ABSTRACT
Engineered nanomaterials induce hazardous effects at the cellular and molecular levels. We investigated different mechanisms underlying the neurotoxic potential of zinc oxide nanoparticles (ZnONPs) on cerebellar tissue and clarified the ameliorative role of Quercetin supplementation. Forty adult male albino rats were divided into control group (I), ZnONPs-exposed group (II), and ZnONPs and Quercetin group (III). Oxidative stress biomarkers (MDA & TOS), antioxidant biomarkers (SOD, GSH, GR, and TAC), serum interleukins (IL-1β, IL-6, IL-8), and tumor necrosis factor alpha (TNF-α) were measured. Serum micro-RNA (miRNA): miRNA-21-5p, miRNA-122-5p, miRNA-125b-5p, and miRNA-155-3p expression levels were quantified by real-time quantitative polymerase-chain reaction (RT-QPCR). Cerebellar tissue sections were stained with Hematoxylin & Eosin and Silver stains and examined microscopically. Expression levels of Calbindin D28k, GFAP, and BAX proteins in cerebellar tissue were detected by immunohistochemistry. Quercetin supplementation lowered oxidative stress biomarkers levels and ameliorated the antioxidant parameters that were decreased by ZnONPs. No significant differences in GR activity were detected between the study groups. ZnONPs significantly increased serum IL-1β, IL-6, IL-8, and TNF-α which were improved with Quercetin. Serum miRNA-21-5p, miRNA-122-5p, miRNA-125b-5p, and miRNA-155-p expression levels showed significant increase in ZnONPs group, while no significant difference was observed between Quercetin-treated group and control group. ZnONPs markedly impaired cerebellar tissue structure with decreased levels of calbindin D28k, increased BAX and GFAP expression. Quercetin supplementation ameliorated cerebellar tissue apoptosis, gliosis and improved calbindin levels. In conclusion: Quercetin supplementation ameliorated cerebellar neurotoxicity induced by ZnONPs at cellular and molecular basis by different studied mechanisms.Abbreviations: NPs: Nanoparticles, ROS: reactive oxygen species, ZnONPs: Zinc oxide nanoparticles, AgNPs: silver nanoparticles, BBB: blood–brain barrier, ncRNAs: Non-coding RNAs, miRNA: Micro RNA, DMSO: Dimethyl sulfoxide, LPO: lipid peroxidation, MDA: malondialdehyde, TBA: thiobarbituric acid, TOS: total oxidative status, ELISA: enzyme-linked immunosorbent assay, H2O2: hydrogen peroxide, SOD: superoxide dismutase, GR: glutathione reductase, TAC: total antioxidant capacity, IL-1: interleukin-1, TNF: tumor necrosis factor alpha, cDNA: complementary DNA, RT-QPCR: Real-time quantitative polymerase-chain reaction, ABC: Avidin biotin complex technique, DAB: 3′, 3-diaminobenzidine, SPSS: Statistical Package for Social Sciences, ANOVA: One way analysis of variance, Tukey’s HSD: Tukey’s Honestly Significant Difference, GFAP: glial fiberillar acitic protein, iNOS: Inducible nitric oxide synthase, NO: nitric oxide, HO-1: heme oxygenase-1, Nrf2: nuclear factor erythroid 2-related factor 2, NF-B: nuclear factor-B, SCI: spinal cord injury, CB: Calbindin
Introduction
Nanotechnology has prompted scientific research advancements. Nanoparticles (NPs) are being considered nowadays as the materials of the future because of their unique properties and numerous applications.Citation1,Citation2 Therefore, they are fast rising in the industrial, medicinal, and military domains. Natural or produced materials, present in aggregate or dispersed condition, with a diameter of less than 100 nm, are defined as NPs by the European Union. They’re exciting new materials with potential applications in medicine, imaging, cosmetics, and food preservation additives.Citation3 They have various characteristics, including a high surface area/mass ratio, small size, and surface reactive chemical groups, all of which aid in endocytosis and contact with intracellular structures. Despite their beneficial effects, several studies reported their ability to generate reactive oxygen species (ROS), as they interact with intracellular biomolecules such proteins, enzymes, and DNA, and potentially affect their function.Citation4,Citation5
Zinc oxide nanoparticles (ZnONPs) are a type of nanoparticles that are widely present in several products such as building materials, wall paints, cosmetics, toothpaste, and sunscreens. In addition, they’re widely employed in the rubber industry, electronics, and food additives.Citation6,Citation7 The microbial selectivity, stability, and low cost of ZnONPs make them a possible alternative to silver nanoparticles (AgNPs) or antimicrobial peptides. Human exposure to ZnONPs occurs via several routes. They can reach the brain through oral intake or along the olfactory nerve. It has recently been evidenced that NPs can cross the blood–brain barrier (BBB) and induce oxidative stress, cytotoxicity, and inflammatory reactions.Citation8–12 Their toxic effects may produce alterations in synaptic plasticity leading to changes in spatial learning and memory abilities.Citation13,Citation14 The size, shape, and surface material of ZnONPs affect their cytotoxicity.Citation15,Citation16
We all can be frequently exposed to the hazardous effects of NPs therefore, searching for natural substances having the ability to counteract these drawbacks is essential. Quercetin (3,3′,4′,5,7- pentahydroxy-flavanone) is a flavonoid found in seeds, vegetables, fruits, coffee, tea, and bracken fern. It acts as an antioxidant with anticancer, anti-inflammatory, and antiviral properties.Citation17 In addition, previous studies also reported their antihypertensive, antibacterial, and antiprotozoal properties.Citation18,Citation19 Its potential to protect neurodegenerative,Citation20 cardiovascular,Citation21 and neoplastic disordersCitation22 has also gotten a lot of interest.
Studying in-depth the molecular basis underlying the effect of each of ZnONPs or Quercetin on the brain tissue is very essential to explain how they affected it. Non-coding RNAs (ncRNAs) were formerly assumed to be “junk” DNA but recently, they were found to have critical regulatory functions in several vital cellular pathways. They also impact cell physiological and pathological states. Micro RNA (miRNA) with 18–25 nucleotides, is a type of short noncoding RNA (ncRNA) with a length fewer than 200 nucleotides that has recently been implicated in gene regulation.Citation23 According to research, micro-RNA (miRNA) regulates gene expression via several ways as translational suppression, mRNA degradation, and binding to the 5′ UTR and other coding areas.Citation24 Other investigations, on the other hand, have shown that miRNA can stimulate transcription and translation.Citation25,Citation26
As a result, more research is required to fully comprehend and validate the functional connection. Considering the foregoing, we explored the potential harmful effects of ZnONPs on the molecular and histological structure of the rat cerebellum, as well as the many pathways driving Quercetin’s potential preventive role.
Chemicals
All chemicals were purchased from Sigma-Aldrich, 3050 Spruce Street, Saint Louis, MO 63103, USA
ZnONPs: In the form of dispersion of less than 100 nm particle size (≤40 nm average particle size), 20 wt.% in H2O. Product number 721077, CAS number 1314–13-2.Citation27
Quercetin: In the form of solid (CAS-No.: 117–39-5, ≥95% HPLC) that was dissolved in DMSO solution (Dimethyl sulfoxide, CAS-No.:67–68-5) to be administered orally.
Characterization of ZnONPs
The size and shape of ZnONPs were examined by transmission electron microscopy (TEM, JEOL JEM 1010; Jeol Ltd., Tokyo, Japan) at the Electron Microscope Unit, Faculty of Agriculture, Mansoura University, Mansoura, Egypt. Aqueous dispersion of nanoparticles was dropped onto a carbon coated copper grid, which was then dried and studied ().
Experimental animals
Forty adult Wistar male albino rats (5–7 months) weighing (200–250 gm.) were purchased from the Breeding Animal House at Faculty of Medicine, Zagazig University, Zagazig, Egypt. They were kept for acclimatization in stainless-steel cages at room temperature under standard laboratory settings. They were fed standard laboratory food and water ad libitum during the experiment. The experimental protocols were carried out according to the Institutional Animal Care and Use Committee’s guidelines, approved and accepted by the Faculty of Medicine, Zagazig University; Zagazig, Egypt.
Experimental design
The rats were divided into three groups:
Group I (control group): Included 20 rats that were divided into two equal subgroups:
Subgroup Ia (negative control): Ten rats received no treatment.
Subgroup Ib: Ten rats were daily administrated 50 mg/kg body weight orally of Quercetin dissolved in DMSO for 1 month.Citation28
Group II (ZnONPs-treated group): Ten rats were intraperitonially injected with 400 mg/kg b. wt. of ZnONPs, every other day for 1 month.Citation29
Group III (ZnONPs and Quercetin group): Ten rats were injected with ZnONPs at a similar dose and duration as group II and simultaneously administrated a daily dose of Quercetin dissolved in DMSO (50 mg/kg body weight), orally of for 1 month.
Blood collection and tissue preparation
Rats were anaesthetized intraperitoneally with 60 mg/kg body weight of pentobarbital. Blood was collected from the ophthalmic plexus then it was centrifuged at 2000 rpm for 20 minutes to obtain serum samples. The supernatant sera were then kept at −20°C. Biochemical assays were performed on serum samples that had been stored. Each animal’s cerebellar tissue was separated into two parts: one for histological evaluation and the other for biochemical and molecular analysis. 5–10 ml cold buffer (50 mM potassium phosphate, pH 7.4, 1 mM EDTA; Catalog Number G8789) per gramme tissue homogenized the latter. The homogenate was centrifuged at 4,000 rpm for 15 minutes at 4°C, and the supernatant was frozen at −80°C for analysis of oxidative stress markers.
Oxidative biomarkers assay
The lipid peroxidation (LPO) marker malondialdehyde (MDA) was evaluated calorimetrically in cerebellar homogenate using a (Biodiagnostic, Egypt; Catalog Number: MD 25 29) kit. Ohkawa et al.Citation30 developed a method that involves reacting thiobarbituric acid (TBA) with MDA in an acidic medium at 95°C for 30 minutes, yielding a TBA reactive pink product. This product’s absorbance can be measured at 534 nm. In addition, reactive oxygen species (ROS) levels were measured by ELISA kit (Catalog Number: MBS2802061), according to the manufacturer’s instructions (MyBioSource, USA).
Measurement of antioxidant biomarkers
The cerebellar homogenate’s total oxidative status (TOS) was estimated calorimetrically with a (Rel Assay Diagnostics kit, Turkey). The results were expressed in mol H2O2 equivalents per liter after the test was calibrated with hydrogen peroxide (H2O2). The Nishikimi et al. method was used to evaluate the activity of superoxide dismutase (SOD) in U/g cerebellar tissue.Citation31 The level of reduced glutathione (GSH) was tested using Beutler’s technique.Citation32 In addition, the activity of glutathione reductase (GR) was determined, as described by Erden & Bor.Citation33 Serum total antioxidant capacity (TAC) was measured according to Koracevic et al.Citation34 Bio diagnostic, Cairo, Egypt, developed all the above kits (Catalog Number: SD 25 21, Catalog Number: GR 25 11, Catalog Number: GR 25 23, Catalog Number: TA 25 13, respectively).
Measurement of serum cytokines concentrations
The serum levels of interleukin-1 beta (IL-1β), interleukin-6 (IL-6), interleukin-8 (IL-8), and tumor necrosis factor alpha (TNF-α) were determined using (ELISA) kits (MyBioSource, Inc.; USA) according to the manufacturer’s protocol (Catalog Number: MBS825017, Catalog Number: MBS269892, Catalog Number: MBS9141543, Catalog Number: MBS175904, respectively).
miRNA isolation and reverse transcription
Total miRNA was isolated from serum using (miRcut Tiangen biotech, China, Catalog Number: 4992860) kit according to the manufacturer’s instructions. The miRNA was then reverse-transcribed, and polyadenylated to complementary DNA (cDNA) by using (miRCURY LNA RT Kit, Qiagen GmbH, Germany, Catalog Number:339340) kit. The mixture was centrifuged and incubated at 37°C for 60 minutes. After mixing with template RNA, miScript Reverse Transcriptase mix, 5Χ miScript buffer, and RNase-free water in a final volume of 20 μl., samples were finally incubated at 95°C for 5 min and put on ice for inactivation of the reverse transcriptase enzyme.
Expression levels of miRNA-21-5p, miRNA-122-5p, miRNA-125b-5p, and miRNA-155-3p in serum were assessed
Real-time quantitative polymerase-chain reaction (RT-QPCR) with an applied biosystem real-time instrument was used to determine the expression levels miRNA-21-5p, miRNA-122-5p, miRNA-125b-5p, and miRNA-155-3p in serum. Primers were designed and purchased using miRCURY LNA miRNA PCR Assay (Qiagen GmbH, Germany) for amplification of hsa-miR-21-5p (MIMAT0000076), hsa-miR-122-5p (MIMAT0000421), hsa-miR-125b-5p (MIMAT0000423) and has-miR-155-3p (MIMAT0016993). We performed PCR in a 20 µ1 final volume including 2 µl 10X miScript universal reverse primer, 10 µl 2X Quanti-Tect SYBR-Green Polymerase Chain Reaction master mix, and 2 µl template cDNA after dissolving template cDNA and reagents. 2 μl 10 pmol forward specific stem-loop primer, and 4 μl RNase-free water. RT-PCR conditions: initial activation at 95°C for 15 minutes (one cycle), denaturation at 94°C for 40 cycles, annealing at 55°C for 30 seconds, and extension at 70°C for 30 seconds. In quantification investigations of miRNA utilizing the miScript PCR System,Citation35 we employed SNORD68 as a control for normalization of RT-PCR data. We compared the expression of miR-96-5p and miR-183-5p to that of the SNORD68 gene, and all data was standardized to that of the SNORD68 gene.Citation36 In every case, SNORD-68 was found to be highly expressed and stable.
Histopathological study
Hematoxylin and Eosin staining
Sagittal cerebellar specimens were fixed in 10% buffered formalin, dehydrated, embedded in paraffin wax, and cut into 5 µm-thick paraffin sections for light microscopic examination.Citation37
Silver staining
Deparaffinized sections were hydrated then, the slides were immersed in a 1.0% silver nitrate solution in a plastic Coplin jar before being rinsed with distilled water. In a flask, 1% warm silver nitrate was poured, and 28% ammonium hydroxide was added drop by drop with constant shaking until the first precipitate vanished and the solution became clear. 5% silver nitrate was then pipetted, with constant shaking, until the solution became slightly cloudy. The ammoniacal silver solution was poured into a plastic Coplin jar and the slides were placed in this solution and microwave at power level 3 (180 W) for 1 minute, then in 1% ammonium hydroxide solution for not more than 20 seconds. Developer was added to the ammoniacal silver solution for no more than 15 seconds, slides were immersed in a 1% ammonium hydroxide solution, rinsed three times with distilled water followed by 2% sodium thiosulfate solution for 30 seconds. The slides were rinsed three times in distilled water, 5 min each. Finally, they were dehydrated in graded alcohols, cleared in three or four changes of xylene, and mount with synthetic resin.Citation38
Immunohistochemical study
Avidin biotin complex technique (ABC Peroxidase Staining Kits, Code No. 32020, Thermo Scientific, Rockford, IL, USA) was utilized for immunohistochemical staining of the calcium binding protein; Calbindin, GFAP, and Bax protein. Paraffin-embedded specimens were deparaffinized in xylene and hydrated in distilled water. Antigen retrieval was done in the microwave for 15 minutes using citrate buffer. Bovine serum albumin was used for tissue block. Sections were then incubated overnight at 4°C with the following primary antibodies: Anti-CbD28k (rabbit polyclonal antibody; Cat. No. PA5-85669; dilution 1/500; Thermo Scientific, San Jose, CA, USA). Anti-GFAP (Cat. No. MS- 280- R7, Lab Vision Corporation, Fremont, USA) was diluted to1:100 in Lab Vision antibody diluent (Cat. TA- 125- UD). Finally, Bax mouse monoclonal antibody (CAT. MAS-14003, Lab Vision Corporation, Fremont, USA) was diluted to 1:200 in PBS (for 30 min at room temperature). Recognition was accomplished by secondary antibodies and labeled horseradish peroxidase followed by colorimetric detection by 3′, 3-diaminobenzidine (DAB). As a counterstain, Mayer’s Hematoxylin was utilized. As a substitute for the primary antibody, negative control slides were immersed in phosphate-buffered saline. The brown color indicated the antigen location under light microscopy.Citation39
Histomorphometric study and statistical analysis
The Pathology Department, Faculty of Dentistry, Cairo University, Cairo, Egypt, used a Leica Qwin 500 (Leica Imaging system, Ltd., Cambridge, England) image analyzer computer system to perform a morphometric study of the area percentage of calbindin-positive cells, GFAP, and BAX protein immunoexpression in the studied groups. Data analysis by Leica QWin 500 software and a digital camera connected to an optical microscope (Olympus, Tokyo, Japan). Ten non-overlapping fields were randomly selected from each rat in each group, and the means of the parameter measurements in each section were recorded for each animal. The Statistical Package for Social Sciences (SPSS) version 22.0 was used to analyze the data (IBM Corp., Armonk, NY, USA). One way analysis of variance (ANOVA) was used to express the data as means ± standard deviations, followed by Tukey’s Honestly Significant Difference (Tukey’s HSD) test as a post hoc test. The probability (P) values less than 0.05 were considered significant, while P values less than 0.001 were considered highly significant.
Results
Oxidative stress biomarkers concentrations (
No statistically significant differences were detected between the control subgroups regarding levels of the studied parameters.
Significant variations were detected in MDA and TOS in cerebellar homogenate between the three groups (P < .001 for each). Group II showed significantly higher levels of cerebellar MDA and TOS while Quercetin supplementation with ZnONPs (group III), significantly decreased their levels.
On the other side, we detected significant differences of antioxidant parameters (SOD & GSH) in cerebellar homogenate, and TAC in serum between the three groups (P = .004, P < .001, P < .001, respectively). ZnONPs decreased the antioxidant parameters which were improved by Quercetin treatment.
No significant differences were detected regarding GR activity between the study groups (P = .18).
Table 1. Oxidative stress parameters in the study groups (Mean ±SD).
Serum inflammatory cytokines levels in different study groups ()
No significant changes were detected in serum cytokines levels between both control subgroups.
Significant differences in IL-1, IL-6, IL-8, and TNF-α levels were detected between the study groups (P < .001).
Significantly higher serum concentrations of inflammatory cytokines levels in ZnONPs group (group II) compared to the control group.
Quercetin supplementation with ZnONPs (group III), improved the inflammatory status through decreasing serum cytokines levels but still significantly higher than the control subgroups, suggesting potential anti-inflammatory effect.
Table 2. Serum inflammatory cytokines in the study groups (Mean ±SD).
Serum miRNAs expression in different study groups ()
miR-21-5p expression value was significantly higher in group II compared to control subgroups and Quercetin treated group (III). However, we did not find significant changes between groups I and III.
miR-122-5p and miR-155-p expression levels were significantly increased in ZnONPs exposed group as compared to control subgroups or Quercetin treatment group (III).
ZnONPs group also showed significantly higher miR-125b-5p expression levels than the control subgroups while, Quercetin treatment (group III) showed no significant changes in comparison to control subgroups.
Table 3. Serum miR-21-5p, miR-122-5p, miR-125b-5p, and miR-155-p expression levels in the study groups (Mean ± SD).
Histological results
H&E-stained cerebellar sections of the control group showed the three layers of cerebellar cortex; the molecular layer, Purkinje cell layer, and the granular layer. The molecular layer showed small, scattered stellate and basket cells. Purkinje cell layer was formed of pyriform-shaped Purkinje cells with vesicular nuclei, prominent nucleoli, and basophilic cytoplasm. The granular layer composed of closely packed small, rounded granule cells with darkly stained nuclei. Among these cells, cerebellar islands or glomeruli were found. ZnONPs exposure for one month, markedly distorted the cerebellar tissue structure as the molecular layer showed cells with small dark nuclei, and vacuolated neuropil. Purkinje cells were shrunken, irregular in shape and deeply stained. Peri-cellular halos were observed around Purkinje cells and granule cells. Quercetin supplementation with ZnONPs, ameliorated the cerebellar histological structure in the form of normal appearance of the cerebellar layers while some cells showed peri-cellular halo ().
Figure 2. H&E-stained sections of rat cerebellar cortex showing: (a) the control groupshows cerebellar cortex formed of three layers; the molecular layer (ML) shows small, scattered stellate (sc), larger basket cells (bc) and blood vessels (bv). Purkinje cell layer (PL) is formed of pyriform-shaped Purkinje cells (p) with vesicular nuclei, prominent nucleoli, and basophilic cytoplasm. The granular layer (GL) shows tightly packed small, rounded granule cells (arrow) with deeply stained nuclei. Among these cells, cerebellar islands, or glomerulus (asterisks) are located. (b) In group II, the molecular layer (ML) shows cells with small dark nuclei, and vacuolated neuropil (v). Purkinje cells are shrunken, irregular shaped and deeply stained (arrow). Pericellular halos (curved arrows) are observed around Purkinje cells and granule cells. (c) Group III shows normal shaped molecular layer (ML) containing stellate cells (Sc), basket cells (bc) and blood vessels (bv). Some cells show pericellular halo (curved arrow). Purkinje cells (P) are pyriform in shape. They had vesicular nuclei with prominent nucleoli and basophilic cytoplasm. Granule cells (arrowhead) are tightly packed with darkly stained nuclei. Cerebellar islands or glomerulus (asterisks) are located among granule cells (H&E × 400; Scale bar = 30 µm).
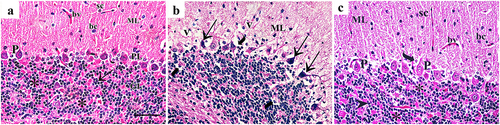
Silver impregnation of cerebellar sections showed many polymorphic neuronal cells in the molecular layer of control group in the form of outer stellate cells and inner basket cells. The Purkinje cells were large, pyriform shaped with vesicular nuclei. The granular layer contained plenty of granule cells arranged in rosettes or cords. ZnONPs exposure showed neuronal degeneration in the three layers in the form of marked decreased cellularity in molecular layer, darkly stained Purkinje cells with abnormal shapes, and vacuolated neuropil. The granular layer showed peri-neuronal spaces around dark stained granule cells. Quercetin supplementation with ZnONPs showed normal appearance of the three cerebellar layers ().
Figure 3. Silver impregnation of cerebellar sections showing (a): the control group shows more polymorphic neuronal cells in the molecular layer (ML) in the form of outer stellate cells (Sc) and inner basket cells (Bc). The Purkinje cells (arrow) are large, pyriform shaped with vesicular nuclei. The granular layer (GL) contains plenty of granule cells arranged in rosettes or cords (circles). (b): Group II shows marked decreased cellularity in molecular layer (ML), Purkinje cells are darkly stained with abnormal shape (arrow), and vacuolated neuropil (asterisks). The granular layer (GL) shows peri-neuronal spaces (curved arrow) around dark stained granule cells. (c): Group III shows normal shaped molecular layer (ML) containing stellate cells (Sc) and basket cells (Bc). The Purkinje cells (arrow) are pyriform shaped with vesicular nuclei. The granular layer (GL) contains plenty of granule cells arranged in rosettes (circles) (Silver impregnation×400; Scale bar = 30 µm).
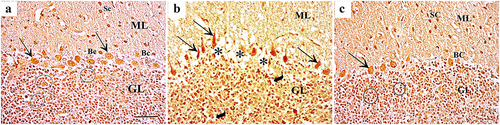
Calbindin D28k immunostaining showed intense cytoplasmic immunoreaction in many Purkinje cells and their processes in control group and Quercetin-treated group (III) while decreased intensity of immunoreaction was observed in few irregular-shaped Purkinje cells of group II. ().
Figure 4. Immunohistochemically stained cerebellar sections for Calbindin D28k showing (a) Intense cytoplasmic immunoreaction in many Purkinje cells (arrow) and their processes (red arrow) in control group. (b): Decreased intensity of immunoreactions in few Purkinje cells of group II (arrow). (c): Positive cytoplasmic immune reaction in Purkinje cells (arrow) and their processes (red arrow) of group III (Anti calbindin D28k ×400; Scale bar = 30 µm).
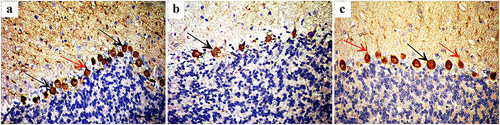
Bax protein immunostaining revealed negative expression in the control group, high expression in the three layers of cerebellar cortex of group II, and weak expression in Purkinje cells of group III. ().
Figure 5. Immunohistochemically stained cerebellar sections for Bax protein showing (a): Negative expression in control group (arrow). (b): Intense expression in Purkinje cells and basket cells (arrow) and some granule cells (red arrow) of group II, while (c): shows weak expression in Purkinje cells of group III (arrow). (Anti Bax×400; Scale bar = 30 µm).
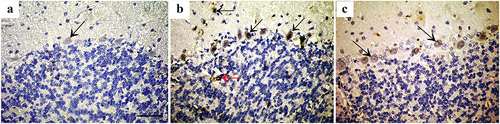
GFAP immunostaining showed positive expression in few astrocytes of control group and Quercetin-treated group (III) while intense expression was observed in cytoplasm and processes of astrocytes in all layers of group II. ().
Figure 6. Immunohistochemically stained cerebellar sections for GFAP showing (a): positive expression in astrocytes of control group. (b): intense expression in cytoplasm and processes of astrocytes in all layers of group II. (c): positive expression in astrocytes of group III (arrows indicate immunoexpression for GFAP) (Anti GFAP ×400; Scale bar = 30 µm).
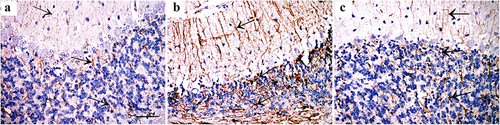
Morphometric and statistical results ()
Highly significant (P < .00001) decrease in the main area % of Calbindin D28k was observed in ZnONPs-exposed rats compared to all groups while, it showed highly significant (P < .00001) increase in Quercetin-treated rats (group III) relative to group II rats.
Highly significant (P < .00001) increase in the mean area % of BAX, and GFAP immunoexpression was observed in ZnONPs-exposed rats compared to all groups while Quercetin-treated rats (group III), showed highly significant (P < .00001) decrease in their levels in comparison to group II.
Non-significant difference was noted between the control subgroups regarding the three measured proteins.
Table 4. Area % of Calbindin D28k, BAX, and GFAP immunoexpression levels in the study groups (Mean ±SD).
Discussion
ZnONPs are one of the most utilized engineered nanomaterials so, exposure to their harmful effects is inevitable.Citation40 Neurotoxic potential of ZnONPs has not been explored in detail so; we aimed to investigate different cellular and molecular mechanisms underlying the neurotoxic effect of ZnONPs and the ameliorative effect of Quercetin.
The present research revealed that oxidative stress caused by ZnONPs can be reversed by Quercetin administered simultaneously with ZnONPs. It could be explained as ZnONPs interfere with cell zinc homeostasis, producing an excess of reactive oxygen species (ROS) that deplete intracellular antioxidants. ZnONPs also induce mitochondrial dysfunction leading to inflammation.Citation41,Citation42 In addition, endothelial cells could internalize ZnONPs of 20–70 nm in diameter, causing inflammatory reaction due to the accumulation of the particles rather than ZnO-released Zn2 + .Citation43 Qiao et al.Citation44 reported that ZnONPs induced significant increases in the serum levels of IL-8, IL-1β, and TNF-α shedding the light on the proinflammatory effect of ZnONPs. IL-1β is a strong pro-inflammatory mediator and is crucial for inflammation induced by nanoparticles.Citation45 In contrast, Abdulmalek et al.Citation46 reported decreased IL-1β, and TNF-α expression values accompanied by increased GSH and TAC in ZnONPs exposed rats as compared to healthy rats, proposing anti-inflammatory and antioxidant effect of ZnONPs. Also, a previous study showed similar anti-inflammatory effects of ZnONPs on downregulating the expression of iNOS, IL-1β, and TNF-α in the cell.Citation47 This discrepancy in results could be attributed to changes in doses and duration of exposure to the nanoparticles.
Much prior research suggested that Quercetin may possess antioxidant and anti-inflammatory properties due to its free radical scavenging and metal-chelating capacities.Citation48–50 The anti-inflammatory/anti-allergic property of Quercetin was attributed to mast cell membranes stabilization so, preventing the release of histamine and other inflammatory mediators in the body. It was shown to reduce IL-6 and TNFα in lipopolysaccharide in murine macrophages.Citation51 Quercetin also prevents glial cells from releasing proinflammatory cytokines. It protects against neuroinflammation by decreasing the formation of nitric oxide (NO) in microglial cells, which inhibits NF-B signaling.Citation52–55 Similarly, Quercetin has been shown to reduce manganese neurotoxicity via reducing neuroinflammation-mediated neurodegeneration, by regulating the heme oxygenase-1 (HO-1)/nuclear factor erythroid 2-related factor 2 (Nrf2) and nuclear NF-B pathways.Citation56
According to Wang et al.,Citation57 Quercetin administration has alleviated cerebral I/R-induced neurological impairments, brain infarction, disruption of the blood–brain barrier, oxidative stress, TNF- and IL-1 mRNA expression, as well as apoptotic caspase3. Additionally, their findings showed that Quercetin reduced the rise in protein tyrosine and serine/threonine phosphatase activity, as well as the decline in extracellular signal-regulated kinase and Akt phosphorylation, all of which may be crucial in the progression of brain injury following cerebral ischemia/reperfusion.
In the same context, we proved significant differences between the three groups of the present study regarding serum miR-21-5p, miR-122-5p, miR-125b-5p, and miR-155-3p expression levels (P < .001 for each). Recent research showed that miRNAs are important regulators of inflammation. miRNAs can influence a variety of biological processes by modulating the expression of several target genes.Citation58 miRNAs are one of the primary epigenetic mechanisms that have been linked to toxicity through altering gene expression post-transcriptionally, according to.Citation59 Differential expression of miRNAs has been observed in neurodegenerative illnesses such as Alzheimer’s disease, Parkinson’s disease, and schizophrenia. Environmental neurotoxicants, such as metals, have been connected to brain miRNA expression and function.Citation60 These genes are involved in apoptosis, vesicle-mediated transport, oxidative stress, chemokine-mediated signaling, IL-1 signaling, inflammation, and autophagy according to Qiao et al.Citation44 Our findings could be explained as miR-155 has been implicated in NF-κB and other inflammation-based signaling pathways.Citation61,Citation62 Furthermore, abnormal miR-155 production and function have been linked to inflammation and have been shown to influence immune cell functioning at several levels by targeting inflammation-related genes, such as TLRs.Citation63
Hu et al.Citation64 discovered six significant miRNAs in response to different NPs. They involve MiR-124, miR-144, miR-148, miR-155, miR-19a, and miR-223. These miRNAs have already been validated in mammalian and zebrafish miRNA profiling investigations, and their predicted targets have been discovered to interact with a variety of signaling pathways. The accumulation of NPs in the environment is unregulated, which is a rising source of worry. It worth noting that, miR-21 is well-known as oncogenic and an unfavorable prognostic factor,Citation65 and increased miR-21 expression was reported in mouse hippocampal neurons associated with neurotoxicity.Citation66 Many studies evaluated miR-21 expression, and Quercetin treatment downregulated its expression regardless the tested model was in vitro or in vivo according to Dostal & Modriansky.Citation67 In addition, Boesch-Saadatmandi et al.Citation68 assumed the mechanism of Quercetin-induced miR-155 regulation to direct and indirect modulation through NFκB. In agreement with our results, the study of Garelnabi and Mahini,Citation69 proved that Quercetin alone caused non-significant downregulation of miR-125b expression in the liver but when administered in combination with exercise, it attenuated the exercise induced upregulation of miR-125b.
The histopathological changes in the present study represented the consequence of the aforementioned molecular results. Both H&E and silver staining revealed the toxic effects of ZnONPs on the three cortical layers that were improved with Quercetin supplementation. Muller et al.Citation70 explained this damaging effect by release of ionic Zn2+ from ZnONPs due to intracellular dissolution. Vacuolated neuropils might result from degeneration and shrinkage of Purkinje cells with retraction of their processes leaving empty spaces according to Garman.Citation71 These findings can be also attributed to apoptotic and oxidative stress pathways which are responsible for the accumulation of damaged molecules (proteins, nucleic acids, and lipids).Citation72 Vacuolation, on the other hand, was described by Eluwa et al.Citation73 as a form of cellular defense mechanism against neurotoxins in the cerebellar cortex. In the same context, the fall in the number of granule cells with ZnONPs, could be linked to oxidative and metabolic stress. Moreover, Purkinje cells are synaptically dependent on granule cells; hence, the loss of Purkinje cells may result in granule cell death.Citation74
Quercetin supplementation in group III showed nearly normal appearance of cerebellar tissue apart from some peri-cellular halos. Accordingly, the antioxidant and anti-inflammatory effects of Quercetin that were proved in our study, were reflected on the histological structure of the rat cerebellar cortex where most of Purkinje cells retained their normal characters. These results were in line with Fereidounni and DhawanCitation75 and Haq and Al AmroCitation76 who proved a remarkable protective effect of Quercetin on the overall morphology and viability of neuronal cells.
Neuronal degeneration may result from mitochondrial fragmentation. However, Quercetin can increase the expression of H2AX gene and mitochondrial transcription factor A to minimize the damages in the mitochondrial structure and activity, while also reducing axonal and neuronal damage.Citation77 Also, Quercetin can reduce the damage of myelin sheath of white matter after cerebral hypoperfusion by increasing the secretion of IL-10 by M2-like microglia.Citation78 Quercetin decreases the apoptosis of cortical neurons, improves degenerative lesions of the central nervous system, and has a neuroprotective effect in Alzheimer’s disease.Citation79 Additionally, Quercetin lessens the gray and white matter damage following spinal cord injury (SCI), which may suggest its potential use in the treatment of SCI.Citation80
Calbindin D28k (CB) is a calcium-binding protein that helps to maintain calcium balance. It inhibits various pro-apoptotic pathways so, prevents neuronal death.Citation81 CB is an antioxidant that protects against oxidative stress and harmful substances.Citation82 Its levels drop dramatically in degenerative neuronal disorders.Citation83 In our investigation, exposure to ZnONPs reduced the strength of CB immunoexpression in Purkinje cells. The buffering ability of neurons is reduced when CB is depleted, resulting in an increase in intracellular and intranuclear calcium.Citation84
From all mentioned above, we can deduce enhancement of cerebellar neuronal cells apoptosis which was evident by immunohistochemical detection of Bax protein as intense expression was found in Purkinje cells and basket cells of group II, while weak expression was observed in Purkinje cells of group III. The genes Bax and Bcl-2 control the intrinsic apoptotic pathway, and ZnONPs significantly raises Bax mRNA expression and protein levels, as well as the Bax/Bcl-2 ratio.Citation85,Citation86
Every chemical, mechanical, or degenerative input to the brain causes astrocyte proliferation and hypertrophy, which lead to increased GFAP production and astrogliosis, according to Sofroniew and Vinters.Citation87 The molecular mechanism of astrocyte activation has been proposed as oxidative stress, which is a result of the increased need for neural protection.Citation88 So, antioxidants administration could play a crucial protective role against astrogliosis. This explains the significant increase in GFAP immunoexpression in ZnONPs-exposed rats of the present study and reversal after Quercetin supplementation. GFAP is an intermediate filament protein known to be specifically expressed in astrocytes; the glial cells that are responsible for repairing and scarring of the brain following injuries.Citation89 The increase in GFAP expression has been identified as a biomarker of neurotoxicity.Citation90 In the CNS, Quercetin reduced the activation of astrocytes and reduced neuroinflammation.Citation91 The blood-brain barrier is crucial in relation to the toxicity of the nanoparticles in the brain tissue. This expanded membrane between the cerebral capillaries and surrounding endothelial cells contains tight junctions.Citation92,Citation93 When nanoparticles enter the circulation, they may change the permeability of the membrane or cause a cascade of chemicals that damage tight junctions, causing direct or indirect toxicity in the brain tissues. NPs may also stimulate the vesicular transport, to get entry inside the microenvironment of CNS where they further disrupt several molecular pathways.Citation94,Citation95
Conclusion
The current study’s findings supported the idea of integrating epigenetic testing during the toxicological evaluation of nanomaterials’ biological interactions. We have shown for the first time that frequently used NPs cause alterations in serum miRNA expression. In addition, these NPs induced changes in oxidative stress parameters and inflammatory cytokines levels which resulted in observable alteration in cerebellar structure with associated apoptosis, gliosis and diminished neuronal Calbindin level. To completely understand the association between observed changes in miRNA expression and NP effects at the cellular level, more research is required. In addition, we also clarified the different mechanisms underlying the neuroprotective effect of Quercetin against the oxidative stress and neuronal injury induced by ZnONPs administration.
Authorship contribution statement
Abdelrahman SA, El-Shal AS, Abdelrahman AA, Saleh EZH, and Mahmoud AA: designed the research and manuscript writing. Abdelrahman SA, Saleh EZH and Mahmoud AA: performed the practical part of histological examination with analysis of data. El-Shal AS and Abdelrahman AA: performed the practical part of biochemical examination and interpretation of data.
Consent for Publication
All authors have read and approved the final version of the manuscript.
Disclosure statement
No potential conflict of interest was reported by the author(s).
Additional information
Funding
References
- Gonzalez-Estrella J, Puyol D, Sierra-Alvarez R, Field JA. Role of biogenic sulfide in attenuating zinc oxide and copper nanoparticle toxicity to acetoclastic methanogenesis. J Hazard Mater. 2015;283:265–280. doi:10.1016/j.jhazmat.2014.10.030.
- Ndukwu MC, Ikechukwu-Edeh CE, Nwakuba NR, Okosa I, Horsefall IT, Orji FN. Nanomaterials application in greenhouse structures, crop processing machinery, packaging materials and agro-biomass conversion. Mater Sci Technol. 2020;3:690–699. doi:10.1016/j.mset.2020.07.006.
- Magaye R, Zhao J, Bowman L, Ding M. Genotoxicity and carcinogenicity of cobalt-, nickel-and copper-based nanoparticles. Exp Ther Med. 2012;4:551–561.
- Robertson TA, Sanchez WY, Roberts MS. Are commercially available nanoparticles safe when applied to the skin? J Biomed Nanotechnol. 2010;6(5):452–468. doi:10.1166/jbn.2010.1145.
- Reidy B, Haase A, Luch A, Dawson KA, Lynch I. Mechanisms of silver nanoparticle release, transformation and toxicity: a critical review of current knowledge and recommendations for future studies and applications. Materials. 2013;6(6):2295–2350. doi:10.3390/ma6062295.
- Wiking L, Larsen T, Sehested J. Transfer of dietary zinc and fat to milk—Evaluation of milk fat quality, milk fat precursors, and mastitis indicators. J Dairy Sci. 2008;91(4):1544–1551. doi:10.3168/jds.2007-0716.
- Ibrahim RK, Hayyan M, AlSaadi MA, Hayyan A, Ibrahim S. Environmental application of nanotechnology: air, soil, and water. Environ Sci Pollut Res. 2016;23(14):13754–13788. doi:10.1007/s11356-016-6457-z.
- Mallevre F, Fernandes TF, Aspray TJ. Silver, zinc oxide and titanium dioxide nanoparticle ecotoxicity to bioluminescent Pseudomonas putida in laboratory medium and artificial wastewater. Environ Pollut. 2014;195:218–225. doi:10.1016/j.envpol.2014.09.002.
- Yang X, Jiang MZ. Research progress on biological toxicity of zinc oxide nanoparticle and its mechanism. Zhejiang da xue xue bao. J Zhejiang Univ Med Sci. 2014;43:218–226.
- Žūkienė R, Snitka V. Zinc oxide nanoparticle and bovine serum albumin interaction and nanoparticles influence on cytotoxicity in vitro. Colloids Surf B Biointerfaces. 2015;135:316–323. doi:10.1016/j.colsurfb.2015.07.054.
- Kao YY, Chen YC, Cheng TJ, Chiung YM, Liu PS. Zinc oxide nanoparticles interfere with zinc ion homeostasis to cause cytotoxicity. Toxicol. Sci. 2012;125(2):462–472. doi:10.1093/toxsci/kfr319.
- Lee CM, Jeong HJ, Kim DW, Sohn MH, Lim ST. The effect of fluorination of zinc oxide nanoparticles on evaluation of their biodistribution after oral administration. Nanotechnology. 2012;23(20):205102. doi:10.1088/0957-4484/23/20/205102.
- Han D, Tian Y, Zhang T, Ren G, Yang Z. Nano-zinc oxide damages spatial cognition capability via over-enhanced long-term potentiation in hippocampus of Wistar rats. Int J Nanomedicine. 2011;6:1453. doi:10.2147/IJN.S18507.
- Shim KH, Hulme J, Maeng EH, Kim MK, An SSA. Analysis of zinc oxide nanoparticles binding proteins in rat blood and brain homogenate. Int J Nanomedicine. 2014;9(Suppl 2):217. doi:10.2147/IJN.S58204.
- Guo D, Wu C, Jiang H, Li Q, Wang X, Chen B. Synergistic cytotoxic effect of different sized ZnO nanoparticles and daunorubicin against leukemia cancer cells under UV irradiation. J Photochem Photobiol B. 2008;93(3):119–126. doi:10.1016/j.jphotobiol.2008.07.009.
- Pujalté I, Passagne I, Brouillaud B, Tréguer M, Durand E, Ohayon-Courtès C, l’Azou B. Cytotoxicity and oxidative stress induced by different metallic nanoparticles on human kidney cells. Part Fibre Toxicol. 2011;8(1):1–16. doi:10.1186/1743-8977-8-10.
- Zeedan GSG, EL-Razik K, Allam AM, Abdalhamed AM, Zeina HAA. Evaluations of potential antiviral effects of green zinc oxide and silver nanoparticles against bovine herpesvirus 1. Adv Anim Vet Sci. 2020;8(4):433–443. doi:10.17582/journal.aavs/2020/8.4.433.443.
- Dias AS, Porawski M, Alonso M, Marroni N, Collado PS, Gonzalez-Gallego J. Quercetin decreases oxidative stress, NF-κB activation, and iNOS overexpression in liver of streptozotocin-induced diabetic rats. J Nutr. 2005;135(10):2299–2304. doi:10.1093/jn/135.10.2299.
- Chan ST, Chuang CH, Yeh CL, Liao JW, Liu KL, Tseng MJ, Yeh SL. Quercetin supplementation suppresses the secretion of pro-inflammatory cytokines in the lungs of Mongolian gerbils and in A549 cells exposed to benzo [a] pyrene alone or in combination with β-carotene: in vivo and ex vivo studies. J Nutr Biochem. 2012;23(2):179–185. doi:10.1016/j.jnutbio.2010.11.014.
- Youdim KA, Shukitt-Hale B, Joseph JA. Flavonoids and the brain: interactions at the blood–brain barrier and their physiological effects on the central nervous system. Free Radic Biol Med. 2004;37(11):1683–1693. doi:10.1016/j.freeradbiomed.2004.08.002.
- Kamada C, da Silva EL, Ohnishi-Kameyama M, Moon JH, Terao J. Attenuation of lipid peroxidation and hyperlipidemia by quercetin glucoside in the aorta of high cholesterol-fed rabbit. Free Radic Res. 2005;39(2):185–194. doi:10.1080/10715760400019638.
- Murakami A, Ashida H, Terao J. Multitargeted cancer prevention by quercetin. Cancer Lett. 2008;269(2):315–325. doi:10.1016/j.canlet.2008.03.046.
- O’Brien J, Hayder H, Zayed Y, Peng C. Overview of microRNA biogenesis, mechanisms of actions, and circulation. Front Endocrinol (Lausanne). 2018;9:402. doi:10.3389/fendo.2018.00402.
- Zhang J, Zhou W, Liu Y, Liu T, Li C, Wang L. Oncogenic role of microRNA-532‑5p in human colorectal cancer via targeting of the 5ʹUTR of RUNX3. Oncol Lett. 2018;15(5):7215–7220. doi:10.3892/ol.2018.8217.
- Truesdell SS, Mortensen RD, Seo M, Schroeder JC, Lee JH, LeTonqueze O, Vasudevan S. MicroRNA-mediated mRNA translation activation in quiescent cells and oocytes involves recruitment of a nuclear microRNP. Sci Rep. 2012;2(1):1–12. doi:10.1038/srep00842.
- Dharap A, Pokrzywa C, Murali S, Pandi G, Vemuganti R. MicroRNA miR-324-3p induces promoter-mediated expression of RelA gene. PLoS one. 2013;8(11):e79467. doi:10.1371/journal.pone.0079467.
- Mohamed DA, Abdelrahman SA. The possible protective role of zinc oxide nanoparticles (ZnONPs) on testicular and epididymal structure and sperm parameters in nicotine-treated adult rats (a histological and biochemical study). Cell Tissue Res. 2019;375(2):543–558. doi:10.1007/s00441-018-2909-8.
- Renugadevi J, Prabu SM. Quercetin protects against oxidative stress-related renal dysfunction by cadmium in rats. Exp Toxicol Pathol. 2010;62(5):471–481. doi:10.1016/j.etp.2009.06.006.
- Somayeh B, Mohammad F. Vitamin C can reduce toxic effects of Nano Zinc Oxide. Int J Biological Sci. 2014;3:65–70.
- Ohkawa H, Ohishi N, Yagi K. Assay for lipid peroxides in animal tissues by thiobarbituric acid reaction. Anal Biochem. 1979;95(2):351–358. doi:10.1016/0003-2697(79)90738-3.
- Nishikimi M, Rao NA, Yagi K. The occurrence of superoxide anion in the reaction of reduced phenazine methosulfate and molecular oxygen. Biochem Biophys Res Commun. 1972;46(2):849–854. doi:10.1016/S0006-291X(72)80218-3.
- Beutler E, Duron O, Kelly BM. Improved method for the determination of blood glutathione. J Lab Clin Med. 1963;61:882–888.
- Erden M, Bor NM. Changes of reduced glutathion, glutathion reductase, and glutathione peroxidase after radiation in Guinea pigs. Biochem Med. 1984;31(2):217–227. doi:10.1016/0006-2944(84)90026-7.
- Koracevic D, Koracevic G, Djordjevic V, Andrejevic S, Cosic V. Method for the measurement of antioxidant activity in human fluids. J Clin Pathol. 2001;54(5):356–361. doi:10.1136/jcp.54.5.356.
- Marabita F, De Candia P, Torri A, Tegner J, Abrignani S, Rossi RL. Normalization of circulating microRNA expression data obtained by quantitative real-time RT-PCR. Brief Bioinform. 2016;17(2):204–212. doi:10.1093/bib/bbv056.
- Li H, Xie S, Liu M, Chen Z, Liu X, Wang L, Zhou Y. The clinical significance of downregulation of mir-124-3p, mir-146a-5p, mir-155-5p and mir-335-5p in gastric cancer tumorigenesis. Int J Oncol. 2014;45(1):197–208. doi:10.3892/ijo.2014.2415.
- Bancroft JD, Gamble M, Eds. Theory and practice of histological techniques. 6th ed. London (UK): Churchill Livingstone; 2008.
- Suvarna KS, Layton C, Bancroft JD, Eds. Bancroft’s theory and practice of histological techniques E-book. Elsevier Health Sciences; 2018. ISBN: 978-0-7020-6864-5. doi:10.1016/B978-0-7020-6864-5.12001-8.
- Ramos-Vara JA, Kiupel M, Baszler T, Bliven L, Brodersen B, Chelack B, Valli VE, Czub S, Del Piero F, Dial S. Suggested guidelines for immunohistochemical techniques in veterinary diagnostic laboratories. J Vet Diagn Investig. 2008;20(4):393–413. doi:10.1177/104063870802000401.
- Srivastava AK, Yadav SS, Mishra S, Yadav SK, Parmar D, Yadav S. A combined microRNA and proteome profiling to investigate the effect of ZnO nanoparticles on neuronal cells. Nanotoxicology. 2020;14(6):757–773. doi:10.1080/17435390.2020.1759726.
- Chevallet M, Gallet B, Fuchs A, Jouneau PH, Um K, Mintz E, Michaud-Soret I. Metal homeostasis disruption and mitochondrial dysfunction in hepatocytes exposed to sub-toxic doses of zinc oxide nanoparticles. Nanoscale. 2016;8(43):18495–18506. doi:10.1039/C6NR05306H.
- Abdel-Daim MM, Eissa IA, Abdeen A, Abdel-Latif HM, Ismail M, Dawood MA, Hassan AM. Lycopene and resveratrol ameliorate zinc oxide nanoparticles-induced oxidative stress in Nile tilapia, Oreochromis niloticus. Environ Toxicol Pharmacol. 2019;69:44–50. doi:10.1016/j.etap.2019.03.016.
- Sun J, Wang S, Zhao D, Hun FH, Weng L, Liu H. Cytotoxicity, permeability, and inflammation of metal oxide nanoparticles in human cardiac microvascular endothelial cells: cytotoxicity, permeability, and inflammation of metal oxide nanoparticles. Cell Biol Toxicol. 2011;27(5):333–342. doi:10.1007/s10565-011-9191-9.
- Qiao Y, Liang X, Yan Y, Lu Y, Zhang D, Yao W, Yan Z, Yan Z. Identification of exosomal miRNAs in rats with pulmonary neutrophilic inflammation induced by zinc oxide nanoparticles. Front Physiol. 2018;9:217. doi:10.3389/fphys.2018.00217.
- Tsugita M, Morimoto N, Nakayama M. SiO 2 and TiO 2 nanoparticles synergistically trigger macrophage inflammatory responses. Part Fibre Toxicol. 2017;14(1):1–9. doi:10.1186/s12989-017-0192-6.
- Abdulmalek S, Eldala A, Awad D, Balbaa M. Ameliorative effect of curcumin and zinc oxide nanoparticles on multiple mechanisms in obese rats with induced type 2 diabetes. Sci Rep. 2021;11(1):1–22. doi:10.1038/s41598-021-00108-w.
- Nagajyothi PC, Cha SJ, Yang IJ, Sreekanth TVM, Kim KJ, Shin HM. Antioxidant and anti-inflammatory activities of zinc oxide nanoparticles synthesized using Polygala tenuifolia root extract. J Photochem Photobiol B. 2015;146:10–17. doi:10.1016/j.jphotobiol.2015.02.008.
- Naderi GA, Asgary S, Sarraf-Zadegan GN, Shirvany H. Antioxidant effect of flavonoids on the susceptibility of LDL oxidation. In: Vascular Biochemistry. Boston (MA): Springer; 2003. p. 193–196.
- Davis JM, Murphy EA, Carmichael MD, Davis B. Quercetin increases brain and muscle mitochondrial biogenesis and exercise tolerance. Am J Physiol Regul Integr Comp Physiol. 2009;296(4):R1071–R1077. doi:10.1152/ajpregu.90925.2008.
- Kang JT, Moon JH, Choi JY, Park SJ, Kim SJ, Saadeldin IM, Lee BC. Effect of antioxidant flavonoids (quercetin and taxifolin) on in vitro maturation of porcine oocytes. Asian-australas J Anim Sci. 2016;29(3):352. doi:10.5713/ajas.15.0341.
- Jung WJ, Sung MK. Effects of major dietary antioxidants on inflammatory markers of RAW 264.7 macrophages. Biofactors. 2004;21(1–4):113–117. doi:10.1002/biof.552210122.
- Rao YK, Fang SH, Tzeng YM. Inhibitory effects of the flavonoids isolated from Waltheria indica on the production of NO, TNF-α and IL-12 in activated macrophages. Biol Pharm Bull. 2005;28(5):912–915. doi:10.1248/bpb.28.912.
- Kao TK, Ou YC, Raung SL, Lai CY, Liao SL, Chen CJ. Inhibition of nitric oxide production by quercetin in endotoxin/cytokine-stimulated microglia. Life Sci. 2010;86(9–10):315–321. doi:10.1016/j.lfs.2009.12.014.
- Liao YR, Lin JY. Quercetin intraperitoneal administration ameliorates lipopolysaccharide-induced systemic inflammation in mice. Life Sci. 2015;137:89–97. doi:10.1016/j.lfs.2015.07.015.
- Chen WW, Zhang XIA, Huang WJ. Role of neuroinflammation in neurodegenerative diseases. Mol Med Rep. 2016;13(4):3391–3396. doi:10.3892/mmr.2016.4948.
- Bahar E, Kim JY, Yoon H. Quercetin attenuates manganese-induced neuroinflammation by alleviating oxidative stress through regulation of apoptosis, iNOS/NF-κB and HO-1/Nrf2 pathways. Int J Mol Sci. 2017;18(9):1989. doi:10.3390/ijms18091989.
- Wang YY, Chang CY, Lin SY, Wang JD, Wu CC, Chen WY, Kuan YH, Liao SL, Wang WY, Chen CJ. Quercetin protects against cerebral ischemia/reperfusion and oxygen glucose deprivation/reoxygenation neurotoxicity. J Nutr Biochem. 2020;83:108436. doi:10.1016/j.jnutbio.2020.108436.
- Sun S, Xuan F, Ge X, Zhu J, Zhang W, Tamada K, Takumi T, Hashimoto R, Otani H, Pazour GJ. Dynamic mRNA and miRNA expression analysis in response to hypoxia and reoxygenation in the blunt snout bream (Megalobrama amblycephala). Sci Rep. 2017;7(1):1–11. doi:10.1038/s41598-016-0028-x.
- Balasubramanian S, Gunasekaran K, Sasidharan S, Mathan VJ, Perumal E. MicroRNAs and xenobiotic toxicity: an overview. Toxicol Rep. 2020;7:583–595. doi:10.1016/j.toxrep.2020.04.010.
- Tal TL, Tanguay RL. Non-coding RNAs—novel targets in neurotoxicity. Neurotoxicology. 2012;33(3):530–544. doi:10.1016/j.neuro.2012.02.013.
- Foster PS, Plank M, Collison A, Tay HL, Kaiko GE, Li J, Mattes J, Hansbro PM, Kumar RK, Yang M. The emerging role of micro-RNA s in regulating immune and inflammatory responses in the lung. Immunol Rev. 2013;253(1):198–215. doi:10.1111/imr.12058.
- Bronevetsky Y, Ansel KM. Regulation of mi RNA biogenesis and turnover in the immune system. Immunol Rev. 2013;253(1):304–316. doi:10.1111/imr.12059.
- Ceppi M, Pereira PM, Dunand-Sauthier I, Barras E, Reith W, Santos MA, Pierre P. MicroRNA-155 modulates the interleukin-1 signaling pathway in activated human monocyte-derived dendritic cells. Proc Natl Acad Sci. 2009;106(8):2735–2740. doi:10.1073/pnas.0811073106.
- Hu M, Jovanović B, Palić D. In silico prediction of MicroRNA role in regulation of Zebrafish (Danio rerio) responses to nanoparticle exposure. Toxicol In Vitro. 2019;60:187–202. doi:10.1016/j.tiv.2019.05.014.
- Feng YH, Tsao CJ. Emerging role of microRNA-21 in cancer. Biomed. Rep. 2016;5(4):395–402. doi:10.3892/br.2016.747.
- Yelamanchili SV, Lamberty BG, Rennard DA, Morsey BM, Hochfelder CG, Meays BM, Fox HS, Fox HS. MiR-21 in extracellular vesicles leads to neurotoxicity via TLR7 signaling in SIV neurological disease. PLoS Pathog. 2015;11(7):e1005032. doi:10.1371/journal.ppat.1005032.
- Dostal Z, Modriansky M. The effect of quercetin on microRNA expression: a critical review. Biomed Pap Med Fac. 2019;163(2):95–106.
- Boesch-Saadatmandi C, Loboda A, Wagner AE, Stachurska A, Jozkowicz A, Dulak J, Rimbach G, Wolffram S, Rimbach G. Effect of quercetin and its metabolites isorhamnetin and quercetin-3-glucuronide on inflammatory gene expression: role of miR-155. J Nutr Biochem. 2011;22(3):293–299. doi:10.1016/j.jnutbio.2010.02.008.
- Garelnabi M, Mahini H. Modulation of microRNA 21, 125 b and 451 expression by quercetin intake and exercise in mice fed atherogenic diet. Biomed Prev Nutr. 2014;4(3):359–363. doi:10.1016/j.bionut.2014.04.005.
- Müller KH, Kulkarni J, Motskin M, Goode A, Winship P, Skepper JN, Porter AE, Porter AE. pH-dependent toxicity of high aspect ratio ZnO nanowires in macrophages due to intracellular dissolution. ACS nano. 2010;4(11):6767–6779. doi:10.1021/nn101192z.
- Garman RH. Histology of the central nervous system. Toxicol Pathol. 2011;39(1):22–35. doi:10.1177/0192623310389621.
- Mattson MP, Chan SL, Duan W. Modification of brain aging and neurodegenerative disorders by genes, diet, and behavior. Physiol Rev. 2002;82(3):637–672. doi:10.1152/physrev.00004.2002.
- Eluwa M, Inyangmme I, Akpantah A, Ekanem T, Ekong MB, Asuquo O, Nwakanma AA. A comparative study of the effect of diet and soda carbonated drinks on the histology of the cerebellum of adult female albino Wistar rats. Afr Health Sci. 2013;13(3):541–545. doi:10.4314/ahs.v13i3.1.
- Gouda S, Naim M, El-Aal H, Mahmoud S. Effect of alpha-phenyl-n-tert-butyl nitrone on aging of the cerebellum of male albino rats (Histological and Immuno-histochemical study). Egypt J Histol. 2010;33:495–507.
- Fereidounni S, Dhawan DK. Understanding the role of quercetin during neurotoxicity induced by Chlorpyrifos. J. Phytopharm. 2018;7(1):33–39. doi:10.31254/phyto.2018.7108.
- Haq SH, AlAmro AA. Neuroprotective effect of quercetin in murine cortical brain tissue cultures. Clin Nutr Exp. 2019;23:89–96. doi:10.1016/j.yclnex.2018.10.002.
- Kang S, Piao Y, Kang YC, Lim S, Pak YK. Qi-activating quercetin alleviates mitochondrial dysfunction and neuroinflammation invivoandinvitro. Arch Pharm Res. 2020;43(5):553–566. doi:10.1007/s12272-020-01238-x.
- Tan Z, Yang G, Qiu J, Yan W, Liu Y, Ma Z, Li J, Liu J, Shan N. Quercetin Alleviates Demyelination Through Regulating Microglial Phenotype Transformation to Mitigate Neuropsychiatric Symptoms in Mice with Vascular Dementia. Mol Neurobiol. 2022;59(5):3140–3158. doi:10.1007/s12035-021-02712-3.
- Olayinka J, Eduviere A, Adeoluwa O, Fafure A, Adebanjo A, Ozolua R. Quercetin mitigates memory deficits in scopolamine mice model via protection against neuroinflammation and neurodegeneration. Life Sci. 2022;292:120326. doi:10.1016/j.lfs.2022.120326.
- Fan H, Tang HB, Shan LQ, Liu SC, Huang DG, Chen X, Chen Z, Yang M, Yin XH, Yang H, et al. Quercetin prevents necroptosis of oligodendrocytes by inhibiting macrophages/microglia polarization to M1 phenotype after spinal cord injury in rats. J Neuroinflammation. 2019;16(1):1–15. doi:10.1186/s12974-019-1613-2.
- Kook SY, Jeong H, Kang MJ, Park R, Shin HJ, Han SH, Mook-Jung I, Song H, Baik SH, Moon M. Crucial role of calbindin-D 28k in the pathogenesis of Alzheimer’s disease mouse model. Cell Death Differ. 2014;21(10):1575–1587. doi:10.1038/cdd.2014.67.
- Yuan HH, Chen RJ, Zhu YH, Peng CL, Zhu XR. The neuroprotective effect of overexpression of calbindin-D 28k in an animal model of Parkinson’s disease. Mol Neurobiol. 2013;47(1):117–122. doi:10.1007/s12035-012-8332-3.
- Servais L, Bearzatto B, Schwaller B, Dumont M, De Saedeleer C, Dan B, Cheron G, Schiffmann SN, Cheron G. Mono‐and dual‐frequency fast cerebellar oscillation in mice lacking parvalbumin and/or calbindin D‐28k. Eur J Neurosci. 2005;22(4):861–870. doi:10.1111/j.1460-9568.2005.04275.x.
- Katsetos CD, Spandou E, Legido A, Taylor ML, Zanelli SA, de Chadarevian JP, Delivoria-Papadopoulos M, Mishra OP, Delivoria-Papadopoulos M. Acute hypoxia-induced alterations of calbindin-D 28k immunoreactivity in cerebellar purkinje cells of the guinea pig fetus at term. J Neuropathol Exp Neurol. 2001;60(5):470–482. doi:10.1093/jnen/60.5.470.
- Pihán P, Carreras-Sureda A, Hetz C. BCL-2 family: integrating stress responses at the ER to control cell demise. Cell Death Differ. 2017;24(9):1478–1487. doi:10.1038/cdd.2017.82.
- Rafiee Z, Khorsandi L, Nejad-Dehbashi F. Protective effect of zingerone against mouse testicular damage induced by zinc oxide nanoparticles. Environ Sci Pollut Res. 2019;26(25):25814–25824. doi:10.1007/s11356-019-05818-3.
- Sofroniew MV, Vinters HV. Astrocytes: biology and pathology. Acta Neuropathol. 2010;119(1):7–35. doi:10.1007/s00401-009-0619-8.
- Peinado MA, Martinez M, Ramirez, MJ, Quesada A, Iribar C, Pedrosa JM. Neuromorphological changes in neuronal and neuroglial population of the cerebral cortex in the aging rat. In: de Vellis, J.S. (eds) Neuroglia in the aging brain. 2002:pp.3-16. Humana Press, Totowa, NJ. doi:10.1007/978-1-59`259-105-3_1.
- Der Perng M, Su M, Wen SF, Li R, Gibbon T, Prescott AR, Quinlan RA, Quinlan RA. The Alexander disease–causing glial fibrillary acidic protein mutant, R416W, accumulates into Rosenthal fibers by a pathway that involves filament aggregation and the association of αB-crystallin and HSP27. Am J Hum Genet. 2006;79(2):197–213. doi:10.1086/504411.
- Li DR, Ishikawa T, Zhao D, Michiue T, Quan L, Zhu BL, Maeda H. Histopathological changes of the hippocampus neurons in brain injury. Histol Histopathol. 2009;24:1113-1120.
- Yang Y, Liu X, Wu T, Zhang W, Shu J, He Y, Tang SJ, Cruz ACR, Vasconcelos BHB, Chiang JO. Quercetin attenuates AZT-induced neuroinflammation in the CNS. Sci Rep. 2018;8(1):1–8. doi:10.1038/s41598-017-17765-5.
- Lanone S, Boczkowski J. Biomedical applications and potential health risks of nanomaterials: molecular mechanisms. Curr Mol Med. 2006;6(6):651–663. doi:10.2174/156652406778195026.
- Sharma HS. A special section on nanoneuroscience: nanoneurotoxicity and nanoneuroprotection. J Nanosci Nanotechnol. 2009;9(8):4992–4995. doi:10.1166/jnn.2009.GR01.
- Karmakar A, Zhang Q, Zhang Y. Neurotoxicity of nanoscale materials. J Food Drug Anal. 2014;22(1):147–160. doi:10.1016/j.jfda.2014.01.012.
- Yaqub A, Faheem I, Anjum KM, Ditta SA, Yousaf MZ, Tanvir F, Raza C. Neurotoxicity of ZnO nanoparticles and associated motor function deficits in mice. Appl Nanosci. 2020;10(1):177–185. doi:10.1007/s13204-019-01093-3.