 ng/ml and rivaroxaban 100 ng/ml and 500 ng/ml + 25-hydroxycholesterol 10 µg/ml on mRNA expression: ICAM-1 (a) VEGF (b) in endothelial cells after 24 hours of culture assessed by real-time PCR, n = 6, mean ± SEM of 3 independent experiments; and VEGF levels in the supernatants (c) assessed by ELISA, n = 3, mean ± SEM;* p < .05, ** p < .01, *** p < .001. (one-way ANOVA with post hoc Tukey’s test)
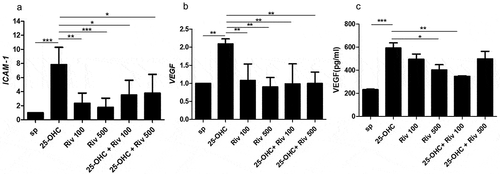
Rivaroxaban increased the expression of VE-cadherin lowered by 25-hydroxycholesterol in endothelial cells
To examine the mechanism behind the stabilizing effect of rivaroxaban on endothelial integrity observed in the RTCA-DP and paracellular flux assay, we analyzed VE-cadherin expression in confocal microscopy. The results indicate that 25-hydroxycholesterol 10 µg/ml decreased the VE-cadherin expression in endothelial cells after 4 hours and 24 hours of culture (p < .05); rivaroxaban 100 ng/ml added to 25-OHC 10 µg/ml increased the concentration of VE-cadherin in confocal microscopy after 24 hours of culture (p < .05) ().
Figure 5. The effect of 25-hydroxycholesterol 10 µg/ml (4 h and 24 h stimulation) Ab, Ac Bb, Bc, and rivaroxaban 100 ng/ml+25-hydroxycholesterol 10 µg/ml Ad, Bd on VE-cadherin expression and endothelial cell morphology in the confocal microscope after 24 hours of culture. (b) Confocal microscopy image of the VE-cadherin of HUVECs (green); a. medium, b. 25-OHC 4 h stimulation, c. 25-OHC 24 h stimulation, d. 100 ng/ml+25-hydroxycholesterol 10 µg/ml. Measurements were taken by, n = 10, mean ± SEM; * p < .05, *** p < .001. (one-way ANOVA with post hoc Tukey’s test)
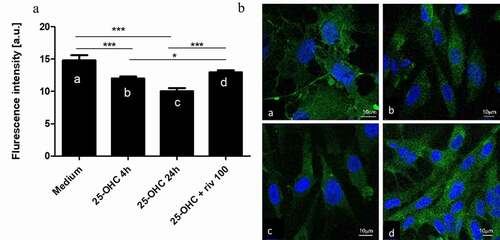
Rivaroxaban inhibits IL-33, MCP-1and TNF-α mRNA expression in endothelial cells
The effect of 25-hydroxycholesterol 10 µg/ml, rivaroxaban 100 ng/ml or 500 ng/ml and rivaroxaban 100 ng/ml and 500 ng/ml+ 25-hydroxycholesterol 10 µg/ml on the expression of IL-33, monocyte chemoattractant protein-1 (MCP-1), TNF-α mRNA in HUVEC cells was performed by real-time PCR after 24 hours of culture ().
Figure 6. The effect of 25-hydroxycholesterol 10 µg/ml, rivaroxaban 100 ng/ml or 500 ng/ml and rivaroxaban 100 ng/ml or 500 ng/ml + 25-hydroxycholesterol 10 µg/ml on the mRNA expression of IL-33 (a), TNFα (b) MCP-1 (c) in endothelial cells damaged after 24 hours of culture. Measurements were taken by real-time PCR, n = 6, mean ± SEM of 3 independent experiments; * p < .05, ** p < .01, *** p < .001. (one-way ANOVA with post hoc Tukey’s test)
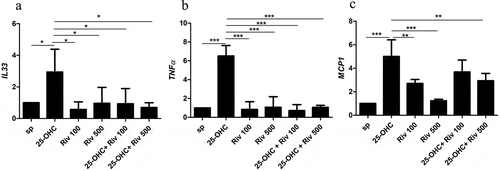
25-hydroxycholesterol significantly increased mRNA expression of IL-33, TNF-α, MCP-1 in HUVECs (p < .001).100 ng/ml and 500 ng/ml of rivaroxaban+ 25-hydroxycholesterol stimulation significantly decreased the mRNA expression of the proinflammatory cytokines IL-33 and TNF-α increased by 25-OHC (p < .01) (, b)). Rivaroxaban of 500 ng/ml + 25-hydroxycholesterol stimulation significantly decreased RNA expression of the pro-inflammatory chemokines MCP-1 in HUVECs as compared to stimulation with 25-OHC (p < .01) ()).
Discussion
Coagulation factors, including FXa which is synthesized in the liver, play a key role not only in the coagulation cascade but also in atherosclerotic disease.Citation13 In this study, we demonstrated that rivaroxaban – a specific inhibitor of FXa may affect direct on the endothelial permeability and the expression of pro-inflammatory agents.
The results of the COMPASS trial have raised important new therapeutic options for rivaroxaban for vascular protection. A low dose of rivaroxaban is the only antithrombotic drug that has demonstrated a net clinical benefit for secondary prevention of atherosclerotic disease, including mortality reduction, with an acceptable bleeding risk. Compared with aspirin alone, rivaroxaban 2.5 mg b.i.d. significantly reduced the risk of the primary endpoint by 24% and the risk of ischemic stroke, myocardial infarction, acute limb ischemia, or death from coronary heart disease by 28%.Citation2,Citation14
25-OHC is a type of oxysterol that belongs to a subgroup of oxidized low-density lipoproteins (oxLDLs), involved in inflammation, vascular proliferation, and promotion of atherosclerosis. There is a strong direct correlation between the total oxysterol levels and the total amount of cholesterol observed in atherosclerotic plaques. This suggests that cholesterol oxidation is an important process in vascular remodeling due to atherothrombosis.Citation15 The mechanism by which 25-OHC injures the endothelial function is based on the eNOS-dependent NO production impairment by 25-OHC. 25-OHC uncouples eNOS activity to generate O2· radicals instead of nitric oxide (NO) to inhibit endothelial cell proliferation, migration, tube formation, and endothelium-dependent vasodilation.Citation16 Furthermore, it has been demonstrated that 25-hydroxycholesterol induced DNA SSBs, as well as oxidative damage to purines and pyrimidines, and increased ROS in human HUVECs.Citation10
A key finding of this study is the fact that rivaroxaban treatment at lower concentrations (100 ng/ml) restored endothelial integrity following by the damage by 25-OHC, as indicated in the RTCA-DP analysis. These observations were confirmed in the paracellular flux assay, in which rivaroxaban decreased the endothelial permeability caused by oxysterol. To our knowledge, this is the first study that shows the repairing effect of rivaroxaban on the endothelial cell damage by oxysterol. The results of our previous study showed that 25-OHC may decrease endothelial integrity in RTCA-DP and cause partial damage in an endothelial monolayer.Citation12 Literature data suggest that rivaroxaban showed endothelium repairing properties, an effect probably mediated by urokinase plasminogen activator (u‐PA) up-regulation and activation, which also contributes to the rivaroxaban‐enhanced fibrinolytic activity on endothelial cells.
Additionally, rivaroxaban neither modified the effect of FXa alone in HUVECs nor stimulated cell growth in the presence of thrombin in the RTCA-DP system.Citation17 Other studies suggest that rivaroxaban may limit the damage to endothelium induced by the thrombin assessed by TEER values. This is most likely due to the fact that rivaroxaban may limit the cleavage of PAR-1 by thrombin.Citation18
To evaluate the mechanism behind the stabilizing effect of rivaroxaban on endothelial integrity observed in the RTCA-DP and paracellular flux assay, the present study analyzed the effect on apoptosis and viability of endothelial cells. The results indicate that neither 25-hydroxycholesterol nor rivaroxaban has any effect on endothelial cell apoptosis and viability. Similar results were obtained by Chalubinski et al., who demonstrated that oxysterols reduce the endothelial cell monolayer confluence in RTCA-DP, without any effect on the viability or apoptosis.Citation12 Other studies have shown that neither rivaroxaban nor 25-hydroxycholesterol were found to affect the endothelial cell viability or apoptosis.Citation10 Moreover, in HUVECs inducted by FXa+rivaroxaban+lipopolysaccharide (LPS) group, the number of apoptotic cells was significantly decreased as compared with FXa+LPS group.Citation19
Healthy endothelial cells express anti-platelet and anti-coagulant agents that prevent platelet aggregation and fibrin formation, respectively. Only activated endothelial cells are able to produce and express TF and other factors of the coagulation cascade such as factor V, specific surface receptors for FXa or thrombin in response to vascular injury or inflammatory stimuli.Citation20 TNFα, MCP-1, VEGF, IL-33, oxLDL, IL-1, IL-6, and LPS are the most important inducers of TF in endothelial cells.Citation20,Citation21 Our findings indicate that rivaroxaban can decrease the expression of TF in HUVECs following the injury by 25-OHC. It has to be noticed that TF has an influence on endothelial cell permeability.Citation22 Plaque rupture exposes TF that activates Factor X and this promotes the conversion of inactive prothrombin to thrombin, which is involved in thrombus formation and coronary occlusion.Citation1 The data showed that rivaroxaban significantly decreased expression of TF in HUVECs activated by thrombin.Citation23
Endothelial cells have two specialized junctional domains, adherens junctions and tight junctions. The tight junctions occupy the most apical position, sealing the cleft edges toward the luminal surface. Their main function in the endothelium is to restrict paracellular permeability. Adherens junctions are localized more basally and, as well as limiting paracellular permeability, they control vessel morphogenesis and stability.Citation24 Vascular endothelial (VE)-cadherin is a strictly endothelial specific adhesion molecule located at junctions between endothelial cells and modulates endothelial permeability.Citation25 Our findings indicate that rivaroxaban 100 mg/ml increased the expression of VE-cadherin lowered by 25-OHC. It has been shown that inflammatory mediators such as thrombin induce intercellular gap formation leading to an increase in endothelial permeability. Furthermore, it has been shown that the activation of ICAM-1 and VEGF signaling leads to increased leukocyte transmigration, vascular permeability, and inflammation, as well as the loss of the endothelial barrier and the rearrangement of the actin cytoskeleton.Citation26 Additionally, VEGF controls endothelial-cell permeability by promoting the β-arrestin-dependent endocytosis of VE-cadherin.Citation25 Our results indicate that 25-OHC may increase VEGF and ICAM-1 mRNA expression, which may be the reason for lowered integrity and increased permeability of endothelial cells; rivaroxaban at concentrations of 100 and 500 ng/ml was able to reduce the expression of VEGF and ICAM-1 in endothelial cells injured with 25-OHC. Similarly, ELISA showed that the level of VEGF in the supernatants from endothelial cells stimulated with rivaroxaban (100 ng/ml) + 25-OHC was lower than in the supernatants of cells treated with 25-OHC alone. Previous studies have demonstrated that rivaroxaban decreased ICAM-1 expression in HUVECs inducted by thrombin.Citation23 There are no data regarding the effect of rivaroxaban on VEGF expression in HUVECs injured by 25-OHC. Literature data suggest that in vitro treatment of endothelial progenitor cells with an activated factor X inhibitor increased the activity of eNOS and the expression of key angiogenic growth factors, such as VEGF.Citation27
Previous studies have demonstrated an anti-inflammatory effect of rivaroxaban. Our results are consistent with a recently reported study in which rivaroxaban was shown to significantly inhibit plasma-induced up-regulation of MCP-1, TNF-α, IL-1β, and IL-6 expression in HUVECs.Citation19,Citation23 In the present study, rivaroxaban was found to decrease the inflammatory activation caused by 25-OHC in endothelial cells. Treatment with 25-OHC significantly increased IL-33, TNFα, and MCP-1 expression, whereas rivaroxaban prevented this increase.
IL-33 is released mostly by injured tissues and serves as both a “danger signal” and the first line of tissue defense to alert immune cells expressing the ST2 receptor. Interestingly, IL-33 has been found to significantly induce TF expression in both HUVECs and human coronary artery endothelial cells. IL-33-induced TF expression was ST2- and NF-κB-dependent, but IL-1-independent. Moreover, IL-33 induces activation of endothelial cells toward an inflammatory phenotype through the up-regulation of IL-6, IL-8, MCP-1, VCAM-1, ICAM-1, and endothelial (E)-selectin.Citation28 The present study is the first one to demonstrate the effect of rivaroxaban on IL-33 expression in endothelial cells. Furthermore, we demonstrate that rivaroxaban may decrease IL-33 mRNA expression in HUVECs damaged by oxysterol.
MCP-1 and TNF-α overexpression has been implicated in several pathological conditions, including atherosclerosis, thrombosis, and inflammatory disease.Citation29,Citation30 Our results showed that rivaroxaban may decrease MCP-1 and TNF-α expression in endothelium injured by oxysterol. Similarly, in HUVEC stimulated with FXa+rivaroxaban+LPS, the levels of inflammatory cytokines such as IL-1β, IL-6, and TNF-α significantly decreased as compared to FXa+LPS stimulation.Citation19 Data demonstrated that 6 and 24 hours after LPS injection, rivaroxaban pre-treatment significantly reduced IL-6, MCP-1, and VCAM-1 plasma concentration.Citation6
To sum up, this work is the first one which presents the direct effect of rivaroxaban on the endothelial cell integrity damage by oxysterol. Additionally, results of our study suggest that rivaroxaban modulates the expression of proinflammatory agents. Thus, rivaroxaban may play an important role in the prevention of atherosclerosis by inhibition of pro-inflammatory activation.
Disclosure statement
No potential conflicts of interest were disclosed.
Additional information
Funding
References
- Sanmartín M, Bellmunt S, Cosín-Sales J, García-Moll X, Riera-Mestre A, Almendro-Delia M, Hernández JL, Lozano F, Mazón P, Suarez Fernández C. Role of rivaroxaban in the prevention of atherosclerotic events. Expert Rev Clin Pharmacol. 2019;12(8):1–12. doi:https://doi.org/10.1080/17512433.2019.1637732.
- Steffel J, Eikelboom JW, Anand SS, Shestakovska O, Yusuf S, Fox KAA. The COMPASS trial: net clinical benefit of low-dose rivaroxaban plus aspirin as compared with aspirin in patients with chronic vascular disease. Circulation. 2020;7:142(1):40–48. doi:https://doi.org/10.1161/CIRCULATIONAHA.120.046048.
- Gimbrone MA Jr, García-Cardeña G. Endothelial cell dysfunction and the pathobiology of atherosclerosis. Circ Res. 2016;118(4):620–636. doi:https://doi.org/10.1161/CIRCRESAHA.115.306301.
- Bono F, Schaeffer P, Hérault JP, Michaux C, Nestor AL, Guillemot JC, Herbert JM. Factor Xa activates endothelial cells by a receptor cascade between EPR-1 and PAR-2. Arterioscler Thromb Vasc Biol. 2000;20(11):E107–12. doi:https://doi.org/10.1161/01.ATV.20.11.e107.
- Kaname S, Yosuke M, Toku S, Shintaro N, Jun T, Yasushi O, Toshihiro M, Shigeyuki N, Takaaki S. Demeanor of rivaroxaban in activated/inactivated FXa. J Pharmacol Sci. 2017;156–161:1347–8613.
- Daci A, Da Dalt L, Alaj R, Shurdhiqi S, Neziri B, Ferizi R, Danilo Norata G, Krasniqi S. Rivaroxaban improves vascular response in LPS-induced acute inflammation in experimental models. PLoS ONE. 2020;15(12):e0240669. doi:https://doi.org/10.1371/journal.pone.0240669.
- Luchetti R, Crinelli E, Cesarini B, Canonico L, Guidi C, Zerbinati G, Di Sario L, Zamai M, Magnani S, Papa L. Iuliano,Endothelial cells, endoplasmic reticulum stress and oxysterols. Redox Biol. 2017;13:581–587. doi:https://doi.org/10.1016/j.redox.2017.07.014.
- Oikonomou E, Leopoulou M, Theofilis P, Antonopoulos AS, Siasos G, Latsios G, Mystakidi VC, Antoniades C, Tousoulis D. A link between inflammation and thrombosis in atherosclerotic cardiovascular diseases: clinical and therapeutic implications. Atherosclerosis. 2020;309:16–26. doi:https://doi.org/10.1016/j.atherosclerosis.2020.07.027.
- Hara T, Fukuda D, Tanaka K, Higashikuni Y, Hirata Y, Nishimoto S, Yagi S, Yamada H, Soeki T, Wakatsuki T, et al. Rivaroxaban, a novel oral anticoagulant, attenuates atherosclerotic plaque progression and destabilization in ApoE-deficient mice. Atherosclerosis. 2015;242(2):639–646. doi:https://doi.org/10.1016/j.atherosclerosis.2015.03.023.
- Woźniak E, Broncel M, Bukowska B, Gorzelak-Pabiś P. The protective effect of dabigatran and rivaroxaban on DNA oxidative changes in a model of vascular endothelial damage with oxidized cholesterol. Int J Mol Sci. 2020;21(6):1953. doi:https://doi.org/10.3390/ijms21061953.
- Gulseth MP, Michaud J, Nutescu EA. Rivaroxaban: an oral direct inhibitor of factor Xa. Am J Health Syst Pharm. 2008;65:1520–1529. doi:https://doi.org/10.2146/ajhp070624.
- Chalubinski M, Zemanek K, Skowron W, Wojdan K, Gorzelak P, Broncel M. The effect of 7-ketocholesterol and 25-hydroxycholesterol on the integrity of the human aortic endothelial and intestinal epithelial barriers. Inflamm Res. 2013;62(12):1015–1023. doi:https://doi.org/10.1007/s00011-013-0660-x.
- Beer JH, Bonetti N. COMPASS to REACH the right patients with thrombocardiology: benefits, risks, and future of the new concept. Eur Heart J. 2018;39(9):758–761. doi:https://doi.org/10.1093/eurheartj/ehx702.
- Abola MTB, Golledge J, Miyata T, Rha SW, Yan BP, Dy TC, Ganzon MSV, Handa PK, Harris S, Zhisheng J, et al. Asia-Pacific consensus statement on the management of peripheral artery disease: a report from the Asian Pacific society of atherosclerosis and vascular disease Asia-Pacific peripheral artery disease consensus statement project committee. J Atheroscler Thromb. 2020;27(8):809–907. doi:https://doi.org/10.5551/jat.53660.
- Leonarduzzi G, Gamba P, Gargiulo S, Biasi F, Poli G. Inflammation‐related gene expression by lipid oxidation‐derived products in the progression of atherosclerosis. Free Radic Biol Med. 2012;52:19–34.
- Ou ZJ, Chen J, Dai WP, Liu X, Yang YK, Li Y, Lin ZB, Wang TT, Wu YY, Su DH, et al. 25-Hydroxycholesterol impairs endothelial function and vasodilation by uncoupling and inhibiting endothelial nitric oxide synthase. Am J Physiol Endocrinol Metab. 2016;311(4):E781–E790. doi:https://doi.org/10.1152/ajpendo.00218.2016.
- Álvarez E, Paradela-Dobarro B, Raposeiras-Roubín S, González-Juanatey JR. Protective, repairing and fibrinolytic effects of rivaroxaban on vascular endothelium. Br J Clin Pharmacol. 2018;84(2):280–291. doi:https://doi.org/10.1111/bcp.13440.
- Puech C, Delavenne X, He Z, Forest V, Mismetti P, Perek N. Direct oral anticoagulants are associated with limited damage of endothelial cells of the blood-brain barrier mediated by the thrombin/PAR-1 pathway. Brain Res. 2019;1719:57–63. doi:https://doi.org/10.1016/j.brainres.2019.05.024.
- Shi M, Huang J, Sun X, Wang F, Chu X, Jiang R, Wang Y, Pang L. Effect of rivaroxaban on the injury during endotoxin-induced damage to human umbilical vein endothelial cells. Zhonghua Wei Zhong Bing Ji Jiu Yi Xue. 2019;31(4):468–473.
- Yau JW, Teoh H, Verma S. Endothelial cell control of thrombosis. BMC Cardiovasc Disord. 2015;15:130. doi:https://doi.org/10.1186/s12872-015-0124-z.
- Kaneko T, Fujii S, Matsumoto A, Goto D, Makita N, Hamada J, Moriuchi T, Kitabatake A. Induction of tissue factor expression in endothelial cells by basic fibroblast growth factor and its modulation by fenofibric acid. Thromb J. 2003;1(1):6. doi:https://doi.org/10.1186/1477-9560-1-6.
- Khakpour S, Wilhelmsen K, Hellman J. Vascular endothelial cell toll-like receptor pathways in sepsis. Innate Immun. 2015;21(8):827–846. doi:https://doi.org/10.1177/1753425915606525.
- Ellinghaus P, Perzborn E, Hauenschild P, Gerdes C, Heitmeier S, Visser M, Summer H, Laux V. Expression of pro-inflammatory genes in human endothelial cells: comparison of rivaroxaban and dabigatran. Thromb Res. 2016;142:44–51. doi:https://doi.org/10.1016/j.thromres.2016.04.008.
- Lampugnani MG. Endothelial cell-to-cell junctions: adhesion and signaling in physiology and pathology. Cold Spring Harb Perspect Med. 2012;2(10). doi:https://doi.org/10.1101/cshperspect.a006528.
- Noda K, Zhang J, Fukuhara S, Kunimoto S, Yoshimura M, Mochizuki N. Vascular endothelial-cadherin stabilizes at cell-cell junctions by anchoring to circumferential actin bundles through alpha- and beta-catenins in cyclic AMP-Epac-Rap1 signal-activated endothelial cells. Mol Biol Cell. 2010;21(4):584–596. doi:https://doi.org/10.1091/mbc.e09-07-0580.
- Sarelius IH, Glading AJ. Control of vascular permeability by adhesion molecules. Tissue Barriers. 2015;3(1–2):e985954. doi:https://doi.org/10.4161/21688370.2014.985954.
- Wu TC, Chan JS, Lee CY, Leu HB, Huang PH, Chen JS, Lin SJ, Chen JW. Rivaroxaban, a factor Xa inhibitor, improves neovascularization in the ischemic hindlimb of streptozotocin-induced diabetic mice. Cardiovasc Diabetol. 2015;14:81. doi:https://doi.org/10.1186/s12933-015-0243-y.
- Stojkovic S, Kaun C, Basilio J, Rauscher S, Hell L, Krychtiuk KA, Bonstingl C, De Martin R, Gröger M, Ay C, et al. Tissue factor is induced by interleukin-33 in human endothelial cells: a new link between coagulation and inflammation. Sci Rep. 2016;6:25171. doi:https://doi.org/10.1038/srep25171.
- Chen PJ, Wang YL, Kuo L-M, Lin CF, Chen CY, Tsai YF, Shen -J-J, Hwang T-L. Honokiol suppresses TNF-α-induced neutrophil adhesion on cerebral endothelial cells by disrupting polyubiquitination and degradation of IκBα. Sci Rep. 2016;6:26554. doi:https://doi.org/10.1038/srep26554.
- Deshmane SL, Kremlev S, Amini S, Sawaya BE. Monocyte chemoattractant protein-1 (MCP-1): an overview. J Interferon Cytokine Res. 2009;29(6):313–326. doi:https://doi.org/10.1089/jir.2008.0027.