Abstract
The silver nanoparticles synthesized from Turbinaria ornata (To-AgNPs) showed spherical with crystalline nature (20–32 nm) was evaluated against fourth instar larvae of three mosquitoes. The maximum activity of To-AgNPs was recorded on Aedes aegypti followed by Anopheles stephensi and Culex quinquefasciatus with the following lethal concentration values (μg/ml): LC50 of 0.738, 1.134, and 1.494; and LC90 of 3.342, 17.982, and 22.475, respectively. The obtained respective values (μg/ml) vis-a-vis aqueous extract (To-AE) were: 2.767 and 40.577; 4.347 and 158.399, and 7.351 and 278.994. The findings revealed that To-AgNPs could form a base for the development of an eco-friendly, low-cost pesticide.
Introduction
Mosquitoes are blood sucking insects, which cause numerous diseases to millions of people throughout the world (WHO Citation2009). Through their bites, they transmit parasites and cause skin allergy and diseases to human beings. There are 350–500 million clinical cases of malaria per year with about one million deaths. Especially in India, every year two million malaria cases are being reported (Veerakumar et al. Citation2014). The mosquito species, Aedes aegypti is a vector of chikungunya and dengue virus (Flavivirus) and it is an anthropophilic mosquito, which has got an intimate relationship with human by exhibiting several behavioral traits like oviposition in man-made and man-used natural and artificial containers (Yu et al. Citation2015). It causes chikungunya, a severe viral disease which has been recently considered to be an important public health problem in India and in other countries like Senegal and West Africa (Yamar et al. Citation2005). Dengue fever is prevalent throughout the tropics and subtropics. It is the most significant mosquito spread viral disease and a major international public health concern. Dengue hemorrhagic fever (DHF) is caused by dengue virus which belongs to the genus, Flavivirus, of the family Flaviviridae and it includes serotypes 1, 2, 3, and 4 (Den-1, Den-2, Den-3, and Den-4) (Veerakumar et al. Citation2013). The World Health Organization estimates that around 2.5 billion people are at risk to dengue and such infections have dramatically increased in recent decades due to increased urbanization, trade and travel (WHO Citation2010). As there is no effective drug or vaccine against dengue, the only way of its prevention is to combat the disease-carrying mosquitoes from breeding and biting humans.
In view of the effectiveness and recognized non-toxic effects on non-target organisms, the plant-based insecticides have been regularly used to control the mosquitoes (Govindarajan and Rajeswary Citation2015, Jayaraman et al. Citation2015, Santhosh et al. Citation2015, Suganya et al. Citation2013). Of late, mosquitocidal silver nanoparticles (AgNPs) are being synthesized from the oil-seed extracts and seaweed-borne compounds (Murugan et al. Citation2015, Pavela Citation2015). Nanoparticles play a major role in disease diagnostics, sensing, imaging, delivering gene and drug, artificial implants and in tissue engineering (Morones et al. Citation2005). Plant based NPs synthesis is advantageous over chemical and physical methods, as it is cheaper, rapid, single step method and eco-friendly, does not require high pressure, energy, temperature, and the use of highly toxic chemicals (Huang et al. Citation2007). Moreover, NPs synthesis using different plant parts are more advantageous rather than with microbes because it eliminates the elaborate process of maintaining cell cultures (Shankar et al. Citation2004).
As it is essential to find a novel mosquito-insecticide, there is growing interest in looking for natural alternatives especially from the widely distributed but under exploited seaweeds. Therefore, it is necessary to explore the bioactive components of seaweeds so as to assess their mosquitocidal activity. Understanding the seaweed chemical constituents would provide information on the structure-activity relationships of active compounds that are responsible for the insecticidal action (Dias and Moreas Citation2014, Yu et al. Citation2014). Recently, some seaweed-based AgNPs synthesis have been reported; Padina tetrastromatica (Rajeshkumar et al. Citation2012), Sargassum wightii (Shanmugam et al. Citation2013), Codium capitatum (Kannan et al. Citation2013), Padina gymnospora (Singh et al. Citation2013), Chaetomorpha linum (Ragupathi Raja Kannan et al. Citation2013), Gracilaria corticata (Kumar et al. Citation2013), Hypnea musciformis (Ganapathy Selvam and Sivakumar Citation2014), Caulerpa scalpelliformis (Murugan et al. Citation2015) Caulerpa racemosa (Kathiraven et al. Citation2015). The Turbinaria ornata extract have been reported to have antibacterial (Vijayabaskar and Shiyamala Citation2011), anti-coagulant (Arivuselvan et al. Citation2011), anti-inflammatory (Ananthi et al. Citation2011), anti-oxidant properties (Kelman et al. Citation2012, Chakraborty et al. Citation2013). In view of the above, this study was focused on the structural characterization and mosquito-larvicidal potential of the AgNPs synthesized from the seaweed, Turbinaria ornata (Turner) J. Agardh.
Materials and methods
Collection of seaweed samples
Fresh marine brown algal samples were collected from the Mandapam, Ramanathapuram District, Tamil Nadu, Southeast coast of India (9° 22' N; 78° 52' E). The marine macroalga, Turbinaria ornata (Turner) J. Agardh 1848 (Sargassaceae) () have been identified based on standard keys, (Dinabandhu Citation2010) and the identification was confirmed by Dr. N. Kaliaperumal, Principal Scientist (Retd.), Central Marine Fisheries Research Institute, Mandapam Camp, Ramanathapuram District, India. The reference specimens have been kept in the Department of Biotechnology, Periyar University, Salem. Silver nitrate (AgNO3) was purchased from Hi-media Pvt. Ltd, India. All the chemicals used in this study were of analytical grade with maximum purity.
Mosquito culture
The fourth instar mosquito larvae of Aedes aegypti, Anopheles stephensi, and Culex quinquefasciatus collected from Salem District, Tamil Nadu, India, were kept in plastic trays containing tap water and maintained in laboratory condition. Biscuits were served as larval food. All the experiments were carried out at 28 ± 2°C, 70–80% relative humidity and photoperiod of 12 h in light and dark conditions, respectively.
Preparation of Turbinaria ornata aqueous extract (To-AE)
The fresh seaweed sample was washed thoroughly with tap water and then with distilled water to remove marine soil debris and associated biota. The washed seaweeds were dried under shadow for three weeks. Then the dried seaweeds were ground using electric blender. Aqueous extract was prepared by mixing 10 g of dried seaweed powder in 100 ml of sterile double distilled water with constant stirring on a magnetic stirrer. Finally, the extract was filtered with Whatman no. 1 filter paper and the extract was used within 1 h.
Synthesis of silver nanoparticles (AgNPs)
Twelve milliliters of To-AE was treated with 88 ml of 1 mM AgNO3 (16.96 mg in 100 ml Milli-Q water) solution in an Erlenmeyer flask and incubated at room temperature to observe the color change from brownish yellow to brown solution that indicates the formation of AgNPs (Chitra et al. Citation2015).
Characterization of the synthesized To-AgNPs
The silver nanoparticle formation was observed periodically using UV–vis spectrophotometer (Cyber Lab UV-100, Millbury, MA) in the range of 300–800 nm operated at a resolution of 1 nm. The synthesized AgNPs were subjected to centrifugation at 10,000 ×g for 10 min and the pellet was dissolved in deionized water, filtered, and air dried. The centrifuged and parched material containing silver nanoparticles was used for X-ray diffraction (XRD) (Advance power X-ray diffractometer D8, Brucker, Karlsruhe, Germany) and Fourier transform infrared spectroscopy (FTIR) analysis (Perkin-Elmer Spectrum 2000, Waltham, MA). In addition, the presence of silver ions in the sample was analyzed by energy dispersive X-ray spectroscopy (EDX). The size and morphology of the To-AgNPs were analyzed by field emission-scanning electron microscope (FE-SEM; JEOL, Model JFC-1600, Zeiss, Germany). The measurement of particle size and zeta potential of To-AgNPs was carried out by particle size analyzer system (Zeta-sizer, Malvern Instruments Ltd, Worcestershire, UK).
Mosquito larvicidal bioassay
The larvicidal efficacy of To-AE and synthesized To-AgNPs were tested as per the guidelines of World Health Organization procedure (WHO Citation1996), with some modifications. Different concentrations (10, 5, 2.5, 1.25, and 0.625 ppm) of To-AE and To-AgNPs were used, respectively, to test larvicidal activity on A. aegypti, A. stephensi, and C. quinquefasciatus mosquito larvae and a control without test samples. Batches of 25 healthy 4th instar larvae were added into the glass beaker containing 250 ml of tap water with desired concentration of To-AgNPs and To-AE for each mosquito species. The number of dead larvae was counted after 24 h of exposure and their percentage mortality was calculated. Three replicates were performed and control group (Tap water) was assigned to each mosquito species. Percentage mortality was calculated using Abbott’s formula (Abbott Citation1925), Mortality (%) = X – Y/*100; where, X: survival in the untreated control and Y: survival in the treated sample (Gnanadesigan et al. Citation2011).
Statistical analysis
The average larval mortality data were subjected to Probit Analysis (Finney Citation1971) for calculating LC50, LC90, and other statistics at 95% confidence limit with upper and lower confidence limit, and Chi-square values were calculated using the SPSS 16.0 software (Armonk, NY). Results were considered to be statistically significant at p < 0.05.
Results
Characterization of To-AgNPs
The formation of AgNPs was observed visually by change in color of aqueous extract of T. ornata from brownish yellow to brown with 1 mM AgNO3. The To-AE without AgNO3 did not show any color change (). The bio-reduction of Ag+ ions had occurred within 10 min, with a strong surface plasmon resonance at 420 nm (), thus indicating the formation of AgNPs. The XRD analysis of To-AgNPs showed the 2θ values of 38.00°, 46.55°, 65.06°, and 77.40° corresponding to (1 1 1), (2 0 0), (2 2 0) and (3 1 1), respectively (). The FTIR spectrum of To-AE () showed bands at, 3415.72, 2070.59 1576.46 and 1399.15 cm−1. The band at 3415.72 cm−1 could be assigned to the O−H stretching vibration of alcohol groups. The band at 2070.59 cm−1 corresponds to the N=C stretching vibration of miscellaneous groups. The bands at 1576.46 and 1399.15 cm−1 were corresponding to the C–O stretching vibration of carboxylic acids. FTIR spectrum of To-AgNPs () showed the presence of bands at 3402.60, 2201.21, 1572.62, 1401.87, 1481.88, 1260.35, 1123.26, 1036.32, 924.47, 830.85, 762.70, 651.74, 697.18, 618.05, and 429.22 cm−1. The band at 3402.60 cm−1 could be assigned to the O–H stretching vibration of alcohol groups. Similarly, the band at 2201.21 cm−1 signals to the C≡C stretching vibration of alkynes groups. The bands at 1572.62 and 1401.87 cm−1 also represents the C–O stretching vibration of carboxylic acids. The band at 1481.88 and cm−1 consigned to the C–C stretching vibration of aromatics groups. The bands at 1260.35, 1123.26 and 1036.32 cm−1 were signaling to the C–H stretching vibration of alkyl halides. The bands at 924.47 and 830.85 cm−1 were of miscellaneous groups. The band at 762.70 cm−1 represents the C–Cl stretching vibration of alkyl halides. The bands at 651.74 and 697.18 cm−1 were of alkynes with C≡H stretching vibrations. Finally, the bands at 618.05 and 429.22 cm−1 were assigned to the alkynes of C≡H and alkyl halides of C–H wag groups. In addition, the FTIR peaks indicated the presence of alcohols, alkynes, aromatics, carboxylic acids, and alkyl halides. The FE-SEM micrograph of To-AgNPs () clearly indicated the presence of spherical particles with size ranges between 20 and 32 nm. The energy dispersive X-ray spectroscopy (EDX) analysis of To-AgNPs confirmed the presence of elemental silver as the major constituent (). The zeta potential analysis of To-AgNPs showed the negative value −2.86 mV, confirming that the nanoparticles are in colloidal state (). The particle size analysis exposed the Z-average of To-AgNPs are 655.6 (d.nm) and PdI is 0.926 ().
Figure 2. Synthesis of silver nanoparticles using T. ornata: (a) AgNO3. (b) Aqueous extract of T. ornata. (c) T. ornata- silver nanoparticles (To-AgNPs).
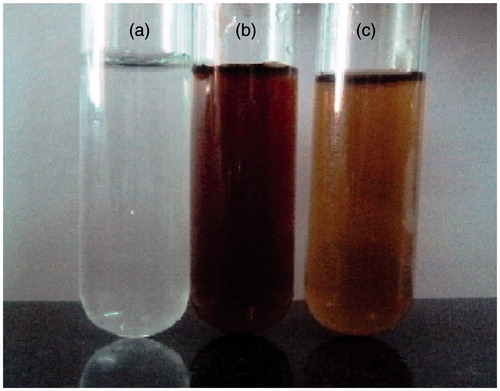
Mosquito larvicidal activity of To-AE and To-AgNPs
The percentage mortality of To-AE and To-AgNPs for 4th instar larvae of A. aegypti, A. stephensi, and C. quinquefasciatus are depicted in and . The obtained LC50 and LC90 values are presented in and . The effective LC50 and LC90 values (μg/ml) of To-AE were: 2.767 and 40.577 (A. aegypti) followed by 4.347 and 158.399 (A. stephensi), and 7.351 and 278.994 (C. quinquefasciatus), respectively. Whereas the To-AgNPs showed the LC50 and LC90 values (μg/ml) of: 0.738 and 3.342 (A. aegypti), 1.134 and 17.982 (A. stephensi), and 1.494 and 22.475 (C. quinquefasciatus), respectively. The control did not show any mortality. χ2 value was significant at p < 0.05 level.
Table 1. Larvicidal activity of T. ornata aqueous extract against fourth instar larvae of A. aegypti, A. stephensi, and C. quinquefasciatus at 24-h observation.
Table 2. Larvicidal activity of T. ornata AgNPs against fourth instar larvae of A. aegypti, A. stephensi, and C. quinquefasciatus at 24-h observation.
Discussion
Nano-biotechnology is fast-emerging to be an important discipline of modern science and engineering, which provides scope for the synthesis of raw materials of distinctive sizes, shapes, and compositions. The nanoparticles of silver, gold, and palladium are being widely used in the development of medical and pharmaceutical products (Song et al. Citation2010). The earlier study has reported that nano or colloidal state of metallic silver gives different color to aqueous solution due to the excitation of electrons from the valence bond to conduction bond (Mulvaney Citation1996). Currently, the formation of AgNPs was confirmed by the color change of seaweed aqueous extract, when AgNO3 was added. The occurrence of brown color was due to the excitation of ions that signals a strong surface plasmon vibration at 420 nm, which indicated the formation of silver nanoparticles. The XRD analysis of To-AgNPs showed four intense peaks (1 1 1), (2 0 0), (2 2 0), and (3 1 1), that are in agreement to the Bragg’s reflection of silver nanocrystals (Lu et al. Citation2003). Further, the XRD-pattern with sharp Bragg peaks are in agreement with the Joint Committee on Power Diffraction Standards (JCPDS no. 040783), confirming the crystalline nature of the AgNPs, as reported earlier (Gnanadesigan et al. Citation2012). The observed other minor unassigned peaks in their vicinity might have been due to the capping agent that stabilizes the nanoparticles. Independent crystallization of the capping agents, is ruled out due to the process of centrifugation and redispersion of the pellet in deionized distilled water after nanoparticle formation as part of purification process (Haldar et al. Citation2013). The presence of functional groups viz: alkane, methylene, alkene, amine, and carboxylic acids in the aqueous plant leaf extract, has already been proved to be of potential reducing agents for the synthesis of AgNPs (Cho et al. Citation2005). Interestingly, the FTIR spectrum of To-AE () showed the presence of amine groups and carboxylic acids, which might act as a capping agent, for the reduction of silver ions. The recent study has reported that the different functional groups present in the aqueous extract would stabilize the AgNPs (Chanthini et al. Citation2015). The FE-SEM micrograph of To-AgNPs showed spherical shape, which evidenced the nature of silver that have already been reported by previous researchers (Merin et al. Citation2010, Veerakumar et al. Citation2013). The metallic silver nanocrystals that generally show typical absorption peak approximately at 3 keV due to surface plasmon resonance (Haldar et al. Citation2013). The EDX pattern of To-AgNPs showed strong signals for Ag, whereas the Cl and O peaks might be originated from the aqueous extract. In addition, the zeta potential measurement of To-AgNPs showed a sharp peak at −2.86 mV suggesting that the surface of the nanoparticles is negatively charged. The zeta potential value of To-AgNPs is an indication of repulsive force which could be used to predict the long term stability of the product (Patil et al. Citation2012).
Several researchers have found that the seaweeds possess good insecticidal property like that of the terrestrial plants-derived bio-insecticides (Ghosh et al. Citation2012, Oliveira et al. Citation2010, Yu et al. Citation2014). Presently, we have investigated the larvicidal effect of T. ornata on the fourth instar larvae of Aedes aegypti, Anopheles stephensi, and Culex quinquefasciatus. Generally, the fourth instar larvae are considered to be having more immune-competency than the younger larvae (Patil et al. Citation2012, Vogelweith et al. Citation2013). To-AE showed moderate larvicidal effect with the lethal concentrations (LC) at the LC50 values of (μg/ml): 2.767, 4.347, and 7.351 and LC90 values of (μg/ml): 40.577, 158.399, and 278.994 against A. aegypti, A. stephensi, and C. quinquefasciatus, respectively. The recorded inhibitory concentrations of To-AgNPs (μg/ml) towards the highest mortality were: LC50; 0.738, 1.134, and 1.494; and LC90; 3.342, 17.982, and 22.475 against A. aegypti, A. stephensi, and C. quinquefasciatus, respectively. These mortality effects could have been due to the presence of various phytal-constituents of seaweed, T. ornata. The phytal-constituents, including phenols, terpenoid, flavonoids, saponin, and alkaloids of the seaweed, Hypnea musciformis might be responsible for the larvicidal property (Roni et al. Citation2015). Recent study reported that, the bio-toxicity of Ulva lactuca-mediated AgNPs showed the LC50 and LC90 values (μg/ml): 2.111 and 8.350; 3.090 and 10.292; 4.629 and 13.254; 5.261 and 14.851 against the malarial mosquito larvae A. stephensi (1st to 4th instars), respectively (Murugan et al. Citation2015).
Some earlier researchers have found that the acetone extracts of the marine seaweeds; Caulerpa scapelliformis, Dictyota dichotoma, Enteromorpha clathrata, E. intestinalis, and U. lactuca showed good activity against 4th instar larvae of A. aegypti with the following LC50 values (μg/ml): 53.70, 61.65, 85.11, 67.70, and 91.20, respectively (Thangam and Kathiresan Citation1991). The larvicidal activity of acetone extracts of seaweed, C. scalpelliformis against 2nd and 3rd instar larvae of Culex pipiens with the following LC50 and LC90 values (μg/ml): 338.91 and 1891.31, respectively (Cetin et al. Citation2010). Recent report also confirms the larvicidal efficacy of seaweed; Caulerpa scalpelliformis mediated AgNPs that showed maximum LC50 and LC90 values (μg/ml) of 5.86 and 12.38, respectively, for the 4th instar mosquito larvae of C. quinquefasciatus (Murugan et al. Citation2015). The larvicidal activity of seaweed, Hypnea musciformis-mediated AgNPs on A. aegypti (1st to 4th instars) showed the following LC50 and LC90 values (μg/ml): 18.14 and 246.59, 20.54 and 269.05, 26.61 and 301.66, 27.99 and 319.30, respectively (Roni et al. Citation2015).
Many reports have appeared on the larvicidal property of terrestrial plants based AgNPs. The larvicidal activity of AgNPs from aqueous leaf extract of Sida acuta against the third-instar larvae of C. quinquefasciatus (LC50: 26.13 and 130.30 μg/ml), A. stephensi (LC50: 21.92 and 109.94 μg/ml), and A. aegypti LC50 (23.96 and 119.32 μg/ml), respectively (Veerakumar et al. Citation2013). Recently, larvicidal activity of AgNPs and aqueous leaf extract of Annona muricata were tested against A. aegypti (LC50: 12.58 and 51.13 μg/ml; LC90: 26.46 and 82.08 μg/ml), A. stephensi (LC50: 15.28 and 61.38 μg/ml; LC90: 31.91 and 156.55 μg/ml) and C. quinquefasciatus (LC50: 18.77 and 88.72 μg/ml; LC90: 35.72 and 199.67 μg/ml), respectively (Santhosh et al. Citation2015).
Conclusion
In this study, we reported the mosquitocidal property of T. ornata-mediated AgNPs that was found to be higher when compared to the larvicidal properties recorded by the previous researchers. The nano-size and spherical shape of the synthesized AgNPs from T. ornata were confirmed through FE-SEM micrograph analysis and the To-AgNPs showed high mosquito-larvicidal potential.
Acknowledgements
The authors are grateful to the authorities of Periyar University for providing the necessary facilities to carry out this work.
Disclosure statement
No potential conflict of interest was reported by the authors.
References
- Abbott WS. 1925. A method of computing the effectiveness of insecticides. J Eco Ento. 18:265–267.
- Ananthi S, Gayathri V, Chandronitha C, Lakshmisundaram R, Vasanthi HR. 2011. Free radical scavenging activity and anti-inflammatory potential of a marine brown alga Turbinaria ornata (Turner). J Agardh Indian J Geo Mar Sci. 40:664–670.
- Arivuselvan N, Radhiga M, Anantharaman P. 2011. In vitro antioxidant and anticoagulant activities of sulphated polysaccharides from brown seaweed (Turbinaria ornata) (Turner). J Agardh Asian J Pharm Biol Res. 1:232–239.
- Cetin H, Gokoglu M, Oz E. 2010. Larvicidal activity of the extract of seaweed, Caulerpa scalpelliformis, against Culex pipiens. J Am Mosq Control Assoc. 26:433–435.
- Chakraborty K, Praveen NK, Vijayan KK, Rao GS. 2013. Evaluation of phenolic contents and antioxidant activities of brown seaweeds belonging to Turbinaria spp. (Phaeophyta, Sargassaceae) collected from Gulf of Mannar. Asian Pac J Trop Biomed. 3:8–16.
- Chanthini AB, Balasubramani G, Ramkumar R, Sowmiya R, Balakumaran MD, Kalaichelvan PT, Perumal P. 2015. Structural characterization, antioxidant and in vitro cytotoxic properties of seagrass, Cymodocea serrulata (R. Br.) Asch. & Magnus mediated silver nanoparticles. J Photochem Photobiol B Biol. 153:145–152.
- Chitra G, Balasubramani G, Ramkumar R, Sowmiya R, Perumal P. 2015. Mukia maderaspatana (Cucurbitaceae) extract-mediated synthesis of silver nanoparticles to control Culex quinquefasciatus and Aedes aegypti (Diptera: Culicidae). Parasitol Res. 114:1407–1415.
- Cho K, Park J, Osaka T, Park S. 2005. The study of antimicrobial activity and preservative effects of nanosilver ingredient. Electrochim Acta. 51:956–960.
- Dias CN, Moreas DFC. 2014. Essential oils and their compounds as Aedes aegypti L. (Diptera: Culicidae) larvicides: review. Parasitol Res. 113:565–592.
- Dinabandhu S. 2010. Common Seaweed of India. New Delhi (India): IK International Pvt Ltd, pp. 1–196.
- Finney DJ. 1971 Probit analysis. Vol 551. London: Cambridge University Press, pp. 68–72.
- Ganapathy Selvam G, Sivakumar K. 2014. Phycosynthesis of silver nanoparticles and photocatalytic degradation of methyl orange dye using silver (Ag) nanoparticles synthesized from Hypnea musciformis (Wulfen) J.V. Lamouroux. Appl Nanosci. 5:617–622.
- Ghosh A, Chowdhury N, Chandra G. 2012. Plant extracts as potential mosquito larvicides. Indian J Med Res. 135:581–598.
- Gnanadesigan M, Anand M, Ravikumar S, Maruthupandy M, Syed Ali M, Vijayakumar V, Kumaraguru AK. 2012. Antibacterial potential of biosynthesized silver nanoparticles using Avicennia marina mangrove plant. Appl Nanosci. 2:143–147.
- Gnanadesigan M, Anand M, Ravikumar S, Maruthupandy M, Vijayakumar V, Selvam S, Dhineshkumar M, Kumaraguru AK. 2011. Biosynthesis of silver nanoparticles by using mangrove plant extract and their potential mosquito larvicidal property. Asian Pac J Trop Med. 4:799–803.
- Govindarajan M, Rajeswary M. 2015. Ovicidal and adulticidal potential of leaf and seed extract of Albizia lebbeck (L.) Benth. (Family: Fabaceae) against Culex quinquefasciatus, Aedes aegypti, and Anopheles stephensi (Diptera: Culicidae). Parasitol Res. 114:1949–1961.
- Haldar KM, Haldar B, Chandra G. 2013. Fabrication, characterization and mosquito larvicidal bioassay of silver nanoparticles synthesized from aqueous fruit extract of putranjiva, Drypetes roxburghii (Wall.). Parasitol Res. 112:1451–1459.
- Huang J, Li Q, Sun D, Lu Y, Su Y, Yang X, et al. 2007. Biosynthesis of silver and gold nanoparticles by novel sundried Cinnamomum camphora leaf. Nanotechnology. 18:105104.
- Jayaraman M, Senthilkumar A, Venkatesalu V. 2015. Evaluation of some aromatic plant extracts for mosquito larvicidal potential against Culex quinquefasciatus, Aedes aegypti, and Anopheles stephensi. Parasitol Res. 114:1511–1518.
- Kannan RRR, Stirk WA, Van Staden J. 2013. Synthesis of silver nanoparticles using the seaweed Codium capitatum P.C. Silva (Chlorophyceae). S Afr J Bot. 31:1–4.
- Kathiraven T, Sundaramanickam A, Shanmugam N, Balasubramanian T. 2015. Green synthesis of silver nanoparticles using marine alga Caulerpa racemosa and their antibacterial activity against some human pathogens. Appl Nanosci. 5:499–504.
- Kelman D, Posner EK, McDermid KJ, Tabandera NK, Wright PR, Wright AD. 2012. Antioxidant activity of Hawaiian marine algae. Mar Drugs. 10:403–416.
- Kumar P, Senthamil Selvi S, Govindaraju M. 2013. Seaweed-mediated biosynthesis of silver nanoparticles using Gracilaria corticata for its antifungal activity against Candida spp. Appl Nanosci. 3:495–500.
- Lu HW, Liu SH, Wang XL, Qian XF, Yin J, Jhu JK. 2003. Silver nanocrystals by hyperbranched polyurethane-assisted photochemical reduction of Ag+. Mater Chem Phys. 81:104–107.
- Merin DD, Prakash S, Bhimba BV. 2010. Antibacterial screening of silver nanoparticles synthesized by marine micro algae. Asian Pac J Trop Med. 3:797–799.
- Morones JR, Elechiguerra JL, Camacho A, Holt K, Kouri JB, Ramfrez JT, Yacaman MJ. 2005. The bactericidal effect of silver nanoparticles. Nanotechnology. 16:2346–2353.
- Mulvaney P. 1996. Surface plasmon spectroscopy of nanosized metal particles. Langmuir. 12:788–800.
- Murugan K, Benelli G, Ayyappan S, Dinesh D, Panneerselvam C, Nicoletti M, et al. 2015. Toxicity of seaweed-synthesized silver nanoparticles against the filariasis vector Culex quinquefasciatus and its impact on predation efficiency of the cyclopoid crustacean Mesocyclops longisetus. Parasitol Res. 114:2243–2253.
- Oliveira PV, Ferreira JC, Jr., Moura FS, Lima GS, de Oliveira FM, Oliveira PE, et al. 2010. Larvicidal activity of 94 extracts from ten plant species of northeastern of Brazil against Aedes aegypti L. (Diptera: Culicidae). Parasitol Res. 107:403–407.
- Patil CD, Borase HP, Patil SV, Salunkhe RB, Salunke BK. 2012. Larvicidal activity of silver nanoparticles synthesized using Pergularia daemia plant latex against Aedes aegypti and Anopheles stephensi and nontarget fish Poecillia reticulata. Parasitol Res. 111:555–562.
- Patil CD, Patil SV, Borase HP, Salunke BK, Salunkhe RB. 2012. Larvicidal activity of silver nanoparticles synthesized using Plumeria rubra plant latex against Aedes aegypti and Anopheles stephensi. Parasitol Res. 110:1815–1822.
- Pavela R. 2015. Acute toxicity and synergistic and antagonistic effects of the aromatic compounds of some essential oils against Culex quinquefasciatus Say larvae. Parasitol Res. 114:3835–3853.
- Ragupathi Raja Kannan R, Arumugam R, Ramya D, Manivannan K, Anantharaman P. 2013. Green synthesis of silver nanoparticles using marine macroalga Chaetomorpha linum. Appl Nanosci. 3:229–233.
- Rajeshkumar S, Kannan C, Annadurai G. 2012. Synthesis and characterization of antimicrobial silver nanoparticles using marine brown seaweed Padina tetrastromatica. Drug Invent Today. 4:511–513.
- Roni M, Murugan K, Panneerselvama C, Subramaniam J, Nicoletti M, Madhiyazhagan P, et al. 2015. Characterization and biotoxicity of Hypnea musciformis-synthesized silver nanoparticles as potential eco-friendly control tool against Aedes aegypti and Plutella xylostella. Ecotoxicol Environ Safety. 121:31–38.
- Santhosh SB, Yuvarajan R, Natarajan D. 2015. Annona muricata leaf extract-mediated silver nanoparticles synthesis and its larvicidal potential against dengue, malaria and filariasis vector. Parasitol Res. 114:3087–3096.
- Shankar SS, Rai A, Ahmad A, Sastry M. 2004. Rapid synthesis of Au, Ag, and bimetallic Au core-Ag shell nanoparticles using Neem (Azadirachta indica) leaf broth. J Colloid Interface Sci. 275:496–502.
- Shanmugam N, Rajkamal P, Cholan S, Kannadasan N, Sathishkumar K, Viruthagiri G, Sundaramanickam A. 2013. Biosynthesis of silver nanoparticles from the marine seaweed Sargassum wightii and their antibacterial activity against some human pathogens. Appl Nanosci. 4:881–888.
- Singh M, Kalaivani R, Manikandan S, Sangeetha N, Kumaraguru AK. 2013. Facile green synthesis of variable metallic gold nanoparticle using Padina gymnospora, a brown marine macroalga. Appl Nanosci. 3:145–151.
- Song JY, Kwon EY, Kim BS. 2010. Biological synthesis of platinum nanoparticles using Diopyros kaki leaf extract. Bioprocess Biosyst Eng. 33:159–164.
- Suganya A, Murugan K, Kovendan K, Mahesh Kumar P, Hwang JS. 2013. Green synthesis of silver nanoparticles using Murraya koenigii leaf extract against Anopheles stephensi and Aedes aegypti. Parasitol Res. 112:1385–1397.
- Thangam TS, Kathiresan K. 1991. Mosquito larvicidal activity of marine plant extracts with synthetic insecticides. Bot Mar. 34:537–539.
- Veerakumar K, Govindarajan M, Rajeswary M. 2013. Green synthesis of silver nanoparticles using Sida acuta (Malvaceae) leaf extract against Culex quinquefasciatus, Anopheles stephensi, and Aedes aegypti (Diptera: Culicidae). Parasitol Res. 112:4073–4085.
- Veerakumar K, Govindarajan M, Rajeswary M, Muthukumaran U. 2014. Low-cost and eco-friendly green synthesis of silver nanoparticles using Feronia elephantum (Rutaceae) against Culex quinquefasciatus, Anopheles stephensi, and Aedes aegypti (Diptera: Culicidae). Parasitol Res. 113:1775–1785.
- Vijayabaskar P, Shiyamala V. 2011. Antibacterial activities of brown marine algae (Sargassum wightii and Turbinaria ornata) from the Gulf of Mannar biosphere reserve. Adv Biol Res. 5:99–102.
- Vogelweith F, Thiéry D, Moret Y, Moreau J. 2013. Immunocompetence increases with larval body size in a phytophagous moth. Physiol Entomol. 38:219–225.
- WHO. 1996. Report of the WHO informal consultation on the evaluation on the testing of insecticides CTD/WHO PES/IC/96.1:69.
- WHO. 2009. [Internet]. 10 facts on malaria. Available from: http://www.who.int/features/factfiles/malaria/en/.
- World Health Organization. 2010. Dengue Transmission Research in WHO Bulletin. Geneva: WHO.
- Yamar BA, Diallo D, Kebe CM, Dia I, Diallo M. 2005. Aspects of bio ecology of two Rift valley fever virus vectors in Senegal (West Africa) Aedes vexanus and Culex poicilipes (Diptera; Culicidae). J Med Entomol. 42:739–750.
- Yu KX, Jantan I, Ahmad R, Wong CL. 2014. The major bioactive components of seaweeds and their mosquitocidal potential. Parasitol Res. 113:3121–3141.
- Yu KX, Wong CL, Ahmad R, Jantan I. 2015. Larvicidal activity, inhibition effect on development, histopathological alteration and morphological aberration induced by seaweed extracts in Aedes aegypti (Diptera: Culicidae). Asian Pac J Trop Med. 8:1006–1012.