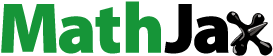
Abstract
Curcumin is more soluble in ethanol, dimethylsulfoxide, methanol and acetone than in water. In this study, nanocurcumin combined with 8 mT AC static magnetic field was used to enhance cellular uptake, bioavailability, and ultimate efficiency of curcumin against prostate cancer cell line (PC3), four bacteria strains (two Gram positive: Micrococcus luteus ATCC 9341, Staphylococcus aureus ATCC 29213 and two Gram negative: Escherichia coli ATCC 25922, Pseudomonas aeruginosa ATCC 27853), mammalian cell line (HEK) and human erythrocytes (RBC). The efficiency (E%) between IC50 of nanocurcumin combined with magnetic field (NANOCUR-MF) and control against PC3 was 35.93%, which is three times higher compared to curcumin combined with magnetic field (CUR-MF); i.e., 10.77%. However, their E% against HEK was not significant; 1.4% for NANOCUR-MF and 1.95% for CUR-MF. Moreover, depending in minimum bacterial concentration (MBC), the use of MF leads to a reduction of MBCs for all tested bacteria compared with control. The obtained results established the applicability of (MF) in enhancing cellular uptake for PC3 and tested bacteria strains by increasing the penetration of drug (nanocurcumin and parent curcumin) into cell with fixing mild cytotoxic profile for HEK and RBC.
Introduction
Curcumin, commonly called diferuloylmethane, is a hydrophobic polyphenol derived from the rhizome (turmeric) of the herb curcuma longa. Turmeric has been used traditionally for many ailments due to its wide spectrum of pharmacological activities. Curcumin has been identified as the active principle of turmeric (Anand et al. Citation2008).
Cancer and normal cells have a different cell–cell communication, repair mechanisms, characteristic cell death, or other cellular activities (Shaw et al. Citation2014). Recently, curcumin has shown a strict capability to kill cancer cell line, where it demonstrated death being more pronounced in cancer than in normal cells (Sa and Das Citation2008). Moreover, preparing nanoparticles of curcumin (NANOCUR) leads to further enhancement of the uptake into the cells, which in turn leads to increase preferential cytotoxicity to cancer cells (Mukerjee and Vishwanatha Citation2009). Other studies indicated that MF appeared ability to inhibit the growth of many types of human tumor cell lines in vitro (Casciari and Riordan Citation2001, Raylman et al. Citation1996). On the other hand, the exposure to static magnetic field alone has no or extremely small effects on cell growth and genetic toxicity regardless of the magnetic density. Nevertheless, when combined with other external factors such as ionizing radiation and some chemicals drugs, it was evident that a static magnetic field modifies their effects (Miyakoshi Citation2005).
Moreover, other research works have been devoted to examine the effect of MF on human erythrocytes (Chionna et al. Citation2009, Elblbesy Citation2010) where Elblbesy (Citation2010) reported that SMF might increase the erythrocytes adhesion, hence erythrocytes aggregation. Likewise, another study indicated that exposure of animals to 0.2 mT magnetic fields (50 Hz) resulted in a decrease of RBCs membrane elasticity and permeability as well as changes in the molecular structure of hemoglobin (Hb) (Ali et al. Citation2003).
Moreover, the magnetic field could be used as an inhibitory factor against the bacteria such as sterilization of food products. Otherwise, the study devoted to the effect of static magnetic field on Pseudomonas aeruginosa indicated that the magnetic field decreased the growth rate of the bacteria, while increased the logarithmic phase within 4 h of exposure (Kamel et al. Citation2013). On the other hand, Feng (Citation2004) reported that the viability decreases with longer exposure time and/or higher induction Bm for all strains, but the quantity of the effect is strain-dependent.
So far, several studies have demonstrated that curcumin is very active as anti-tumor and antimicrobial agent, but being water-soluble and due to its poor bioavailability, it presents some disadvantages. This has encouraged some researchers to use curcumin as nanoparticles (Basniwal et al. Citation2011) in order to enhance its solubility and bioavailability. On the other hand, Miyakoshi (Citation2005) reported that exposure to static magnetic fields alone has no or extremely small effects on cell growth and genetic toxicity regardless of the magnetic density. Nevertheless, when combined with other external factors (ionizing radiation and some chemicals drugs), no evidence suggesting that a static magnetic field modifies their effects.
Many previous studies indicate that curcumin as an excellent safe drug, has a great potential for prevention and/or treatment of cancer and other chronic diseases. However, it could not achieve the optimum therapeutic outcome in the previous reported clinical studies, generally due to its low solubility and poor bioavailability (Basnet and Skalko-Basnet Citation2011). The aim of this research work consists of (i) improving curcumin anti-cancer and anti-microbial activities combined with fixing its mild cytotoxic profile against mammalian cell line HEK and human erythrocytes (curcumin efficiency as an anti-cancer and anti-microbial agent); (ii) enhancement of absorption, cellular uptake, and bioavailability of curcumin by preparing curcumin nanoparticles (wet-milling technique); (iii) increase membrane-permeation by using magnetic field with better drug penetration into cells.
Materials and methods
Preparation of curcumin nanoparticles
Curcumin nanoparticles were prepared by wet-milling process as reported previously (Adahoun et al. Citation2016). Environmental scanning electron microscopy (ESEM) (Quanta 450 FEG, Hillsboro, OR) was used to characterize the morphology and size of the nanoparticles. Small amount of the powder was spread over a carbon tab, then dried by nitrogen stream. The obtained ESEM revealed agglomerates and it was hard to define the shape and size of the nanoparticles. The powder was then coated by platinum (Pt). The ESME was adjusted and calibrated under 15 kV and working distance 9.2 mm; an image was taken using a magnification 60,000× and scale bar 1 μm.
Cell proliferation assay
The cytotoxicity of nanocurcumin and parent curcumin against cultured cells was determined by the MTT assay and both of PC3 and HEK cell line were seeded and performed as reported previously (Adahoun et al. Citation2016). The plates were incubated for 1 h at 37 °C under 5% CO2, and then divided into two groups, where the first group was exposed to 6 h at (8 mT) MF and second group remained unexposed and served as control group (were located at a 5 m distance from MF under the same conditions). Then, all plates were incubated at 37 °C under 5% CO2 for 24 h. After incubated 24 h, 20 μL of 5 mg/mL of 3-(4,5-dimethylthiazol-2-yl)-2,5-diphenyltetrazolium bromide (MTT) (Santa Cruz Biotechnology, Santa Cruz, CA) was added and incubated for 4 h. After replaced by 200 μL of dimethylsulfoxide (DMSO) (AZ Chem, Jacksonville, FL), the absorbance was measured using an ELISA Microplate Reader (BioTek, Shoreline, WA) at 550 nm.
Erythrocyte hemolysis assay
The determination of the hemolytic activity of nanocurcumin and parent curcumin as well as the preparation of drugs solution (nanocurcumin and parent curcumin stock solution), were performed as reported previously (Adahoun et al. Citation2016). Then the RBC's samples divided to two groups, first group from erythrocyte suspension exposed with both nanocurcumin and curcumin to 8 mT magnetic field 60 min, where the samples fixed in the geometrical center of the solenoid magnet. The second group used as control was kept at a distance of 5 m from the magnetic field. The cells were then centrifuged at 900 × g for 5 min until the supernatant was separated from the pellet. About 200 μL were transferred from each sample supernatant as four replicates into a 96-well plate and their absorbance measured at 570 nm. 0.9% NaCl was used as the negative control, 0.1% Triton X-100 as the positive control. The percentage hemolysis was calculated using the following equation:
(1)
(1)
where A is OD 570 nm with the drug solution, A° is OD 570 nm for drug alone without cells (control drug solution). A0 is OD 570 nm in NaCl, and AX is OD 570 nm with 0.1% Triton X-100.
Bacteria strains and minimum bactericidal concentration
The bacterial strains used for the determination and testing of the antimicrobial activity of nanocurcumin and parent curcumin (Susceptibility testing), by evaluating the minimum bacteria concentration (MBC) against four bacteria strains including two Gram positive (Micrococcus luteus ATCC 9341, Staphylococcus aureus ATCC 29213) and two Gram negative (Escherichia coli ATCC 25922, Pseudomonas aeruginosa ATCC 27853), were performed as reported previously (Adahoun et al. Citation2016). The control wells for parent curcumin and nanocurcumin contain 10 μl from bacteria strain and 190 μl nutrient broth to reach a final volume of 200 μl and each test was carried out in triplicate. The samples were incubated 1 h at 37 °C, and then divided into two groups. For the first group, the microplate was exposed 24 h to 8 mT MF while the second group serving as control, remained unexposed at 5 m distance from the magnetic field (Ruiz-Gomez et al. Citation2004). This stage of our experiments was carried out at room temperature (24–26 °C) and at the similar environmental conditions. Then 10 μl of each well was inoculated onto nutrient agar plate and incubated at 37 °C for 24 h. The bacterial colonies were enumerated and the average number of colony counts for each group was determined. The inhibition was calculated using the following formula:
(2)
(2)
where T is colony forming unit of the test sample (cfu/mL) and C is cfu/mL of the control. The MBC value was defined as the lowest concentration of antimicrobial agent that can kill >99% of the microorganism population (Basri et al. Citation2012).
Magnetic field
Sinusoidal AC 8 mT Magnetic field 50 Hz (MF; length 33.5 cm and diameter 15 cm) as shown in , was used to examine the effect of MF with combined parent curcumin CUR-MF and nanocurcumin NANOCUR-MF against all cancer cells (PC3), normal cells (HEK), human erythrocytes (RBCs), and four selected bacteria strains. The solenoid was set on supports made of non-magnetic material, and the samples were fixed in the geometrical center of the solenoid magnet (where the uniform magnetic field lines exist). The temperature near the cultured plates was monitored and no variation was recorded throughout the experiments.
Statistical analysis
The data were processed using Kaleidagraph software version 4.02 (Synergy Software, Reading, PA) and presented as the mean ± standard error of the mean. Statistical analysis was performed using SPSS version 21 (SPSS Software Inc., Chicago, IL). Data were shown as the mean values calculated from all experiments. All comparisons between the experimental groups were performed by AKNOVA test. p values less than 5% were considered to be statistically significant.
Results
Characterization of curcumin nanoparticles
First, small amount of the powder was spread over a carbon tab then dried by nitrogen stream. ESEM image revealed the existence agglomerates containing nanoparticles, hence it was difficult to determine the size. After that, the powder was coated by platinum (Pt). The new ESEM image () showed clearly the formation of nanoparticles with an average size in the range 34–359 nm.
The effect magnetic field on cell lines
Sinusoidal AC 8 mT magnetic field (MF) was used to examine the effect MF with combined nanocurcumin NANOCUR-MF and parent curcumin CUR-MF. IC50 values for both nanocurcumin and parent curcumin were summarized in .
Table 1. IC50 values for NANOCUR-MF and CUR-MF.
shows the cell viability percentage of PC3 prostate cancer after treatment by nanocurcumin (NANOCUR-MF) compared with control. It can be seen that the cell viability of NANOCUR-MF is lower than control at all concentrations. Overall, the viability of both NANOCUR-MF and control samples decreases with increasing the concentration. There is a significant difference between NANOCUR-MF and control groups at all concentrations (p < 0.05). However, also shows that the difference between two error bars of both NANOCUR-MF and control is clear at concentrations (50, 100, and 450 μM) than other concentrations. The IC50 for NANOCUR-MF is 221.67 μM which is smaller than 346 μM for control; the IC50 efficiency between NANOCUR-MF and control is 35.93%, which clearly indicates that NANOCUR-MF has good effect on PC3.
Figure 3. (A) Viability of PC3 cancer cells for NANOCUR-MF at different nanocurcumin concentrations versus control sample. (B) Viability of PC3 cancer cells for CUR-MF at different curcumin concentrations versus control sample. The Y-axis indicates the cell viability at λ = 550 nm, and the X-axis corresponding to curcumin concentration. Data represent three independent experiments.
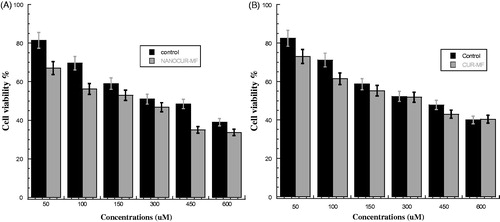
shows the cell viability percentage of PC3 prostate cancer after treatment by CUR-MF compared with control. Some differences between the two samples can be noticed. Overall, the viability of both CUR-MF and control samples decreases with increasing the concentration. It can be seen that the cell viability of CUR-MF is lower than control at all concentrations except at highest concentration 600 μM, which is almost same and that could be due to the saturation threshold for the cells. There is a significant difference between CUR-MF and control groups at all concentrations (p < 0.05). Moreover, from , we can notice that the difference between two error bars of both CUR-MF and control is clear at lower concentrations (50 and 150 μM) than other concentrations. The IC50 for CUR-MF was 331.3 μM compared with 371.3 μM for control, and the MF efficacy to reduce the cell viability (E%) is 10.77% between the IC50 of CUR-MF and control. This indicates that there is a small effect of magnetic field on PC3 cancer cells after exposure for 6 h.
shows the cell viability percentage of HEK after treatment by NANOCUR-MF compared with control. The viability for NANOCUR-MF is found lower than the viability of control at all concentrations except at concentration 600 μM. In addition, there is a significant difference between NANOCUR-MF and control groups at all concentrations (p < 0.05). However, from , we can observe that the difference between two error bars of both CUR-MF and control is clear at low concentrations (50, 100, and 150 Mμ) than other concentrations. However, it can be noticed that the viability decreases with increasing concentration for both NANOCUR-MF and control, and almost in the same trend. The IC50 for NANOCUR-MF is 390.8 μM compared with 396.7 μM for control, and the E% at IC50 is then 1.4%, which means once again no significant effect of NANOCUR-MF on HEK cells.
Figure 4. (A) Viability of HEK cancer cells for NANOCUR-MF at different nanocurcumin concentrations versus control sample. (B) Viability of HEK cancer cells for CUR-MF at different curcumin concentrations versus control sample. The Y-axis indicates the cell viability at λ = 550 nm, and the X-axis corresponding to curcumin concentration. Data represent three independent experiments.
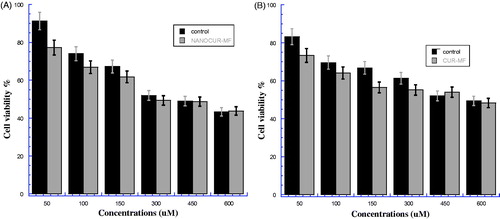
shows the cell viability percentage of HEK after treatment by CUR-MF compared with control. There is a significant difference between the CUR-MF and control samples at all concentrations on viability in favor of CUR-MF (p < 0.05). Moreover, we can notice that the difference between two errors bars of both CUR-MF and control is unclear at high concentrations (450 and 600 μM) than lower concentrations. The viability of CUR-MF is found smaller than control at all concentrations except at 450 μM. In addition, the viability decreases with increasing concentration for both CUR-MF and control, and almost in the same trend. The IC50 for CUR-MF is 556.11 μM which is slightly smaller than 567.2 μM for control. The IC50 efficiency between CUR-MF and control is quite small about 1.95%, which means that there is no significant effect of CUR-MF on HEK normal cells.
The effect magnetic field on human erythrocytes
Sinusoidal AC 8 mT magnetic field (MF) was used to examine the effect MF with combined nanocurcumin NANOCUR-MF and curcumin CUR-MF at five different concentrations (50, 100, 200, 400, and 600 μM) against Human Erythrocyte. In this test, the ability of the NANOCUR-MF and CUR-MF to lyse mammalian erythrocytes were assessed using fresh 4% (packed cell volume) defibrinated human red blood cells (RBCs) was investigated.
The effective magnetic field combined with nanocurcumin (NANOCUR-MF) after exposed 60 min was examined as shown in . There was significant difference between two samples groups, where p < 0.05 (p = 0.02). However, from the figure, we can see that the differences can be considered negligible (less significant), within the range of error bars for both samples at concentrations (50, 100, 200, and 400 μM). We can notice also that the difference between two bars of both NANOCUR-MF and control is clear at concentration 600 μM. The hemolysis percentage for NANOCUR-MF sample is slightly higher than control at all concentrations. The NANOCUR-MF hemolysis percentage did not reach to IC50 value up to high concentration, where the highest hemolysis percentage value was 23.769% ± 1.828 (20.657% ± 1.201 for control) with E% ∼13.093% at highest concentration (600 μM), which can be considered the highest E% value between all concentrations (). This means that the NANOCUR-MF has slight effect on RBCs after exposed 60 min.
Figure 5. (A) Hemolysis percentage for nanocurcumin with combined 8 mT magnetic field (NANOCUR-MF). Different concentrations of solutions (50, 100, 200, 400, and 600 μM) were incubated with 4% human erythrocytes suspension, Results were recorded at λ = 570 nm after exposed 60 min where p < 0.05 (p = 0.02). (B) Hemolysis percentage for curcumin with combined 8 mT magnetic field (CUR-MF). Different concentrations of solutions (50, 100, 200, 400 and 600 μM) were incubated with 4% human erythrocytes suspension. Results were recorded at λ = 570 nm after exposed 60 min where p < 0.05 (p = 0.024).
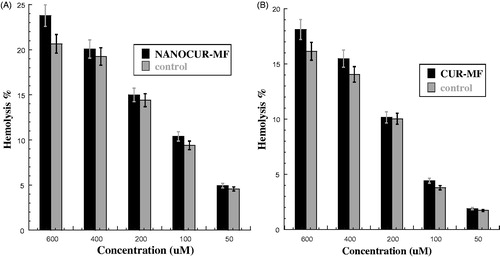
Table 2. Hemolytic effect of NANOCUR-MF versus control with efficiency percentage on human erythrocytes after 60 min of incubation.
The effective magnetic field combined with curcumin (CUR-MF) after exposed 60 min was examined as shown in . There was significant difference between two samples groups, where p < 0.05 (p = 0.024). However, we can see that the differences can be considered negligible (less significant), within the range of error bars for both samples at concentrations (50, 100, and 200 μM). Also, we can notice that the difference between two error bars of both CUR-MF and control is clear at concentrations 400 μM and 600 μM. The hemolysis percentage for CUR-MF sample is slightly higher than control at all concentrations. However, The CUR-MF hemolysis percentage did not reach to IC50 value up to high concentration; the highest hemolysis percentage was 18.112% ± 1.146 (16.136% ± 0.975 for control) with E% is ∼10.91% at highest concentration (600 μM) and the highest E% was ∼14.353 at concentration 100 μM (). This means that the CUR-MF has slight effect on RBCs after exposed 60 min.
Table 3. Hemolytic effect of CUR-MF versus control with efficiency percentage on human erythrocytes after 60 min of incubation.
The effect magnetic field on MBC
Bacteria are a microorganism that causes infectious disease. Therefore, the scientists put all efforts to decrease the bacterial resistance for cure (drugs) by improving these drugs with less toxicity and more ability to inhibit the bacterial growth. This work is devoted to assess CUR-MF and then NANOCUR-MF effects on some selected bacteria strains in order to improve the ability of curcumin to inhibit bacterial growth.
The bacterial strains used for the determination and examination of the antimicrobial activity of nanocurcumin when combined with magnetic field (NANOCUR-MF), by evaluated the minimum bacteria concentration (MBC) against four bacteria strain, two Gram positive: Micrococcus luteus, Staphylococcus aureus, two Gram negative: Escherichia coli, Pseudomonas aeruginosa. Then, the MBCs were summarized in (). shows that the inhibition percentage for all tested bacteria strains increased with increasing concentration in a dose dependent manner. On the other hand, the figure clearly shows that NANOCUR-MF has more ability to inhibit all tested bacteria strains compared to control. Moreover, we can notice that the differences between two error bars of both NANOCUR-MF and control were clear at low concentrations for all type of bacteria (), then it became slightly different at high concentration. Moreover, the differences between two error bars of both NANOCUR-MF and control were clear for Escherichia coli and Staphyloccus aureus () than Micrococcus luteus and Pseudomonas aeruginosa (). This means that the Staphyloccus aureus and Escherichia coli have more sensitivity to magnetic field. The MBCs value for NANOCUR-MF were 795.5, 616.89, 848.4, and 839.06 μg/ml, respectively, compared with 915.56, 822.86, 936.94, and 908.88 μg/ml for control with efficiency (E%) 13.11, 25.03, 9.45, and 7.68% for all of Micrococcus luteus, Staphylococcus aureus, Escherichia coli, and Pseudomonas aeruginosa, respectively (). Hence, the bacteria strains sensitivity for NANOCUR-MF is in the following order: Staphylococcus aureus > Micrococcus luteus > Escherichia coli > Pseudomonas aeruginosa. This means that, NANOCUR-MF displayed potent antimicrobial activities against all studied bacterial strains with Escherichia coli, Micrococcus luteus, Pseudomonas aeruginosa, and Staphyloccus aureus comparing with control. This can be due to MF and its activity on bacteria strains. Moreover, Selective Gram-positive displayed more sensitivity than selective Gram-negative for both NANOCUR-MF and control.
Figure 6. The bacteria growth after treatment by nanocurcumin combined with 8 mT magnetic field (NANOCUR-MF) at different concentrations then incubated overnight at 37 °C: (A) Escherichia coli, (B) Micrococcus luteus, (C) Pseudomonas aeruginosa, and (D) Staphyloccus aureus. The Y-axis represents the inhibition, and the X-axis corresponds to nanocurcumin concentrations. The results were repeated in triplicates. The standard deviation was in the range (0–1.64) for NANOCUR-MF samples, while was in the range (0.005–1.55) for control.
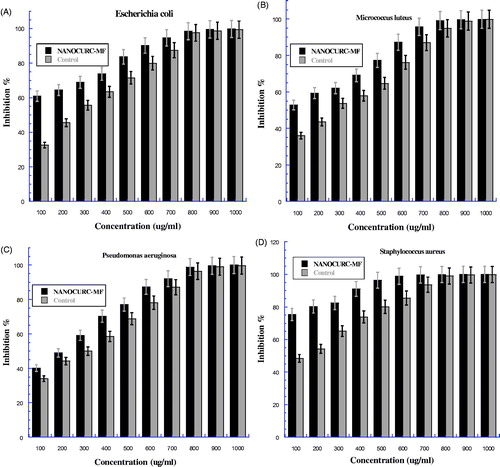
Table 4. Minimum bactericidal concentration (MBCs) of NANOCUR-MF comparing with control against the test microorganisms.
shows the bacterial strains used for the determination and examination of the antimicrobial activity of parent curcumin when combined with magnetic field (CUR-MF), by evaluated the minimum bacteria concentration (MBC) against four bacteria strain, two Gram-positive: Micrococcus luteus, Staphylococcus aureus, two Gram-negative: Escherichia coli, Pseudomonas aeruginosa. The MBCs were summarized in (). shows that, the inhibition percentage for all tested bacteria strains increased with increasing concentration in a dose dependent manner. Moreover, CUR-MF has more ability to inhibit all tested bacteria strains in comparison with control. The differences between two error bars of both CUR-MF and control were clear at low concentrations for all type of bacteria (), then it became slightly different at high concentration. Moreover, the differences were clear for the Staphyloccus aureus and Escherichia coli (), which mean they have more sensitivity to magnetic field. On the other hand, selective Gram-positive displayed more sensitivity than selective Gram-negative for both CUR-MF and control. The MBCs value for CUR-MF were 923.61, 865.11, 869.42, and 748.26 μg/ml, compared with 954.03, 934.83, 926.25, and 844.42 μg/ml for control with efficiency (E %) were 3.19, 7.46, 6.14, and 11.39 for all of Escherichia coli, Micrococcus luteus, Pseudomonas aeruginosa, and Staphylococcus aureus ATCC 29213, respectively (). This indicates that the bacteria strains sensitivity for CUR-MF follows this order: Staphylococcus aureus > Micrococcus luteus > Pseudomonas aeruginosa > Escherichia coli. This means that CUR-MF as well NANOCUR-MF displayed potent antimicrobial activities against all studied bacterial strains with Escherichia coli, Micrococcus luteus, Pseudomonas aeruginosa, and Staphyloccus aureus comparing with control. That is due to MF has an effective on bacteria strains.
Figure 7. The bacteria growth after treatment by curcumin combined with 8 mT magnetic field (CUR-MF) at different concentrations then incubated overnight at 37 °C: (A) present Escherichia coli, (B) Micrococcus luteus, (C) Pseudomonas aeruginosa, and (D) Staphyloccus aureus. The Y-axis represents the inhibition and the X-axis corresponds to curcumin concentrations. The results were repeated in triplicates. The standard deviation was in the range (0–1.91) for CUR-MF samples, while was in the range (0.138–1.56) for control.
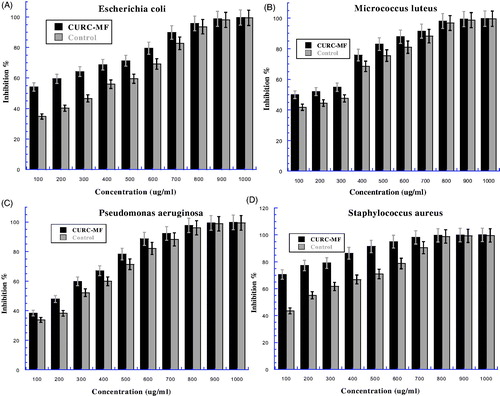
Table 5. Minimum bactericidal concentration (MBCs) of CUR-MF comparing with control against the test microorganisms.
Discussion
The effects of magnetic field (MF 8 mT) combined nanocurcumin (NANOCUR-MF) and parent curcumin (CUR-MF) were examined against PC3 and HEK cells after exposure for 6 h then compared with control ( and )). The NANOCUR-MF and CUR-MF mostly led to reduce cell viability in both type of cells in comparison with control. Where, the IC50 for PC3 treated with CUR-MF was 331.3 μM (371.3 μM for control) giving a small E% of 10.77%. For NANOCUR-MF, the IC50 was 221.67 μM (346 μM for control) giving a much higher E%, almost three times, with a value of 35.93% (). On the other hand, the IC50 for HEK treated by CUR-MF was 556.11 μM (567.2 μM for control), hence the E% is quite small; i.e., 1.95%. Moreover, the IC50 for HEK treated by NANOCUR-MF was 390.8 μM (396.7 μM for control) leading to much lower E% of 1.4%. This means that there is no effect of NANOCUR-MF to kill HEK cells.
By taking into account both IC50 and E%, it can be demonstrated that both NANOCUR-MF and CUR-MF have effect to increase the death of PC3 cells compared with control. However, depending on the efficiency, NANOCUR-MF has great effect E% (35.93%) on PC3 than CUR-MF (10.77%), which indicates that the reduction of curcumin particle size (nanocurcumin) combined with magnetic field (NANOCUR-MF) is more effective to kill PC3 than CUR-MF. This is due to an increase in the membrane permeability leading to an increase in nanocurcumin penetration into cellular much more than curcumin; due to better bioavailability and efficacy of nanocurcumin compared to curcumin. On the other hand, both NANOCUR-MF and CUR-MF have little effect in increasing HEK cell death. In conclusion, the MF leads to an increase of curcumin activity (efficiency) as an anti-cancer agent. While MF leads to a decrease in cell viability percentage for cancer cells PC3 treated by both nanocurcumin and curcumin compared with control, which is due to a better penetration of both nanocurcumin and curcumin into cellular.
In spite of the effects in several biosystems of moderate-intensity static MF (8 mT used in this study), the reported results are still controversial (Pagliara et al. Citation2009). Many previous studies have demonstrate no significant effect on cancer and normal cell lines, and reported that there is no statistically significant differences in the cell growth rate between control and exposed cells. Some other studies revealed that there are no differences in cell morphology or orientation (Gamboa et al. Citation2007, Yoshie et al. Citation2011), while other studies reported that MF has little or no effect on the expression of cytoskeletal, stress proteins and on proliferation in cultured astroglial cells (Bodega et al. Citation2005). Furthermore, most of studies in the literature report inconsistent and controversial results and it was difficult to present some clear explanations. However, something very important that need to be highlighted is that, species-dependent effect, cell-dependent effect, the type, intensity and frequencies of MF, as well as the duration of exposure used in these protocols, were very different and hence must be taken into account when comparing the results (Bodega et al. Citation2005). Therefore, the results obtained in this study are found in general, in good agreement with other studies (Casciari and Riordan Citation2001, Raylman et al. Citation1996, Sa and Das Citation2008). This indicates that the plasma membrane is a very sensitive target for the action of MF. Moreover, it also indicates that the MF of very low intensity can generate cell modifications by the described experiments with the use of 6 mT (here we used 8) magnet, and in spite of that it may not be enough high magnetic field that is able to produce some changes in the cell function and structure (Chionna et al. Citation2009). It is worth mentioning that the results obtained in this study once again are in good agreement with the study reporting changes in human lymphocytes and U937 cells due to a static magnetic field (Chionna et al. Citation2009). In addition, the results of this study are also in good agreement with (Miyakoshi Citation2005) study, which reported that exposure to static magnetic fields alone has no or extremely small effects on cell growth and genetic toxicity regardless of the magnetic density. Nevertheless, when combined with other external factors (ionizing radiation and some chemicals drugs), no evidence suggesting that a static magnetic field modifies their effects. Our present finding supports the results of some previous studies which underlined that the killing effect of antineoplastic drugs on cancer cells is enhanced with a combined treatment of SMF and chemotherapeutic drugs, indicating that SMF acts synergically with the pharmacological treatment. The authors reported that the killing effects of SMF combined with antineoplastic drugs on cancer cells are greater than those of SMF or anticancer drugs taken separately (Hao et al. Citation2011, Okonogi et al. Citation1996, Sabo et al. Citation2002).
The possible explanation for all the bio-effects attributed to moderate SMF could be due to the effects of these fields on the molecular structure of excitable membranes, consequently, the function of embedded ion-specific channels is modified, which confirms that the plasma membrane is the primary site of action of SMF (Pagliara et al. Citation2009). The authors have predicted that the synergistic action of SMF was related to the effect of the magnetic field on tumor cell membranes (Gray et al. Citation2000, Tofani et al. Citation2002), where SMF influenced tumor cells by altering their membrane permeability and increasing the flow rate of anticancer drugs (Liu et al. Citation2011). And because SMF can influence the basic cellular processes, then it is evident that fast growing malignant tumor cells should be affected more than the normal cells, which could be of particular significance to cancer therapists (Sabo et al. Citation2002). This, in turn, leads to reduce the cell viability (IC50) in tumor cells much more than normal ones, particularly when treated with nanocurcumin, that is due to more bioavailability and efficacy of nanocurcumin compared to curcumin (Bharali et al. Citation2011, Muqbil et al. Citation2011).
In this work, the effect of 8 mT magnetic field with combined nanocurcumin (NANOCUR-MF) and curcumin (CUR-MF) on human erythrocytes after exposed 60 min was investigated. There was little difference between the effect of both NANOCUR-MF (p = 0.024) and CUR-MF (p = 0.02) compared with control ()). The hemolysis percentage for both NANOCUR-MF and CUR-MF samples was slightly higher than control at all concentrations; the highest hemolysis percentage for NANOCUR-MF was 23.769% ± 1.828 (20.657% ± 1.201 for control) at concentration 600 μM giving a little small E% of 13.093%. Likewise, for CUR-MF, the highest hemolysis percentage was 18.112% ± 1.146 (16.136% ± 0.975 for control) giving a lower E% of 10.91% at the same concentration 600 μM ( and ). Moreover, the highest E% between CUR-MF and control was 14.353% at concentration 100 μM and was 13.093% at concentration 600 μM for NANOCUR-MF with control ( and ). Depending on all results reported by this study, it was indicated that the hemolysis percentage has a very low value at all different concentrations for both NANOCUR-MF and CUR-MF and it did not reach IC50 value even up to higher concentrations. By taking into consideration that, the highest hemolysis percentage for both NANOCUR-MF and CUR-MF remain low (23.769% ± 1.828 for NANOCUR-MF and 18.112% ± 1.146 for CUR-MF). The use of MF has no or little effect on human erythrocyte as reported previously (Adahoun et al. Citation2016); both nanocurcumin and parent curcumin had no important effect on human erythrocytes. According to the obtained results (this and previous studies), the magnetic field might increase the compounds penetration (nanocurcumin and curcumin) into human erythrocytes cell, while the drugs have a weak toxicity against RBC.
Although, it was clearly noticed that both NANOCUR-MF and CUR-MF have slight effect on human erythrocytes after exposed 60 min, the obtained results are in agreement with some previous studies (Ali et al. Citation2003, Elblbesy Citation2010, Sicinska et al. Citation2006). Nevertheless, both NANOCUR-MF and CUR-MF have slight effect against human erythrocytes with more beneficial effect of NANOCUR-MF after short exposure time (60 min). This could due to modifications in cell shape and plasma membrane to the cells exposed to MF (Chionna et al. Citation2009). Moreover, MF could alter membrane permeability and increasing the flow rate of drugs into cells (Liu et al. Citation2011), which allows the compounds (nanocurcumin and curcumin) thereby favoring cellular uptake (Le et al. Citation2013). Therefore, due to the fact that nanocurcumin has more bioavailability and efficacy compared to curcumin (Bharali et al. Citation2011, Muqbil et al. Citation2011), nanocurcumin will be more cellular uptake into MF exposed cells than curcumin, leading to more membrane damage then lysis of cells and death of erythrocytes (Sicinska et al. Citation2006). In spite of this slightly effect, NANOCUR-MF overall has enhanced the cellular uptake into cells and still can be considered safe on human erythrocytes (RBCs) after exposed to 60 min with very low hemolysis percentage.
In this research study, the effect of magnetic field combined with nanocurcumin (NANOCUR-MF) and curcumin (CUR-MF) against bacteria strains was also examined by investigating the MBCs and bacteria growth (inhibition %) in vitro against the same bacteria strains two Gram-positive (Micrococcus luteus, Staphylococcus aureus), two Gram-negative (Escherichia coli, Pseudomonas aeruginosa). The results demonstrate that, the MBCs of NANOCUR-MF for Micrococcus luteus, Staphylococcus aureus, Escherichia coli, and Pseudomonas aeruginosa were 795.5, 616.89, 848.4, and 839.06 μg/ml, respectively, compared with 915.56, 822.86, 936.94, and 908.88 μg/ml for control (). Moreover, from , we can see that nanocurcumin combined with 8 mT MF leads to a reduction of MBCs value; E% was 13.11, 25.03, 9.45, and 7.68%, for all different bacteria strains of Micrococcus luteus, Staphylococcus aureus, Escherichia coli, and Pseudomonas aeruginosa comparing with control, respectively. Likewise, the MBCs of CUR-MF for Micrococcus luteus, Staphylococcus aureus, Escherichia coli, and Pseudomonas aeruginosa were 865.11, 748.26, 923.61, and 869.42 μg/ml, respectively, compared to 934.83, 844.42, 954.03, and 926.25 μg/ml for control (). Moreover, from , we can see that the parent curcumin combined with 8 mT MF reduces the MBCs value; E% was 7.46, 11.39, 3.19, and 6.14% for all different bacteria strains of Micrococcus luteus, Staphylococcus aureus, Escherichia coli, and Pseudomonas aeruginosa comparing with control, respectively. There was significant difference between MBCs values for NANOCUR-MF and CUR-MF compared with controls; the MBCs value for NANOCUR-MF and CUR-MF were lower than control at all different tested bacteria strains. On the other hand, the bacteria growth curve for NANOCUR-MF and CUR-MF was compared with control depending on inhibition percentage and it was found higher than control for all different bacteria strains at all concentrations ( and ). The obtained results concluded that there is an effect for both NANOCUR-MF and CUR-MF against all tested bacteria strains, with some variance between them (NANOCUR-MF was more efficient); this effect has the ability to decrease the number of CFU (colony forming units) and then reduce the MBCs values. Moreover, from the results, it can be concluded that, NANOCUR-MF and CUR-MF demonstrate more ability to inhibit the selected Gram-positive bacteria than the selected Gram-negative bacteria. This is due to differences between their cell membrane constituents and structure. It is known that Gram-positive bacteria contain an outer peptidoglycan layer, while Gram-negative bacteria contain an outer phospholibidic membrane, which leads to different type of interaction when encountered by both nanocurcumin and curcumin (Basniwal et al. Citation2011). In conclusion, NANOCUR-MF and CUR-MF have effect against all the examined bacteria; it can be seen that MF causes the decrease in the number of CFU (colony forming units) due to its effects on the permeability of the ionic channels in the membrane (Fojt et al. Citation2004). This leads to an increase in the flow rate of anti-microbial drugs (Nanocurcumin and Curcumin) into cells which in turn leads to an increase in inhibition percentage compared with control (decreasing the CUF), as well as reduces the MBCs value. In addition, depending on E%, NANOCUR-MF has more ability to reduce the MBCs value compared with control than CUR-MF for all tested bacteria strains (the efficiency of NANOCUR-MF was better than CURC-MF), because of its bioavailability and efficacy compared to curcumin (Bharali et al. Citation2011, Muqbil et al. Citation2011). However, our results are in disagreement with the results reported by other studies indicating that there is no effect of MF on bacteria growth (Gao et al. Citation2005, Grosman et al. Citation1991, Kohno et al. Citation2000, Ruiz-Gomez et al. Citation2004). Our results are in disagreement also with (Laszlo and Kutasi Citation2010) study concerning the effect of magnetic field against many different types of bacteria (Escherichia coli, Micrococcus luteus, and Staphylococcus aureus) at different periods of exposure. The authors reported that even the longest period of exposure failed to produce any effect in bacteria growth that could be correlated with SMF exposure. On the other side, our results are in agreement with (Strasak et al. Citation2002) results, where the authors reported that the growth curve for the bacteria exposed to MF was lower than the control one. Moreover, our results are also in is agreement with the study conducted on Escherichia coli, Leclercia adecarboxylata, and Staphylococcus aureus by (Fojt et al. Citation2004). It was concluded that the viability decreases with increased exposure time and also the CFU decrease starts immediately when MF was turned on. Our present finding supports the results of some previous studies (Ahmed et al. Citation2013, Ali et al. Citation2013, Inhan-Garip et al. Citation2011): bacteria growth reduced with exposure to MF.
The question on how MF can kill the bacteria was not yet clearly resolved, but the main theories that try to explain the biological effects of electromagnetic fields are based on the possible effects on the permeability of the ionic channels in the membrane. This can affect ion transport into the cells and result in biological changes within organisms. There is another possible effect consisting on the possible formation of free radicals due to MF exposure (Fojt et al. Citation2004). However, it is not clear yet that the effect of MF on bacteriostatic growth and production of certain enzymes, and more investigations in this direction is needed (Rai Citation2012).
Conclusion
In summary, we report on the synthesis of nanocurcumin and its effect when combined with 8 mT magnetic field and functional characterization of anti-cancer, antimicrobial, and cytotoxic activities. The obtained results showed enhanced potent anti-cancer and antibacterial activities as well as low hemolytic and cytotoxic activities against eukaryotic cells. The potency of NANOCUR-MF combined with its mild cytotoxic profile indicate that NANOCUR-MF has a great potential as an effective anti-tumor (PC3 antiproliferative) and antimicrobial agent, and that’s due to its solubility than parent curcumin, in addition that MF increases the membrane permeability. Therefore, it can be proposed that MF could be used in future with combined anti-cancer and anti-microbes drugs to increase drug's penetration into cells. Thereby, further investigation for MF combined with anti-cancer and anti-microbes drugs are needed, in order to optimize its pharmacodynamic and pharmacokinetic profiles.
Acknowledgements
The authors would like to acknowledge the technical support provided from Do'aa Khaleli and all staff at the Laboratory of Bio-Medical Physics (Department of Physics) and Microbiology Laboratory (Department of Biology) at Jordan University of Science and Technology, Jordan.
Disclosure statement
The authors report no declarations of interest. The authors alone are responsible for the content and writing of the paper.
References
- Adahoun MAA, Al-Akhras MAH, Jaafar MS, Bououdina M. 2016. Enhanced anti-cancer and antimicrobial activities of curcumin nanoparticles. Artif Cells Nanomedicine Biotechnol. [Epub ahead of print]. DOI: 10.3109/21691401.2015.1129628.
- Ahmed I, Istivan T, Cosic I, Pirogova E. 2013. Evaluation of the effects of extremely low frequency (ELF) pulsed electromagnetic fields (PEMF) on survival of the bacterium Staphylococcus aureus. EPJ Nonlinear Biomed Phys. 1:1–17.
- Ali F, El Khatib A, Sabry S, Abo Neima S, Motaweh H. 2013. Control of Staphylococcus aureus growth by electromagnetic therapy. Nat Sci. 6:169–178.
- Ali FM, Mohamed SW, Mohamed MR. 2003. Effect of 50 Hz, 0.2 mT magnetic fields on RBC properties and heart functions of albino rats. Bioelectromagnetics. 24:535–545.
- Anand P, Thomas SG, Kunnumakkara AB, Sundaram C, Harikumar KB, Sung B, et al. 2008. Biological activities of curcumin and its analogues (Congeners) made by man and Mother Nature. Biochem Pharmacol. 76:1590–1611.
- Basnet P, Skalko-Basnet N. 2011. Curcumin: an anti-inflammatory molecule from a curry spice on the path to cancer treatment. Molecules. 16:4567–4598.
- Basniwal RK, Buttar HS, Jain V, Jain N. 2011. Curcumin nanoparticles: preparation, characterization, and antimicrobial study. J Agric Food Chem. 59:2056–2061.
- Basri DF, Luoi CK, Azmi AM, Latip J. 2012. Evaluation of the combined effects of stilbenoid from Shorea gibbosa and vancomycin against methicillin-resistant Staphylococcus aureus (MRSA). Pharmaceuticals. 5:1032–1043.
- Bharali DJ, Siddiqui IA, Adhami VM, Chamcheu JC, Aldahmash AM, Mukhtar H, Mousa SA. 2011. Nanoparticle delivery of natural products in the prevention and treatment of cancers: current status and future prospects. Cancers. 3:4024–4045.
- Bodega G, Forcada I, Suarez I, Fernandez B. 2005. Acute and chronic effects of exposure to a 1-mT magnetic field on the cytoskeleton, stress proteins, and proliferation of astroglial cells in culture. Environ Res. 98:355–362.
- Casciari JJ, Riordan HD. 2001. The effect of alternating magnetic field exposure and vitamin C on cancer cells. J Orthomol Med. 16:177–182.
- Chionna A, Dwikat M, Panzarini E, Tenuzzo B, Carla E, Verri T, et al. 2009. Cell shape and plasma membrane alterations after static magnetic fields exposure. Eur J Histochem. 47:299–308.
- Elblbesy MA. 2010. Effect of static magnetic field on erythrocytes characterizations. J Biomed Sci Eng. 3:300.
- Feng SS. 2004. Nanoparticles of biodegradable polymers for new-concept chemotherapy. Expert Rev Med Devices. 1:115–125.
- Fojt L, Strasak L, Vetterl V, Smarda J. 2004. Comparison of the low-frequency magnetic field effects on bacteria Escherichia coli, Leclercia adecarboxylata and Staphylococcus aureus. Bioelectrochemistry. 63:337–341.
- Gamboa O, Gutierrez P, Alcalde I, de la Fuente I, Gayoso M. 2007. Absence of relevant effects of 5 mT static magnetic field on morphology, orientation and growth of a rat Schwann cell line in culture. Histol Histopathol. 22:4.
- Gao W, Liu Y, Zhou J, Pan H. 2005. Effects of a strong static magnetic field on bacterium Shewanella oneidensis: an assessment by using whole genome microarray. Bioelectromagnetics. 26:558–563.
- Gray JR, Frith CH, Parker JD. 2000. In vivo enhancement of chemotherapy with static electric or magnetic fields. Bioelectromagnetics. 21:575–583.
- Grosman Z, Kolár M, Tesaríková E. 1991. Effects of static magnetic field on some pathogenic microorganisms. Acta Univ Palacki Olomuc Fac Med. 134:7–9.
- Hao Q, Wenfang C, Xia A, Qiang W, Ying L, Kun Z, Runguang S. 2011. Effects of a moderate-intensity static magnetic field and adriamycin on K562 cells . Bioelectromagnetics. 32:191–199.
- Inhan-Garip A, Aksu B, Akan Z, Akakin D, Ozaydin AN, San T. 2011. Effect of extremely low frequency electromagnetic fields on growth rate and morphology of bacteria. Int J Radiat Biol. 87:1155–1161.
- Kamel FH, Saeed CH, Qader SS. 2013. The static magnetic field effect on Pseudomonas aeruginosa. Trends Biotechnol Res 40:M371–M376.
- Kohno M, Yamazaki M, Kimura I, Wada M. 2000. Effect of static magnetic fields on bacteria: Streptococcus mutans, Staphylococcus aureus, and Escherichia coli. Pathophysiology. 7:143–148.
- Laszlo J, Kutasi J. 2010. Static magnetic field exposure fails to affect the viability of different bacteria strains. Bioelectromagnetics. 31:220–225.
- Le TMP, Pham VP, Dang TML, La TH, Le TH, Le QH. 2013. Preparation of curcumin-loaded pluronic F127/chitosan nanoparticles for cancer therapy. Adv Nat Sci Nanosci Nanotechnol. 4:025001.
- Liu Y, Cao YD, Ye WX, Sun YY. 2011. Cerca un articolo. Tumori. 97:386–392.
- Miyakoshi J. 2005. Effects of static magnetic fields at the cellular level. Prog Biophys Mol Biol. 87:213–223.
- Mukerjee A, Vishwanatha J.K. 2009. Formulation, characterization and evaluation of curcumin-loaded PLGA nanospheres for cancer therapy. Anticancer Res. 29:3867–3875.
- Muqbil I, Masood A, Sarkar FH, Mohammad RM, Azmi AS. 2011. Progress in nanotechnology based approaches to enhance the potential of chemopreventive agents. Cancers. 3:428–445.
- Okonogi H, Nakagawa M, Tsuji Y. 1996. The effects of a 4.7 tesla static magnetic field on the frequency of micronucleated cells induced by mitomycin C. Tohoku J Exp Med. 180:209–215.
- Pagliara P, Lanubile R, Dwikat M, Abbro L, Dini L. 2009. Differentiation of monocytic U937 cells under static magnetic field exposure. Eur J Histochem. 49:75–86.
- Rai Y. 2012. Effect of static & low frequency magnetic fields on bacterium Streptococcus mutans. Int J IT Eng Appl Sci Res (IJIEASR) 1:34–37.
- Raylman RR, Clavo AC, Wahl RL. 1996. Exposure to strong static magnetic field slows the growth of human cancer cells in vitro. Bioelectromagnetics. 17:358–363.
- Ruiz-Gomez M, Prieto-Barcia M, Ristori-Bogajo E, Martinez-morillo M. 2004. Static and 50 Hz magnetic fields of 0.35 and 2.45 mT have no effect on the growth of Saccharomyces cerevisiae. Bioelectrochemistry. 64:151–155.
- Sa G, Das T. 2008. Anti cancer effects of curcumin: cycle of life and death. Cell Div. 3:14.
- Sabo J, Mirossay L, Horovcak L, Sarissky M, Mirossay A, Mojzis J. 2002. Effects of static magnetic field on human leukemic cell line HL-60. Bioelectrochemistry. 56:227–231.
- Shaw J, Raja SO, Dasgupta AK. 2014. Modulation of cytotoxic and genotoxic effects of nanoparticles in cancer cells by external magnetic field. Cancer Nanotechnol. 5:12–15.
- Sicinska P, Bukowska B, Michałowicz J, Duda W. 2006. Damage of cell membrane and antioxidative system in human erythrocytes incubated with microcystin-LR in vitro. Toxicon. 47:387–397.
- Strasak L, Vetterl VR, Smarda J. 2002. Effects of low-frequency magnetic fields on bacteria Escherichia coli. Bioelectrochemistry. 55:161–164.
- Tofani S, Cintorino M, Barone D, Berardelli M, de Santi MM, Ferrara A, et al. 2002. Increased mouse survival, tumor growth inhibition and decreased immunoreactive p53 after exposure to magnetic fields. Bioelectromagnetics. 23:230–238.
- Yoshie S, Suzuki Y, Wada K, Wake K, Sakai T, Nakasono S, et al. 2011. Estimation of mutagenic effects of intermediate frequency magnetic field using mammalian cells. IEEE Conference on General Assembly and Scientific Symposium, 2011 XXXth URSI, Istanbul, 1–4.