Abstract
Mosquito control is facing key challenges, including outbreaks of new arbovirus threats. We proposed an eco-friendly synthesis of silver nanoparticles (AgNPs) employing a low-cost extract of Hugonia mystax. AgNPs were specified by UV, XRD, FTIR and EDX spectroscopy, SEM and TEM. AgNPs were more toxic to Anopheles stephensi, Aedes aegypti, and Culex quinquefasciatus larvae (LC50: 14.45, 15.86, and 17.46 μg/mL) if compared to aquatic biocontrol organisms Gambusia affinis, Diplonychus indicus, and Anisops bouvieri (LC50: 2567.15, 1075.16, and 829.63 μg/ml). Overall, we shed light on the mosquito larvicidal efficacy of H. mystax, a possible biological resource for low-cost fabrication of AgNPs.
Introduction
Mosquitoes (Diptera: Culicidae) are important vectors of a wide number of parasites and pathogens, which often represents severe public health problems in tropical and subtropical areas worldwide (Benelli Citation2015a, Citation2015b, Benelli et al. Citation2016a, Citation2016b, Citation2016c, Mehlhorn et al. Citation2012). Mosquito control programs are facing a number of crucial challenges, including the blooming to mosquito resistance to synthetic pesticides and the current outbreaks of new arbovirus threats, such as chikungunya and Zika virus (Benelli and Mehlhorn Citation2016, Pavela and Benelli Citation2016). Indeed, current control strategies mainly rely on synthetic pesticides, insect growth regulators, and microbial control agents. However, synthetic chemicals lead to a number of negative implications, including high operational costs, development of resistance and toxic effects on non-target organisms and human health (Benelli Citation2015a, Naqqash et al. Citation2016). Limited tools are currently available against the main pathogens and parasites vectored by mosquitoes (Benelli Citation2015a, Benelli and Mehlhorn Citation2016).
To deal with the above-mentioned issues, in latest years an increasing number of flora-borne secondary metabolites have been proposed for cost-effective and rapid synthesis of nanoparticles (Benelli Citation2016a, Citation2016b, Chao et al. Citation2016, Hassan et al. Citation2016, Priyanka et al. Citation2016a, Citation2016b, Citation2016c, Rajan et al. Citation2015, Ramanibai and Velayutham Citation2015, Thirunavokkarasu et al. Citation2013, Velayutham et al. Citation2013). Recently, in more than one hundred researches, plant-fabricated nanoparticles have been studied for their highly effective mosquitocidal properties (Benelli Citation2016a, Citation2016b, Benelli et al. Citation2015a, Citation2015b, Govindarajan Citation2016). For instance, biosynthesized silver nanoparticles (AgNPs) from Carissa spinarum (Govindarajan et al. Citation2016b), Barleria cristata (Govindarajan and Benelli Citation2016a), Bauhinia variegata (Govindarajan et al. Citation2016e), and Clerodendrum chinense (Govindarajan et al. Citation2016d) were most virulent against the larval mosquitoes. Notably, it has been highlighted that differences in the green route employed for fabrication of mosquitocidal nanoparticles lead to different biophysical properties (e.g. size, crystalline structure, and shape) of the synthesized products (see Benelli Citation2015c, Citation2016a, Citation2016b, Citation2016c for reviews).
The genus Hugonia (Linn.) belongs to the family Linaceae. It comprises about 40 species in the world. Two of them, Hugonia mystax (Linn.) and Hugonia ferruginea (Wight & Arn.) grow in India (Pullaiah and Chennaiah Citation1997, Santapau and Henry Citation1983). H. mystax locally known as Modirakanni is used for skin diseases by the traditional healers of Tiruvannamalai hills, Tamil Nadu, India. Indeed, the leaves are used for anthelmintic (Padel et al. Citation2010) and to treat rheumatism (Sutha et al. Citation2009). Biological activity such as antidiabetic activity of the leaves in rabbits has been also reported (Nammi et al. Citation2003). To the best of our knowledge, the mosquito larvicidal potential of H. mystax is unrevealed.
In this investigation, we proposed a low-cost and swift route to synthesize AgNPs using the aqueous leaf extract of H. mystax. Green fabricated AgNPs were specified by UV, XRD, FTIR, SEM, TEM, and EDX. The severe toxicity of H. mystax leaf aqueous extract and biofabricated AgNPs were assessed against the Culex quinquefasciatus, Aedes aegypti, and Anopheles stephensi larvae.
Materials and methods
Materials
Fresh leaves of H. mystax were collected from the Marakanam reserve forest, located in Tamil Nadu, India. The taxonomist of Annamalai University in India provided identifications of the H. mystax leaves. Silver nitrate was acquired from Sigma-Aldrich, Mumbai, India.
Preparation of plant leaf extract
First, the H. mystax leaves were dried in the shade and then an electric grinder was used to turn them into finely ground powder. Subsequently, 50 g of the sample were mixed with 0.5 L of boiled and cooled distilled water, continually stirring the mixture on a magnetic stirrer, in order to acquire an aqueous extract. The dried leaf powder suspension was left to settle for 3 h and then was filtered by using Whatman n. 1 filter paper, finally, the filtrate was stored at 10 °C, until the time of the experiment.
Green fabrication of AgNPs
The leaves were thoroughly washed and finely ground. Then we took 10 g of the resulting powder and mixed it with 100 mL of sterilized, double-distilled water, in a 300-ml Erlenmeyer flask. The mixture was left to boil for 5 min before transfusion. Filtered the extract through Whatman filter paper n. 1 and then stored it at −15 °C. Treated the filtrate with aqueous 1 mM AgNO3 solution, which was obtained by dissolving 21.2 mg of AgNO3 powder in 125 mL water purified with Milli-Q technology (Merck KGaA, Darmstadt, Germany). The treatment took place in an Erlenmeyer flask, and the mixture was left at room temperature. 12 mL of the leaf extract were used to reduce 88 mL of a 1 mM silver nitrate aqueous solution. The reaction lasted 10 min at room temperature and resulted in the formation of Ag nanoparticles, as evident by the brown–yellow coloring of the solution.
Characterization of synthesized ag nanoparticles
The UV (UV-160v, Shimadzu, Japan) were used to monitor the bioreduction of the Ag+ ions. Subsequently, we employed energy dispersive X-ray spectrum (EDX), transmission electron microscopy (TEM Technite 10 Philips, Koninklijke Philips Electronics N.V.) and SEM (Hitachi S3000 H SEM; Thermo Fisher Scientific, Waltham, MA) to analyze the morphology, size, and composition of the Ag nanoparticles. Moreover, examined the purified Ag nanoparticles for biomolecule pressure by employing FT-IR (Nicolet 380, Thermo Scientific) and finally, XRD analysis was used to determine Ag nanoparticles and KBr pellets.
Target organisms
Laboratory reared mosquitoes were collected at Annamalai University’s Department of Zoology. The mosquitoes were 3–4 days old after emergence at the time of adult feeding, sustained on water and raisins; a 12-h starvation period preceded feeding. Fed 500 mosquitoes per cage each time, using blood through a Parafilm membrane-fitted unit for 4 h. Ae. Aegypti mosquitoes were fed from 12 to 16.00, while An. stephensi and Cx. quinquefasciatus feeding took place between 18.00 and 22.00. The conditions under which the mosquitoes were kept were 70–85% relative humidity (RH), 28 ± 2 °C temperature and a 12-h photoperiod (Govindarajan et al. Citation2016b).
Mosquito larvicidal potential
Larvicidal activity of the aqueous leaf extract and AgNPs was evaluated according to the protocol by WHO (Citation2005). Based on the wide range and narrow range tests, aqueous crude extract was tested at 80, 160, 240, 320, and 400 μg mL−1 concentrations and AgNPs was tested at 7, 14, 21, 28, and 35 μg mL−1 concentrations. About 20 numbers of late third larvae were introduced into a 500-ml beaker containing 249 mL of tap water, and 1 mL of desired concentrations of AgNPs or leaf extract was added. Five replicates were performed.
Bio-safety on non-target organisms
Followed the method described by Sivagnaname and Kalyanasundaram (Citation2004) to assess the mixture’s effect on non-target organisms. The biocontrol organisms, against which the impact of the studied plant’s extract was examined, were Gambusia affinis, Diplonychus indicus, and Anisops bouvieri. The biocontrol organisms were gathered in the wild and kept individually in tanks made from cement, with a depth of 30 cm and a diameter of 85 cm. The tanks contained water with a temperature of 27 ± 3 °C, while the external RH was 85%. The evaluation of the aqueous leaf extract and Ag nanoparticles mixture took place with concentrations that reached levels even 50 times higher than the calculated LC50 dose for mosquito larvae and monitored the mortality after we exposed them to the mixture for 48 h. Moreover, the organisms were exposed for an additional ten days, in order to assess any possible post-treatment effects on swimming activity and survival (Govindarajan and Benelli Citation2016b).
Data analysis
We employed probit analysis to evaluate mortality data. The Finney (Citation1971) method was used to calculate LC50 and LC90 concentrations. Furthermore, we adopted the formula be Deo et al. (Citation1988) to calculate the Suitability Index (SI) for every single biocontrol organisms, as follows:
For data analysis, used the SPSS Statistical Software Package, version 16.0 (IBM, Armonk, NY). We adopted a P < .05 as a level of probability, to assess the statistical significance of the differences between values.
Results and discussion
Fabrication of AgNPs
After incubating it for 180 min in a black chamber, the initially colorless mixture took a dark brown color, providing evidence of Ag nanoparticle formation (). The brown color exhibited by the Ag nanoparticles created by H. mystax may be attributed to the stimulation of surface Plasmon (SPR) vibrations (Veerakumar et al. Citation2014, Vijayakumar et al. Citation2013, Vivek et al. Citation2012, Zhang et al. Citation2011). SPR bands are affected by the Ag nanoparticles’ shape, size, composition, morphology, and dielectric environment (Benelli Citation2016a, Citation2016b, Govindarajan Citation2016, Muthukumaran et al. Citation2015a, Shahverdi et al. Citation2007, Singh et al. Citation2010). Further examination revealed that spherical Ag nanoparticles are responsible for absorption around the 400–480 nm range in the UV-visual spectrum (Sanpui et al., Citation2008, Shameli et al. Citation2012). These bands correspond to the absorption of Ag nanoparticles, while broad SPR was exhibited at 444 nm, in the UV-vis absorption spectrum ().
Spectroscopic and microscopic characterization of Ag nanoparticles
The green fabricated Ag nanoparticles’ crystalline nature was confirmed via XRD intensities of diffraction peaks at (111), (200), (220), and (311), which coincided with 38.74°, 44.27°, 63.88°, and 77.65°. The resulting XRD pattern underlined that Ag nanoparticles exhibited both hexagonal and cubic structures (). Similar findings were stated previously (Veerakumar et al. Citation2013) for Ag nanoparticles biosynthesized using Sida acuta, which exhibited both hexagonal and cubic structures.
Figure 2. XRD pattern of silver nanoparticles biofabricated using the Hugonia mystax aqueous extract.
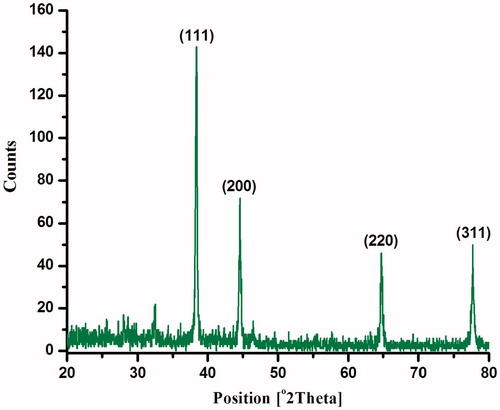
shows the FTIR spectrum of H. mystax-synthesized AgNPs. Over three repetitions, we noted distinguished absorbance bands at around 3356, 2933, 2392, 1622, 1488, 1384, 1192, 1146, 1095, 931, 825, 719, 661, and 593 cm−1. The aforementioned peaks imply N–H bending (aromatic amines), C–H stretching (alkanes), C=C stretching (alkanes), C–H bonds (methyl and methylene), H-bonds (alcohols, phenol), O–H stretching, and C–O stretching (carboxylic group), respectively. According to the observed bands, we infer that there are flavonoids and terpenoids present in the mixture, as potential reducing compounds (Huang et al. Citation2007, Ruparelia et al. Citation2008, Wazed Ali et al. Citation2001), which may also be able to explain the Ag nanoparticles’ efficient capping and stabilization.
Figure 3. FTIR spectrum of silver nanoparticles biofabricated using the Hugonia mystax aqueous extract.
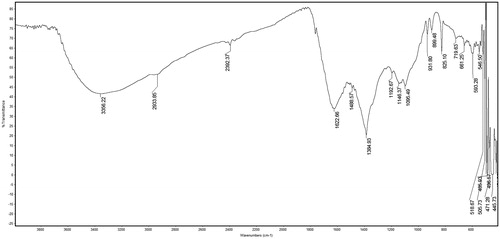
shows the SEM investigation of the Ag nanoparticles’ morphology and size distribution. The Ag nanoparticles show an almost spherical morphology, corresponding to the SPR band’s shape in the UV-vis spectrum. The mean size of the particles as studied from all the SEM images was between 40 and 90 nm. shows the EDX profile of Ag nanoparticles. The typical absorption peak of metallic Ag nanoparticles appears at around 3 keV due to SPR (Panneerselvam et al. Citation2016). TEM images revealed finely configured spherical Ag nanoparticles of crystalline nature, with sizes varying from 10 to 75 nm (), and a mean size of 35 nm. Another noteworthy observation was the capping of Ag nanoparticles by a thin coating of biomolecules, with stabilizing properties, resulting in poly-dispersions of the nanoparticles, with no direct contact, and prolonged stability (Govindarajan and Benelli Citation2016b, Thirunavukkarasu et al. Citation2010).
Figure 4. Scanning electron microscopy (SEM) of Hugonia mystax silver nanoparticles (A. 25 000X; B. 50 000X).
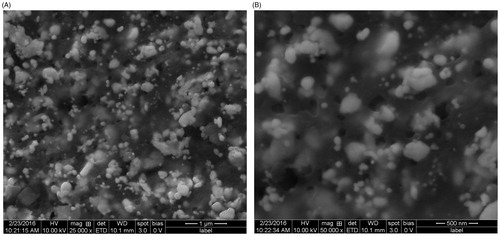
Mosquito larvicidal properties
Both the H. mystax aqueous leaf extract and the Ag nanoparticles have affected the survival of all the tested mosquito larvae ( and ). The H. mystax-synthesized AgNPs exhibited increased toxicity against Cx. quinquefasciatus (LC50 17.46 μg/mL), Ae. aegypti (LC50 15.86 μg/mL), and An. stephensi (LC50 14.45 μg/mL) (), compared to the aqueous leaf extract alone. Recently, significant efforts have been carried out to investigate the mosquito larvicidal activity of flora-derived substances (Pavela Citation2015a, Citation2015b). For instance, Govindarajan et al. (Citation2016c) assessed the acute toxicity of P. barbatus essential oil against Cx. tritaeniorhynchus, Ae. albopictus and An. subpictus larvae, and found LC50 values of 94.34, 87.25, and 84.20 μg/ml, respectively. Also, the larvicidal toxicity of the Cardiospermum halicacabum leaf extract (testing solvents with different polarity, i.e. chloroform, methanol, ethyl acetate, hexane, and benzene) was assessed against Ae. aegypti and Cx. quinquefasciatus. LC50 were 154.95, 150.44, 183.36, 193.31, and 174.24 ppm, and 164.54, 156.80, 192.31, 200.02, and 182.51 ppm, respectively (Govindarajan Citation2011). Mathivanan et al. (Citation2010) showed that the Ervatamia coronaria methanol leaves extract on Cx. quinquefasciatus, Ae. aegypti, and An. stephensi larvae resulted in LC50 values of 72.41, 65.67, and 62.08 mg/L, respectively. Finally, high larvicidal activity was discovered for the essential oil extracted from Clausena anisata, with 24 h LC50 values of 140.96, 130.19, and 119.59 ppm, respectively (Govindarajan Citation2010).
Table 1. Larvicidal potential of Hugonia mystax aqueous leaf extract against the mosquito vectors Anopheles stephensi, Aedes aegypti, and Culex quinquefasciatus.
Table 2. Larvicidal potential of AgNPs synthesized using the Hugonia mystax leaf extract against the Anopheles stephensi, Aedes aegypti, and Culex quinquefasciatus.
Bio-synthesized Ag nanoparticles appeared to have increased larvicidal toxicity at lower concentrations, compared to botanicals alone (Benelli Citation2016c). In recent years, there has been a heightened interest in the exploration of several plants as sources of reducing agents for the green fabrication of Ag nanoparticles with mosquitocidal properties (Benelli Citation2015c, Citation2016a, Citation2016b). Controls, including experiments trial Ag+ particles at the similar applications as Ag nanoparticles, revealed no increased mortality, in accordance with the findings by Marimuthu et al. (Citation2011) and Govindarajan and Benelli (Citation2016b).
Every plant is unique with respect to the nature and combination of compounds it contains that exhibit mosquitocidal and/or repellent properties (Benelli Citation2015b, Citation2016a, Citation2016b, Citation2016c, Haldar et al. Citation2013). Combining nanoparticles with bioactive substances, greater antivectorial efficacy has been achieved. In the current experiment, the addition of bio-stabilized AgNPs increased the mixture’s larvicidal activity by many folds. Our findings are in concordance with the research of Muthukumaran et al. (Citation2015b), who investigated the mosquito larvicidal action of AgNPs that were fabricated using Gmelina asiatica against Cx. quinquefasciatus (LC50 27.83 μg/mL), Ae. aegypti (LC50 25.77 μg/mL) and An. stephensi (LC50 22.44 μg/mL. Govindarajan et al. (Citation2016a) investigated the toicity of Malva sylvestris-fabricated AgNPs on Cx. quinquefasciatus, Ae. Aegypti, and An. stephensi larvae. Nanoparticles exhibited increased toxicity against Cx. quinquefasciatus (LC50 12.19 μg/mL), Ae. aegypti (LC50 11.23 μg/mL), and An. stephensi (LC50 10.33 μg/mL) compared to the leaf extract alone. The same research team examined the acute toxicity of Ag nanoparticles biosynthesized with the mediation of C. spinarum leaf extract against Cx. tritaeniorhynchus, Ae. albopictus and An. subpictus larvae, obtaining LC50 values of 10.04, 9.01, and 8.37 μg/mL, respectively (Govindarajan et al. Citation2016b); Furthermore, bio-fabricated AgNPs that were biosynthesized through B. cristata mediation exhibited larvicidal efficacy against third instar larvae of Cx. tritaeniorhynchus (LC50 15.01 μg/mL), Ae. albopictus (LC50 13.49 μg/mL), and An. subpictus (LC50 12.46 μg/mL); whereas aqueous leaf extract achieved the following LC50 values: 146.31, 135.32, and 124.27 μg/mL, respectively (Govindarajan and Benelli Citation2016a). B. variegata-derived aqueous leaf extract showed toxicity against Cx. tritaeniorhynchus (LC50 157.24 μg/mL), Ae. albopictus (LC50 145.08 μg/mL), and An. subpictus (LC50 132.78 μg/mL). The toxicity of Ag nanoparticles was substantiated against Cx. tritaeniorhynchus, Ae. albopictus and An. subpictus, with respective LC50 values of 51.92, 46.16, and 41.96 μg/mL (Govindarajan et al. Citation2016e). Moreover, in addition to their acutely toxic properties, it has been found that very low concentrations of Ag nanoparticles result in the mosquito larvae displaying reduced motility, thereby rendering them more vulnerable to odonate nymphs and other natural enemies of mosquitoes (Benelli Citation2015c, Citation2016a, Citation2016b).
Toxicity on bio-control aquatic organisms
Interestingly, non-target toxicity testing revealed negligible effects against G. affinis, D. indicus, and A. bouvieri adults, as shown by the achieved LC50 values that ranged from 829 to 32192 μg/ml ( and ). Furthermore, our scrutiny pointed that swimming activity and longevity of the studied species were not affected up to a week following testing. SI indicated that H. mystax-mediated AgNPs exhibited decreased toxicity against the tested non-target organisms, compared to the targeted mosquito larvae (). There is currently limited knowledge regarding mosquitocidal essential oil, plant extracts, and green-fabricated NPs toxicity against bio-control organisms (Benelli Citation2016a, Citation2016b). Recently, the Zanthoxylum monophyllum essential oil, along with its main chemical compounds, were found non-toxic to the predatory fish G. affinis. As the LC50 for G. affinis was calculated at 4234 μg/mL, it is inferred that the essential oil is safe for the fish species, resulting in a high SI value, ranging from 86.39 for the robust Cx. tritaeniorhynchus larvae to 102.02 for the more fragile An. subpictus larvae (Pavela and Govindarajan Citation2016). Govindarajan et al. (Citation2016a) investigated that the M. sylvestris – fabricated AgNPs also exhibited minimal biotoxicity against aquatic mosquito predators D. indicus (LC50 813 μg/mL) and G. affinis (LC50 10 459 μg/mL). Furthermore, Govindarajan et al. (Citation2016b) assessed the toxicity of C. spinarum aqueous extract and biosynthesized AgNPs on the biocontrol organisms A. bouvieri, D. indicus, and G. affinis. Toxicity testing revealed minimal toxicity, obtaining LC50 values in the range of 424–6402 μg/mL.
Table 3. Effect of Hugonia mystax aqueous leaf extract against three bio-control organisms sharing the same ecological niche of Anopheles and Aedes mosquito vectors.
Table 4. Effect of synthesized AgNPs using the Hugonia mystax leaf extract against three bio-control organisms sharing the same ecological niche of Anopheles and Aedes mosquito vectors.
Table 5. Suitability index of three bio-control organism over young instars of Anopheles stephensi, Aedes aegypti, and Culex quinquefasciatus exposed to Hugonia mystax aqueous leaf extract and AgNPs.
Conclusions
In this study, the H. mystax-mediated synthesis of AgNPs was reported as a one-step, low-cost and effective fabrication route of mosquitocidal. Indeed, we biosynthesized AgNPs at room temperature, using inexpensive and non-toxic H. mystax leaf extract as starting raw material. A variety of bio-physical methods, including XRD analysis, SEM, TEM, EDX spectroscopy, FTIR spectroscopy, and UV-vis spectrophotometry were used to characterize the bio-reduced Ag nanoparticles. We assessed the effect of H. mystax aqueous leaf extract and AgNPs against larvae of filariasis and St. Louis encephalitis vector Cx. quinquefasciatus, the dengue and Zika virus vector Ae. aegypti, and the malaria vector An. stephensi. Our results showed that the bio-fabricated Ag nanoparticles exhibited high larvicidal action versus the third instar of these tested mosquitoes, achieving LC50 values that ranged from 14 to 17 μg/mL. A further noteworthy point is that the low toxicity testing against natural mosquito predators stressed the eco-friendly profile of these bio-fabricated nanoparticles. Therefore, we proposed the H. mystax-mediated biosynthetic pathway as a future good sustainable route for nanomosquitocide fabrication.
Acknowledgements
The authors extend their sincere appreciations to the Deanship of Scientific Research at King Saud University for its funding this Prolific Research Group (PRG-1437-36).
Disclosure statement
No potential conflict of interest was reported by the authors.
References
- Benelli G, Bedini S, Cosci F, Toniolo C, Conti B, Nicoletti M. 2015a. Larvicidal and ovi-deterrent properties of neem oil and fractions against the filariasis vector Aedes albopictus (Diptera: Culicidae): a bioactivity survey across production sites. Parasitol Res. 114:227–236.
- Benelli G, Bedini S, Flamini G, Cosci F, Cioni PL, Amira S, et al. 2015b. Mediterranean essential oils as effective weapons against the West Nile vector Culex pipiens and the Echinostoma intermediate host Physella acuta: what happens around? An acute toxicity survey on non-target may flies. Parasitol Res. 114:1011–1021.
- Benelli G, Canale A, Higuchi A, Murugan K, Pavela R, Nicoletti M. 2016a. The outbreaks of Zika virus: mosquito control faces a further challenge. Asia Pac J Trop Dis. 6:253–258.
- Benelli G, Lo Iacono A, Canale A, Mehlhorn H. 2016b. Mosquito vectors and the spread of cancer: an overlooked connection? Parasitol Res. 115:2131–2137.
- Benelli G, Mehlhorn H. 2016. Declining malaria, rising of dengue and Zika virus: insights for mosquito vector control. Parasitol Res. 115:1747–1754.
- Benelli G, Pavela R, Canale A, Mehlhorn H. 2016c. Tick repellents and acaricides of botanical origin: a green road map to control tick-borne diseases? Parasitol Res. 115:2545–2560.
- Benelli G. 2015a. Research in mosquito control: current challenges for a brighter future. Parasitol Res. 114:2801–2805.
- Benelli G. 2015b. Plant-borne ovicides in the fight against mosquito vectors of medical and veterinary importance: a systematic review. Parasitol Res. 114:3201–3212.
- Benelli G. 2015c. Plant-synthesized nanoparticles in the fight against mosquito vectors: an eco-friendly tool against mosquito vectors. In: Mehlhorn H, Ed. Nanoparticles in the Fight Against Parasites – Parasitology Research Monographs. Geneva, Switzerland: Springer International Publishing. doi:10.1007/978-3-319-25292-6. P.115-172.
- Benelli G. 2016a. Plant-mediated biosynthesis of nanoparticles as an emerging tool against mosquitoes of medical and veterinary importance: a review. Parasitol Res. 115:23–34.
- Benelli G. 2016b. Spread of Zika virus: the key role of mosquito vector control. Asia Pac J Trop Biomed. 6:468–471.
- Benelli G. 2016c. Green synthesized nanoparticles in the fight against mosquito-borne diseases and cancer ? a brief review. Enzyme Microbial Technol. [Epub ahead of print]. doi: 10.1016/j.enzmictec.2016.08.022.
- Chao W, Yeon JK, Priyanka S, Ramya M, Yan J, Deok CY. 2016. Green synthesis of silver nanoparticles by Bacillus methylotrophicus, and their antimicrobial activity. Artif Cells Nanomed Biotechnol. 44:1127–1132.
- Deo PG, Hasan SB, Majumdar SK. 1988. Toxicity and suitability of some insecticides for household use. Int Pest Control. 30:118–129.
- Finney DJ. 1971. Probit Analysis, 3rd ed. UK: Cambridge University Press.
- Govindarajan M, Benelli G. 2016a. Facile biosynthesis of silver nanoparticles using Barleria cristata: mosquitocidal potential and biotoxicity on three non-target aquatic organisms. Parasitol Res. 115:925–935.
- Govindarajan M, Benelli G. 2016b. One-pot green synthesis of silver nanocrystals using Hymenodictyon orixense: A cheap and effective tool against malaria, chikungunya and Japanese encephalitis mosquito vectors? RSC Adv. 6:59021–59029.
- Govindarajan M, Hoti SL, Rajeswary M, Benelli G. 2016a. One-step synthesis of polydispersed silver nanocrystals using Malva sylvestris: an eco-friendly mosquito larvicide with negligible impact on non-target aquatic organisms. Parasitol Res. 115:2685–2695.
- Govindarajan M, Nicoletti M, Benelli G. 2016b. Bio-physical characterization of poly-dispersed silver nanocrystals fabricated using Carissa spinarum: A potent tool against mosquito vectors. J Cluster Sci. 27:745–761.
- Govindarajan M, Rajeswary M, Hoti SL, Bhattacharyya A, Benelli G. 2016c. Eugenol, α-pinene and β-caryophyllene from Plectranthus barbatus essential oil as eco-friendly larvicides against malaria, dengue and Japanese encephalitis mosquito vectors. Parasitol Res. 115:807–815.
- Govindarajan M, Rajeswary M, Hoti SL, Murugan K, Kovendan K, Arivoli S, Benelli G. 2016d. Clerodendrum chinense–mediated biofabrication of silver nanoparticles: mosquitocidal potential and acute toxicity against non-target aquatic organisms. J Asia Pac Entomol. 19:51–58.
- Govindarajan M, Rajeswary M, Veerakumar K, Hoti SL, Mehlhorn H, Barnard DR, Benelli G. 2016e. Novel synthesis of silver nanoparticles using Bauhinia variegata: a recent eco-friendly approach for mosquito control. Parasitol Res. 115:723–733.
- Govindarajan M. 2016. Green synthesized silver nanoparticles: a potential new insecticide for mosquito control. In: H. Mehlhorn, Ed. Nanoparticles in the Fight Against Parasites – Parasitology Research Monographs, Switzerland: Springer International Publishing, pp. 99–153. doi: 10.1007/978-3-319-25292-6_7.
- Govindarajan M. 2010. Chemical composition and larvicidal activity of leaf essential oil from Clausena anisata (Willd.) Hook. f. ex Benth (Rutaceae) against three mosquito species. Asian Pac J Trop Med. 3:874–877.
- Govindarajan M. 2011. Mosquito larvicidal and ovicidal activity of Cardiospermum halicacabum Linn. (Family: Sapindaceae) leaf extract against Culex quinquefasciatus (Say.) and Aedes aegypti (Linn.) (Diptera: Culicidae). Eur Rev Med Pharmacol Sci. 15:787–794.
- Haldar KM, Haldar B, Chandra G. 2013. Fabrication, characterization and mosquito larvicidal bioassay of silver nanoparticles synthesized from aqueous fruit extract of putranjiva, Drypetes roxburghii (Wall.). Parasitol Res. 112:1451–1459.
- Hassan K, Gholamreza A, Mohammad Reza C, Rahim Bahri N, Akbar B, Siavash I. 2016. Green biosynthesis of silver nanoparticles using Althaea officinalis radix hydroalcoholic extract. Artif Cells Nanomed Biotechnol. 44:209–215.
- Huang J, Li Q, Sun D. 2007. Biosynthesis of silver and gold nanoparticles by novel sundried Cinnamomum camphora leaf. Nanotechnology. 18:105–104.
- Marimuthu S, Rahuman AA, Rajakumar G, Santhoshkumar T, Kirthi AV, Jayaseelan C, et al. 2011. Evaluation of green synthesized silver nanoparticles against parasites. Parasitol Res. 108:1541–1549.
- Mathivanan T, Govindarajana M, Elumalai K, Krishnappa K, Ananthan A. 2010. Mosquito larvicidal and phytochemical properties of Ervatamia coronaria Stapf. (Family: Apocynaceae). J Vector Borne Dis. 47:178–180.
- Mehlhorn H, Al-Rasheid KA, Al-Quraishy S, Abdel-Ghaffar F. 2012. Research and increase of expertise in arachno-entomology are urgently needed. Parasitol Res. 110:259–265.
- Muthukumaran U, Govindarajan M, Rajeswary M. 2015a. Mosquito larvicidal potential of silver nanoparticles synthesized using Chomelia asiatica (Rubiaceae) against Anopheles stephensi, Aedes aegypti, and Culex quinquefasciatus (Diptera: Culicidae). Parasitol Res. 114:989–999.
- Muthukumaran U, Govindarajan M, Rajeswary M. 2015b. Synthesis and characterization of silver nanoparticles using Gmelina asiatica leaf extract against filariasis, dengue, and malaria vector mosquitoes. Parasitol Res. 114:1817–1827.
- Nammi S, Ganti SS, Lodagala DS, Babu BSR. 2003. Hypoglycemic and insulin release effects of Hugonia mystax leaves in rabbits. Asia Pac J Pharmacol. 16:1–3.
- Naqqash MN, Gökçe A, Bakhsh A, Salim M. 2016. Insecticide resistance and its molecular basis in urban insect pests. Parasitol Res. 115:1363–1373.
- Padel SB, Murty PP, Rao DS, Venkaiah M. 2010. Ethnomedicinal plants from Paderu Division of Visakapatnam District, A.P. India. J Phytol. 2:70–91.
- Panneerselvam C, Murugan K, Roni M, Thabiani AA, Suresh U, Rajaganesh R, et al. 2016. Fern-synthesized nanoparticles in the fight against malaria: LC/MS analysis of Pteridium aquilinum leaf extract and biosynthesis of silver nanoparticles with high mosquitocidal and antiplasmodial activity. Parasitol Res. 115:997–1013.
- Pavela R, Benelli G. 2016. Ethnobotanical knowledge on botanical repellents employed in the African region against mosquito vectors – a review. Exp Parasitol. 167C:103–108.
- Pavela R, Govindarajan M. 2016. The essential oil from Zanthoxylum monophyllum a potential mosquito larvicide with low toxicity to the non-target fish Gambusia affinis. J Pest Sci. [Epub ahead of print]. doi: 10.1007/s10340-016-0763-6.
- Pavela R. 2015a. Essential oils for the development of eco-friendly mosquito larvicides: A review. Ind Crops Prod. 76:174–187.
- Pavela R. 2015b. Larvicidal property of essential oils against Culex quinquefasciatus Say (Diptera: Culicidae). Ind Crops Prod. 30:311–315.
- Priyanka S, Yeon JK, Chao W, Ramya M, Deok CY. 2016a. Weissella oryzae DC6-facilitated green synthesis of silver nanoparticles and their antimicrobial potential. Artif Cells Nanomed Biotechnol. 44:1569–1575.
- Priyanka S, Yeon JK, Chao W, Ramya M, Mohamed El-Agamy F, Deok CY. 2016b. Biogenic silver and gold nanoparticles synthesized using red ginseng root extract, and their applications. Artif Cells Nanomed Biotechnol. 44:811–816.
- Priyanka S, Yeon JK, Chao W, Ramya M, Deok CY. 2016c. The development of a green approach for the biosynthesis of silver and gold nanoparticles by using Panax ginseng root extract, and their biological applications. Artif Cells Nanomed Biotechnol. 44:1150–1157.
- Pullaiah T, Chennaiah E. 1997. Flora of Andhra Pradesh. Vol. I, Jodhpur, India: Scientific Publishers.
- Rajan R, Chandran K, Harper SL, Yun SI, Kalaichelvan PT. 2015. Plant extract synthesized nanoparticles: an ongoing source of novel biocompatible materials. Ind Crops Prod. 70:356–373.
- Ramanibai R, Velayutham K. 2015. Bioactive compound synthesis of Ag nanoparticles from leaves of Melia azedarach and its control for mosquito larvae. Res Vet Sci. 98:82–88.
- Ruparelia JP, Chatterjee AK, Duttagupta SP, Mukherji S. 2008. Strain specificity in antimicrobial activity of silver and copper nanoparticles. Acta Biomater. 4:707–716.
- Sanpui P, Murugadoss A, Prasad PVD, Ghosh SS, Chattopadhyay A. 2008. The antibacterial properties of a novel chitosan-Ag-nanoparticle composite. Int J Food Microbiol. 124:142–146.
- Santapau H, Henry AN. 1983. A Dictionary of Flowering Plants in India. New Delhi: Council of Scientific and Industrial Research, pp. 103.
- Shahverdi AR, Minaeian S, Shahverdi HR, Jamalifar H, Nohi AA. 2007. Rapid synthesis of silver nanoparticles using culture supernatants of Enterobacteria: a novel biological approach. Proc Biochem. 42:919–923.
- Shameli K, Ahmad MB, Jazayeri SD, Shabanzadeh P, Sangpour P, Jahangirian H, Gharayebi Y. 2012. Investigation of antibacterial properties silver nanoparticles prepared via green method. Chem Cent J. 6:73–78.
- Singh A, Jain D, Upadhyay MK, Khandelwal N. 2010. Green synthesis of silver nanoparticles using Argemone mexicana leaf extract and evaluation of their antimicrobial activities. Dig J Nanomater. 5:483–489.
- Sivagnaname N, Kalyanasundaram M. 2004. Laboratory evaluation of methanolic extract of Atlantia monophylla (Family: Rutaceae) against immature stages of mosquitoes and non-target organisms. Mem Inst Oswaldo Cruz. 99:115–118.
- Sutha S, Mohan VR, Kumaresan S, Murugan C, Athiperumalsami T. 2009. Ethnomedicinal plants used by the tribals of Kalakad Mundanthurai Tiger Reserve (KMTR), Western Ghats, Tamil Nadu for the treatment of rheumatism. Indian J Trad Knowledge. 9:502–509.
- Thirunavokkarasu M, Balaji U, Behera S, Panda PK, Mishra BK. 2013. Biosynthesis of silver nanoparticle from leaf extract of Desmodium gangeticum (L.) DC. and its biomedical potential. Spectrochim Acta A Mol Biomol Spectrosc. 116:424–427.
- Thirunavukkarasu S, Rahuman AA, Govindasamy R, Marimuthu S, Asokan B, Chidambaram J, et al. 2010. Synthesis of silver nanoparticles using Nelumbo nucifera leaf extract and its larvicidal activity against malaria and filariasis vectors. Parasitol Res. 108:693–702.
- Veerakumar K, Govindarajan M, Rajeswary M. 2013. Green synthesis of silver nanoparticles using Sida acuta (Malvaceae) leaf extract against Culex quinquefasciatus, Anopheles stephensi, and Aedes aegypti (Diptera: Culicidae). Parasitol Res. 112:4073–4085.
- Veerakumar K, Govindarajan M, Rajeswary M. 2014. Low-cost and ecofriendly green synthesis of silver nanoparticles using Feronia elephantum (Rutaceae) against Culex quinquefasciatus, Anopheles stephensi, and Aedes aegypti (Diptera: Culicidae). Parasitol Res. 113:1775–1785.
- Velayutham K, Rahuman AA, Rajakumar G, Roopan SM, Elango G, Kamaraj C, et al. 2013. Larvicidal activity of green synthesized silver nanoparticles using bark aqueous extract of Ficus racemosa against Culex quinquefasciatus and Culex gelidus. Asian Pac J Trop Med. 6:95–101.
- Vijayakumar M, Priya K, Nancy FT, Noorlidah A, Ahmed ABA. 2013. Biosynthesis, characterisation and anti-bacterial effect of plant-mediated silver nanoparticles using Artemisia nilagirica. Ind Crops Prod. 41:235–240.
- Vivek R, Thangam R, Muthuchelian K, Gunasekaran P, Kaveri K, Kannan S. 2012. Green biosynthesis of silver nanoparticles from Annona squamosa leaf extract and its in vitro cytotoxic effect on MCF-7 cells. Process Biochem. 47:2405–2410.
- Wazed Ali S, Rajendran S, Joshi M. 2001. Synthesis and characterization of chitosan and silver loaded chitosan nanoparticles for bioactive polyester. Carbohydr Polym. 83:438–446.
- World Health Organization. 2005. Guidelines for laboratory and field testing of mosquito larvicides, WHO/CDS/WHOPES/GCDPP/2005.13.
- Zhang W, Chen Z, Liu H, Zhang L, Gao P, Li D. 2011. Biosynthesis and structural characteristics of selenium nanoparticles by Pseudomonas alcaliphila. Colloids Surf B Biointerfaces. 88:196–201.