Abstract
The purpose of the present study was to develop loteprednol etabonate (LE) loaded poly(d,l-lactide co-glycolide) (PLGA) nanoparticles (NPs) and study their penetration profile into the excised goat cornea. In the present study, LE loaded PLGA NPs were prepared by solvent evaporation with high speed homogenization method and the penetration profile was studied using confocal laser scanning microscopy (CLSM). Rhodamine (Rd) was used as a fluorescent marker to prepare Rd-LE–PLGA-NPs. The NPs were characterized for particle size, X-ray diffraction (XRD), differential scanning calorimetry (DSC), transmission electron microscopy (TEM), drug entrapment, and permeation profile. Intense fluorescence observed across the depths of goat corneal tissue suggested an improved penetration profile of NPs. The entrapment efficiency and mean diameter of the optimized formulation (F5) were found to be 96.31 ± 1.68% and 167.6 ± 0.37 nm, respectively. These findings indicate that LE loaded PLGA NPs may serve as a potential drug carrier for ocular administration in eye disease.
Introduction
The treatment of ophthalmic medical complications has been achieved with various steroidal anti-inflammatory agents. Betamethasone sodium phosphate has been the drug of choice for the management of ocular inflammation, but this conventional steroidal anti-inflammatory agent, has the propensity to increase intra-ocular pressure (IOP) (Yasueda et al. Citation2004). Loteprednol etabonate (LE) is a topical steroidal anti-inflammatory agent used for the effective management of ocular inflammation. For topical ophthalmic applications, liquid eye drops like suspension, emulsion and solution are favored due to ease in application with minimal alteration in vision. But these formulations are associated with some limitations like rapid drainage via nasolacrimal route, quick dilution by tearing and systemic absorption which pilot the systemic side effects (Rafie et al. Citation2010). The constraints posed by the pharmacokinetic profile of the drug clearly warrant the need for novel carriers to overcome these barriers. Polymeric nanoparticles (NPs) offer promising benefits in terms of biodegradability, biocompatibility, non-toxic, and sustained drug release profile (Soppimath et al. Citation2001). Biodegradable polymers are widely studied for the development of various nanoparticulate carrier systems for ophthalmic application, primarily due to their potential to improve the residence time of formulation on precorneal surface (Kaur et al. Citation2012). Poly(d,l-lactide-co-glycolide) (PLGA) is a biodegradable polymer which is approved by FDA for ophthalmic use and have large potential for ocular drug delivery applications (Soppimath et al. Citation2001).
Confocal laser scanning microscopy (CLSM) is a distinctive technique for direct visualization of penetration pathway and depth of penetration of the carriers in excised tissue (Sarwa et al. Citation2014). This technique is devoid of mechanical section and a laser beam is used as the energy source which acts as an optical knife to section tissue at multiple depths. It also allows non-invasive visual evaluation of dynamic changes in real time and the images of optical sections can be captured with reasonable time-resolution. A fluorescent marker is used to label the sample which on interaction with the laser beam moves to an excited state and emits light of a specific wavelength (Sarwa et al. Citation2014). Goat cornea is a well-established model for the permeation as well as depth of penetration studies and is widely reported (Ahuja et al. Citation2006, Pescina et al. Citation2015, Yadav and Ahuja Citation2010). Goat cornea has been used for permeation studies of various nanocarriers like NPs (Gupta et al. Citation2010, Jain et al. Citation2011, Katara and Majumdar Citation2013, Kaur et al. Citation2012, Zhang et al. Citation2009) and aqueous drops (Mohanty et al. Citation2013, Rathore and Majumdar Citation2006). Based on the above facts and considerations, the present study focused on the development of PLGA loaded LE NPs and their ability to transform drug permeation and penetration profile was assessed in excised goat cornea using confocal laser scanning and optical digital microscope.
Materials and methods
Materials
Loteprednol etabonate and PLGA were kindly supplied as a gift sample by M/s Sun Pharma Advance Research Center (SPARC), Ahmedabad, Gujarat, India and Evonik Degassa, Mumbai, India, respectively. Polyvinyl alcohol (PVA), Rhodamine red, and acetone were purchased from HiMedia Pvt. Ltd., Mumbai, India. All other chemicals used were of analytical reagent grade and were used as received.
Preparation of nanoparticles
Loteprednol etabonate loaded PLGA NPs were prepared by modified emulsification/solvent diffusion method, with a minor alteration (Sabzevari et al. Citation2013). Drug/polymer blend (100 mg) at different ratios of 1:1, 1:2 and 1:3 (w/w) was dissolved in 2 ml mixture of acetone and dichloromethane (60:40). The above mixture was slowly injected into 20 ml of PVA solution (1%) at a flow rate of 0.5 ml/min and subjected to high speed homogenization at 15,000 rpm for 10 min (T-10 basic Ultra-Turrax® – IKA, Germany). The resultant dispersion was immediately ultrasonicated at 50 W for 5 min using a probe sonicator (Frontline Sonicator). The homogenization and sonication of the dispersion were carried out over an ice bath. The mixture was left on magnetic stirrer overnight for evaporation of the organic solvent. Thereafter, PLGA-LE-NP dispersion was allowed to centrifuge at 15,000 rpm at 20 °C for 30 min (Remi, Mumbai, India) and the supernatant was discarded to obtain the pellet of PLGA-LE-NPs. The composition of the various NP formulations is presented in .
Table 1. Formulation code and composition used for the PLGA nanoparticles.
Characterization of nanoparticles
Particle size, zeta potential, and polydispersity index (PDI)
The mean particle size (z-average), size distribution, zeta potential and PDI of the NPs were assessed by photon correlation spectroscopy using a Zetasizer NanoZS 90 (Malvern Instruments, UK). For the determination of particle size, the NP dispersion sample was diluted with ultra-pure distilled water (Millipore, Billerica, MA). The measurements were performed at 25 °C with a detection angle of 90°.
Morphology
The morphology of the prepared NP was studied by transmission electron microscopy (TEM) (Hitachi TEM H7500, Japan). The samples of NPs were placed on carbon-coated copper grids with 1% aqueous solution of phosphotungstic acid (PTA) for negative staining and viewed under the microscope at an accelerated voltage of 100 kV (Das et al. Citation2010, Sah et al. Citation2011).
Differential scanning calorimetry (DSC)
Differential scanning calorimetry of various samples viz., pure drug (LE), polymer PLGA 50:50, PVA, physical mixture of drug:polymer (1:1) and optimized formulation of LE-NP was performed using differential scanning calorimeter (Perkin Elmer Jade, CA). The samples were placed into the aluminum pans and were crimped for effective heat conduction. The samples were scanned in the temperature range of 50–300 °C at a heating rate of 20 °C min−1. The thermograms obtained were reviewed for evidence of interactions.
X-ray diffraction (XRD)
The sample was characterized for XRD patterns by using X-ray diffractometer (PANalytical fitted with XPERT-PRO Diffractometer System, Germany) at 35 kW with 20 mA over the 2θ range of 5–70°. The scanning was done at a rate of 1°min−1 (20θ).
Entrapment efficiency
The total percentage of drug entrapped (% EE) was determined by spectrophotometric analysis (UV 1700, Shimadzu, Japan). The unentrapped drug in the dispersion was separated by centrifuging the sample at 13,000 rpm, 4 °C for 20 min (Cooling Centrifuge, Remi Instruments Ltd., Mumbai, India) (Das et al. Citation2010). The supernatant was decanted and the concentration of LE in the supernatant was determined spectrophotometrically at 242 nm (λmax). Each experiment was conducted in triplicate. The % EE was calculated by:
Formulation optimization
On the basis of dependent and independent variables, nine formulations were prepared and optimized on the basis of characterization parameter.
Corneal penetration studies
Preparation of excised cornea
Ex vivo penetration studies were performed on excised goat cornea. Fresh excised eyeball was procured from freshly slaughtered goat at a local abattoir (Raipur, India). The cornea was excised and washed with cold simulated tear fluid (STF). The excised goat cornea was mounted on the modified glass Franz diffusion cell.
Penetration assessment by CLSM
The depth of penetration of the formulation loaded with rhodamine into the excised cornea was examined by CLSM (Leica TCS SP2, Heidelberg, Germany). Following the permeation studies, the excised cornea was immediately removed from the Franz diffusion cell and directly mounted, epithelial side up, on the glass slide and observed without any tissue treatment directly under the CLSM. The full thickness of corneal epithelium tissue was optically scanned at depths of 10–70 μm through the Z-axis of the attached inverted fluorescence microscope (DMIRE 2, CLSM, Germany). The sample was optically excited with argon 488 nm laser beam.
Ocular tolerance of PLGA-NPs
Ocular tolerance of prepared formulation was examined histopathologically by assessing the integrity of epithelial cells and changes in the cellular structure. The tissue was collected after the permeation study, and immediately treated with the formalin solution (8% w/w), dehydrated with alcohol and placed in melted paraffin and solidified in block form. The block was cut into cross-sections and stained with hematoxylin and eosin. These specimens were observed under light microscope (Digi 2, Labomed, Germany) at 10–40× and photographed with a digital camera.
Ex vivo transcorneal permeation studies
Ex vivo transcorneal permeation profile of optimized PLGA-LE-NPs formulation was assessed on excised goat cornea (Gupta et al. Citation2010). The study was carried out in modified glass Franz diffusion cell apparatus. The excised goat cornea was fixed between the donor and receptor compartment with the epithelial side facing the donor compartment. The corneal area available for permeation was 0.50 cm2. The lower chamber served as receptor compartment and was filled with fresh STF solution (pH 7.4) and maintained at 37 °C ± 0.5 °C with continuous stirring at 100 rpm. Optimized LE-NPs aqueous dispersion (2 ml) was placed in the donor compartment, and at periodic time intervals, aliquots of 2 ml were withdrawn from the receptor compartment and replaced with 2 ml of fresh dissolution medium to maintain the sink conditions. The release medium was analyzed for LE content by UV spectrophotometry (Shimadzu UV-1700, Japan) at 242 nm.
Stability studies
The stability studies of optimized LE-NPs formulations in dispersed state were carried out for three months. The storage conditions employed were ambient (25 ± 1 °C), refrigeration (4 ± 1 °C), and accelerated temperature (45 ± 1 °C). The formulations were stored in well-closed amber colored glass containers. At predetermined time intervals, samples were withdrawn and evaluated for entrapment efficiency and particle size.
Statistical analysis
All the data were presented as mean ± (SEM) and statistically data were treated by Student’s t-test using GraphPad Prism 5 software (GraphPad Software Inc., San Diego, CA). P < 0.05 was considered significant.
Results and discussion
Preparation of nanoparticles
Modified emulsification/solvent diffusion method is one of the most preferred method for preparation of polymeric NPs at laboratory scale due to its feasibility and ease of preparation. In the present study, PLGA (50:50) and PVA were used as biodegradable polymer and thickening agent, respectively. The homogenization speed was optimized at 20,000 rpm for 30 min and the sonication time was 15 min at 50 W.
Particle size, zeta potential, and polydispersity index (PDI)
The particles of the nano-dimension formulations were in size ranging 167.6–511.6 nm. The zeta potential was in the range of −7.41 to −16.17 mv. The size distribution was almost monodisperse in all the dispersions with PDI in the range of 0.11–0.43 ().
Table 2. Mean particle size, zeta potential, and polydispersity index of various formulations.
Differential scanning calorimetry
Differential scanning calorimetry studies were performed to investigate the drug–excipient interaction in the formulation (Sahu et al. Citation2014). The DSC thermogram was obtained by running samples of pure drug LE, PLGA (50:50), physical mixture of LE and PLGA at 1:1 ratio and LE loaded freeze dried PLGA NPs ().
Figure 1. Differential scanning calorimetry thermogram. (A) Drug-loteprednol etabonate, (B) poly(d,l-lactide-co-glycolide), (C) physical mixture of drug and polymer, and (D) poly(d,l-lactide-co-glycolide) loaded loteprednol etabonate nanoparticles.
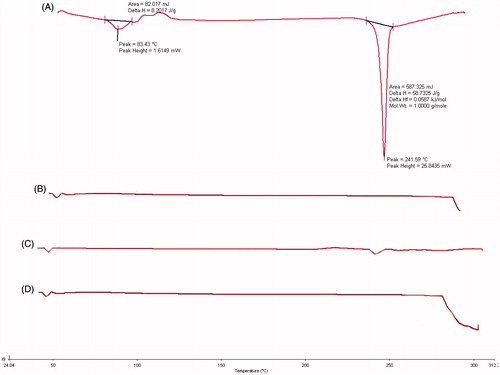
The melting point of LE was observed in the range of 225–240 °C which indicated crystalline anhydrous nature of the drug. The DSC thermogram presented the glass transition temperature of PLGA as 50 °C. There were less intense and broader peaks at the position of endothermic peaks with physical mixture of LE–PLGA in the ratio of 1:1 and indicated point at 240 °C. Thus, no chemical interaction was evident between LE and PLGA. Also no drug peak was observed in LE–PLGA-NP formulation due to dilution factor and entrapment of drug in polymeric matrix. It indicated stability of the nanosystem (Gupta et al. Citation2010).
XRD studies
XRD was performed to assess the state of encapsulated drug and to confirm whether it was dispersed in microcrystalline form, without polymorphic change or transition change in amorphous form. depicts the diffractograms of the pure drug, polymer, physical mixture, and NP. Drug peaks in the formulation were suppressed and this may be attributed to solubility of drug in the polymer solution and higher polymer concentration. The peaks of formulation were similar to polymer peaks, indicating that the drug was completely mixed with the polymer and entrapped within the polymer matrix.
Transmission electron microscopy
The morphological characteristics, shape and size of the optimized formulation of LE–PLGA-NP, were assessed by TEM (). TEM indicated smooth and spherical surface morphology of the NPs. Photomicrograph confirmed that the particles were spherical in shape with narrow size distribution without any aggregation.
Entrapment efficiency (EE)
The EE of all nine formulations of LE–PLGA-NPs is depicted in and was found to be in the range of 71.35%–96.31%. Ratio of drug:polymer and nature of drug played a significant role on EE, and it may be due to solubility of drug in the polymeric phase.
Formulation optimization
Formulation F5 was selected as an optimized formulation on the basis of characterization parameter ().
Penetration study by CLSM
To assess the depth of penetration of NP into the deeper layers of cornea, the cross-section of corneal tissue was examined by CLSM. The confocal image of rhodamine labeled PLGA–LE NPs is depicted in . Depth of penetration of corneal epithelium was assessed at 10–70 μm and it can be clearly visualized from the CLSM image that nanoformulations penetrated into the deeper corneal layers. The penetration efficacy was further confirmed by optical intensity micrograph between the depth of tissue and intensity ().
Figure 4. Confocal images of excised goat cornea illustrating the depth penetration of rhodamine labeled nanoparticle into the deeper layers of corneal epithelium at (10–75 μm). The uniform fluorescence intensity was observed from first to last section.
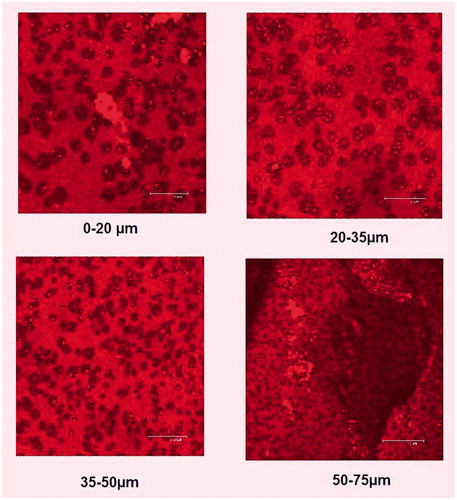
Figure 5. Confocal laser scanning micrograph of poly(d,l-lactide-co-glycolide) loaded loteprednol etabonate nanoparticles penetrated across the excised goat cornea in which X-axis and Y-axis show the depth of penetration and intensity of fluorescence, respectively.
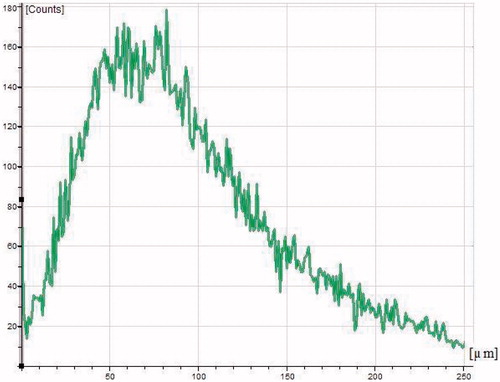
Ocular tolerance
The histopathological studies confirmed the absence of alteration in epithelial structures with cells maintaining normal morphology. This indicated that the formulation was well-tolerated. represents the histopathological slide of goat corneal tissue confirming the presence of normal cell structures, an indicator of the maintenance of integrity of corneal cell.
Ex vivo transcorneal permeation studies
The ex vivo transcorneal permeation of optimized formulation (F5) and plain drug suspension (DS) was conducted on excised goat cornea for a period of 4 h (). The nanoformulation showed higher permeation across the cornea after 4 h (55.32%) as compared to the pure drug suspension (38.21%) (Aukunuru and Kompella Citation2002, Calvo et al. Citation1994, Gupta et al. Citation2010, Qaddoumi et al. Citation2003, Rafie et al. Citation2010, Souza et al. Citation2013).
Stability studies
Stability studies of optimized F5 formulations of LE–PLGA-NP were performed for three months at three storage temperatures (4 ± 1 °C, 25 ± 1 °C, and 45 ± 1 °C) and were protected from exposure to light and concomitant photodegradation. The change in particle size and the EE were assessed as the prime parameters. There was a small alteration in size distribution with a slight increment as compared with initial particle size () (Pignatello et al. Citation2002). During storage, the particle size changed from 167.6 ± 0.37 to 169 ± 0.47, 167.6 ± 0.37 to 169.51 ± 0.52 and 167.6 ± 0.37 to 176 ± 0.59 at 4 ± 1 °C, 25 ± 1 °C and 45 ± 1 °C, respectively. In contrast, % EE within the NPs was also remarkable high (96.31 ± 1.68) when stored at 4 ± 1 °C as compared with the room temperature 95.4 ± 0.87, and 84.6 ± 7.63 at accelerated temperature after three month.
Table 3. Effect of different temperature on the particle size and entrapment efficiency of drug loaded PLGA-NPs. Results are presented as mean ± SD (n = 3).
Discussion
In the present work, LE loaded PLGA NPs were prepared using modified emulsification/solvent diffusion method and their penetration profile were investigated on excised goat cornea. The benefits of these nanosystems in ocular drug delivery include capability of intimate contact with the precorneal segment, with improved delivery to the anterior segment of ocular tissue without compromising the internal structure of the eye and thus providing long-term therapeutic drug level.
On the basis of characterization parameters, the formulation F5 was selected as optimized formulation. Owing to the low particle size (167.6 nm), this novel formulation has the potential to easily penetrate into the excised cornea. Additionally, particle size less than 250 nm and PDI near to 0.25 have been considered as safe and optimum for ophthalmic application (Aukunuru and Kompella Citation2002, Kohane et al. Citation2006). Zeta potential is an important physicochemical parameter determining the stability of the nanosystem. Extreme positive or negative values result in high repulsive force, while optimum repulsion between similar charged particles prevent the aggregation and confirms the redispersibility (Mainardes and Evangelista Citation2005, Muller and Jacob Citation2002). The optimized formulation showed −16.17 mV zeta potential value which is in accordance with the observations of Kohane et al. (Citation2006). DSC and XRD investigations were carried out to assess if the drug was incorporated into the nanoparticulate system in a crystalline or amorphous state. The studies confirmed that the drug was completely encapsulated into the nanoparticulate system and the nanosystem comprised of a homogenous drug–polymer mixture without any interaction between the two components. The NPs was further studied by TEM which showed the nano and spherical size of the particles. Depth of penetration was analyzed by CLSM and it is apparent from the image that the NPs accumulated not only in the first layer of corneal epithelium, but also penetrated into the stroma and deeper endothelial layer of the cornea which is in contact with the anterior chamber of the eye. These studies clearly demonstrate that LE loaded polymeric NPs have the potential to accumulate in all segments of cornea, with subsequent LE release and thereby allowing better therapeutic action.
Ocular tolerance is the key feature to designate the formulation as safe for corneal cells. After the application of prepared PLGA-LE-NPs, it was observed that the formulation did not alter the normal arrangement of cellular membrane and there were no apparent changes in the corneal epithelium. These observations advocate that the prepared nanoformulation would be well-tolerated and safe for corneal surface.
The ex vivo transcorneal permeation was carried out, which indicated the improved permeation profile of the optimized formulation as compared with the plain drug solution. It may be attributed to the higher retention of the PLGA NPs in the corneal surface due to their nano-dimensions (Aukunuru and Kompella Citation2002, Calvo et al. Citation1994, Qaddoumi et al. Citation2003). Additionally smaller size offers several benefits like improved solubility, higher surface area, dissolution rate, corneal penetration, and bioadhesion of the nanomaterial (Rafie et al. Citation2010). Particle size smaller than 10 μm lowered eye irritation profile, tearing, and drainage of the administered drug as compared to larger particle with improved therapeutic profile in the treatment of the disease (Souza et al. Citation2013). Moreover, PVA is a swellable, hydrophilic macromolecule, and it stabilized the PLGA which may also facilitate retention of NP in the eye (Gupta et al. Citation2010). In addition, CLSM image of rabbit conjuctival epithelial cells exhibited improved uptake of particle with 100 nm as compared with 800–1000 nm (Qaddoumi et al. Citation2004). The stability studies of optimized LE-NPs formulations in dispersed state were carried out for three months. The storage conditions employed were ambient (25 ± 1 °C), refrigerated (4 ± 1 °C), and accelerated temperature (45 ± 1 °C). During the storage of the formulation for three months, there were minor changes in the particle size and it may be due to the formation of aggregates on storage (Pignatello et al. Citation2002). It was observed that there were negligible changes in particle size at freezing temperature while a slight increase in particle size was apparent at room temperature and significant changes at accelerated temperature was observed, suggesting that the formulation was more stable at freezing temperature due to controlled environmental factor. The lowering of % EE significantly during storage at 45 ± 1 °C might be due to rupturing of the polymer sheath at high temperature. Thus, optimized formulation was found to be more stable at two different temperatures for three months.
Conclusion
In the present investigation, LE–PLGA-NPs were developed for topical ophthalmic application. It could be concluded that LE–PLGA-NPs possessed better penetrability across excised goat cornea and adhered to the ocular surface for prolonged period of time as compared to the pure drug suspension. The CLSM gave substantial evidence that drug from NPs penetrated into the deeper layers of the cornea. Histopathological studies corroborated that nanoformulation assisted in maintaining the normal morphological structure after application and no alteration in cellular structure was observed. CLSM and optical microscopy presented insight into the penetration and fate of NPs. Further in vivo studies may be required to quantify these results and establish its reproducibility at the backdrop of other biological factors.
Funding information
One of the authors (AKS) is thankful to the University Grant Commission (UGC) New Delhi, India, for awarding the Senior Research Fellowship (SRF) during the doctoral program. Authors are also grateful to the Director, University Institute of Pharmacy, Pt. Ravishankar Shukla University, Raipur (C.G.), India for the infrastructure facilities. The authors extend their sincere thanks to Dr. Padam Jain, Senior Veterinary Surgeon for his valuable assistance in conducting corneal excision. Authors acknowledge Department of Physiology, All India Institute of Medical Science (AIIMS), New Delhi, India and School of Studies in Biotechnology, Pt. Ravishankar Shukla University, Raipur (C.G.) for CLSM and Histopathology studies, respectively. The authors gratefully acknowledge Sophisticated Analytical Instrument Facility (SAIF), Punjab University for TEM facility and M/s Sun Pharma Advance Research Center (SPARC), Ahmadabad, Gujarat, India for providing the gift samples of Loteprednol etabonate.
Disclosure statement
The authors report that this article has no conflict of interest.
References
- Ahuja M, Dhake AS, Majumdar DK. 2006. Effect of formulation factors on in-vitro permeation of diclofenac from experimental and marketed aqueous eye drops through excised goat cornea. Yakugaku Zasshi. 126:1369–1375.
- Aukunuru JV, Kompella UB. 2002. In vitro delivery of nano and microparticles to retinal pigment epithelial (RPE) cells. Drug Del Technol. 2:50–57.
- Calvo P, Thomas C, Alonso MJ, Vila Jato JL, Robinson J. 1994. Study of the mechanisms of interaction of poly E-caprolactone nanocapsules with the cornea by confocal laser scanning microscopy. Int J Pharm. 103:283–291.
- Das S, Suresh PK, Deshmukh R. 2010. Design of Eudragit RL 100 nanoparticles by nanoprecipitation method for ocular drug delivery. Nanomedicine. 6:318–323.
- Gupta H, Aquil M, Khar RK, Ali A, Bhatnagar A, Mittal G. 2010. Sparfloxacin-loaded PLGA nanoparticles for sustained ocular drug delivery. Nanomedicine. 6:324–333.
- Jain GK, Pathan SA, Akhter S, Jayabalan N, Talegaonkar S, Khar RK, Ahmad FJ. 2011. Microscopic and spectroscopic evaluation of novel PLGA-chitosan nanoplexes as an ocular delivery system. Colloids Surf B Biointerfaces. 82:397–403.
- Katara R, Majumdar DK. 2013. Eudragit RL 100-based nanoparticulate system of aceclofenac for ocular delivery. Colloids Surf B Biointerfaces. 103:455–462.
- Kaur H, Ahuja M, Kumar S, Dilbaghi N. 2012. Carboxymethyl tamarind kernel polysaccharide nanoparticles for ophthalmic drug delivery. Int J Biol Macromol. 50:833–839.
- Kohane DS, Tse JY, Yeo Y, Padera R, Shubina M, Robert L. 2006. Biodegradable polymeric microspheres and nanospheres for drug delivery in the peritoneum. J Biomed Mater Res A. 77:351–361.
- Mainardes RM, Evangelista RC. 2005. PLGA nanoparticles containing praziquantel: effect of formulation variables on size distribution. Int J Pharm. 290:137–144.
- Mohanty B, Mishra SK, Majumdar DK. 2013. Effect of formulation factors on in vitro transcorneal permeation of voriconazole from aqueous drops. J Adv Pharm Technol Res. 4:210–216.
- Muller RH, Jacob C. 2002. Production and characterization of a budenoside nanosuspension for pulmonary administration. Pharm Res. 19:189–194.
- Pescina S, Govoni P, Potenza A, Padula C, Santi P, Nicoli S. 2015. Development of a convenient ex vivo model for the study of the transcorneal permeation of drugs: histological and permeability evaluation. J Pharm Sci. 104:63–71.
- Pignatello R, Bucolo C, Ferrar P, Maltese A, Puleo A, Puglisi G. 2002. Eudragit RS100 nanosuspensions for the ophthalmic controlled delivery of ibuprofen. Eur J Pharm Sci. 16:53–61.
- Qaddoumi MG, et al. 2004. The characteristics and mechanisms of uptake of PLGA nanoparticles in rabbit conjunctival epithelial cell layers. Pharm Res. 21:641–648.
- Qaddoumi MG, Gukasyan HJ, Davda J, Labhasetwar V, Kim KJ, Lee HLV. 2003. Clathrin and caveolin-1 expression in primary pigmented rabbit conjunctival epithelial cells: role in PLGA nanoparticle endocytosis. Mol Vision. 9:559–568.
- Rafie F, Javadzadeh Y, Javadzadeh AR, Ghavidel LA, Jafari B, Moogooee M, Davaran S. 2010. In vivo evaluation of novel nanoparticles containing dexamethasone for ocular drug delivery on rabbit eye. Curr Eye Res. 35:1081–1089.
- Rathore MS, Majumdar DK. 2006. Effect of formulation factors on in vitro transcorneal permeation of gatifloxacin from aqueous drops. AAPS PharmSciTech. 7:57.
- Sah AK, Jain SK, Pandey RS. 2011. Microemulsion based hydrogel formulation of methoxsalen for the effective treatment of psoriasis. Asian J Pharm Clin Res. 4:140–145.
- Sahu AK, Kumar T, Jain V. 2014. Formulation optimization of erythromycin solid lipid nanocarrier using response surface methodology. BioMed Res Int. 2014. doi:http://dx.doi.org/10.1155/2014/689391.
- Sarwa KK, Suresh PK, Rudrapal M, Verma VK. 2014. Penetration of tamoxifen citrate loaded ethosomes and liposomes across human skin: a comparative study with confocal laser scanning microscopy. Curr Drug Deliv. 11:332–337.
- Sabzevari A, Adibkia K, Hashemi H, Hedayatfar A, Mohsenzadeh N, Atyabi F, Ghahremani MH, Dinarvand R. 2013. Polymeric triamcinolone acetonide nanoparticles as a new alternative in the treatment of uveitis: in vitro and in vivo studies. Eur J Pharm Biopharm. 84:63–71.
- Soppimath KS, Aminabhavi TM, Kulkarni AR, Rudzinski WE. 2001. Biodegradable polymeric nanoparticles as drug delivery devices. J Control Release. 70:1–20.
- Souza JG, Dias K, Pereira TA, Bernardi DS, Lopez RFV. 2013. Topical delivery of ocular therapeutics: carrier systems and physical methods. J Pharm Pharmacol. 66:507–530.
- Yadav M, Ahuja M. 2010. Preparation and evaluation of nanoparticles of gum cordia, an anionic polysaccharide for ophthalmic delivery. Carbohydr Polym. 81:871–877.
- Yasueda SI, Higashiyama M, Shirasaki Y, Inada K, Ohtori A. 2004. An HPLC method to evaluate purity of a steroidal drug, loteprednol etabonate. J Pharm Biomed Anal. 36:309–316.
- Zhang L, Li Y, Zhang C, Wang Y, Song C. 2009. Pharmacokinetics and tolerance study of intravitreal injection of dexamethasone-loaded nanoparticles in rabbits. Int J Nanomed. 4:175–183.