Abstract
Porous scaffolds composed of gelatin/poly (vinyl alcohol), (Gel/PVA), were prepared using combination of freeze gelation and freeze drying methods. The effect of polymer concentration, gelatin/PVA ratio, and glutaraldehyde/gelatin ratio (GA/Gel) was investigated on morphology of pores, swelling ratio, biodegradation, and skin cell culture. At optimum preparation conditions the scaffolds had uniform pore size distributions showing high swelling ratio of 23.6. The scaffolds were of biodegradable nature and almost degraded in 28 days. Human dermal fibroblast cells (HDF) were cultured on the scaffolds and MTS assay was conducted to evaluate the influence of PVA on growth and proliferation of the cells.
Introduction
Porous polymeric scaffolds provide desirable conditions for growth and proliferation of cells among its porous structure and could play an important role in the treatment of skin wounds occurred by burn or diabetes. An ideal scaffold should have (i) suitable interconnected pore size to facilitate cell migration and nutrients transfer; (ii) high-surface area and surface biocompatibility to enhance cellular attachment and proliferation; (iii) mechanical strength to keep integrity during and after implantation; and (iv) appropriate biodegradation rate (Boyce Citation2001, Chong et al. Citation2007, El Ghalbzouri et al. Citation2004, Lee et al. Citation2000, Perng et al. Citation2008, Zhong et al. Citation2010). Both the synthetic and natural polymers have been used to make biomedical scaffolds. Guan et al. made porous silk fibroin scaffolds by freeze drying method. The scaffolds showed biocompatibility and promoted skin recovery from full thickness defect (Guan et al. Citation2009). Tchemtchoua et al. produced chitosan nanofibrillar scaffolds that demonstrated good cell adhesion and proliferation in vitro (Tchemtchoua et al. Citation2011). Lin et al. reported electrospun chitosan-pectin scaffolds and showed its potential for proliferation of fibroblast cells (Lin et al. Citation2013).
Gelatin is a water soluble and biocompatible polymer, which is produced by partial hydrolysis of collagen extracted from skin and bones. It has been used as scaffolds for cell culture. However, porous gelatin scaffolds are very brittle that limits its biomedical applications. On the other hand, poly (vinyl alcohol), PVA, which is a water-soluble and biocompatible synthetic polymer with good mechanical properties has been blended with some other polymers to make biomedical scaffolds. A number of works have been reported on preparation of scaffolds based on gelatin and PVA.
Asran et al. prepared nanofibers from pure PVA, polyhydroxybutyrate (PHB), and their blends. Cell culture experiments with a dermal fibroblast showed adhesion and proliferation on PVA/PHB nanofibers (Asran et al. Citation2010). Zhou et al. investigated electrospun water-soluble carboxyethyl chitosan/poly (vinyl alcohol) scaffolds as potential wound dressing for skin regeneration (Zhou et al. Citation2007). Aramwit et al. assessed the physical properties of a three-dimensional silk sericin and PVA scaffold with and without glycerin as a plasticizer and genipin (as a cross-linking agent) at various concentrations. Smaller pore sizes and better uniformity were found as the concentration of genipin in the scaffold increased. The sericin/PVA scaffold had a low water sorption capacity, but adding glycerin significantly increased this property (Aramwit et al. Citation2010). Oh et al. fabricated poly (lactic-co-glycolic acid)/poly (vinyl alcohol) blend, PLGA/PVA, scaffolds by melt-molding particulate-leaching method. The scaffolds exhibited highly porous and open-cellular pore structures with pore size, 200–300 μm. It was observed that the PLGA/PVA blend scaffolds had better cell adhesion and growth than the neat PLGA scaffold. It seemed that 5% addition of PVA to PLGA was enough for improving the hydrophilicity and cell compatibility of the scaffolds (Oh et al. Citation2003). A porous chitosan/gelatin scaffold for tissue engineering was prepared via polyelectrolyte complex formation, freeze drying and post-crosslinking with glutaraldehyde (GA). The porosity and mean pore diameters were controlled within 30–100 μm by varying the original water content and the freezing conditions. Dipping the scaffolds in poly(lactic acid) provided good mechanical properties making it a promising candidate toward tissue engineering (Shen et al. Citation2000). Wu et al. (Citation2010) prepared porous gelatin scaffolds by freeze drying and studied the effect of parameters such as concentration of gelatin and a cross-linking agent on the morphology of pores, compressive strength, water absorption, and enzymatic degradation. Han et al. (Citation2014) blended gelatin and chitosan and used GA as a cross-linking agent to prepare scaffolds for wound healing purposes. The effect of chitosan and gelatin ratio on the morphology of pores, pore size, porosity, water retention capacity, and degradability of the scaffolds was reported.
Rahman et al. (Choi et al. Citation2013) studied gamma-irradiated gelatin-based polyvinyl alcohol solution-cast films (PVA content varied from 5 to 15 wt %) at low doses of 0.5–5 kGy and characterized the mechanical and thermal properties. The films were rigid and were not meant as scaffolds. It was found that in nonirradiated films PVA contributed to ductility of gelatin brittle films. However, gelatin was chain-scissioned even at these low irradiation doses and tensile strength and elongation of the blends decreased sharply. There was no indication of PVA cross-linking at the applied irradiation doses.
You et al. (Zhang et al. Citation2011) studied gelatin-PVA nonporous hydrogels using gamma irradiation at 25–50 kGy. The effect of irradiation dose and gelatin concentration on physical properties of these hydrogels was evaluated. The cytocompatibility of these hydrogels was evaluated in vitro in cultures of mouse fibroblasts. The fibroblast adhesion on the surface of hydrogels increased upon increasing the gelatin content. Although they have not pointed out the effect of irradiation on gelatin but from the gel strength and gel content data it could be concluded that PVA was cross-linked at these moderate irradiation doses, while gelatin was degraded.
In the present work, we prepared highly porous scaffolds of gelatin/PVA blend through combination of freeze gelation and freeze drying methods. GA was used as cross-linking agent for both PVA and gelatin. Cross-linking by gamma ray was also carried out and the results of which will be presented later. The effects of various parameters such as polymer solution concentration, gelatin/PVA, and GA/gelatin ratios and also preparation method on the microstructure, mechanical, and water absorption capacity of the scaffolds were studied. Also, biodegradability and biocompatibility of the scaffolds for human dermal fibroblast cells (HDF) were evaluated.
Materials and methods
Material
Gelatin (Gel) from porcine skin and poly (vinyl alcohol), PVA, (99% hydrolyzed and Mw 72,000 g/mol) were obtained from Fluka (Milwaukee, WI). GA 25 wt.% aqueous solution and Lysozyme from Sigma Aldrich (St. Louis, MO) were used.
Methods
Preparation of Gel/PVA scaffold
PVA was dissolved in distilled water at 80 °C and stirred for 5 h until homogenous solutions were obtained at 2, 3, 4, and 5 wt % concentrations. Gelatin was also separately dissolved at the same concentrations in distilled water at 40 °C and stirred for 30 min. Then, Gelatin and PVA solutions of the same concentration were mixed at Gel/PVA ratios of 9:1, 8:2, and 7:3 and stirred at room temperature for 3 h to achieve homogenous solutions. The Gel/PVA solutions (each about 18 g) were poured into polystyrene petri dishes and frozen at −20 °C. GA was used as cross-linking agent for gelatin (Gel) at GA/Gel ratios of 0.7, 1.4, and 2.8. shows the various compositions at which the samples were made.
Table 1. Composition of the samples.
A mixture of 15 g ethanol and 15 g glutaraldehyde aqueous solution was prepared and placed in −20 °C freezer. After freezing the polymer solution into a rigid frozen part, it was removed from the petri dish and immersed in the cold mixture of water/ethanol/glutaraldehyde and then stored at −20 °C for 48 h. During this period, ethanol/water mixture dissolved the ice crystals of frozen polymer solution, while gelatin and PVA remained undissolved. This procedure led to a porous structure of Gel/PVA filled with ethanol/water/GA solution. Also, simultaneous with this extraction process, it was expected that the amine groups of polypeptides of gelatin react with aldehyde groups of glutaraldehyde and form a cross-linked gelatin (Bigi et al. Citation2001). It is important to note that PVA would react with glutaraldehyde only at low pH values (Rudra et al. Citation2015). Thus, PVA was expected essentially to remain unreacted with glutaraldehyde during the extraction process. After 48 h, the samples were immersed in distilled water five times for 30 min each to remove the excess ethanol and glutaraldehyde, and then lyophilized by freeze-dryer to obtain dry porous scaffolds. The samples denoted as s-g:p-c (s: concentration of gelatin/PVA solution; g:p Gel/PVA ratio; c: GA/Gel ratio) e.g., 3–7:3–2.8.
Morphology
The morphology of pores inside the scaffolds was investigated using scanning electron microscopy (SEM) (TESCAN VEGS Series, Brno, Czech Republic) at 30 kV. The samples were immersed in liquid nitrogen and broken. The cross-section areas of the samples were coated with gold and observed under SEM. Analysis of SEM images was performed by an image processing program “ImageJ” (National Institutes of Health, Bethesda, MD) to study pore size distributions and the average pore size of each scaffold.
Water absorption
All samples were cut into specified dimensions and then they were immersed in distilled water for 24 h at room temperature (around 27 °C, at this temperature PVA is not soluble in water). Then, the surfaces of the samples were dried using filter papers to remove excess water and weighed. Swelling ratios of the scaffolds were calculated according to the following definition:
where Wd and Ww are dry and wet weights of the scaffolds. The results were reported as an average of five measurements.
Mechanical properties
A tensile machine (HIWA 2126, Iran) was used for this purpose with a 32kgf load cell. Samples were cut into rectangular 4 cm ×1 cm pieces. The crosshead speed and gauge length was set at 10 mm/min and 10 mm, respectively. Stress and strain data at break point were recorded. Three experiments were done for each sample.
In vitro biodegradation
Lyophilized samples were cut into 1 cm ×1 cm squares and were weighted before being immersed in 0.01M phosphate-buffered saline (PBS) solution of pH 7.4 containing 0.1 mg/mL of lysozyme. Samples were then maintained in a shaker incubator at 37 °C and 80 rpm to simulate the body environment. PBS/Enzyme solution was replaced every 2 days with fresh solution. At 7 day intervals the samples were taken out of solution and rinsed with distilled water properly to avoid any error at weight measurement caused by remained salt particles of PBS. Samples were then freeze-dried and weighted to evaluate their weight loss due to enzymatic degradation. The percentage of weight loss was calculated using the following relation
where wi is the initial weight and wf is the final weight of the scaffold after biodegradation at specified time interval and freeze-drying. Three specimens of each scaffold were used and the mean value was reported.
Human skin cell culture
MTS assay evaluates cell viability (growth and proliferation) of the scaffolds. Indeed, MTS is a tetrazolium compound which is reduced by living cells into a soluble compound (formazan) in a culture medium. Then, the light absorbance of solutions in culture mediums is a function of the extent of reduction by living cells and as a result it gives a measure of number of the living cells in the medium. Thus, the light absorbance of solutions in the culture mediums was determined at 490 nm by spectrophotometer with the following procedure. The scaffolds were cut into 1 cm ×1 cm squares and sterilized by immersion in 70% ethanol for 24 h and then in PBS solution for another 24 h. HDF cells were cultured to a concentration of 105 cells per cm2 of scaffolds in a medium containing 10% fetal bovine serum (FBS), 1% L-glutamine and 1% penicillin/streptomycin and incubated at 37 °C and 5% CO2, 95% air condition. At days 1, 3, and 7 the scaffolds were removed from the culture plate. A mixture of MTS and serum-free culture medium in 5:1 ratio was poured on each scaffold. After 3 h of incubation (at dark, 37 °C and 5% CO2, 95% air condition), light absorbance measurements were performed at 490 nm.
Results and discussions
Morphology of scaffolds
Length of a fibroblast cell is almost 30–50 μ and so the pores must be sufficiently large and highly interconnected to facilitate cell and nutrient transportation to the interior parts of scaffold. shows SEM images at cross section of samples with different Gel/PVA ratios beside their pore size distribution graphs obtained by Image J software. For the pure gelatin and the sample with 7:3 ratio an almost Gaussian pore size distribution is obtained, which occurs at all the areas of cross section, but at the sample with 9:1 ratio pore size distribution is not uniform at all the areas of cross section and small pores aggregate at most areas while bigger pores are found irregularly among them. This kind of irregular distribution is intensified at 8:2 ratio while at 7:3 ratio the Gaussian distribution is again achieved and uniformly repeats at cross section area of the sample. These alterations may be attributed to the transposition of phase separation and gelation temperatures during cooling the polymer solutions. Phase separation temperature is dependent on polymers ratio and concentration. If gelation occurs prior to cloud point at cooling process the macro phase separation will not occur and phase separation via spinodal decomposition will happen. On the other hand, if cloud point temperature precedes the gelation temperature, microphase separation will happen and nucleation and growth will be the phase separation mechanism (Asran et al. Citation2010). First mechanism causes more uniform distribution of polymers and thus more uniform viscosities across the solution, which leads to more uniform ice crystal growth.
Figure 1. Cross sectional SEM image of scaffolds prepared by 3 wt % polymer solution concentration, GA/Gel ratio of 2.8 and Gel/PVA ratio of (A) 10:0 (B) 9:1 (C) 8:2 (D) 7:3.
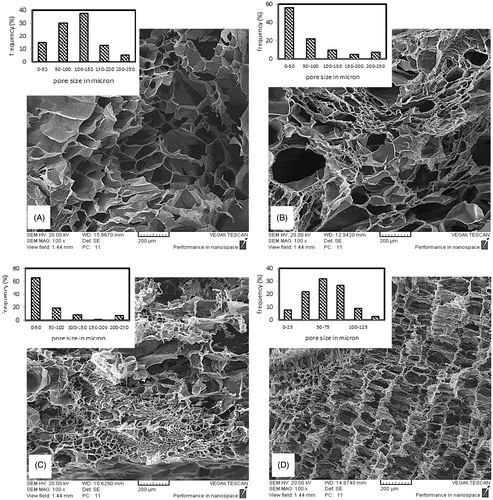
shows SEM images at the cross section of samples with different polymer solution concentrations. Samples with higher concentrations show smaller pore sizes. The average pore size at 2, 3, 4, and 5 wt % are 78, 65.6, 14.4, and 12.4 μ, respectively. The reason is the higher viscosity at solutions of higher concentration. Higher viscosity causes more resistance in polymer solution against ice crystal growth and so limits their size and interconnectivity. shows SEM images at cross section of samples with different GA/Gel ratios. It can be deduced from SEM images that there is no substantial relation between GA/Gel ratio and the morphology of scaffolds. This would be attributed to the procedure of fabrication. To make three samples of different GA/Gel ratio, the first step is freezing the solutions with the same concentration and Gel/PVA ratio. It is clear that similar ice crystal patterns would form in all the three samples. Next step is removing ice particles and formation of cross-links in polymer structure by immersion of the frozen samples in a mixture of ethanol and aqueous glutaraldehyde solution at a low temperature of −20 °C. During this step due to low temperature, the morphology of pores is not varied significantly and remains almost intact.
Water absorption
Water absorption of porous scaffolds is affected by two factors: (1) Nature of constituent polymers and how much hydrophilic they are. (2) The ratio of pores volume to the total volume. The effect of polymer solution concentration, Gel/PVA ratio, and GA/Gel ratio is illustrated in and . All the samples show high water absorption capacity of more than 10 times of their dry weight. Swelling ratio decreases noticeably as the polymer concentration increases. This is because of lower porosity and interconnectivity and also smaller pore sizes in scaffolds fabricated by higher concentrations. Increasing PVA ratio from 10% to 30% result in more water absorption. PVA has numerous hydroxyl groups interacting with water molecules through hydrogen bonds very well. PVA reacts with glutaraldehyde at low pH, thus at current fabrication method hydroxyl groups of PVA are all free and no chemical cross-link is formed between them, consequently they are capable of absorbing so much water molecules (Aramwit et al. Citation2010, Zhou et al. Citation2007). As mentioned earlier, the amount of glutaraldehyde does not affect morphology significantly and this is consistent with the results of swelling ratio of samples with different GA/Gel ratio. In these samples, the swelling ratio is almost constant.
Mechanical properties
Mechanical properties were investigated to evaluate the scaffold’s ability to withstand tensions at implantation and after the implantation inside the body. Porosity and intrinsic mechanical properties of constituent materials are the main parameters affecting the mechanical properties of scaffolds. reveal values of stress and strain at break point for samples with different polymer concentrations and Gel/PVA ratios. Samples are named according to the polymer concentration, Gel/PVA ratio, and GA/Gel ratio used for their fabrication. For example 3–91–2.8 is the sample prepared by 3 wt % solutions of gelatin and PVA mixed in Gel/PVA ratio of 9:1 and the ratio of GA/Gel is 2.8. PVA has better mechanical properties compared with brittle gelatin so the addition of PVA enhances the maximum tolerable stress and strain of scaffolds. Although no improvement is observed at sample with 20% PVA ratio in comparison with 10% PVA ratio and that would be related to the phase separation, discussed earlier at morphology investigation section, and consequently the weak distribution of PVA among the sample. As it would be expected, an increase in polymer concentration would lead to less porosity and consequently improved the mechanical properties.
In vitro biodegradation
In this experiment weight loss of each scaffold was recorded during a period of 28 days. shows the percentage of weight loss occurred by enzymatic degradation for seven selected scaffolds. These scaffolds were selected in order to evaluate the influence of polymer solution concentration, Gel/PVA ratio, and GA/Gel ratio on degradation of scaffolds. The highest degradation belongs to the sample 3–9:1–2.8 that is totally degraded in 21 days. By raising the PVA ratio to 20% and 30%, the samples degrade in less extent of 85% and 76% at day 28. This is due to higher biodegradation rate of gelatin. An increase in polymer concentration would cause a drop in scaffold degradation. For instance, the degradation of samples 3–7:3–2.8 and 5–7:3–2.8 at 28th day is 76% and 55%, respectively. To investigate the effect of GA/Gel ratio on biodegradability of scaffolds the samples 4–8:2–2.8 and 4–8:2–0.7 were included in this experiment. The results show the great influence of GA/Gel ratio on degradation rate. For comparison, the sample with the GA/Gel ratio of 0.7 degrades 93% in 28 days while the sample with the GA/Gel ratio of 2.8 degrades just 66% at the same time interval. The higher GA/Gel ratio forms more chemical bonds in gelatin chains and thus the degradation rate becomes slower, while according to the previous results higher glutaraldehyde ratio does not change morphology and water absorption capacity. Consequently, in this fabrication method glutaraldehyde can be applied at desired amount in order to regulate degradation of scaffold without any significant changes in morphology and swelling behavior of scaffolds.
Cytotoxicity and viability of HDF cells on scaffold
The previous results demonstrated the effective role of PVA on improvement of scaffold characteristics such as water absorption capacity, mechanical properties, and regulation of degradation rate. So the principal question is whether the presence of PVA would affect the good interaction between gelatin and cells greatly or not? To answer this question the sample 3–7:3–2.8 was selected as the sample with optimized characteristics and beside that two other samples with the same polymer concentration and GA/Gel ratio but different amounts of PVA (0 and 10%) were used to compare the influence of PVA on cell viability. All three samples were cultured with HDF cells and MTS assay was conducted at 1, 3, and 7 days. shows the results achieved by MTS assay. According to the results, all the three samples show an upward trend from day 1 to day 7. The absorbance of all samples is almost equivalent at days 1 and 3, but at day 7 the samples with lower PVA ratio show higher cell density. Most synthetic polymers, such as PEG and PVA, are known to prevent cellular adhesion for long periods of time, because most cells do not have receptors for synthetic polymers (Oh et al. Citation2003). However, gelatin, as a denatured collagen, contains a large number of glycine, proline, and hydroxyproline residues. Therefore, incorporation of gelatin to synthetic hydrogels provides various kinds of ligands that bind to the receptors on cell surfaces, providing enhanced adhesion. The results obtained by MTS assay reveals that the increase in PVA ratio slows down cell growth and proliferation, but it does not stop it. Also, the results show that no residual glutaraldehyde has remained in scaffolds as no sign of cytotoxicity was observed.
Conclusions
In this study, combination of freeze gelation and freeze drying methods were used to make Gel/PVA sponge scaffolds. The effect of different parameters such as concentration of Gel/PVA solution, Gel/PVA, and GA/Gel ratios was investigated on the microstructure and various properties of the scaffolds. The results showed the effective role of PVA in enhancing water absorption and mechanical strength and also adjusting biodegradation rate. The main advantage of this method was the ability to control the biodegradation rate, while keeping the other important characteristics including morphology and water absorption almost constant. This could be achieved by changing the GA/Gel ratio. The sample 3–7:3–2.8 was selected as the sample with optimal characteristics because it had uniform pore size distribution, suitable pore size for HDF cells migration, high porosity, high swelling ratio, good mechanical properties, and suitable degradation rate. HDF cells were cultured on this scaffold and the results showed good biocompatibility of scaffold along with growth and proliferation of cells. Although PVA decreased the growth rate of cells compared with pure gelatin, it did not stop their growth and proliferation.
Disclosure statement
The authors report no conflicts of interest. The authors alone are responsible for the content and writing of this article.
References
- Aramwit P, Siritientong T, Kanokpanont S, Srichana T. 2010. Formulation and characterization of silk sericin-PVA scaffold crosslinked with genipin. Int J Biol Macromol. 47:668–675.
- Asran AS, Razghandi K, Aggarwal N, Michler GH, Groth T. 2010. Nanofibers from blends of polyvinyl alcohol and polyhydroxy butyrate as potential scaffold material for tissue engineering of skin. Biomacromolecules. 11:3413–3421.
- Bigi A, Cojazzi G, Panzavolta S, Rubini K, Roveri N. 2001. Mechanical and thermal properties of gelatin films at different degrees of glutaraldehyde crosslinking. Biomaterials. 22:763–768.
- Boyce ST. 2001. Design principles for composition and performance of cultured skin substitutes. Burns. 27:523–533.
- Choi SM, Singh D, Kumar A, Oh TH, Cho YW, Han SS. 2013. Porous three-dimensional PVA/gelatin sponge for skin tissue engineering. Int J Polym Mater Polym Biomater. 62:384–389.
- Chong EJ, Phan TT, Lim IJ, Zhang YZ, Bay BH, Ramakrishna S, Lim CT. 2007. Evaluation of electrospun PCL/gelatin nanofibrous scaffold for wound healing and layered dermal reconstitution. Acta Biomater. 3:321–330.
- El Ghalbzouri A, Lamme EN, van Blitterswijk C, Koopman J, Ponec M. 2004. The use of PEGT/PBT as a dermal scaffold for skin tissue engineering. Biomaterials. 25:2987–2996.
- Guan G, Bai L, Zuo B, Li M, Wu Z, Li Y, Wang L. 2009. Promoted dermis healing from full-thickness skin defect by porous silk fibroin scaffolds (PSFSs). BioMed Mater Eng. 20:295–308.
- Han F, Dong Y, Su Z, Yin R, Song A, Li S. 2014. Preparation, characteristics and assessment of a novel gelatin–chitosan sponge scaffold as skin tissue engineering material. Int J Pharm. 476:124–133.
- Lee DY, Ahn HT, Cho KH. 2000. A new skin equivalent model: dermal substrate that combines de-epidermized dermis with fibroblast-populated collagen matrix. J Dermatol Sci. 23:132–137.
- Lin HY, Chen HH, Chang SH, Ni TS. 2013. Pectin-chitosan-PVA nanofibrous scaffold made by electrospinning and its potential use as a skin tissue scaffold. J Biomater Sci Polym Ed. 24:470–484.
- Oh SH, Kang SG, Kim ES, Cho SH, Lee JH. 2003. Fabrication and characterization of hydrophilic poly (lactic-co-glycolic acid)/poly (vinyl alcohol) blend cell scaffolds by melt-molding particulate-leaching method. Biomaterials. 24:4011–4021.
- Perng CK, Kao CL, Yang YP, Lin HT, Lin WB, Chu YR, et al. 2008. Culturing adult human bone marrow stem cells on gelatin scaffold with pNIPAAm as transplanted grafts for skin regeneration. J Biomed Mater Res A. 84:622–630.
- Rudra R, Kumar V, Kundu PP. 2015. Acid catalysed cross-linking of poly vinyl alcohol (PVA) by glutaraldehyde: effect of crosslink density on the characteristics of PVA membranes used in single chambered microbial fuel cells. RSC Adv. 5:83436–83447.
- Shen F, Cui YL, Yang LF, Yao KD, Dong XH, Jia WY, Shi HD. 2000. A study on the fabrication of porous chitosan/gelatin network scaffold for tissue engineering. Polym Int. 49:1596–1599.
- Tchemtchoua VT, Atanasova G, Aqil A, Filée P, Garbacki N, Vanhooteghem O, et al. 2011. Development of a chitosan nanofibrillar scaffold for skin repair and regeneration. Biomacromolecules. 12:3194–3204.
- Wu X, Liu Y, Li X, Wen P, Zhang Y, Long Y, et al. 2010. Preparation of aligned porous gelatin scaffolds by unidirectional freeze-drying method. Acta Biomater. 6:1167–1177.
- Zhang F, He C, Cao L, Feng W, Wang H, Mo X, Wang J. 2011. Fabrication of gelatin–hyaluronic acid hybrid scaffolds with tunable porous structures for soft tissue engineering. Int J Biol Macromol. 48:474–481.
- Zhong SP, Zhang YZ, Lim CT. 2010. Tissue scaffolds for skin wound healing and dermal reconstruction. Wiley Interdiscip Rev Nanomed Nanobiotechnol. 2:510–525.
- Zhou Y, Yang D, Chen X, Xu Q, Lu F, Nie J. 2007. Electrospun water-soluble carboxyethyl chitosan/poly (vinyl alcohol) nanofibrous membrane as potential wound dressing for skin regeneration. Biomacromolecules. 9:349–354.