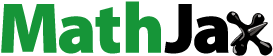
Abstract
Herein a novel amperometric biosensor based on a conducting polymer with anionic dopant modified electrode was successfully developed for detection of cholesterol. Polypyrrole is deposited on a platinum surface and the sodium dodecylbenzene sulphonate (DBS) ion-doped polypyrrole film was electrochemically prepared by scanning the electrode potential between −0.8 and +0.8 V at a scan rate of 20 mV/s. The present electrochemical biosensor was optimized in terms of working potential, number of cycles, concentrations of monomer, and anionic dopant. Cholesterol oxidase (ChOx) was physically entrapped in PPy–DBS to construct an amperometric cholesterol biosensor. Amperometric determination is based on the electrochemical detection of H2O2 generated in the enzymatic reaction of cholesterol. Kinetic parameters, operational and storage stabilities, pH, and temperature dependencies were determined. Km and Imax were calculated as 0.11 μM and 0.967 nM/min, respectively. The operational stability results showed that 90.0% of the response current was retained after 30 activity assays. Morphology of electrodes was characterized by SEM and AFM. Additionally, contact angle measurements were made with 1 μL water of polymer film and enzyme electrode. As a result, the cholesterol biosensor suggested in this study is easy to prepare and is highly cost-effective. This composite (PPy–DBS) can supply a biocompatible and electrochemical microenvironment for immobilization of the enzyme, making this material a good candidate for the fabrication of highly sensitive and selective cholesterol biosensors.
Introduction
Cholesterol is a fundamental diagnostic parameter for coronary heart disease, arteriosclerosis, and other clinical (lipid) disorders. It is used to identify the risks involved in thrombosis and myocardial infarction. The clinical analysis of cholesterol in serum samples is important to diagnose and prevent such clinical disorders as hypertension, cerebral thrombosis, and heart attack. Hence, it is important to develop a reliable and sensitive biosensor which can permit a suitable and rapid determination of cholesterol. Ideally, the total cholesterol concentration should be less than 200 mg/dL (<5.17 mM). The borderline high cholesterol is defined as 200–239 mg/dL (5.17–6.18 mM), and 240 mg/dL (≥6.21 mM) and above is accepted as high.
Different analytical methods have been used for the determination of cholesterol such as colorimetric, spectrometric and electrochemical methods. Among these methods, electrochemical detection has attracted significant attention due to its speed, simplicity, and low cost. Thus, amperometric biosensors are more attractive due to their low detection limit as enzyme stabilization can easily be achieved. Especially, the enzyme based cholesterol sensors have gained special focus due to their long term stability, high sensitivity, low cost, fast response, and wide linear range. Therefore, they hold a leading position among the presently available biosensor systems (Moraes et al. Citation2009, Ram et al. Citation2001).
Most of the literature on cholesterol biosensors has focused on diagnosing disorders (Bongiovanni et al. Citation2001, Moraes et al. Citation2009, Muhammed et al. Citation2009), mostly on such cardiovascular diseases as heart disease, atherosclerosis, hypertension, coronary artery disease, and cerebral thrombosis (Ansari et al. Citation2008, Nauck et al. Citation2000, Yu and Ju Citation2002).
In the fabrication of cholesterol biosensor for the estimation of free cholesterol and total cholesterol, mainly cholesterol oxidase (ChOx) and cholesterol esterase (ChEt) have been employed as the sensing elements (Arya et al. Citation2008, Feng and Liu Citation2015, Rahman and Asiri Citation2015, Umar et al. Citation2009). Electrochemical transducers have been effectively utilized for the estimation of cholesterol in the system (Arya et al. Citation2007, Charpentier and Murr Citation1995, Singh et al. Citation2006, Yıldırımoğlu et al. Citation2009, Zhou et al. Citation2006). Based on the number and reliability of optical methods, a variety of optical transducers have been used for cholesterol sensing, namely monitoring: luminescence, change in color of dye, fluorescence, and the others (Arya et al. Citation2008).
Different analytical methods have been used for the determination of cholesterol for instance colorimetric, spectrometric and electrochemical methods (Ahmad et al. Citation2012, Krug et al. Citation1994, Valentini et al. Citation2004, Yao et al. Citation1995). Among these methods, electrochemical detection of cholesterol has achieved significant attention due to the rapid determination, simplicity, and low cost. Thus, amperometric biosensors are more attractive due to their low detection limit and enzyme stabilization can be easily achieved. An amperometric enzyme electrode has also been used for the determination of cholesterol, but the disadvantage is the long-term stability of the enzyme. It has been mentioned in the literature that free cholesterol is determined by enzyme electrode, which is immobilized on the conducting polymer matrix (polypyrrole (PPy) or polyaniline) (Srivastava et al. Citation2014, Soylemez et al. Citation2014).
Amperometric biosensors are also used for the accurate measurement of cholesterol in a rapid manner without any need for sample pretreatment. ChOx contains flavin adenine dinucleotide (FAD) as the active redox centre. The amperometric detection of cholesterol can be based on enzymatic reaction employing ChOx and this reaction is followed by electrochemical oxidation on the electrode surface.
Several detection schemes have been proposed for enzymatic monitoring of cholesterol. Production of hydrogen peroxide during the above-mentioned enzymatic reaction can be measured using an electrochemical reaction (Çete and Bal Citation2013).
Conductive polymer electrodes in biosensor application have started to become widespread. The immediate advantage of conductive polymer electrodes is the lower cost of the raw materials and the economical production steps. Among various conducting polymers, PPy as an intelligent material plays an important role in the electrochemical biosensors for the purpose of increased electrochemical activity and sensitivity, owing to its good biocompatibility, conductivity, stability, and efficient polymerization at neutral pH as well as easy synthesis. PPy films can easily be formed from aqueous solutions by chemical or electrochemical routes, and have a high degree of selectivity due to their inherent size-exclusion property. A recent review on the applications of polymers in electrochemical biosensors could be found in the literature (Cete et al. Citation2006, Çete et al. Citation2007, Dolmaci et al. Citation2012, Gorgulu et al. Citation2013, Teles and Fonseca Citation2008), in which PPy is highlighted.
Polymers present themselves as advantageous materials for the following reasons (Guiseppi-Elie et al. Citation1995). Firstly, there is considerable flexibility in the available monomeric structures. Secondly, many of the available monomers are compatible with the conditions required for enzyme activity such as neutral aqueous solutions. Thirdly, electroactive polymers are capable of being reversibly doped and undoped, a feature which may be exploited to develop enzyme-based biosensors. Finally, these materials can be deposited electrochemically, a method that offers a simple approach for enzyme immobilization. Now there exists a growing literature on the use of electroactive polymers and its derivatives for sensor fabrication (Kim et al. Citation2000, Shin et al. Citation1996, Soylemez et al. Citation2013a, Soylemez et al. Citation2013b).
In the present paper, we report the results of a systematic study on the technical development and optimization of an amperometric biosensor observed by physically entrapped cholesterol oxidase in PPy–DBS films. DBS was used as anionic dopant. Several parameters such as working potential, concentrations of pyrrole and DBS, cyclic number were optimized and substrate concentration was studied. Besides, effect of pH, temperature, operational and storage stabilities were determined. Furthermore, AFM, SEM, and contact angle techniques were used for PPy–DBS electrodes morphology.
Materials and methods
Equipment and reagents
The electrochemical experiments were carried out by using EpsilonEC electrochemical analyzer with a three-electrode cell. The working electrode was a Pt plate (0.5 cm2). Auxiliary and reference electrodes were Pt wire and Ag/AgCl electrode (3.0 M KCl), respectively. The pH values of the buffer solutions were measured using an ORION Model 720 A pH/ionmeter. Temperature control was achieved with Grant GD120 thermostat. Cholesterol oxidase (EC 1.7.3.3 from Aspergillus niger purified from the microorganism and with an activity of 10 unit mL−1) and cholesterol were purchased from Sigma. The supporting electrolyte sodium perchlorate, pyrrole, and sodium dodecylbenzene sulphonate (DBS) were supplied by Aldrich. All other chemicals were obtained from Sigma. All the solutions were prepared using twice-distilled water.
Preparation of PPy–DBS film electrode
The surface of the Pt plate electrode was cleaned (Gros et al. Citation2000) and covered with PPy–DBS film by electropolymerization of pyrrole in an anionic dopant. The Pt plate electrode was immersed in 10.0 mL solution containing 0.15 M pyrrole and 5.0 mL of sodium DBS. The solution was purged with argon to remove the oxygen. Pyrrole electropolymerization was carried out on the Pt electrode surface by cyclic voltammetric scans between −0.8 and +0.8 V at a scan rate of 20 mV/s (vs Ag/AgCl electrode (3.0 M KCl)). PPy was obtained after 4 cycles ( and ). After electropolymerization, PPy–DBS film was rinsed with deionized water to remove the unreacted pyrrole monomer.
The sensitivity of PPy–DBS film electrode to hydrogen peroxide
The response current of electrode covered with PPy was determined as follows. A buffer solution of pH 7.0 (10 mL, 25° C) was added to the cell. The solution included 0.1 M phosphate to adjust pH and 0.1 M sodium perchlorate as a supporting electrolyte. The electrodes were maintained at 0.4 V for approximately 4 h and steady current (ia) was saved. The different concentration of hydrogen peroxide was added to the cell. The currents acquired at 0.4 V were recorded every 200 s. The difference in current values was plotted against the hydrogen peroxide concentration. Linear region of current values was determined ().
Immobilization of cholesterol oxidase on PPy–DBS film
Pt/PPy–DBS–ChOx film electrode was used against the Pt electrode, which was used as the counter electrode. The reference electrode was composed of Ag/AgCl. Immobilization of cholesterol oxidase was carried out by the physical entrapment approach. The concentrations of pyrrole and DBS were 0.15 M and 0.1 M, respectively. 0.5 mL of ChOx enzyme (10 U/mL) is added into the solution. The solution was purged with argon to remove the oxygen. Electropolymerization was carried out on the Pt electrode surface by cyclic voltammetric scans between −0.8 and 0.8 V at a scan rate of 20 mV/s (vs Ag/AgCl electrode (3.0 M KCl)). PPy was obtained after 4 cycles. After the fabrication of cholesterol oxidase entrapped PPy film onto the PPy–DBS working electrode was finished, the electrode was rinsed with deionized water to remove the unreacted pyrrole monomer and free cholesterol oxidase. Immobilized enzyme electrode was kept in a refrigerator at the 4 °C in phosphate buffer when it was not in use (Nauck et al. Citation1997).
The sensitivity of PPy–DBS–ChOx enzyme electrode to hydrogen peroxide
The response currents of enzyme electrode were determined as follows. A buffer solution of pH 7.0 (10 mL, 25 °C) was added to the cell. The solution included 0.1 M phosphate to adjust pH and 0.1 M sodium perchlorate as a supporting electrolyte. The enzyme electrodes were kept at 0.4 V for approximately 5 h and steady current (ia) was saved. The different concentration of cholesterol was added to the cell. The currents acquired at 0.4 V were recorded every 200 s. The difference in current values was plotted against the cholesterol concentration.
Amperometric measurements
Quantification of cholesterol was achieved via electrochemical detection of enzymatically released H2O2.
Enzyme electrode was immersed into phosphate buffer (0.1 M) at pH 7.0. The solution contained 0.1 M sodium chloride as supporting electrolyte. Electrode was brought to equilibrium by keeping it at 0.4 V [vs. Ag/AgCl electrode (3.0 M KCl)]. Steady-state current (ia) was recorded. Cholesterol solution was added to the cell from stock solution and the system was stirred. Current (ib) values obtained at 0.4 V were recorded and current values (Δi = ib−ia) were plotted against the cholesterol concentration.
Results and discussion
In this study, we prepared a new hydrogen enzyme-based biosensor for the quantification of cholesterol. All parameters were examined. The electrochemical responses of the biosensor electrode to cholesterol were investigated. Immobilization of cholesterol oxidase upon of PPy–DBS film was carried out. Besides, we determined pH, temperature, operational and storage stabilities.
SEM (scanning electron microscope) studies of PPy–DBS film
In these experiments, SEM JEOL Model 6060 LV was used. The morphology of electrode was characterized by SEM ( and ). The SEM image of PPy–DBS is illustrated in . The SEM image () acquired for the Pt/PPy–DBS electrode demonstrates fine microspheres resulting in the porous surface. The SEM image of Pt/PPy–DBS–ChOx is given in .
AFM (atomic force microscope) studies of PPy–DBS film
In these experiments, AFM JEOL Model 6060 LV was used. The morphology of electrode was characterized by AFM ( and ). The AFM image of PPy–DBS is illustrated in . The AFM image () acquired for the Pt/PPy–DBS electrode demonstrates fine microspheres resulting in the porous surface. The AFM image of Pt/PPy–DBS–ChOx is given in .
Contact angle studies of PPy–DBS film
In these experiments, contact angle Kruss model DSA 100 was used. The hydrophilicity of electrode was characterized by contact angle ( and ). The contact angle picture of PPy–DBS is shown in . The contact angle image () acquired for the Pt/PPy–DBS electrode demonstrates it to be a hydrophobic surface. The contact angle of Pt/PPy–DBS–ChOx is given in . It has shown that enzyme electrode is more hydrophilic than free enzyme electrode. This state can be attributed to hydrophilic groups of protein.
Amperometric response measurements of Pt/PPy–DBS electrode
As the PPy–DBS coated electrode was designed to be utilized as a biosensor, the determination of its sensitivity to hydrogen peroxide is a result of enzymatic reactions. The results of amperometric response currents detected for Pt/PPy–DBS electrodes are illustrated in . This figure illustrates that the PPy–DBS electrode gives a great linear response.
The determination of working potential
After preparing Pt/PPy–DBS electrodes, oxidation of the hydrogen peroxide was examined at a different potential (). For this purpose, four different potentials (0.4, 0.5, 0.6, and 0.7 V) were examined. The biggest current value was achieved at 0.70 V. At high potentials, interferences caused by exogenous substances present in body fluids (e.g., ascorbic acid) (Zhang et al. Citation2007). Therefore, 0.4 V was selected as working potential.
The pyrrole concentration experiments
The electrodes sensitivity to hydrogen peroxide was studied to explain the important of pyrrole concentration (). For this reason, several pyrrole concentrations (0.05, 0.1, 0.15, and 0.2 M) were examined. The lowest correlation coefficient of electrode was found at 0.05 M pyrrole concentration. It was shown that high pyrrole concentration was not suitable for polymer film. Surface of these electrodes prepared by 0.15 M pyrrole was smoother and stronger than the other electrodes. Therefore, the pyrrole concentration was used as 0.15 M in the next trial.
The effect of DBS concentration
In this study, DBS was used as an anionic dopant. The sensitivities of electrodes to hydrogen peroxide were determined to clarify the role of DBS concentration. Three different DBS concentrations (0.05, 0.1, 0.15 M) were examined (). shows that correlation coefficients of film electrodes covered in 0.05 and 0.15 M DBS were lower than 0.10 M. The best linear response was appeared with the use of 0.05 M sodium DBS. All of the experiments were carried out with Pt/PPy–DBS electrodes prepared by maintaining the DBS concentration at 0.1 M.
The effect of cycle number of PPy–DBS film
Film thickness was determined using cycle numbers. The effects of film cycle number on the sensitivity of the electrodes against hydrogen peroxide concentration were examined for different cycles (). indicates that the electrodes prepared by 2, 4, 6, and 12 cycles have high linearity. When cycle numbers increase, film thickness grows up. Sensitivity decreases with increasing film thickness because it is proportional to conductivity. The highest response currents were obtained for electrodes prepared by 4 cycles. Moreover, this electrode has much more mechanical strength and reproducibility. The observed higher value of amperometric response current may be attributed to the thin film. Pt surface was too thick for the higher cycles. Therefore, amperometric response currents were poor. For the electrodes prepared by 4 cycles, the surface of the electrode was smoother and more durable than the others.
Amperometric response measurements of PPy/DBS–ChOx
Since the PPy–DBS coated electrode prepared was designed to be utilized as a biosensor, the determination of its sensitivity to hydrogen peroxide is a result of enzymatic reactions. The results of amperometric response currents detected for Pt/PPy–DBS–ChOx electrodes are illustrated in . The figure shows that the enzyme electrode was very sensitive to cholesterol. This enzyme electrode can be used to determine cholesterol in various samples.
pH dependence of the response of biosensor
The pH of buffer has a great influence on enzyme activity and the catalytic capacity for hydrogen peroxide reduction. The change in ionization state of amino acid side chain can perform critical functions in the enzyme active site; thus, the enzyme activity should change with the pH of buffer. The effect of the buffer pH on the amperometric response of biosensor was examined by using phosphate buffer solutions in a range of 6.0 ≤ pH ≤9.0 in the presence of 0.05 mM of cholesterol. The effect of solution pH on the cholesterol response at the proposed biosensor is illustrated in . The graph shows that the best pH for cholesterol biosensor is 7.0. This value is possible. At extreme pH values, the enzyme was denaturated. Therefore, pH 7.0 is selected by considering both the sensitivity and the stability of the biosensor and consequently further experiments were performed at pH 7.0. Besides, the phosphate buffer (0.1 M) was used as the buffer system for all other experiments.
Figure 14. The effect of pH on the response of the biosensor (in the phosphate buffer (pH 7.0) containing 50 μM cholesterol at 0.4 V working potential).
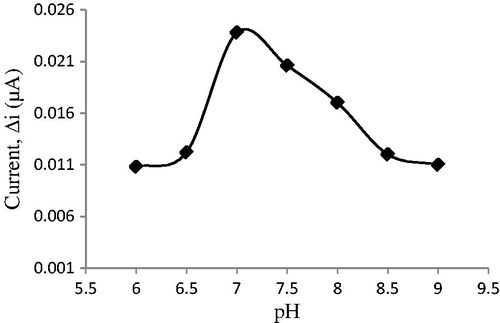
For cholesterol biosensor, optimum pH was reported to be 7 in the previous studies (Abdelwahab et al. Citation2010, Khan et al. Citation2008, Singh et al. Citation2004, Singh et al. Citation2011, Turkarslan et al. Citation2009, Zhao et al. Citation2008). However, pH values different from 7.0 were also used in literature (pH 7.5; 6.5; 6.8; 6.0) (Ahmad et al. Citation2010, Basu et al. Citation2007, Brahim et al. Citation2001, Singh et al. Citation2006). The difference in pH values was attributed to the fact that the used polymer and the type of immobilization were different, environment changes in the enzyme’s active site can cause different interactions between enzyme and immobilization materials (Ahmadalinezhad and Chen Citation2011, Gholivand and Khodadadian Citation2014, Tiwari and Gong Citation2008). Furthermore, enzymes become stronger after immobilization. Immobilized enzymes can show high activities under different pH conditions when compared with free enzyme and may form the stable substrate–enzyme complex.
Temperature dependence of the response of biosensor
Temperature exerts a marked influence on the enzymatic activity as well as on the performance of the amperometric cholesterol biosensor employed in this work, the sensitivity of which increases at lower temperatures. The effect of this parameter on the sensor response was evaluated in the range 20–70 °C. As illustrated in , the current response gradually increased with increasing temperature and reached a maximum at 50 °C. This value is higher than those in many other studies (Basu et al. Citation2007, Chauhan et al. Citation2010, Singh et al. Citation2004, Singh et al. Citation2006, Turkarslan et al. Citation2009). This was attributed to the fact that the used polymer and immobilization materials and the type of immobilization were different. Additionally, immobilized enzymes can show high activity under temperatures different from physiological conditions compared to that of free enzymes. Enzyme can be denaturated after a long incubation period at a temperature of 50 °C. However, for practical convenience, all other experiments were carried out at 25 °C.
Effect of substrate amount on the response of biosensor
The value of the Michaelis–Menten kinetic parameter (Km), which shows the enzyme–substrate kinetics, was determined by analyzing the slope of enzymatic reaction. Vmax is the maximum rate for enzymatic reaction. The effect of the substrate concentration on the reaction rate, catalyzed by immobilized ChOx, was studied using varying initial concentration (0.1 μM–1 mM) of cholesterol substrate (). PPy–DBS film, used in this study, increased the affinity of enzyme to cholesterol. Km(app) constant and Imax, a specific parameter of enzymes, was found out using 1/[ACh] – 1/Δi graph (Lineweaver–Burke plot; ). With the increase in substrate concentration, there was an increase in amperometric current signal. Kinetic parameters Km(app) and Imax for the enzyme biosensor were detected at constant temperature (25 °C) and pH 7.0 while varying the substrate concentration. Km(app) and Imax were calculated as 0.11 μM and 0.967 nM/min, respectively. Km can be understood from the Km(app) value, which is lower than the Km(app) values in literature (Khan et al. Citation2008, Matharu et al. Citation2007, Singh et al. Citation2004, Singh et al. Citation2011).
Operational stability and storage stability
In order to test the operational stability of the prepared enzyme electrode, the current changes, obtained after subsequent use, were plotted against the number of measurements () . By the end of the 30 measurements, the biosensor had lost 10.0% of its initial activity. The operational stability was examined by applying activity assay (under optimum conditions) for 30 times in the same day at a constant temperature, pH, and substrate concentration.
Figure 18. Operational stability of the biosensor in pH 7.0 phosphate buffer, 50 μM cholesterol 25 °C, 0.4 V working potential.
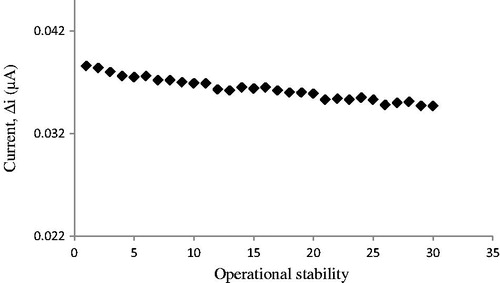
Response of the enzyme electrode, prepared under optimum conditions, was measured for a period of 48 days at a constant cholesterol concentration (50 μM). Results of nine measurements during this period are plotted in . In general, an enzyme is not stable in aqueous solution during storage and the activity is gradually reduced (Hendji et al. Citation1993). The reusability was tested because of its importance for repeated applications in a batch reactor. The main advantage of reusability is to reduce the cost of the treatment. The electrode showed 36% of the initial amperometric response at the end of the 30 days.
Interference effects
The influence of electrochemical interferents on the current response of cholesterol was evaluated at Pt/PPy–DBS–ChOx biosensor. A few common substances found in serum or urine were studied for any interfering effect on the analysis of cholesterol. Known concentrations of ascorbic acid, uric acid, glucose, and paracetamol (acetoaminophen) were added. The results are shown in , which represents the amperometric responses of cholesterol (50 μM), in the presence of glucose (5 mM), uric acid (0.1 mM), paracetamol (0.1 mM), ascorbic acid (0.1 mM) in PBS (pH 7.0) solution. These interferences were almost removed by diluting the solution in cell. Thus, Pt/PPy–DBS–ChOx biosensor exhibited high selectivity for the determination of cholesterol.
Table 1. Interfering substances on the amperometric response of the biosensor.
Conclusion
In this study, we report a new enzyme-based amperometric biosensor for the determination of cholesterol. ChOx was physically entrapped onto a PPy–DBS film surface. All parameters were examined. Sodium DBS was used as an anionic dopant. The experimental results showed clearly that the biosensor exhibited good performance in the determination of cholesterol. It has a very low detection limit and an acceptable response time for a biosensor. Herein prepared cholesterol biosensor can be used in a large concentration range. Hence, cholesterol determination can be made in a variety of different samples with our biosensor. The biosensor had a minimum detectable concentration of 10 nM. It was seen that the cholesterol biosensor was highly sensitive, selective and its operational stability was found to be very good. Thus, cholesterol determination can be made with our biosensor repeatedly. The cholesterol biosensor suggested in this study is easy to prepare and is highly cost-effective. This composite (PPy–DBS) can supply a biocompatible and electrochemical microenvironment for immobilization of the enzyme, making this material a good candidate for the fabrication of highly sensitive and selective cholesterol biosensors.
Funding information
The authors are very grateful for the financial support of Gazi University Research Fund (Project no: 05/2013-04).
Disclosure statement
The authors report no conflicts of interest. The authors alone are responsible for the content and writing of the paper.
References
- Abdelwahab AA, Won MS, Shim YB. 2010. Direct electrochemistry of cholesterol oxidase immobilized on a conducting polymer: application for a cholesterol biosensor. Electroanalysis. 22:21–25.
- Ahmad M, Pan C, Gan L, Nawaz Z, Zhu J. 2010. Highly sensitive amperometric cholesterol biosensor based on Pt-incorporated fullerene-like ZnO nanospheres. J Phys Chem C. 114:243–250.
- Ahmad R, Tripathy N, Hahn YB. 2012. Wide linear-range detecting high sensitivity cholesterol biosensors based on aspect-ratio controlled ZnO nanorods grown on silver electrodes. Sens Actuators B: Chem. 169:382–386.
- Ahmadalinezhad A, Chen A. 2011. High-performance electrochemical biosensor for the detection of total cholesterol. Biosens Bioelectron. 26:4508–4513.
- Ansari AA, Kaushik A, Solanki PR, Malhotra BD. 2008. Sol–gel derived nanoporous cerium oxide film for application to cholesterol biosensor. Electrochem Commun. 10:1246–1249.
- Arya SK, Datta M, Malhotra BD. 2008. Recent advances in cholesterol biosensor. Biosens Bioelectron. 23:1083–1100.
- Arya SK, Prusty AK, Singh SP, Solanki P, Pandey MK, Datta M, Malhotra BD. 2007. Cholesterol biosensor based on N-(2-aminoethyl)-3-amino propyl trimethoxysilane self-assembled monolayer. Anal Biochem. 363:210–218.
- Basu AK, Chattopadhyay P, Roychoudhuri U, Chakraborty R. 2007. Development of cholesterol biosensor based on immobilized cholesterol esterase and cholesterol oxidase on oxygen electrode for the determination of total cholesterol in food samples. Bioelectrochemistry. 70:375–379.
- Bongiovanni C, Ferri T, Poscia A, Varalli M, Santucci R, Desideri A. 2001. An electrochemical multienzymatic biosensor for determination of cholesterol. Bioelectrochemistry. 54:17–22.
- Brahim S, Narinesingh D, Guiseppi-Elie A. 2001. Amperometric determination of cholesterol in serum using biosensor of cholesterol oxidase contained within a polypyrrole-hydrogel membrane. Anal Chim Acta. 448:27–36.
- Çete S, Bal Ö. 2013. Preparation of Pt/polypyrrole–para toluene sulfonate hydrogen peroxide sensitive electrode for the utilizing as a biosensor. Artif Cells Nanomed Biotechnol. 41:414–420.
- Cete S, Yasar A, Arslan F. 2006. An amperometric biosensor for uric acid determination prepared from uricase immobilized in polypyrrole film. Artif Cells Blood Substit Immobil Biotechnol. 34:367–380.
- Çete S, Yaşar A, Arslan F. 2007. Immobilization of uricase upon polypyrrole-ferrocenium film. Artif Cells Blood Substit Immobil Biotechnol. 35:607–620.
- Charpentier L, Murr NE. 1995. Amperometric determination of cholesterol in serum with use of a renewable surface peroxidase electrode. Anal Chim Acta. 318:89–93.
- Chauhan N, Narang J, Pundir CS. 2010. Amperometric determination of serum cholesterol with pencil graphite rod. Am J Anal Chem. 2:41–46.
- Dolmaci N, Cete S, Arslan F, Yasar A. 2012. An amperometric biosensor for fish freshness detection from xanthine oxidase immobilized in polypyrrole–polyvinylsulphonate film. Artif Cells Blood Subst Biotechnol. 40:275–279.
- Feng B, Liu YN. 2015. A disposable cholesterol enzyme biosensor based on ferrocene-capped gold nanoparticle modified screen-printed carbon electrode. Int J Electrochem Sci. 10:4770–4778.
- Gholivand MB, Khodadadian M. 2014. Amperometric cholesterol biosensor based on the direct electrochemistry of cholesterol oxidase and catalase on a graphene/ionic liquid-modified glassy carbon electrode. Biosens Bioelectron. 53:472–478.
- Gorgulu M, Cete S, Arslan H, Yasar A. 2013. Preparing a new biosensor for hypoxanthine determination by immobilization of xanthine oxidase and uricase in polypyrrole–polyvinyl sulphonate film. Artif Cells Blood Subst Biotechnol. 41:327–331.
- Gros P, Durliat H, Comtat M. 2000. Use of polypyrrole film containing Fe(CN)63− as pseudo-reference electrode: application for amperometric biosensors. Electrochim Acta. 46:643–650.
- Guiseppi-Elie A, Wallace GG, Matsue T. 1995. In: Skotheim T, Elsenbaumer R, Reynolds JR, Eds. Handbook of Conductive Polymers. New York: Marcel Dekker.
- Hendji AN, Bataillard P, Renault NJ. 1993. Covalent immobilization of glucose oxidase on silanized platinum microelectrode for the monitoring of glucose. Sens Actuators B. 15:127–134.
- Khan R, Kaushik A, Solanki PR, Ansari AA, Pandey MK, Malhotra BD. 2008. Zinc oxide nanoparticles-chitosan composite film for cholesterol biosensor. Anal Chim Acta. 616:207–213.
- Kim JH, Cho JH, Sig Cha G, Lee CW, Kim HB, Paek SH. 2000. Conductimetric membrane strip immunosensor with polyaniline-bound gold colloids as signal generator. Biosens Bioelectron. 14:907–915.
- Krug A, Gobel R, Kellner R. 1994. Flow-injection analysis for total cholesterol with photometric detection. Anal Chim Acta. 287:59–64.
- Matharu Z, Sumana G, Arya SK, Singh SP, Gupta V, Malhotra BD. 2007. Polyaniline Langmuir–Blodgett film based cholesterol biosensor. Langmuir. 23:13188–13192.
- Moraes ML, de Souza NC, Caio OH, Marystela F, Ubirajara P, Rodrigues F Jr, et al. 2009. Immobilization of cholesterol oxidase in LbL films and detection of cholesterol using ac measurements. Mater Sci Eng C. 29:442–447.
- Muhammed M, Cete S, Arslan F, Yasar A. 2009. Amperometric cholesterol biosensors based on the electropolymerization of pyrrole and aniline in sulphuric acid for the determination of cholesterol in serum. Artif Cells Blood Substit Immobil Biotechnol. 37:273–278.
- Nauck M, Marz W, Jarausch J, Cobbaert C, Sagers A, Bernard D, et al. 1997. Multicenter evaluation of a homogeneous assay for HDL-cholesterol without sample pretreatment. Clin Chem. 43:1622–1629.
- Nauck M, Marz W, Wieland H. 2000. Is lipoprotein(a) cholesterol a significant indicator of cardiovascular risk? Clin Chem. 46:436–437.
- Rahman MM, Asiri AM. 2015. One-step electrochemical detection of cholesterol in the presence of suitable K3Fe(CN)6/phosphate buffer mediator by an electrochemical approach. Talanta. 140:96–101.
- Ram MK, Bertoncello P, Ding H, Paddeu S, Nicolini C. 2001. Cholesterol biosensors prepared by layer by layer technique. Biosens Bioelectron. 16:849–856.
- Shin MC, Yoon HC, Kim HS. 1996. In situ biochemical reduction of interference in an amperometric biosensor with a novel heterobilayer configuration of polypyrrole/glucose oxidase/horseradish peroxidase. Anal Chim Acta. 329:223–230.
- Singh J, Kalita P, Singh MK, Malhotra BD. 2011. Nanostructured nickel oxide-chitosan film for application to cholesterol sensor. Appl Phys Lett. 98:123702.
- Singh S, Chaubey A, Malhotra BD. 2004. Amperometric cholesterol biosensor based on immobilized cholesterol esterase and cholesterol oxidase on conduction polypyrrole films. Anal Chim Acta. 502:229–234.
- Singh S, Solanki PR, Pandey MK, Malhotra BD. 2006. Covalent immobilization of cholesterol esterase and cholesterol oxidase on polyaniline films for application to cholesterol biosensor. Anal Chim Acta. 568:126–132.
- Soylemez S, Kanik FE, Ileri M, Hacioglu SO, Toppare L. 2014. Development of a novel biosensor based on a conducting polymer. Talanta. 118:84–89.
- Soylemez S, Kanik FE, Nurioglu AG, Akpinar H, Toppare L. 2013. A novel conducting copolymer: investigation of its matrix properties for cholesterol biosensor applications. Sens Actuators B: Chem. 182:322–329.
- Soylemez S, Kanik FE, Tarkuc S, Udum YA, Toppare L. 2013. A sepiolite modified conducting polymer based biosensor. Colloids Surf B Biointerfaces. 111:549–555.
- Srivastava M, Srivastava SK, Nirala NR, Prakash R. 2014. A chitosan-based polyaniline Au nanocomposite biosensor for determination of cholesterol. Anal Methods. 6:817–824.
- Teles FRR, Fonseca LP. 2008. Applications of polymers for biomolecule immobilization in electrochemical biosensors. Mater Sci Eng: C. 28:1530–1543.
- Tiwari A, Gong S. 2008. Electrochemical study of chitosan-SiO2-MWNT composite electrodes for the fabrication of cholesterol biosensors. Electroanalysis. 20:2119–2126.
- Turkarslan O, Kayahan SK, Toppare L. 2009. A new amperometric cholesterol biosensor based on poly(3,4-ethylenedioxypyrrole). Sens Actuators B: Chem. 136:484–488.
- Umar A, Rahman MM, Vaseem M, Hahn YB. 2009. Ultra-sensitive cholesterol biosensor based on low-temperature grown ZnO nanoparticles. Electrochem Commun. 11:118–121.
- Valentini F, Orlanducci S, Terranova ML, Amine A, Palleschi G. 2004. Carbon nanotubes as electrode materials for the assembling of new electrochemical biosensors. Sens Actuators B: Chem. 100:117–125.
- Yao T, Satomura M, Nakahara T. 1995. Simultaneous assays of glucose, urate, and cholesterol in blood serum by amperometric flow injection analysis. Electroanalysis. 7:143–146.
- Yıldırımoğlu F, Arslan F, Çete S, Yaşar A. 2009. Preparation of a polypyrrole–polyvinlysulphonate composite film biosensor for determination of cholesterol based on entrapment of cholesterol oxidase. Sensors. 9:6435–6445.
- Yu J, Ju H. 2002. Preparation of porous titania sol–gel matrix for immobilization of horseradish peroxidase by a vapor deposition method. Anal Chem. 74:3579–3583.
- Zhang Y, Wen G, Zhou Y, Shuang S, Dong C, Choi MMF. 2007. Development and analytical application of an uric acid biosensor using an uricase-immobilized eggshell membrane. Biosens Bioelectron. 22:1971–1797.
- Zhao C, Wan L, Jiang L, Wang Q, Jiao K. 2008. Highly sensitive and selective cholesterol biosensor based on direct electron transfer of hemoglobin. Anal Biochem. 383:25–30.
- Zhou N, Wang J, Chen T, Yu Z, Li G. 2006. Enlargement of gold nanoparticles on the surface of a self-assembled monolayer modified electrode: a mode in biosensor design. Anal Chem. 78:5227–5230.