Abstract
In this work, microemulsion-based gels were prepared for transdermal delivery of paeonol. Microemulsions containing eutectic mixtures of paeonol and menthol were developed. The obtained microemulsions were evaluated for particle size, viscosity and physical stability. The selected microemulsions were incorporated into Carbopol gels. Drug crystallization behavior during a short-term storage was compared and in vitro permeation and deposition study were conducted on mouse skin. Results showed that the eutectic liquids of paeonol and menthol at all ratio (6:4, 5:5 and 4:6) could form microemulsions but with significantly different physical characteristics. As the ratio of paeonol increased, the prepared microemulsions exhibited larger droplet size, higher viscosity and quicker crystal growth. Microemulsion containing paeonol and menthol at a ratio of 4:6 possessed the smallest size of 27 nm. Accordingly, the related gel showed better physical stability during 10 days of storage, as well as the highest percent of drug deposition (111.8 μg/cm2) and steady-state flux (0.3 μg/cm2 h). These results suggested that the microemulsion formulation is a preferable approach for enhanced skin permeation, and the microemulsion based on drug–menthol eutectic mixture might be used as a potential transdermal delivery system for better therapeutic efficacy.
Introduction
The eutectic system is defined as a mixture of two or more substances with a melting point lower than that of any of its constituents (Cherukuvada and Nangia Citation2014). Eutectic mixtures are widely used in drug designing and delivery processes (Cherukuvada and Nangia Citation2014). In the past two decades, more reports become to focus on the application of eutectic mixtures in improving solubility (Morrison et al. Citation2009), dissolution (Aroso et al. Citation2016) and bioavailability of poorly water soluble drugs (Tarate and Bansal Citation2015). And their enhancement effects on skin penetration have aroused special concern of researchers (Stott et al. Citation1998).
Eutectic mixtures can be formed between excipients [borneol and menthol (Shen et al. Citation2011)], between drug and excipient [Ibuprofen and menthol (Yong et al. Citation2003)] or between drugs [lidocaine and prilocaine (Chun et al. Citation2012)]. Each of them has proven to be effective in facilitating drug transport through the skin corneum. For example, the eutectic mixture of camphor and menthol was used in a spray formulation of clotrimazole simultaneously as the solvent for film former materials and as the penetration enhancers (Paradkar et al. Citation2015). The inclusion of eutectic mixture was confirmed to be efficient in promoting drug release through rat skin and therefore improving its antifungal efficacy. The lidocaine–prilocaine (1:1) eutectic cream (EMLA®) is commercial available for local anesthesia, and its skin penetration was enhanced by up to six-fold compared to the silicone vehicle (Chun et al. Citation2012). Although the mechanism of the eutectic mixture as percutaneous penetration enhancer is still not fully understood, it may be explained in several aspects (Park et al. Citation2012): (i) it may cause leaching of the lipids in the skin and thereby formation of pores, (ii) it may depress the melting point of drug to below skin temperature thereby increase drug solubility. Furthermore, the combination usage of the eutectic mixtures and some nanoscale drug vehicles [e.g. microemulsion, phospholipid microemulsion (Negi et al. Citation2016)] can lead to even better permeability. However, different ratios of same eutectic components were paid much less attention on their physical and transdermal properties.
The aim of this work was to investigate the influence of drug–excipient ratio in eutectic mixtures on physical characteristics (especially stability) and permeation performance of the preparations. Paeonol (an antioxidant and anti-inflammatory agent) and menthol (a commonly used skin penetration enhancer) were employed as the model drug and excipient. A series of paeonol–menthol eutectic liquids was introduced into microemulsion as the oil phase and further adopted into gels. The eutectic mixtures were then compared on drug solubility, droplet size, rheology and storage stability of microemulsion and/or gel formulations. Drug permeation and deposition on mouse skin were also investigated.
Materials and methods
Materials
Paeonol and menthol of 99% purity were purchased from Jingzhu Biology Technology Co., Ltd. (Nanjing, China) and Ruiyong Biology Technology Co., Ltd. (Shanghai, China), respectively. Cremophor EL was obtained from BASF Chemical Co., Ltd. (Florham Park, NJ); Carbopol 934 was supplied by Chineway Pharmaceutical Technology Co., Ltd. (Shanghai, China). Glycerol and methanol were from Damao Chemical Reagent Factory (Tianjin, China). Deionized water from a Millipore purification system (Millipore Synergy UV-R, Billerica, MA) was used for all experiments.
Preparation of eutectic mixtures
The eutectic mixtures of paeonol and menthol were prepared according to previous report (Chun et al. Citation2012). Briefly, paeonol and menthol (pae:men) at different ratios (4:6, 5:5 and 6:4) were accurately weighed into a glass tube. The powders were then mixed and heated to 40 °C. After melting and cooling, the eutectic samples were obtained and stored in a −4 °C refrigerator before use.
Saturated solubility test
The apparent solubility of paeonol and its eutectic mixtures with menthol was measured using the shaking flask method. Excess amount of each sample was added into a tube containing 1 ml water. The samples were then shaken for 72 h at 100 rpm in a THZ-100B air bath (Yiheng Scientific Instruments Co., Ltd, Shanghai, China). After centrifugation at 12,000 rpm for 5 min, the supernatant was immediately diluted with methanol and filtered through a 0.22 μm syringe filter for determination of drug content. The analysis was performed by a HPLC system (Agilent 1260, Agilent Technologies, Santa Clara, CA). The separation process was carried out on a Pypersil ODS2-C18 analytical column (5 μm, 4.6 × 250 mm) using a mixture of methanol and water (70:30, v/v) as the mobile phase at a flow rate of 1.0 ml/min. And the detection was performed at 274 nm using UV–Vis detector (G1315D 1260 DAD VL, Agilent Technologies).
Construction of phase diagrams
To determine the formulation of microemulsions based on eutectic mixtures, the pseudo-ternary phase diagrams were constructed by a water titration method (Luo et al. Citation2011, Boonme et al. Citation2006). The eutectic sample was accurately weighed, melted and directly applied as the oil phase. Surfactant (Cremophor EL) and co-surfactant (Glycerin) were mixed in 2:1 (w/w) ratio and used as the Smix. For each phase diagram, the oil and Smix were vortex-mixed at a series of weight ratios (1:9, 2:8, 3:7, 4:6, 5:5, 6:4, 7:3, 8:2 and 9:1). Then, the mixture was dropped with water under continuous vortexing, and the physical state of the system was observed and recorded. The percentage of each phase was calculated at different physical state, and the pseudo three-component phase diagram was then plotted using the Origin software (version 8.0).
Preparation of microemulsions and gels
According to the pseudo-ternary phase diagrams, a formulation with high level of oil phase were selected from the microemulsion area, and the components were listed in . Firstly, the eutectic mixture of paeonol and menthol was weighed and melted. Then, Cremophor EL and Glycerin were added and mixed, the microemulsions were obtained by dropping with hot water (40 °C) under vortexing. To facilitate the topical application and prolong the retention time on skin, Carbopol 934 (1%, w/v) was dispersed into the obtained microemulsions and stirred for 10 min. Finally, the microemulsion-based gels were obtained by further adjusting viscosity by adding 50 μl of 1 mol/L NaOH solution.
Table 1. Formulation of microemulsions containing paeonol–menthol eutectic mixture.
Another paeonol-loaded gel was also prepared as the control and its formulation was the same as F4. Paeonol was mixed with Cremophor EL, and menthol dissolved in glycerin and followed by adding hot water (40 °C) respectively. The two mixtures were poured together, added with Carbopol 934 and 50 μl NaOH solution, and then vortexed to obtain the paeonol gel.
Shape and size characterization of the microemulsions
The particle size and size distribution of microemulsions were analyzed by a NICOMPTM 380 Submicron Particle Analyzer (PSS Nicomp, Santa Barbara, CA) at 20, 30 and 40 °C, respectively. The morphology of droplets in microemulsion and gel was further investigated by a transmission electron microscopy (TEM, H-7650, Hitachi, Japan).
Rheological measurement of the microemulsions
A viscometer (Model DV-III, Brookfield, Stoughton, MA) was used to analyze the varying viscosity of the microemulsions at a constant shear rates (spindle #63, 50 rpm) while heating from 20 to 40 °C. For the measurements at different temperatures, the container with the microemulsions was immersed in a thermostat (with digital temperature setting with maximum displacements of ±0.1 °C). The viscosity was recorded at an interval of 1.0 °C and the experiment was repeated for three times.
Storage stability of the microemulsions and gels
The obtained microemulsions and gels were stored at room temperature for 10 days. At various time intervals, the changes in appearance of different microemulsions were observed. For the microemulsion-based gels, the possible crystallization behavior was observed by a reverse microscopy (Phoenix optics, Jiangxi, China).
In vitro skin permeation and drug-deposition study
The abdominal skin of Kunming mice (20–25 g) was excised, then the hair and subcutaneous tissues were removed. The skin permeation of microemulsion-based gels was studied using a vertical Franz diffusion cell (RYJ-6A, Tianjin, China) with an effective diffusion area of 2.89 cm2 and the receptor cell volume of 6.5 ml, respectively. The receiver medium (methanol:normal saline = 1:1, w/w) was constantly stirred at 300 rpm and the temperature was maintained at 32 ± 1 °C. An amount of each formulation equivalent to 28.9 mg of paeonol was placed in the donor compartment. At predetermined time intervals (0.5, 1, 2, 3, 4, 6, 8, 10, 12 and 24 h), an aliquot of 1 ml was withdrawn and immediately replaced by an equal volume of fresh medium. The samples were then analyzed for drug content by above-mentioned HPLC method. Similar experiments were performed with a conventional formulation of paeonol and menthol physical mixture as the control. The cumulative amount of drug penetrated per unit area (Qt, μg/cm2) versus time (t, h) was plotted. The steady-state flux (Jss, μg/cm2 h) was calculated from the slope of the linear portion of the plot. And the lag time (Tlag) was determined by extrapolating the plot at apparent steady state to the abscissa. The apparent permeability coefficients (P, cm/h) were calculated by dividing Jss with the initial donor drug concentration (C0, μg/ml).
At the end of the experiment, the skin surface of each specimen was washed with 5 ml of water for three times and then dried with filter paper. The skin tissues were cut into small pieces, added with 10 ml methanol and sonicated for 2 h followed by centrifugation for 5 min at 4000 rpm. After that, the supernatant was taken and filtered for high-performance liquid chromatography (HPLC) analysis. Drug deposition (Qs, μg/cm2) in the skin for each formulation was calculated by dividing the total drug amount with the skin area.
Statistical analysis
The results were presented as mean ± standard deviation (SD). Statistical analyses were performed using the Student’s t-test or one-way analysis of variance (ANOVA). The P < .05 was taken as statistically significant.
Results and discussion
Saturated solubility
The saturated solubility of paeonol is shown in . Practically, paeonol is very slightly soluble in water. Although its water solubility was slightly varied by the formation of eutectic mixture between paeonol and menthol, the difference is not statistically significant (P > .05). Among the eutectic mixtures, the pae:men 4:6 system showed the highest solubility of paeonol (593.3 ± 26.6 μg/ml), which was even higher than the solubility of pure paeonol itself (537.9 ± 42.3 μg/ml). Similarly, Yong et al. (Citation2003) observed that the solubility of ibuprofen in water increased as the ratio of menthol to ibuprofen increased from 0:10 to 4:6, and then decreased as the ratio increasing from 4:6 to 10:0. These results suggested that the improved solubility of paeonol might be contributed by the formation of eutectic mixture of paeonol with menthol in the aqueous solution.
Study of pseudo-ternary phase diagrams
Three pseudo-ternary phase diagrams using different eutectic mixtures as oil phase are given in . The dark area represents the region in which microemulsions can be formed. As shown in , the area of microemulsion region was getting larger as the ratio of paeonol to menthol decreased from 6:4 to 4:6, which was in accordance with the results of solubility test. The largest microemulsion area for the eutectic mixture of pae:men (4:6, w/w) might be mainly contributed to the highest paeonol solubility of the mixture in water (Wang and Pal Citation2014). From these diagrams, one point was taken and further applied to prepare the microemulsions. lists the formulation of the developed microemulsions. The obtained microemulsions were subsequently subjected to characterization tests to compare the formulations with different eutectic mixtures.
Shape and size characterization of the microemulsions
As listed in , the mean droplet size of microemulsions decreased as the ratio of paeonol decreasing in the eutectic mixture, suggesting significant surface tension difference among these eutectic mixtures. The size of microemulsion with the eutectic mixture of pae:men 6:4 and 5:5 dropped sharply at higher temperatures. Several previous reports also observed similar result (Alkrad et al. Citation2016, Xu et al. Citation2016). There are multiple possible causes for the size change as follows: Firstly, the interfacial tension decreases with temperature increase because of higher molecular thermal activity and as results the droplet size decreases. Secondly, the eutectic components might precipitate out as crystals at lower temperature and changed back to be tiny drops at higher temperature. Also probably, the increase in temperature leads to an increase on the solubility of Cremophor EL in the inner phase and followed by a reduction of the droplet size.
Table 2. Droplet size and polydispersity index (PI) of microemulsions at different temperature (n = 3).
The result of the morphological detection on the microemulsion and gel containing pae:men 4:6 was shown in . The images clearly showed the presence of the droplets with spherical shape. The droplets of the microemulsion were <100 nm, and those of microemulsion gel were <500 nm under transmission electron microscopy.
Rheological measurements
As is shown in , the viscosity of all microemulsions decreased with the increasing temperature. However, the range of viscosity change for three microemulsions was much different. A 1.30-fold decrease in viscosity occurred over a range of temperatures from 20 to 40 °C for microemulsion with pae:men 6:4, and that was 2.35- or 2.98-fold for those with pae:men 5:5 or 4:6, respectively. At a certain temperature, both the components content and the droplet size can affect the viscosity. Therefore, the viscosity difference among microemulsions may be mainly assigned to the massive gap among their particle size, which can be further confirmed from the data listed in .
Low viscosity is essential for microemulsions since it influence the stability of the emulsified nanodroplets, and the efficient drug release as well (Alvarado et al. Citation2015). Thus, we may expect better stability and drug penetration for the microemulsion system with pae:men 4:6 based on its lowest viscosity at different temperature.
Storage stability of the microemulsions and gels
The images of microemulsions during a short-term storage are shown in . The microemulsions with pae:men 5:5 and 4:6 seemed more transparent than that with pae:men 6:4, which was consistent with the results of particle size analysis. During 10 days of storage at ambient temperature, the system with pae:men 6:4 maintained a milk-like appearance. By contrast, the system with pae:men 4:6 exhibited as a clear transparent liquid. For the formulation with pae:men 5:5, its appearance changed from transparent to slightly turbid after stored for 7 days, and some very small needle-like crystals were observed under microscope.
Figure 5. The appearance of microemulsions during a short-term storage (A: initial; B: 3 days; C: 7 days; D: 10 days, and from left to right is pae:men 6:4, 5:5 and 4:6).
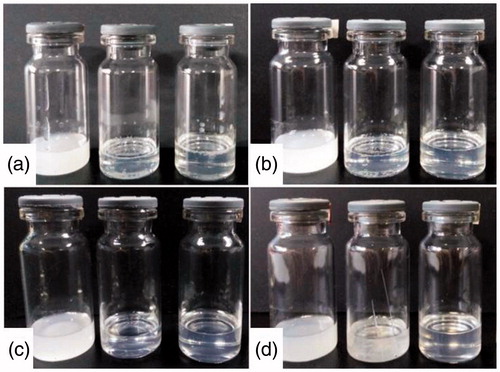
The appearance of microemulsion-based gels was shown in . Right after adoption of microemulsion into gels, some acicular crystals were observed under microscope for the systems with pae:men 6:4 and 5:5. The crystals for system 6:4 turned to be rod-like crystals at 3 days and further aggregated to be block mass at 10 days, and those for system 5:5 developed to be short bars at 10 days. But for the system 4:6, no needle/rod-like crystals were found under microscope.
Figure 6. The micrograph of microemulsion-based gels during a short-term storage (magnification 400×).
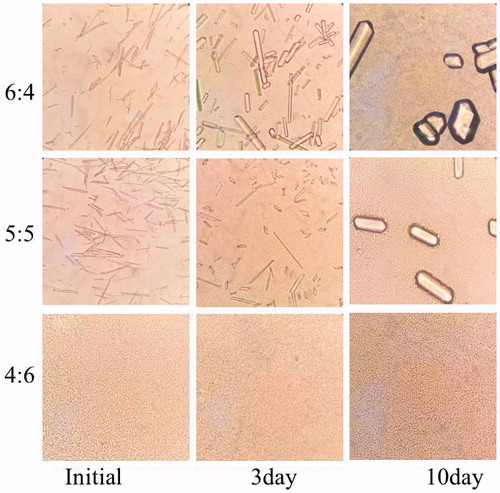
Based on these results, the formulation with pae:men 4:6 showed better physical stability than that with pae:men 6:4 or 5:5 in both microemulsion and gel systems.
In vitro skin permeation and drug deposition study
The microemulsion-based gels with different pae:men ratios were further compared on their performance of skin penetration and drug deposition. As shown in , drug permeation profiles of the test groups (microemulsion-based gel formulations) were significantly different from that of the control group (paeonol and menthol mixture gel), and that of pae:men 4:6 was better than those of pae:men 6:4 and 5:5 (one-way ANOVA, P < .05). These results suggested that drug permeation across the skin was facilitated by the microemulsion formulation, similar phenomenon was commonly observed in previous reports (Pillai et al. Citation2015). The formulation using the eutectic mixture of pae:men 4:6 as the oil phase showed the shortest lag time of 0.5 h and the highest steady-state flux of 0.3 μg/cm2 h ().
Figure 7. Drug permeation profiles of different formulation gels (n = 6). *P < .05, statistical significance compared with pae + men 4:6 (the paeonol gel). #P < .05, statistical significance compared with the pae:men 6:4 and 5:5.
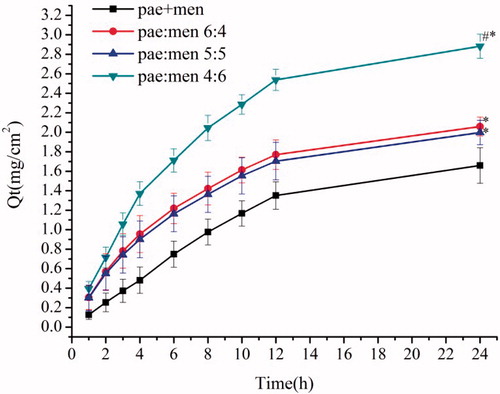
Table 3. In vitro percutaneous penetration parameters of different formulation gels (n = 6).
Also as is shown in , significantly higher amounts of paeonol deposition in mice skin for the formulation of pae:men 4:6 were observed than that for other formulations (Student’s t-test, P < .01). The enhanced skin permeation and drug deposition performance of the formulation 4:6 may be mainly due to smaller size of the obtained particles. In addition, the low viscosity of this formulation at 32 °C may increase its fluidity and hence its ability to penetrate skin deeply.
Conclusion
Both eutectic mixture and microemulsion have paved the way for enhancement of transdermal delivery of active pharmaceutical ingredients. In the present study, the eutectic mixture of drug and menthol was directly adopted as the oil phase into the microemulsion formulation. The formulation was optimized by comparing the droplet size, short-term stability, in vitro skin permeation and drug deposition of the obtained preparations. At a certain ratio of drug and menthol (4:6), skin penetration and deposition of paeonol was significantly enhanced by formation of nanoscale droplets, which suggesting that the microemulsion formulation based on drug–menthol eutectic mixture can be applied as a potential transdermal delivery system for better therapeutic efficacy.
Disclosure statement
The authors report no conflict of interest. The authors alone are responsible for the content and writing of this article.
References
- Alkrad JA, Mrestani Y, Neubert RH. 2016. Development and characterization of microemulsions containing hyaluronic acid. Eur J Pharm Sci. 86:84–90.
- Alvarado HL, Abrego G, Souto EB, Garduño-Ramirez ML, Clares B, García ML, Calpena AC. 2015. Nanoemulsions for dermal controlled release of oleanolic and ursolic acids: in vitro, ex vivo and in vivo characterization. Colloids Surf B Biointerfaces. 130:40–47.
- Aroso IM, Silva JC, Mano F, Ferreira AS, Dionisio M, Sá-Nogueira I, et al. 2016. Dissolution enhancement of active pharmaceutical ingredients by therapeutic deep eutectic systems. Eur J Pharm Biopharm. 98:57–66.
- Boonme P, Krauel K, Graf A, Rades T, Junyaprasert VB. 2006. Characterization of microemulsion structures in the pseudoternary phase diagram of isopropyl palmitate/water/brij 97:1-butanol. AAPS PharmSciTech. 7:E1–E6.
- Cherukuvada S, Nangia A. 2014. Eutectics as improved pharmaceutical materials: design, properties and characterization. Chem Commun. 50:906–923.
- Chun MK, Hossain K, Choi SH, Ban SJ, Moon H, Choi HK. 2012. Development of cataplasmic transdermal drug delivery system containing eutectic mixture of lidocaine and prilocaine. J Pharma Investig. 42:139–146.
- Luo M, Shen Q, Chen J. 2011. Transdermal delivery of paeonol using cubic gel and microemulsion gel. Int J Nanomed. 6:1603–1610.
- Morrison HG, Sun CC, Neervannan S. 2009. Characterization of thermal behavior of deep eutectic solvents and their potential as drug solubilization vehicles. Int J Pharm. 378:136–139.
- Negi P, Singh B, Sharma G, Beg S, Raza K, Katare OP. 2016. Phospholipid microemulsion-based hydrogel for enhanced topical delivery of lidocaine and prilocaine: QbD-based development and evaluation. Drug Deliv. 23:951–967.
- Paradkar M, Thakkar V, Soni T, Gandhi T, Gohel M. 2015. Formulation and evaluation of clotrimazole transdermal spray. Drug Dev Ind Pharm. 41:1718–1725.
- Park CW, Kim JY, Rhee YS, Oh TO, Ha JM, Choi NY, et al. 2012. Preparation and valuation of a topical solution containing eutectic mixture of itraconazole and phenol. Arch Pharm Res. 35:1935–1943.
- Pillai AB, Nair JV, Gupta NK, Gupta S. 2015. Microemulsion-loaded hydrogel formulation of butenafine hydrochloride for improved topical delivery. Arch Dermatol Res. 307:625–633.
- Shen Q, Li X, Li W, Zhao X. 2011. Enhanced intestinal absorption of daidzein by borneol/menthol eutectic mixture and microemulsion. AAPS PharmSciTech. 12:1044–1049.
- Stott PW, Williams AC, Barry BW. 1998. Transdermal delivery from eutectic systems: enhanced permeation of a model drug, ibuprofen. J Control Release. 50:297–308.
- Tarate B, Bansal AK. 2015. Characterization of CoQ 10-lauric acid eutectic system. Thermochim Acta. 605:100–106.
- Wang Z, Pal R. 2014. Enlargement of nanoemulsion region in pseudo-ternary mixing diagrams for a drug delivery system. J Surfact Deterg. 17:49–58.
- Xu Z, Jin J, Zheng M, Xu X, Liu Y, Wang X. 2016. Co-surfactant free microemulsions: preparation, characterization and stability evaluation for food application. Food Chem. 204:194–200.
- Yong CS, Jung SH, Rhee JD, Choi HG, Lee BJ, Kim DC, Choi YW, Kim CK. 2003. Improved solubility and in vitro dissolution of Ibuprofen from poloxamer gel using eutectic mixture with menthol. Drug Deliv. 10:179–183.