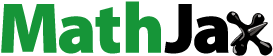
Abstract
To concentrate a potent anticancer drug (Arteether) in tumor microenvironment, we encapsulated it in biodegradable and pH sensitive polyurethane (PU) nanomicelles (NMs). The nanocomplex was characterized by Fourier transform infrared (FTIR), dynamic light scattering (DLS). The loading capacity and release profile in pH of 5.4 and 7.4 were considered. The cytotoxicity effect was evaluated in vitro and in vivo settings. The level of IFN-γ and IL-4 cytokines of mice splenocytes were assessed by enzyme-linked immunosorbent assay (ELISA). The nanocomplex showed negative zeta charge of −26.2 mV, size of 42.30 nm and high loading capacity (92%). Release profile showed a faster rate of drug liberation at pH 5.4 as compared to that of pH 7.4. It indicated significant inhibitory effect on the growth of 4T1 cell line and increased IFN-γ level.
Introduction
Artemisinin (ARM) and its derivatives have drawn a great deal of attention in cancer therapy in current years (Tran et al. Citation2016). Of these derivatives including artesunate, artemether, arteannuin, and arteether (ARE), in this study we have focused on the effect of the latest in mice bearing breast cancer. Interestingly, this group has exhibited anticancer effects like induction of apoptosis, inhibition of tumor growth, metastasis and angiogenesis (Jiao et al. Citation2007, Nakase et al. Citation2008). Because of high frequency of transferrin receptors on tumor cell surface compared to normal cells, there exists abundance of ferrous iron inside the tumor cells (Bystrom et al. Citation2014, Esserman et al. Citation1989, Gatter et al. Citation1983). On the other hand, the endoperoxide linkage in ARM analogs is sensitive to the iron (Ferreira et al. Citation2010, Ghantous et al. Citation2010, Kjær et al. Citation2013). On exposing this linkage to the iron, it would be broken and results in free radicals constitution following cell damage and ultimately cell death. A lot of studies have been performed on the immunomodulatory effects of ARM and its derivatives so far (Njuguna et al. Citation2012, Shakir et al. Citation2011) while a few studies in terms of immunostimulatory or immunosuppressive impacts of ARMs against cancer have been carried out. Previous investigations in our team indicated that ARM and Artemether could induce the immune response and decrease T regulatory cells in breast cancer models (Farsam et al. Citation2011, Langroudi et al. Citation2010) whereas ARE didn’t show any stimulatory effect on mice bearing breast cancer (Mohamadabadi et al. Citation2013). As ARE is not water-soluble, bioavailability of this drug would be restricted to the tumor site (Gupta and Chadha Citation2013). Hence, proper and adequate therapeutic effects might not be achieved. As a result, delivery of ARE in structures like nanomaterials could be a promising approach towards induction of immune response in cancer treatment.
Cancer nanotechnology as an interdisciplinary research creates an opportunity to early cancer diagnosis and treatment (Hosseini et al. Citation2016, Vienna and Wien Citation2015). Nanotechnology composes different structures at the nanoscale for drug delivery purposes (Dhankhar et al. Citation2010). Successful efforts have resulted in nanomaterials production. These nanomaterials include polymers, dendrimers, liposomes, carbon nanotubes, and metals such as iron oxide and gold (Nazir et al. Citation2014, Rahman et al. Citation2016, Singh et al. Citation2016). Among them polymeric micelles have also attracted much attention (Abyaneh et al. Citation2014, Ahmad et al. Citation2014, Ke et al. Citation2014). Polymeric nanomicelles (NMs) are made of two parts, a hydrophobic core bearing insoluble anticancer agents and a hydrophilic cover which keeps the nanoparticle in aqueous medium. This structure of NMs enables them to deliver hydrophobic drugs efficiently (Zhu et al. Citation2014).
In cancer drug delivery the presence of stimuli like pH or temperature is a necessity for a controlled profile of release around or inside the tumor cells (Kim et al. Citation2008, Lee et al. Citation2008). While the physiologic pH of blood stream is estimated to be 7.4, the tumoral pH is 4–6 (Bae et al. Citation2005). Thus, application of a nanomaterial like polyurethane (PU) micelles which are pH sensitive sould be paid more attention (Chen et al. Citation2010). PU micelles are considered as biodegradable self-assemble spherical and nanosized (10–100) materials which are able to physically entrap the hydrophobic drugs and improve the solubility pattern of drug. As a result, the circulation time of drug in blood stream would be enhanced and therefore sufficient amounts of it would be available for tumor tissue (Bae et al. Citation2005, Savic et al. Citation2003).
In the current study, with regard to low solubility of ARE and therefore low circulation time in vivo, for achieving an intelligent drug delivery we loaded the drug in PU NMs. Due to their sensitivity to acidic pH and possessing micelle structure, these NMs would be able to entrap the hydrophobic ARE and release it around or within the tumor cells. Accordingly, by providing sufficient amount of ARE in tumor site, the therapeutic effects of ARE might be elevated and stimulate the immune response.
Methods
Animals
Six to 8 week-old female BALB/c mice were bought from laboratory animal production center of Pasteur Institute of Iran (Alborz, Karaj, Iran). All experiments were carried out based on the guidelines of the Medical Ethics Committee of Tabriz University.
Synthesis of waterborne anionic PU nanocarrier
Poly tetra methylene ether glycol (PTMEG, Mn = 2000 g mol−1) (Sigma-Aldrich, St. Louis, MO) was dried at 90 °C under vacuum for 24 h just before use. Toluene diisocyanate (TDI mixture of 2, 4 and 2,6-isomers) (Merck, Kenilworth, NJ) was purified through vacuum distillation. Dimethylol-propionic acid (DMPA), triethanolamine (TEA) and phenol (Merck), were dried at 60 °C under vacuum for 24 h. N-methyl-2-pyrrolidone (NMP) (Sigma-Aldrich) dried via vacuum distillation over CaH2.
PTMEG (0.06 mol), DMPA (0.06 mol) and equal weight of NMP as solvent was placed into a four-necked round-bottomed reaction kettle equipped with a mechanical stirrer, heating mantle, reflux condenser, dropping funnel, and N2 inlet. The reaction mixture was stirred at 70 °C under N2 atmosphere. After complete mixing, TDI (0.16 mol) was dropped into the reactor at such a rate that the reaction temperature would not surpass 80 °C. The temperature was then increased to 85 °C and the reaction was continued till the NCO content reached the theoretical value as determined by dibutyl amine back titration method. Then the reaction mixture was cooled down to 60 °C and phenol (0.08 mol) diluted with acetone (150 mL) was dropped into the reactor to mask free isocyanate groups. The reaction was continued till no NCO peak was detected in Fourier transform infrared (FTIR) spectrum of sample collected from reaction mixture. The blocked PU pre-polymers were cooled to 50 °C and the neutralizing agent, TEA (0.06 mol) was added and allowed to react to form ionomer for 30 min. Finally, deionized water was added to accomplish the dispersion under vigorous stirring. A uniform dispersion was obtained, from which the acetone was removed under low vacuum at 60 °C. The final solution was diluted with deionized water in a way that each mL of solution contained 24 mg PU.
Preparation of ARE-loaded PU NMs
20 mg ARE (Exim-Pharm International Co, Mumbai, India) was dissolved in 280 μL Dimethyl Sulfoxide (DMSO) (Merck) and 200 μL polysorbate 80 (Merck). Then 1 mL PU solution containing 24 mg PU was added and stirred moderately. The resulting solution was reached to a volume of 4 mL with distilled water (DW) (Merck) and sonicated afterward using probe sonicator (Dehan, Seoul, Korea) with power of 250 KJ for 50 s.
Spectroscopic characterization of PU
FTIR spectroscopy was carried out using Bruker Instruments (Equinox 55, New York, NY) in the range of 4000–400 cm−1 at a resolution of 4 cm−1 and signal averaged over 8 scans. Small amounts of PU was put on a petri dish and evacuated till complete dryness. The solid material was mixed thoroughly with KBr (Sigma-Aldrich) and pressed. Consequently, a circular disk with 1 cm diameter and 100 micron thickness was formed.
Size and zeta potential characterization of ARE-loaded PU NMs
Size and zeta potential charge of ARE-loaded PU NMs were assessed by dynamic light scattering (DLS) using a Zeta sizer and Scattering Particle Size Analyzer (Malvern, Pennsylvania).
Determination of loading capacity
5 mg ARE was dissolved in 70 μL DMSO and supplemented with 70 μL polysorbate 80. Then 250 μL (6 mg) PU was added to the solution. The resulting solution was reached to a volume of 1 mL with DW and then sonicated as mentioned above with the same condition. The ARE-loaded PU NMs centrifuged in 5800g for 15 min (Hermle, Gosheim, Germany). The amount of nonencapsulated ARE in supernatant was then measured using ultraviolet (UV)-visible spectroscopy (Shimadzu, Kyoto, Japan) in wavelength of 250 nanometer (nm). The amount of free drug in supernatant then calculated with the standard curve provided. To this end, different concentrations of ARE must be used and a serial dilution was carried out for drawing a standard curve (0.00975, 0.0195, 0.039, 0.0758, 0.15, 0.313, 0.625, 1.25, 2.5, and 5 mg/mL). The amount of free and non-entrapped drug was compared to the total drug added to the solution of ARE loaded PU NMs and quantified as follow (Qu et al. Citation2013):
In vitro-release studies
The release profile of ARE-loaded PU NMs was considered in two pH including physiologic pH of 7.4 and acidic pH of 5.4. The later indicates endolysosomes pH of cancer cells. 7 mg of ARE-loaded PU NMs was redispersed in 7 mL of 0.01 M phosphate-buffered saline solution (pH 7.4) and this amount was also redispersed in 7 mL of 0.1 M citrate buffer (pH 5.4) separately. The total volume of each buffer was divided into 14 microtubes with volume of 500 μL in seven different sets in duplicate at the predetermined time intervals of 2, 4, 6, 12, 24, and 48 h. All sets were incubated in 37 °C under gentle agitation. At these determined intervals, samples were centrifuged at 5800 g for 15 min. Then the solution was analyzed and quantified with UV-visible spectroscopy (Shimadzu) in wavelength of 250 nm as follows (Bisht et al. Citation2007):
In vitro studies
Cell culture
4T1 cell line was obtained from National Cell Bank of Iran (NCBI). The cells were maintained in Dulbecco’s Modified Eagle’s Medium (DMEM) containing 2 mM l-glutamine, supplemented with 10% fetal bovine serum (FBS) (Gibco, Big Cabin, OK) and 100 U/mL penicillin, 100 μg/mL streptomycin solution (Gibco) in humidified 5% CO2 and 95% O2 at 37 °C (Gallenkamp, London, UK).
Isolation of peripheral blood mononuclear cell (PBMC)
Peripheral blood mononuclear cells (PBMCs) were obtained from 10 mL of human blood. This amount of blood was diluted with PBS and then for each 5 mL of diluted blood, 3 mL ficoll (Biosera, Colorado, UK) was applied. To begin the isolation procedure, first ficoll was poured into a sterile tube and after that, the diluted blood was added in a drop-wise manner. The tube was centrifuged in 350g at 22 °C for 20 min. The interface between the plasma and ficoll layers was collected and washed with 5 mL PBS in 350g at 4 °C for 10 min.
Cytotoxicity assay
The cytotoxicity activity of free ARE and ARE-loaded PU NMs was studied by 3-[4,5-dimethylthiazol-2-yl]-2,5-diphenyl tetrazolium bromide (MTT) (Merck) assay. 4T1 cells at a density of 1 × 104 per well in a volume of 200 μL DMEM supplemented with 10% FBS and 100 U/mL penicillin, 100 μg/mL streptomycin solution (Gibco) were seeded on two 96-well cell culture plates (Nunc, Roskilde, Denmark). After 24 h, they were treated with a series of dilutions 10, 20, 30, 40, and 50 μM of free ARE, PU and ARE-loaded PU NMs (each concentration contained 92% ARE according to loading capacity). Then, MTT at the final concentration of 0.5 mg/mL was added to each well after passing 24 h from exposing of our treatment. Then after incubation of 4 h and removing the medium of each well, 100 μL DMSO was added to dissolve the blue formazan crystals. The absorbance further was measured at 540 nm using enzyme-linked immunosorbent assay (ELISA) reader (Multiskan, San Diego, CA). The same procedure was carried out on human PBMCs at a density of 5 × 104 per well in a volume of 200 μL Roswell Park Memorial Institute Medium (RPMI) 1640 (Gibco) supplemented with 10% FBS and 100 U/mL penicillin, 100 μg/mL streptomycin solution (Gibco). The viability of cells was presented as the percentage of control as follows:
In vivo studies
Six-to-eight-week BALB/c mice were inoculated subcutaneously (SC) with 6 × 105 4T1 cells in 100 μL PBS (day 0). After 12 days, the tumor size was recorded and the treatment was started (). Groups were treated with ARE, PU, ARE-loaded PU NMs in PBS and cyclophosphamide (CYC) (Sigma-Aldrich) as a positive control and the final group wasn’t treated as a negative control. Mice were intraperitoneally (IP) injected with 0.1 mL of ARE (20 mg/kg) in DMSO and a little between 80 (Merck), 0.1 mL of PU (20 mg/kg) in PBS, 0.1 mL of ARE-loaded PU NMs (20 mg/kg) in PBS and 20 mg/kg CYC, once every other day on days of 11, 13, and 15. The tumor size of mice was measured on days of 11, 13, 15, and 17. The tumor volume of each group was measured using a digital caliper vernier (Mitutoyo, Singapore) and calculated with the following formula.
Figure 1. Tumor tissue formation. 106 4T1 cells were injected subcutaneously and the mass of tumor was generated after 12 days.
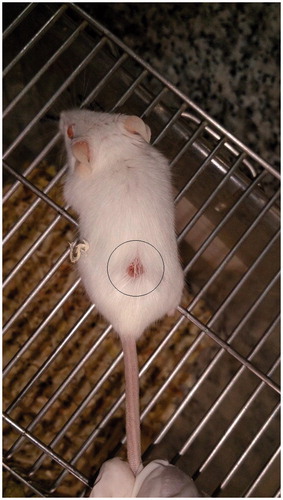
On day 21, mice were sacrificed by cervical dislocation method and immunoassays were carried out as outlined below.
Preparation of tumor lysis antigen
A piece of tumor tissue of breast cancer bearing BALB/c mouse with the volume of 3000 mm3 was segmented in 10 mL PBS. The obtained substance underwent the freeze-thaw process for five times. After that, to inactivate serin proteases, 1 mM phenylmethylsulfonyl fluoride (PMSF) (Sigma-Aldrich) was added and then sonicated. The cell lysate was centrifuged in 3000 g for 15 min at 4 °C and the supernatant was dialyzed using bag dialysis (Spectrapor, MW cutoff 3500 g/mol) for 24 h. The extract was filtered with 0.22 μm filter (GVS, San Jose, CA). For determining the concentration, Bradford method was utilized. Finally, for further use, the extract was maintained at −20 °C (GFL, Lugano, Switzerland).
Cytokine assay
On day 21, mice were sacrificed with cervical dislocation method and the spleen was aseptically resected. Splenocytes were seeded at a density of 1 × 106/mL per well (in RPMI1640 medium culture containing 2 mM l-glutamine, supplemented with 10% FBS (Gibco) and 100 U/mL penicillin, 100 μg/mL streptomycin solution (Gibco) in 24-well culture plates (Nunc) in the presence of 20 μg/mL tumor lysis antigen. The supernatants of spleen cells were collected after three days incubation at 37 °C in humidified 5% CO2 (Gallenkamp) and the levels of IFN-γ and IL-4 cytokines concentration were evaluated using ELISA technique. An ELISA kit (R&D, Minneapolis, MN) was purchased to measure IFN-γ and IL-4 and all the procedures were carried out according to the procedure.
Statistical analysis
Statistical analyses were performed using GraphPad software version 6.01 (Prism, Manila, Philippines). P values less than 0.05 were considered as statistically significant. The data are presented here as mean ± SD of three independent experiments.
Results
Synthesis and characterization of waterborne PU nanocarrier
The synthetic route followed for the preparation of waterborne PU is depicted in Scheme 1. The mixture of PTMEG and DMPA was reacted with excessive amount of TDI. The resulting prepolymer was end-capped with proper amount of phenol to restrict the molecular weight growth of PU. The embedded carboxylic acids in the backbone of PU were then neutralized by TEA. The generated ionic centers provided water reducibility for prepared PU. The structure of this compound was elucidated by examination of its FTIR spectrum. FTIR spectrum of PU, is presented in . The absence of characteristic peak of NCO group around 2270 cm−1 indicated the complete reaction of this functional group with alcohol and phenol moieties. Broad peak detected at about 3200 cm−1 was related to stretching vibration of urethane NH and OH groups of TEA neutralizing agent. Peaks at 2850 and 2939 cm−1 related to stretching vibration of methylene groups (CH2) of PTMEG moiety. Strong peak appeared at 1729 cm−1 was related to stretching vibration of urethane group (NH−CO−O). Combination of N–H bond bending and C–N bond stretching vibrations was appeared as a peak at 1535 cm−1. The presence of ether bond (C–O–C) in PU structure originating from PTMEG moieties was also confirmed by detection of peak appeared at 1112 cm−1.
Size-zeta potential
The DLS analysis showed the size of 42.30 nm and the zeta charge of −26.2 mV for ARE-loaded PU NMs as exhibited in .
Drug loading consideration
According to the standard curve of ARE different concentrations and the amount of free drug from the total drug or the whole NMs, 920 μg of drug was entrapped from 1 mg of ARE consumed. In other words, the loading capacity percentage was determined 92%.
Drug release response
The amount of free drug released from the NMs in the time interval of 2, 4, 6, 12, 24, and 48 h was determined using UV spectrophotometer and the profile of release is shown in .
Figure 4. The release profile of ARE from PU NMs in citrate and phosphate buffer. After 24 h, 60.80% of total ARE embedded in PU NMs was released in citrate buffer, while this percentage was 3.2% in phosphate buffer in this time. After 48 h, 91.4% of total ARE was released in citrate buffer whereas just 4.2% of total drug in PU NMs was released in phosphate buffer.
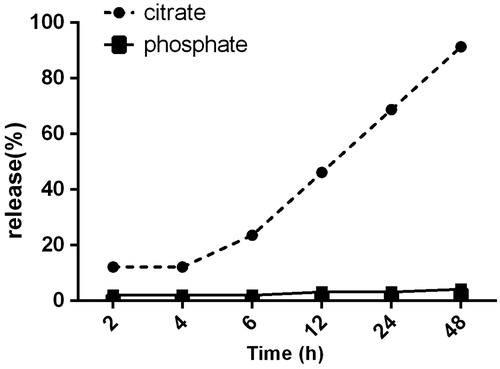
Cytotoxicity assay
The cells were treated with the predetermined concentrations of 10–50 μM of ARE of which, the concentration of 30 μM of drug killed nearly 50% of cells after 24 h. Besides, PU showed no toxicity on 4T1 cell line. The viability indicated that the concentration of 40 μM ARE-loaded PU NMs caused the death of approximately 50% after 24 h (). Based on , ARE, PU, and ARE-loaded PU NMs exhibited no toxicity on PBMCs.
Tumor volume measurements
The groups receiving ARE, ARE-loaded PU NMs, and CYC reduced the cell growth of tumor on the day of 17 as compared with the untreated group and this reduction in cell growth was significant as compared with the day of 11 in groups receiving ARE and ARE-loaded PU NMs. It seems that PU by itself caused no effect on the rate of tumor growth. On the other hand, no significant difference (P < 0.05) was observed between the groups treated with ARE and ARE-loaded PU NMs on the last day (17th day). According to , ARE-loaded PU NMs and CYC showed similar effects on tumor growth.
Figure 6. The tumor size in groups of ARE, ARE-loaded PU and PU NMs in comparison with CYC and the untreated group are shown. On the day of 17, the groups received ARE, ARE-loaded PU and CYC indicated a significant reduction in tumor growth as compared with the untreated group. There was no significant difference between the day of 17 as compared to the day of 11 in groups received ARE, ARE loaded PU and CYC and it is indicative of inhibition of tumor growth. The data also showed that PU did not have any effect on tumor growth control. Additionally, ARE loaded PU acts similarly with CYC in tumor growth inhibition.
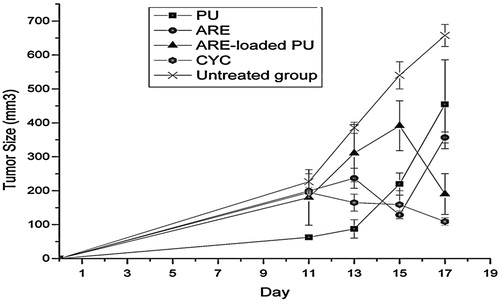
Cytokine assay
ARE-loaded PU NMs had significant effect on production of IFN-γ (P < 0.0001) in comparison with the groups receiving blank PU, ARE, CYC, and the untreated group which received PBS (. ARE-loaded PU NMs showed no effect on production of IL-4 together with the untreated and ARE receiving groups while a little production of IL-4 is observed in the group treated with PU (about 9 pg/mL).
Figure 7. The assay of IFN-γ (A) and IL-4 (B) cytokines by enzyme-linked immunosorbent assays (ELISA). ARE-loaded PU NMs revealed a significant effect on production of IFN-γ while no effect on IL-4 generation was observed. According to the information, ARE did not show any effect on immune response.
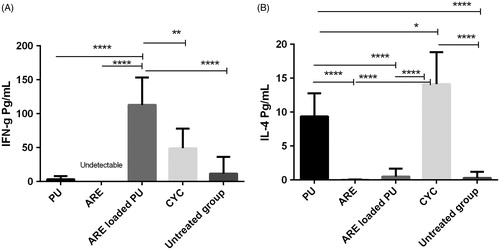
Discussion
Because of the therapeutic effects of ARE in cancer field (Nakase et al. Citation2008) and on the other side the outstanding characteristics of PU in drug delivery, in this research we encapsulated ARE in this NM. The main aim of this study was to concentrate the drug of ARE around the tumor microenvironment, to arrive an efficient transportation and to minimize the probable side effects of this drug like displaying nervous system damage (Brewer et al. Citation1994). To pursue this goal, we synthesized a NM complex of ARE-loaded PU and evaluated the cytotoxicity and its immune response in vitro and in vivo.
The size and zeta potential of loaded NMs were analyzed applying Malvern zeta sizer. Primarily, particles in the size of 20–50 nm are managed to pass through the leaky endothelial cells of tumor vessels easier and faster (Iversen et al. Citation2011). On the other hand, there is a direct relation between the charge of particles and their attachment to tumor cells and this chance increases as the particles gain more positive charge. In a parallel study on application of paclitaxel-loaded PU against MCF-7 cell lines, the charge of −43 mV and size of 50 nm were achieved (Khosroushahi et al. Citation2012). Accordingly the size of 42.30 nm and the charge of −26.2 mV in ARE-loaded PU NMs were approvable and considerable findings.
Depending on circulation time in body and pH sensitivity, the ARE-loaded PU NMs might act differently. As a result, the release profile and the loading capacity of these NMs were evaluated in vitro. High loading capacity of 92% is considered as an advantage not only for the encapsulation potential of PU, but also for solubilizing the hydrophobic ARE and therefore concentrating it in tumor surrounding. In the determined time intervals, each set of samples in buffers of citrate and phosphate showed different profile of release. The release of 60.8% and 91.4% after 24 h and 48 h respectively in citrate buffer and the release of 3.2% and 4.2% after 48 h in order in phosphate buffer is indicative of high sensitivity of this NM to acidic pH. Hence, it is expected to reach to the same result in vivo as well. As mentioned in other studies, PU is sensitive to acidic pH around the tumor (Zhou et al. Citation2012). It exhibited that this NM is a promising material to trigger the release of drug in tumor environment and ultimately concentrate it in the site of tumor for further bioavailability.
For checking the cytotoxicity rate of ARE-, PU-, and ARE-loaded PU NMs, 4T1 murine cell line and human PBMCs were considered as breast cancer and normal cell respectively. As demonstrated in other studies, PU NMs are regarded as biocompatible and biodegradable materials in clinical use (Santerre et al. Citation2005). In the current research, the same result was achieved as well and this nanoparticle exhibited no toxicity on PBMCs and 4T1 cell line. Based on the results obtained here, this could be a positive point for applying this material in drug delivery. Since there exists high amounts of Fe2+ iron in the cancerous cells as compared to normal cells, it is expected to observe more cytotoxicity on tumor cells than that of in normal ones as usual. ARE in the concentration of 30 μM caused to death of about 50% of 4T1 cells while 40 μM of ARE-loaded PU NMs functioned in almost the same way and it was a little bit less effective than ARE itself. Obviously, according to the release profile of ARE from NMs after 24 h in citrate buffer in the present study, around about 60% of drug would be released after 24 h. As a result, this could be a good evidence for analyzing this difference in vitro. It is clarified in other studies that anionic NMs with the size of around 40 nm would enter the cells via clatherin-dependent endocytosis (Sahay et al. Citation2010). Thus, our designed nanocomplex with the same properties might release the determined drug with the mentioned manner around or more likely within the tumor cells. Consequently, in this manner ARE would take more time to be released gradually from PU NMs than utilizing just ARE directly. Notably, one of the remarkable characteristics of nanoparticles application in drug delivery is to release the entrapped material in a gradual way.
Both IP injection of ARE and ARE loaded PU NMs with dose of 20 mg/kg led to tumor growth inhibition on the day of 17 as compared to day of 11. 20 mg/kg of nanocomplex contained definitely less ARE in comparison with the same dose in ARE alone. This implies that ARE-loaded PU NMs carrying less drug had the same effect on tumor growth. Despite the absence of significant difference between ARE and ARE loaded PU NMs in growth of tumor on the day of 17, the latter indicated similar impact statistically on the growth with CYC.
TH1 polarization in tumor surrounding provides a suitable condition for anti-tumor responses. It is of great importance that the generation of IFN-γ by TH1 is a help for the cytotoxic T cells to be activated and to be the effector cells in killing tumor cells and finally making the immune response stronger against cancerous cells (Grivennikov et al. Citation2010, Wang et al. Citation2012). Our results clarify that IP injection of NMs leads to significant anti-tumor activity in BALB/c model of breast cancer.
In the previous study it had been shown that ARE solely was not able to stimulate immune response from the aspect of IFN-γ and IL-4 cytokines (Mohamadabadi et al. Citation2013). Although ARE by itself showed no effects on production of these two cytokines in present study, significant changes in immune response and tumor growth following NC injection were observed comparing to control group. It seems that there is more likely a causal relation between increased IFN-γ and inhibited tumor growth. Based upon the results obtained in our study, ARE-loaded PU NMs can have hopeful therapeutic effects on decrease in tumor growth and increase in the stimulation of TH1. In addition, the NC indicated no effect on IL-4 production, while PU NMs had a little increase in its generation which it was statistically significant in comparison with ARE-loaded PU NMs received group and the untreated ones.
Conclusion
The findings in the present study indicated that the synthesized PU NMs show a high-loading capacity. Also, they are able to present high amounts of ARE with a controlled delivery in acidic pH. ARE loaded PU NMs can reduce the rate of tumor growth in breast cancer model and enhance the level of IFN-γ as well. Therefore, this NC could be presented as a therapeutic drug for stimulating the immune response to TH1 and also for killing the tumor cells. However, the mechanisms of tumor growth inhibition should be investigated in future. More examinations should be experimented on the impact of NC on the patterns of other regulatory cytokines and infiltration of lymphocytes into tumor sites.
Acknowledgements
The authors express gratefulness to the Tabriz University of Medical Sciences for execution of this study and Immunology research center of Tabriz for funding.
Disclosure statement
The authors declare that they have no competing interests.
References
- Abyaneh HS, Vakili MR, Lavasanifar A. 2014. The effect of polymerization method in stereo-active block copolymers on the stability of polymeric micelles and their drug release profile. Pharm Res. 31:1485–1500.
- Ahmad Z, Shah A, Siddiq M, Kraatz HB. 2014. Polymeric micelles as drug delivery vehicles. RSC Adv. 4:17028–17038.
- Bae Y, Jang WD, Nishiyama N, Fukushima S, Kataoka K. 2005. Multifunctional polymeric micelles with folate-mediated cancer cell targeting and pH-triggered drug releasing properties for active intracellular drug delivery. Mol Biosyst. 1:242–250.
- Bisht S, Feldmann G, Soni S, Ravi R, Karikar C, Maitra A, Maitra A. 2007. Polymeric nanoparticle-encapsulated curcumin (“nanocurcumin”): a novel strategy for human cancer therapy. J Nanobiotechnol. 5:1–18.
- Brewer TG, Peggins JO, Grate SJ, Petras J, Levine BS, Weina PJ, et al. 1994. Neurotoxicity in animals due to arteether and artemether. Trans R Soc Trop Med Hyg. 88:33–36.
- Bystrom LM, Guzman ML, Rivella S. 2014. Iron and reactive oxygen species: friends or foes of cancer cells? Antioxid Redox Signal. 20:1917–1924.
- Chen D, Jiang X, Liu J, Jin X, Zhang C, Ping Q. 2010. In vivo evaluation of novel pH-sensitive mPEG-Hz-Chol conjugate in liposomes: pharmacokinetics, tissue distribution, efficacy assessment. Artif Cells Blood Substit Biotechnol. 38:136–142.
- Dhankhar R, Vyas SP, Jain AK, Arora S, Rath G, Goyal AK. 2010. Advances in novel drug delivery strategies for breast cancer therapy. Artif Cells Blood Substit Immobil Biotechnol. 38:230–249.
- Esserman L, Takahashi S, Rojas V, Warnke R, Levy R. 1989. An epitope of the transferrin receptor is exposed on the cell surface of high-grade but not low-grade human lymphomas. Blood. 74:2718–2729.
- Farsam V, Hassan ZM, Zavaran-Hosseini A, Noori S, Mahdavi M, Ranjbar M. 2011. Antitumor and immunomodulatory properties of artemether and its ability to reduce CD4+ CD25+ FoxP3+ T reg cells in vivo. Int Immunopharmacol. 11:1802–1808.
- Ferreira JFS, Luthria DL, Sasaki T, Heyerick A. 2010. Flavonoids from Artemisia annua L. as antioxidants and their potential synergism with artemisinin against malaria and cancer. Mol Basel Switz. 15:3135–3170.
- Gatter KC, Brown G, Trowbridge IS, Woolston RE, Mason DY. 1983. Transferrin receptors in human tissues: their distribution and possible clinical relevance. J Clin Pathol. 36:539–545.
- Ghantous A, Gali-Muhtasib H, Vuorela H, Saliba NA, Darwiche N. 2010. What made sesquiterpene lactones reach cancer clinical trials? Drug Discov Today. 15:668–678.
- Grivennikov SI, Greten FR, Karin M. 2010. Immunity, inflammation, and cancer. Cell. 140:883–899.
- Gupta S, Chadha R. 2013. Studies on the preparation and evaluation of antimalarial activity of arteether and complexed arteether with β-CD loaded chitosan/lecithin nanoparticles. Pharm Nanotechnol. 1:204–212.
- Hosseini M, Haji-Fatahaliha M, Jadidi-Niaragh F, Majidi J, Yousefi M. 2016. The use of nanoparticles as a promising therapeutic approach in cancer immunotherapy. Artif Cells Nanomed Biotechnol. 44:1051–1061.
- Iversen TG, Skotland T, Sandvig K. 2011. Endocytosis and intracellular transport of nanoparticles: present knowledge and need for future studies. Nano Today. 6:176–185.
- Jiao Y, Ge C, Meng Q, Cao J, Tong J, Fan S. 2007. Dihydroartemisinin is an inhibitor of ovarian cancer cell growth. Acta Pharmacol Sin. 28:1045–1056.
- Ke XY, Ng VWL, Gao SJ, Tong YW, Hedrick JL, Yang YY. 2014. Co-delivery of thioridazine and doxorubicin using polymeric micelles for targeting both cancer cells and cancer stem cells. Biomaterials. 35:1096–1108.
- Khosroushahi AY, Naderi-Manesh H, Yeganeh H, Barar J, Omidi Y. 2012. Novel water-soluble polyurethane nanomicelles for cancer chemotherapy: physicochemical characterization and cellular activities. J Nanobiotechnol. 10:2–15.
- Kim D, Lee ES, Oh KT, Gao ZG, Bae YH. 2008. Doxorubicin-loaded polymeric micelle overcomes multidrug resistance of cancer by double-targeting folate receptor and early endosomal pH. Small Weinh Bergstr Ger. 4:2043–2050.
- Kjær A, Verstappen F, Bouwmeester H, Ivarsen E, Fretté X, Christensen LP, Grevsen K, Jensen M. 2013. Artemisinin production and precursor ratio in full grown Artemisia annua L. plants subjected to external stress. Planta. 237:955–966.
- Langroudi L, Hassan ZM, Ebtekar M, Mahdavi M, Pakravan N, Noori S. 2010. A comparison of low-dose cyclophosphamide treatment with artemisinin treatment in reducing the number of regulatory T cells in murine breast cancer model. Int Immunopharmacol. 10:1055–1061.
- Lee ES, Gao Z, Kim D, Park K, Kwon IC, Bae YH. 2008. Super pH-sensitive multifunctional polymeric micelle for tumor pH(e) specific TAT exposure and multidrug resistance. J Control Release. 129:228–236.
- Mohamadabadi MA, Hassan ZM, Hosseini AZ, Noori S, Mahdavi M, Maroufizadeh S, Maroof H. 2013. Study of immunomodulatory effects of arteether administrated intratumorally. Iran J Allergy Asthma Immunol. 12:57–62.
- Nakase I, Lai H, Singh NP, Sasaki T. 2008. Anticancer properties of artemisinin derivatives and their targeted delivery by transferrin conjugation. Int J Pharm. 354:28–33.
- Nazir S, Hussain T, Ayub A, Rashid U, MacRobert AJ. 2014. Nanomaterials in combating cancer: Therapeutic applications and developments. Nanomed Nanotechnol Biol Med. 10:19–34.
- Njuguna NM, Ongarora DS, Chibale K. 2012. Artemisinin derivatives: a patent review (2006-present). Expert Opin Ther Pat. 22:1179–1203.
- Qu JB, Shao HH, Jing GL, Huang F. 2013. PEG-chitosan-coated iron oxide nanoparticles with high saturated magnetization as carriers of 10-hydroxycamptothecin: preparation, characterization and cytotoxicity studies. Colloids Surf B Biointerfaces. 102:37–44.
- Rahman M, Kumar V, Beg S, Sharma G, Katare OP, Anwar F. 2016. Emergence of liposome as targeted magic bullet for inflammatory disorders: current state of the art. Artif Cells Nanomed Biotechnol. 44. [Epub ahead of print]. doi: 10.3109/21691401.2015.1129617.
- Sahay G, Alakhova DY, Kabanov AV. 2010. Endocytosis of nanomedicines. J Control Release. 145:182–195.
- Santerre JP, Woodhouse K, Laroche G, Labow RS. 2005. Understanding the biodegradation of polyurethanes: from classical implants to tissue engineering materials. Biomaterials. 26:7457–7470.
- Savic R, Luo L, Eisenberg A, Maysinger D. 2003. Micellar nanocontainers distribute to defined cytoplasmic organelles. Science. 300:615–618.
- Shakir L, Hussain M, Javeed A, Ashraf M, Riaz A. 2011. Artemisinins and immune system. Eur J Pharmacol. 668:6–14.
- Singh J, Jain K, Mehra NK, Jain NK. 2016. Dendrimers in anticancer drug delivery: mechanism of interaction of drug and dendrimers. Artif Cells Nanomed Biotechnol. 44. [Epub ahead of print]. doi: 10.3109/21691401.2015.1129625.
- Tran TH, Nguyen AN, Kim JO, Yong CS, Nguyen CN. 2016. Enhancing activity of artesunate against breast cancer cells via induced-apoptosis pathway by loading into lipid carriers. Artif Cells Nanomed Biotechnol. 44. [Epub ahead of print]. doi: 10.3109/21691401.2015.1129616.
- Vienna S, Wien S. 2015. Cancer nanotechnology. Cancer. 6:1–8.
- Wang B, Zaidi N, He LZ, Zhang L, Kuroiwa JMY, Keler T, Steinman RM. 2012. Targeting of the non-mutated tumor antigen HER2/neu to mature dendritic cells induces an integrated immune response that protects against breast cancer in mice. Breast Cancer Res. 14:R39.
- Zhou L, Liang D, He X, Li J, Tan H, Li J, Fu Q, Gu Q. 2012. The degradation and biocompatibility of pH-sensitive biodegradable polyurethanes for intracellular multifunctional antitumor drug delivery. Biomaterials. 33:2734–2745.
- Zhu L, Perche F, Wang T, Torchilin VP. 2014. Matrix metalloproteinase 2-sensitive multifunctional polymeric micelles for tumor-specific co-delivery of siRNA and hydrophobic drugs. Biomaterials. 35:4213–4222.