Abstract
Recently, silver nanoparticles (AgNPs) have been used for cancer treatment. To achieve a successful anticancer activity, AgNP needs to be delivered sufficiently to the cells. Cell-penetrating peptides (CPPs) are small cationic peptides that have ability to transport various cargos across cell membranes. In this study, a strategy was developed for cancer treatment, where AgNP modified with CPPs displayed dramatically antitumor activity in MCF-7 cell lines. Peptides-modified AgNPs showed significant enhancement in killing tumor cells by increasing the uptake of AgNP into cell lines and could be a new class of nano-drug for cancer treatment.
Introduction
Nowadays, with the emergence of multidrug-resistance and various side effects related to chemotherapies, cancer becomes one of the most health problems and life-threatening disease that enforces severe mortality worldwide. To overcome this problem, there is an urgent need to develop new strategies for the early diagnosis and treatment of cancer with minimal side effects (Jeyaraj et al. Citation2013, Yallapu et al. Citation2012).
In the recent years with the development of nanotechnology, the use of nanomaterials for cancer therapy and diagnosis greatly increased. Because of the unique properties that appear at the nanoscale, nanomaterials are attractive as therapeutic and imaging agents in oncology that provide many benefits and new opportunities to address the complexity of cancer (Huang et al. Citation2011, Toy et al. Citation2014). Among various nanomaterials, silver nanoparticles (AgNPs) have been widely applied in biomedicine due to its unique properties, such as biocompatibility, stability, catalytic, and potent antimicrobial property. Recently, AgNPs have been reported for its anticancer effect (Sanpui et al. Citation2011, Sriram et al. Citation2010). AgNP is becoming favorable for cancer controlling since it targets the mitochondrial system leading to production of ROS (reactive oxygen species) and interruption of ATP synthesis, which causes DNA damage and alter other biological mechanistic pathways for cell survival (AshaRani et al. Citation2008, Gengan et al. Citation2013, Sukirtha et al. Citation2012). To achieve a successful anticancer activity, AgNP needs to be delivered sufficiently to the cells. It was indicated that nanoparticles size, shape, surface charge, and coating (e.g., protein, polymer) all affect their cellular uptake. Liu et al. reported that the cytotoxicity caused by AgNP is size-dependent and AgNP of ∼5 nm in size produced higher toxicity than those of ∼20 nm and ∼50 nm in size (Liu et al. Citation2010). Sur et al. demonstrated that lactose-modified AgNP causes more DNA damage and cytotoxicity increases in A549 cells compared to oligonucleotide-modified AgNP (Sur et al. Citation2012). In another study, it was found that lactose-modified AgNP enter the L929 cells at a higher rate (Sur et al. Citation2010). People have tried several strategies to increase the cellular uptake of nanoparticles. One of the most effective ways to reach this, is achieved by conjugation of nanoparticles to cell-penetrating peptides (CPPs) (Farkhani et al. Citation2014, Yuan et al. Citation2012). In one work AgNP modified with TAT peptide displayed extraordinary antitumor activity in both MDR cells and non-resistant cells at an indiscriminating manner (Liu et al. Citation2012).
CPPs are a class of small cationic peptides (typically less than 30 amino acids) that have ability to transport various cargos across cell membrane. These peptides have been conjugated to a wide range of cargos to improve their membrane penetration, including siRNA (Nakase et al. Citation2013), peptides, small drug molecules (Nakase et al. Citation2012), various nanoparticles (Farkhani et al. Citation2015a, Koshkaryev et al. Citation2013, Liu et al. Citation2013, Mussa Farkhani and Valizadeh Citation2014, Park et al. Citation2013). CPPs generally are composed of positively charged amino acids such as lysine and arginine. The mechanism of their cellular translocation is still obscure, but it has been proposed that they penetrate inside cell by endocytosis or direct penetration through the plasma membrane (Farkhani et al. Citation2015b, Park et al. Citation2013). The cellular uptake of poly-arginine tends to be more efficient than that other cationic CPPs. It was indicated that the highest translocation efficiencies were achieved by using octa-arginine (R8) or nona-arginine (R9) peptides (Xu et al. Citation2010). Recently, it has been shown that insertion of tryptophan as a hydrophobic amino acid to the poly-arginine sequence enhances translocation of CPPs into the cell (Bechara et al. Citation2013, Jobin et al. Citation2015, Rydberg et al. Citation2012). The aim of this study was to investigate the effect of CPPs on the cytotoxicity of AgNP in MCF-7 cell line.
Materials and methods
Materials
AgNO3 (99%), NaI (99%), NaBH4 (98%), N-hydroxysulfosuccinimide (NHS), 1-ethyl-3(3-dimethylaminopropyl) carbodiimides hydrochloride (EDC), and mercaptopropionic acid (98%) were obtained from Sigma (St. Louis, MO). FMOC-Rink-Amide AM resin and amino acid derivatives were obtained from AAPPTec (Louisville, KY). Coupling agents (TBTU and DIEPA), scavengers (ethanedithiol, phenol, and TIPS), and cleavage reagents (piperidine and TFA) were purchased from Sigma (St. Louis, MO). 3-(4,5-dimethylthiazol-2-yl)-2,5-diphenyltetrazolium bromide (MTT), RPMI1640, fetal bovine serum (FBS), trypsin-EDTA, and penicillin/streptomycin were purchased from Invitrogen (Carlsbad, CA).
AgNP preparation
The synthesis of MPA-capped AgNP was performed following the previously reported procedure by Li et al. (Citation2003). A total of 5 mL of 10−2 M AgNO3 was added to 150 mL of triply distilled water. A total of 10 mL of 10−2 M 3-mercaptopropionic acid was added as stabilizer to the solution with stirring. After 15 min of mixing, 5 mL of 10−2 M NaI was dropped into the solution slowly. Then, 40 mg of NaBH4 was added to the AgI colloidal solution, and the reaction mixture was continually stirred for about 25 min. The silver colloid was finally obtained.
Peptides synthesis
All CPPs were synthesized manually by solid-phase peptide synthesis method on a Rink-Amide AM resin by FMOC strategy in a fritted glass vessel. Resin was swelled in DMF for about 30 min under dry nitrogen. FMOC deprotection of resin was carried out using piperidine in DMF solution (20% v/v, 2 mL, 30 min). FMOC-Arg (Pbf)-OH (0.14 mmol) was coupled to the resin in the presence of TBTU (0.12 mmol), and DIPEA (50 μL) in DMF (2 mL) by mixing for 2 h. After the coupling was completed, the reaction solution was filtered off and resin was washed with DMF (4 × 2 mL) and DCM (4 × 2 mL), followed by FMOC deprotection. The resin was washed with DMF and DCM. In each step, ninhydrin test was used to monitor FMOC deprotection and coupling of amino acids. After the coupling of all amino acids, the resin was washed with DMF, DCM, and ethanol, respectively (each 2 × 2 mL). The resin was dried in a vacuum desiccator for 24 h. Fresh cleavage cocktail, reagent B that includes TFA/TIPS/phenol/water (88:2:5:5 v/v/v/v, 3 mL), was added to the resin for side-chain deprotection and the final cleavage of the synthesized peptide from the solid support. The mixture was shaken at room temperature for 2 h. The resin was collected by filtration and washed with another 2 mL of fresh cleavage cocktail. The crude peptide was precipitated by adding 100 mL diethyl ether and centrifuged at 4000 rpm for 5 min, followed by decantation to obtain the solid precipitates. The obtained peptide was further washed with ether (2 × 50 mL) for 2 times and lyophilized ().
Table 1. Peptides sequences (Farkhani et al. Citation2015b, Mandal et al. Citation2011).
SEM
The sample was prepared by placing a drop of AgNP on carbon-coated copper grid and subsequently drying in air, before transferring it to the microscope operated at 10 KV (SEM, Mira3 FEG-SEM Tescan).
UV–visible spectroscopy
The yellowish-brown colloidal solution was characterized using UV–Vis spectrometer (Shimadzu, Japan) in the range of 200–600 nm.
Size and zeta potential of AgNP and AgNP-CPP complexes
The particle size distribution of AgNP and AgNP-CPP were evaluated using DLS measurements. The zeta potential analysis was conducted with a Malvern Zetasizer (Malvern, UK). The AgNP were dissolved in physiological saline for zeta potential and the data were analyzed using Zetasizer software.
Preparation of AgNP-CPP conjugates
In this method, reaction was carried out in Borate Buffer 1X (10 mM) at pH 6.5. Briefly, 3.5 mg of MPA-capped AgNP was added in Borate buffer (4 mL). Then under stirring, EDC (2 mM) and NHS (5 mM) were added to the reaction buffer. The reaction proceeded for more than 25 min after which each peptide (1 mg/mL) was added in five different reaction vessels and thoroughly mixed with continuous stirring for more than 24 h. Then, AgNP-CPP was centrifuged at 14,000 rpm for 30 min and washed three times with distilled water to remove the excess of peptide and stored at 4 °C for the future use.
Cell culture
MCF-7 human breast cancer cell lines were obtained from Pasteur Institute, Iran. The cells were maintained in RPMI 1640 medium supplemented with 10% FBS, 100 units/mL penicillin, and 100 μg/mL streptomycin. The cells were maintained at 37 °C with 5% CO2 in a humidified CO2 incubator.
Cytotoxicity of AgNP and AgNP-CPP complexes
In vitro cytotoxicity of AgNP and AgNP-CPP conjugates against MCF-7 cells was assessed by the standard MTT assay. Cells (1 × 104 cells/well) were placed on 96-well plates, and incubated 24 h at 37 °C in 5% CO2 atmosphere. After incubation, the medium was discarded and then cells were treated with varying concentrations (0–500 μg/mL) of AgNP and AgNP-CPP. Following 24 h incubation, the MTT assay was performed by adding 50 μl of 2 mg/mL MTT to each well for 4 h. The resulting formazan crystals were dissolved in DMSO (200 μl/well) and the absorbance of individual wells was obtained at 570 nm. Furthermore, the IC50 was calculated as the concentration required inhibiting the growth of tumor cells in culture by 50% compared to the untreated cells using the dose-response curves.
Results and discussion
Synthesis and characterization of peptides, AgNP and AgNP-CPP conjugates
Prior to the study of the antitumor effect of AgNPs and AgNP-CPP complexes, characterization was performed. All the peptides were prepared manually by solid phase peptide synthesis on the Rink-Amide AM resin. Kaiser test was used to control the completeness of each deprotection and coupling step. After synthesis, each resin bound peptide was dried overnight, washed, and cleaved by cleavage cocktail to afford five CPPs, which were lyophilized. The synthesized peptides in this study include nona-arginine, and others contain three to six arginine residues and three to six tryptophans, which the tryptophans positioned either in the middle, or evenly distributed along the amino acid sequence.
Thiol-capped AgNPs are particularly attractive nanomaterials for application in biomedicine. The AgNP functionalized with the thiol could prevent the formation of aggregation and are also critical for controlling the chemical reactions and subsequently responsible for its uniformity (Kumar et al. Citation2012). AgNPs coated with 3-mercaptopropionic acid were prepared by chemical reduction method using sodium borohydride. The free carboxyl group presented in MPA-capped AgNP strongly participates in covalent coupling with various biological molecules. The carboxyl group on the surface of AgNP, conjugated to the amino group of each peptides through EDC/NHS method. The synthesized AgNP displays dark yellowish-brown color in aqueous solution due to the surface Plasmon resonance phenomenon (Gengan et al. Citation2013, Mei et al. Citation2013). The UV–Vis absorption spectrum of AgNP () revealed that the maximum absorbance occurs at 400 nm, which is characteristic for the formation of AgNP. SEM and DLS were used to determine the size and shape of AgNP. The SEM image () demonstrated that AgNPs are morphologically homogeneous and most of the particles are spherical or near spherically shaped. DLS analysis showed the mean particle size of 31.61 nm (). Zeta potential was employed to characterize of each AgNP-CPP conjugates. The data showed that AgNP have a zeta potential of −28.5 mV alone. However, the surface property was significantly changed after peptides coupling, reflected by changing zeta potential that showed in .
Cytotoxicity of AgNP and AgNP-CPP complexes
The mechanism of cellular entrance of AgNP could involve phagocytosis or a passive, nonspecific diffusion pathway through the plasma membrane. Because of its negative surface, the non-modified AgNP was less favorable for cell adhesion and delivery. Besides, due to lack of active mechanisms interacting with the cell membrane and various surface receptors, cellular uptake of AgNP is poorly low. It was showed that AgNP synthesized by sodium borohydride could not efficiently enter into A549 cells (Kaur and Tikoo Citation2013). Recently, CPP-mediated delivery has become one of the most efficient methods for improving intracellular delivery of nanoparticles (Liu et al. Citation2012).
For comparison of the antitumor activity of AgNP and CPP-modified AgNP the MTT assay was done in MCF-7 cells. The results are presented in . The AgNPs were able to reduce viability of the MCF-7 cells in a dose-dependent manner. The viability results showed that conjugation of CPPs to the AgNP enhance their activity against cancer cell line. Cytotoxicity of AgNP up to 16 μg/mL was insignificant, with cell viability of 90%. However, employing peptides for delivery of AgNP significantly affect the toxicity particularly in concentration below 16 μg/mL. The highest cytotoxicity was observed in case of AgNP-[RW]4. The IC50 values of AgNPs and AgNP-CPP complexes were calculated that shown in . As shown in the table, AgNPs display IC50 value of 104.1 μg/mL against MCF-7 cell line. CPPs modification of nanosilver dramatically change their cytotoxicity, where the values of IC50 were measured to be 10.2, 24.1, 27.4, 29.7, 43.5, 92 for AgNP-[RW]4, AgNP-[RW]6, AgNP-R5W3R4, AgNP-[RW]3, AgNP-[RW]5, and AgNP-R9, respectively. The data showed that the peptides that have tryptophan in sequence could increase antitumor activity of AgNP better than R9.
Figure 5. Comparing the toxicity of AgNP and AgNP-CPP complexes in MCF-7 cell lines. MTT assay was done after 24-h incubation. The experiments were performed in triplicates; data shown represent mean ± SD of three independent experiments.
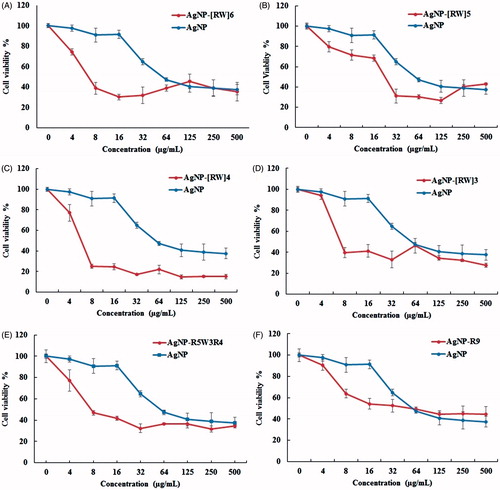
Table 2. IC50 values of AgNP and AgNP-CPP conjugates.
The zeta potential reflects the charge on the AgNP surface. In this study, the results show a direct correlation between the toxicity of AgNPs and their surface charge. The more negative MAP-capped AgNPs were the least toxic in cell lines. The MCF-7 cell lines showed a zeta potential values of −19.4 mV (Bondar et al. Citation2012). Regarding the similarity between zeta of AgNP and MCF-7, there is a high degree of repulsion between the negatively charged AgNP and the cell membrane, thus, forms an electrostatic barrier, which limits the cell-particle interactions and decrease the entrance of AgNP into cells. Due to decrease of the negative potential after peptide coupling, the electrostatic barrier is reduced which increases the chances of cell-particle interactions and results in higher uptake and subsequent toxicity. Once the electrostatic barrier is overcome, the AgNP-CPP complexes can interact with the cellular membrane and pass through the membrane that ultimately leads to cellular death, for example, by chemically induce ROS. In addition, these peptides with intrinsic cell ability increase the amount of AgNPs that entered to the cell. The improved antitumor activity of AgNPs were attributed to decreased repulsion between the negatively charged AgNP and the cell membrane, enhanced cellular uptake efficiency prompted by CPPs and the consequent elevating drug accumulation inside cells (Liu et al. Citation2012).
Also these peptides enhance uptake of AgNP into cells, they maybe increase its transport across mitochondria membrane. The recent studies on AgNP toxicity have recognized the mitochondria as primary intracellular target of AgNP (Kaur and Tikoo, Citation2013). A possible mechanism suggested for cell toxicity of AgNP is disruption of the mitochondrial respiratory chain that leads to production of ROS and interruption of ATP synthesis, which finally cause DNA damage (AshaRani et al. Citation2008). Gaining access to mitochondrial by AgNP can be problematic, as the inner membrane is a barrier limiting diffusive transport. It was showed that a class of CPPs that efficiently enter human cells can specifically localize to mitochondria. The peptides identified are cationic and lipophilic; this combination of characteristics facilitates permeation of the hydrophobic mitochondrial membrane (Horton et al. Citation2008). It is possible that CPPs used for the delivery of AgNP could transport their cargo specifically to the mitochondria, the best destination for AgNP. The capability of these CPPs as mitochondria-penetrating peptides need to be studied in the future works.
Conclusion
To overcome the current difficulties in the treatment of cancer, we developed a new strategy by modifying AgNP with the CPPs. Due to decrease of the negative potential of AgNP after peptide coupling, the electrostatic barrier of cell membrane is reduced, which increases the chances of cell-particle interactions and results in higher uptake and subsequent toxicity. In addition, these peptides with intrinsic cell ability increase the amount of AgNP that entered to the cell. Furthermore, it is possible that these CPPs have mitochondria-penetrating characteristic and after uptake to the cell with transporting of AgNP across membranes of this organelle, cause in cell toxicity. Overall, peptides modified AgNPs show significant enhancement in killing tumor cells and could be a new class of nano-drug for cancer treatment.
Funding information
The financial support from the “Drug Applied Research Center” and “Research Center for Pharmaceutical Nanotechnology” of Tabriz University of Medical Sciences is greatly acknowledged.
Disclosure statement
The authors report no conflicts of interest.
References
- AshaRani PV, Low Kah Mun G, Hande MP, Valiyaveettil S. 2008. Cytotoxicity and genotoxicity of silver nanoparticles in human cells. ACS Nano. 3:279–290.
- Bechara C, Pallerla M, Zaltsman Y, Burlina F, Alves ID, Lequin O, et al. 2013. Tryptophan within basic peptide sequences triggers glycosaminoglycan-dependent endocytosis. FASEB J. 27:738–749.
- Bondar OV, Saifullina DV, Shakhmaeva II, Mavlyutova II, Abdullin TI. 2012. Monitoring of the zeta potential of human cells upon reduction in their viability and interaction with polymers. Acta Naturae. 4:78.
- Farkhani SM, Johari-ahar M, Zakeri-Milani P, Shahbazi Mojarrad J, Valizadeh H. 2015a. Enhanced cellular internalization of CdTe quantum dots mediated by arginine- and tryptophan-rich cell-penetrating peptides as efficient carriers. Artif Cells Nanomed Biotechnol. 44. [Epub ahead of print]. doi: 10.3109/21691401.2015.1031906..
- Farkhani SM, Shirani A, Mohammadi S, Zakeri-Milani P, Mojarrad JS, Valizadeh H. 2015b. Effect of poly-glutamate on uptake efficiency and cytotoxicity of cell penetrating peptides. IET Nanobiotechnol. 10:87–95.
- Farkhani SM, Valizadeh A, Karami H, Mohammadi S, Sohrabi N, Badrzadeh F. 2014. Cell penetrating peptides: efficient vectors for delivery of nanoparticles, nanocarriers, therapeutic and diagnostic molecules. Peptides. 57:78–94.
- Gengan RM, Anand K, Phulukdaree A, Chuturgoon A. 2013. A549 lung cell line activity of biosynthesized silver nanoparticles using Albizia adianthifolia leaf. Colloids Surf B Biointerfaces. 105:87–91.
- Horton KL, Stewart KM, Fonseca SB, Guo Q, Kelley SO. 2008. Mitochondria-penetrating peptides. Chem Biol. 15:375–382.
- Huang H-C, Barua S, Sharma G, Dey SK, Rege K. 2011. Inorganic nanoparticles for cancer imaging and therapy. J Control Release. 155:344–357.
- Jeyaraj M, Sathishkumar G, Sivanandhan G, MubarakAli D, Rajesh M, Arun R, et al. 2013. Biogenic silver nanoparticles for cancer treatment: an experimental report. Colloids Surf B Biointerfaces. 106:86–92.
- Jobin M-L, Blanchet M, Henry S, Chaignepain S, Manigand C, Castano S, et al. 2015. The role of tryptophans on the cellular uptake and membrane interaction of arginine-rich cell penetrating peptides. Biochim Biophys Acta. 1848:593–602.
- Kaur J, Tikoo K. 2013. Evaluating cell specific cytotoxicity of differentially charged silver nanoparticles. Food Chem Toxicol. 51:1–14.
- Koshkaryev A, Piroyan A, Torchilin VP. 2013. Bleomycin in octaarginine-modified fusogenic liposomes results in improved tumor growth inhibition. Cancer Lett. 334:293–301.
- Kumar A, Ma H, Zhang X, Huang K, Jin S, Liu J, et al. 2012. Gold nanoparticles functionalized with therapeutic and targeted peptides for cancer treatment. Biomaterials. 33:1180–1189.
- Li X, Zhang J, Xu W, Jia H, Wang X, Yang B, et al. 2003. Mercaptoacetic acid-capped silver nanoparticles colloid: formation, morphology, and SERS activity. Langmuir. 19:4285–4290.
- Liu BR, Winiarz JG, Moon J-S, Lo S-Y, Huang Y-W, Aronstam RS, et al. 2013. Synthesis, characterization and applications of carboxylated and polyethylene-glycolated bifunctionalized InP/ZnS quantum dots in cellular internalization mediated by cell-penetrating peptides. Colloids Surf B Biointerfaces. 111:162–170.
- Liu J, Zhao Y, Guo Q, Wang Z, Wang H, Yang Y, et al. 2012. TAT-modified nanosilver for combating multidrug-resistant cancer. Biomaterials. 33:6155–6161.
- Liu W, Wu Y, Wang C, Li HC, Wang T, Liao CY, et al. 2010. Impact of silver nanoparticles on human cells: effect of particle size. Nanotoxicology. 4:319–330.
- Mandal D, Nasrolahi Shirazi A, Parang K. 2011. Cell‐penetrating homochiral cyclic peptides as nuclear‐targeting molecular transporters. Angew Chem Int Ed. 50:9633–9637.
- Mei L, Lu Z, Zhang W, Wu Z, Zhang X, Wang Y, et al. 2013. Bioconjugated nanoparticles for attachment and penetration into pathogenic bacteria. Biomaterials. 34:10328–10337.
- Mussa Farkhani S, Valizadeh A. 2014. Review: three synthesis methods of CdX (X = Se, S or Te) quantum dots. IET Nanobiotechnol. 8:59–76.
- Nakase I, Konishi Y, Ueda M, Saji H, Futaki S. 2012. Accumulation of arginine-rich cell-penetrating peptides in tumors and the potential for anticancer drug delivery in vivo. J Control Release. 159:181–188.
- Nakase I, Tanaka G, Futaki S. 2013. Cell-penetrating peptides (CPPs) as a vector for the delivery of siRNAs into cells. Mol Biosyst. 9:855–861.
- Park H, Tsutsumi H, Mihara H. 2013. Cell penetration and cell-selective drug delivery using α-helix peptides conjugated with gold nanoparticles. Biomaterials. 34:4872–4879.
- Rydberg HA, Matson M, Amand HL, Esbjorner EK, Norden B. 2012. Effects of tryptophan content and backbone spacing on the uptake efficiency of cell-penetrating peptides. Biochemistry. 51:5531–5539.
- Sanpui P, Chattopadhyay A, Ghosh SS. 2011. Induction of apoptosis in cancer cells at low silver nanoparticle concentrations using chitosan nanocarrier. ACS Appl Mater Interfaces. 3:218–228.
- Sriram MI, Kanth SBM, Kalishwaralal K, Gurunathan S. 2010. Antitumor activity of silver nanoparticles in Dalton’s lymphoma ascites tumor model. Int J Nanomed. 5:753.
- Sukirtha R, Priyanka KM, Antony JJ, Kamalakkannan S, Thangam R, Gunasekaran P, et al. 2012. Cytotoxic effect of Green synthesized silver nanoparticles using Melia azedarach against in vitro HeLa cell lines and lymphoma mice model. Proc Biochem. 47:273–279.
- Sur I, Altunbek M, Kahraman M, Culha M. 2012. The influence of the surface chemistry of silver nanoparticles on cell death. Nanotechnology. 23:375102.
- Sur I, Cam D, Kahraman M, Baysal A, Culha M. 2010. Interaction of multi-functional silver nanoparticles with living cells. Nanotechnology. 21:175104.
- Toy R, Bauer L, Hoimes C, Ghaghada KB, Karathanasis E. 2014. Targeted nanotechnology for cancer imaging. Adv Drug Deliv Rev. 76:79–97.
- Xu Y, Liu BR, Lee HJ, Shannon KB, Winiarz JG, Wang TC, et al. 2010. Nona-arginine facilitates delivery of quantum dots into cells via multiple pathways. J Biomed Biotechnol. 2010:948543.
- Yallapu MM, Jaggi M, Chauhan SC. 2012. Curcumin nanoformulations: a future nanomedicine for cancer. Drug Discov Today. 17:71–80.
- Yuan H, Fales AM, Vo-Dinh T. 2012. TAT peptide-functionalized gold nanostars: enhanced intracellular delivery and efficient NIR photothermal therapy using ultralow irradiance. J Am Chem Soc. 134:11358–11361.