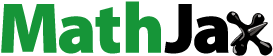
Abstract
In this study, negatively charged acrylic acid was used as a functional ligand to synthesise net-poly(2-hydroxyethyl methacrylate-co-acrylic acid) microparticles for the removal of hemoglobin having a positively charged Fe2+ ion in the core, as an alternative to conventional techniques to achieve a cost effective high-capacity purification. The characterization of microparticles was performed via Fourier transform infrared spectroscopy, scanning electron microscopy, transmission electron microscopy, atomic force microscopy, the Brunauer – Emmett – Teller surface area analysis, and swelling test methods. Optimum adsorption conditions such as pH, initial hemoglobin concentration, temperature, and interaction time were studied batch wise. The highest adsorption capacity of microparticles was observed at pH 7.4 as 1276.4 mg hemoglobin/g polymer. The reusability of microparticles was also promising with ∼5% decrease in the adsorption capacity at the end of the five adsorption-desorption cycles.
Introduction
Proteomics is evolving very quickly and gaining a considerable attention in recent years (Gao et al. Citation2016). Understanding the mechanism of useful protein expression, including translation and protein–protein interaction in the process of disease and the cell metabolism is gaining more and more importance, so it is necessary to deal with large-scale protein characteristics (Anagnostopoulos et al. Citation2011, Goh et al. Citation2012). Trace amounts of the biomarkers in the blood such as alanine aminotransferase (Vozarova et al. Citation2002), HBsAg (Samuel et al. Citation1991), and Anti-HCV (Shepard et al. Citation2005) for liver and microRNAs for cancer (Kosaka et al. Citation2010) work as disease indicator (Ding et al. Citation2015). Hereditary diseases such as hemoglobinopathy, sickle cell disease, and thalassemia, tearing of the red blood cell membrane resulting in intravascular hemolysis cause the high concentration of hemoglobin in plasma (Bain et al. Citation1998, Lezcano et al. Citation2006, Sowemimo-Coker Citation2002). Therefore, hemoglobin in excess makes the identification of potential lower-level protein biomarkers difficult (Tetala et al. Citation2013). These proteins may become the new molecular targets for drug design or may allow to early diagnosis of the diseases (Gao et al. Citation2016). Therefore, the removal of the histidine-rich proteins like hemoglobin in the blood prior to such analysis is very necessary for biomedical applications (Ding et al. Citation2015).
Moreover, today, hemoglobin is a basic need of blood donations and blood substitute in the recombinant form (Haney et al. Citation2000). For this purpose, a highly purified called as stroma-free hemoglobin should be obtained (Haney et al. Citation2000, Hsia and Er Citation1986). In the literature, the high level of hemoglobin has been reduced via molecular recognition, ion exchange chromatography, immobilized metal affinity chromatography (IMAC). The various materials, like polymer beads or gels, membranes, and nanoparticles for the purification of stroma-free hemoglobin, have been prepared via centrifugation/precipitation (Sakai et al. Citation1993), ion exchange chromatography in the presence of PEG (polyethylene glycol) (Li et al. Citation2000), liquid–solid extraction based on Cu(II)IDA-PEG6000-Tween 80-(NH4)2SO4 (IDA, imminodiacetic acid) (Shen et al. Citation2002), cation-exchange chromatography of PEGylated hemoglobin (Lu et al. Citation2004a), aqueous two-phase systems (Kan and Lee Citation1994), as well as anion-exchange chromatography (Andrade et al. Citation2004, Haney et al. Citation2000, Lu et al. Citation2004b) in several studies (Altintas et al. Citation2011, Beeskow et al. Citation1997, Bhattacharya et al. Citation2007, Derazshamshir et al. Citation2010, Dimino and Palmer Citation2007, Ding et al. Citation2008, Levine et al. Citation1998, Ma et al. Citation2005a, Citationb, Citation2006, Min et al. Citation2011, Ringrose et al. Citation2008, Shi and Deng Citation2015, Williams et al. Citation2010, Zhang et al. Citation2010a, Citationb). However, small beads in the column and the use of membrane have some disadvantages such as high cost, difficult sample preparation, long experiment time, the necessity for a qualified person, high-pressure drop, low mass transfer in a packed column, a low surface area and the low membrane adsorption capacity (Tetala et al. Citation2013). Selective separation of biomolecules such as proteins from these complex samples is a considerable problem in biotechnology and biomedical fields. As a result, an effective method for improving the selective removal of proteins from complex samples needs to be improved (Anirudhan and Rejeena Citation2013). The selective separation of protein via adsorption is considered to be an effective technique (Anirudhan and Senan Citation2011, Shukoor et al. Citation2007).
In this study, we have developed a net-poly(HEMA-co-AA) [net-poly(2-hydroxyethyl methacrylate-co-acrylic acid] microparticles to be used for the adsorption, especially for in-vitro applications, based on affinity chromatography. The quality and also performance of adsorption of hemoglobin onto the microparticles are thought to be much higher those from the literature because of the advantages of the high surface area of target-specific micro (Köse Citation2016, Kose et al. Citation2015) or nanoparticles (Kose and Denizli Citation2013). The method used in this study is intended to be very effective for the removal of hemoglobin or hemoglobin-like histidine-rich large biomolecules by combining the selectivity, simplicity, and speed with separation technique of the present.
Materials and methods
Materials
Hemoglobin and toluene were purchased from Sigma-Aldrich (St. Louis, MO). 2-hydroxyethyl methacrylate (HEMA), N, N-methylene bisacrylamide (NNMBAA), polyvinyl alcohol (PVA, cold water soluble), acrylic acid (AA) was obtained from Aldrich (Munich, Germany). Azobisisobutyronitrile (AIBN) is provided from Fluka (St. Gallen, Switzerland). All other chemicals used are of analytical grade and were used as supplied.
Physico-chemical characterization
Synthesis of net-poly(2-hydroxyethyl methacrylate-co-acrylic acid) microparticles
The synthesis of net-poly(HEMA-co-AA) microparticles was performed according to the literature (Erol et al. Citation2015) without using any magnetic core through microemulsion polymerization techniques using structural monomer (HEMA, 2 mL) and crosslinker (NNMBA, 3.27 g), functional monomer (AA, 500 μL), toluene (6 mL), PVA (200 mg) and distilled water of 50 mL. At the last stage, the azobisisobutyronitrile (AIBN) was added as an initiator.
The characterization of net-poly (2-hydroxyethyl methacrylate-co-acrylic acid) microparticles
The scanning electron microscope (SEM) FEI/Quanta 450 FEG, OR) was used for the surface analysis of net-poly(HEMA-co-AA) microparticles. Moreover, the particular structure of microparticles was analyzed using the transmission electron microscope (TEM; FEI Tecnai G2 Spirit BioTwin CTEM Microscope, OR). According to the TEM images, the differences between microparticles and hemoglobin-adsorbed microparticles and also Fe2+ core with a high contrast can be seen clearly. In order to be sure about the surface characteristic of microparticles, the atomic force microscopy, (Pieper et al. Citation2000) (MMafm-2/1700 EXL; Digital Instruments, Santa Barbara, CA) was used. The Fourier transform infrared (FT-IR) (Thermo Scientific Nicolet 6700 FT-IR spectrometer, Waltham, MA) device was used for FT-IR spectroscopy for the functional group determination. The surface area of microparticles was measured using Brunauer–Emmett–Teller (BET) (Quantachrome AutosorbVR iQ-Chemi, FL) method.
The adsorption-desorption experiments
All experiments were performed batch-wise using the hemoglobin solution of 1 mL, 1 mg/mL in concentration, with phosphate buffer (pH 7.4, 0.1 M, 4 mL, sodium phosphate buffer). The equilibration time for all experiments was chosen as 1 h (from the time of the addition of 10 mg net-poly(HEMA-co-AA) microparticles). The formula given below was used to determine the adsorption performance of microparticles:
(1)
(1)
Wherein; q is the adsorption capacity (mg/g), Ci and Cf are the concentrations of hemoglobin before and after adsorption (mg/L), respectively, V is the volume of the adsorption medium (L), and m is the mass of the net-poly(HEMA-co-AA) microparticles (g).
The desorption experiments were also performed batch wise with the desorption time of 1 h using the solution of NaCl (1.0 M in concentration). The reusability performance was determined at the end of the five adsorption-desorption cycles with the same microparticles. The formula given below was used for the calculation of desorption ratio:
(2)
(2)
For the determination of hemoglobin adsorption performance of microparticles, a real blood sample was also studied. The blood sample taken from a voluntary was studied without any preservatives used. A centrifugation process was applied (14000 rpm, 3 min) at room conditions. After removal of the plasma, the red blood cells remained were eluted with a salt solution to get rid of the buffy coat. The ice-cold distilled water was used to get hemolysis and erythrocyte were centrifuged at 12000 rpm (40 mins).
The hemolysate solution of 10 mL was incubated with the net-poly(HEMA-co-AA) microparticles for 2 h at 25 °C after the removal of the cell content carefully. UV-Vis spectrophotometry (Double Beam PC 8 Auto Cell Scanning UVD-3200 Labomed INC., Los Angeles, CA) was used (at 280 nm) to determine the hemoglobin binding capacity of microparticles and the formula given by EquationEquation 1(1)
(1) was used to calculate the amount of adsorption.
Results and discussion
The characterization of net-poly(2-hydroxyethyl methacrylate-co-acrylic acid) microparticles
The spherical shape with the smooth surface of microparticles can be seen clearly from the images (). The surface and bulk properties of the polymeric structure have been affected by the addition of acrylic acid as can be seen in . According to the TEM image, the spherical structure of microparticles is obviously achieved with the smooth surface proved by AFM image. Moreover, the specific surface area of the microparticles obtained in this study was found as 26.673 m2/g, which is quite comparable with the literature (Idil et al. Citation2015, Tüzmen et al. Citation2012).
Figure 1. The (a) SEM, and TEM images of (b) poly(HEMA-co-AA), (c) hemoglobin-adsorbed, and (d) The AFM image of poly(HEMA-co-AA) microparticles.
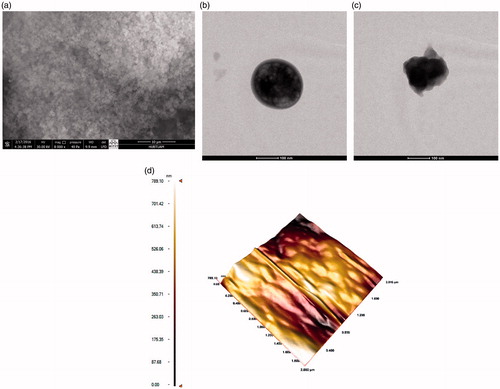
The bands obtained from the FTIR spectra are as follows (): 1722 cm−1 (C = O stretching), 1454 cm−1 (–CH2– bending), 1387 (CH–CO bending) and 1226 cm−1 (OH bending and C–O stretching). It can be concluded that the desired polymeric structure was obtained successfully with the incorporation of the functional monomer.
The water swelling ratio of microparticles (10 mg) has been determined at the end of the stirring period with distilled water (10 mL) in the rotator for 30 min at room temperature. After centrifugation (5000 rpm) for 10 min, the water uptake ratio of microparticles has been found to be 47%. The diffusion of that much of water into the pores and surface of microparticles enables the more-effective adsorption. This is actually quite significant and comparable result with the literature (Altintas et al. Citation2011).
The surface zeta potential of microparticles has been found as −21.1 mV with a standard deviation of 3.31 mV, a quite efficient result meaning that the interaction between particles is optimum, i.e., minimum or almost no effect on the adsorption. Moreover, average particle size has been determined as 2.620 μm.
The adsorption-desorption experiments
The maximum adsorption capacity of microparticles was obtained at pH 7.4, which is the pH of human blood (Fang et al. Citation2015) (). The value was slightly higher as compared to the pI value of hemoglobin which is 6.9–7.0. This is a very important result for the medical applications due to the ease of application of these microparticles. At this pH value, the carbonyl and –OH groups of AA are negatively charged. Therefore, this pH also enhances the electrostatic interaction between positive groups in hemoglobin and AA to the highest level. The pH values below and over that point do not provide the higher hemoglobin adsorption because of increase and decrease of the charge distribution on the functional groups of hemoglobin and AA with changing pH.
Figure 3. The effect of pH on the adsorption of, hemoglobin. Chemoglobin: 3.0 mg/mL; interaction time: 20 min; mmicroparticle: 10 mg; T: 25 °C.
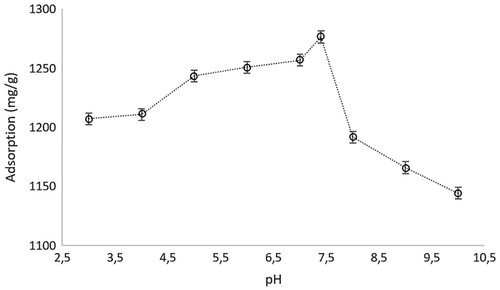
The effect of temperature on the adsorption of hemoglobin onto the microparticles was investigated in the temperature interval of 4–50 °C. Because of the ionic interaction between net-poly(HEMA-co-AA) microparticles and hemoglobin, it seems almost no change observed in a large-scale interval, but if we look at the interval in detail, adsorption performance was significantly decreased with increasing temperature (). Over 37 °C, which is life temperature, the adsorption was significantly decreased. The application of the polymeric structure developed in this study was planned to be used for living things. Therefore, experimental results provide us the usage of these microparticles in biological systems.
Figure 4. The effect of temperature on the adsorption of hemoglobin. pH:7.4; Chemoglobin: 3.0 mg/mL; interaction time: 20 min; mmicroparticle: 10 mg.
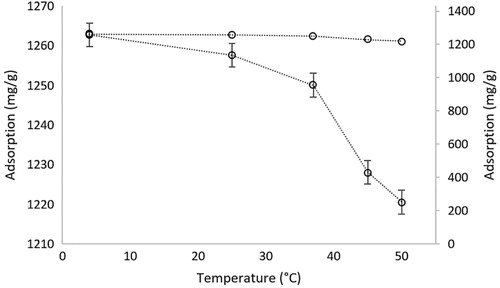
For the determination of optimum adsorption time, all experiments were performed at the interaction times extending from 0 to 90 min. The completion of adsorption was achieved at first 20 min, a considerably short time (). However, it was achieved almost 98% adsorption in 10 min, but the experiments were continued to have total adsorption up to 20 min in rest of the experiments.
Figure 5. The effect of contact time on the adsorption of hemoglobin. pH:7.4; Chemoglobin: 3.0 mg/mL; mmicroparticle: 10 mg; T: 25 °C.
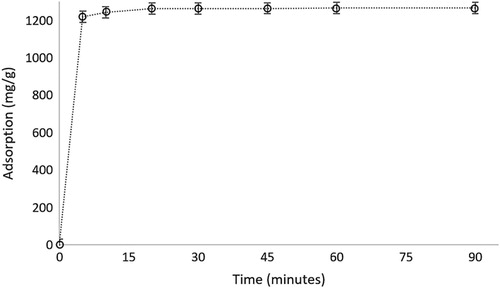
The increasing concentration of hemoglobin results in the increase in adsorption capacity up to a plateau value obtained at 3000 ppm. This explains that there are still free ligands to attract the target molecule (hemoglobin) up to that value. When all binding sites are occupied, there will be no adsorption on the polymeric material ().
Figure 6. The effect of initial concentration of hemoglobin on the adsorption capacity of microparticles. pH: 7.4; interaction time: 20 min; mmicroparticle: 10 mg.
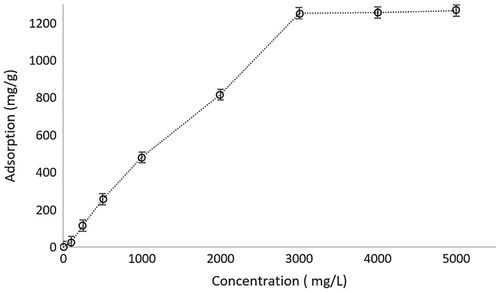
In the case of ionic strength, there was a decrease observed in the hemoglobin adsorption performance of microparticles because of the shielding effect of anion and cation of salts (). The binding sites of microparticles are negatively charged and because of the positively charged iron on the hemoglobin structure, there is an electrostatic interaction between the microparticle and target molecule. As expected, when salt ions interfere with these interactions, binding sites of microparticles will be engaged with salt ions instead of the target molecules. Therefore, our result is in accordance with the theoretical bases.
Figure 7. The effect of ionic strength of hemoglobin solution on the adsorption capacity of microparticles. pH: 7.4; CHemoglobin: 3.0 mg/mL; interaction time: 20 min; mmicroparticle: 10 mg.
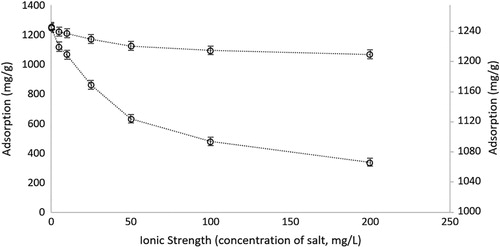
To determine the effect of the ligand on the adsorption of hemoglobin, experiments were conducted using poly(HEMA) and net-poly(HEMA-co-AA) microparticles in parallel in the same optimum conditions. The adsorption performance of poly(HEMA) was very much below that of the net-poly(HEMA-co-AA) microparticles. (). Actually, the addition of acrylic acid as a ligand into the structure significantly increases the hemoglobin adsorption capacity of microparticles.
Figure 8. The adsorption performance of poly(HEMA) and poly(HEMA-co-AA) microparticles and the reusability of poly(HEMA-co-AA) microparticles. pH: 7.4; CHemoglobin: 3.0 mg/mL; interaction time: 20 min; mmicroparticle: 10 mg.
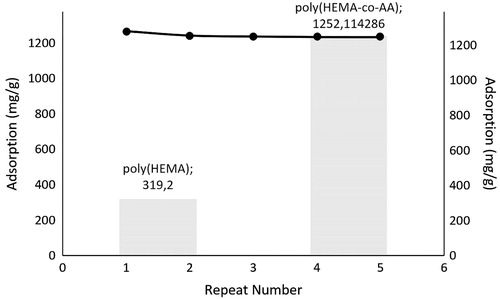
The reusability performance of microparticles was determined via same hemoglobin-adsorbed microparticles at the end of the 5-cycle of adsorption-desorption with NaCl solution of 1.0 M (0.01 g) (Armutcu et al. Citation2014) as eluent at 100 rpm during 1 h through batch-wise experimental setup. The microparticles were washed with NaOH solution of 50 mM between two adsorption-desorption cycles and regenerated.
According to the , the hemoglobin recovery ratio of microparticles was found to be 98% at the end of the first cycle and no significant decrease was observed at the end of the 5-cycle with the decrease to the value ∼93%. This is very important and effective result. A great reusability performance was obtained for future experiments.
Removal of abundant and large proteins from blood may be useful for the determination of properties of blood proteins in detail. Adsorption of hemoglobin was performed in natural blood hemolysate via net-poly(HEMA-co-AA) microparticles using 1:3 diluted blood hemolysate and the performance of microparticles is considerably comparable with the literature (∼320 mg/g, 53.8% ratio of depletion) (Altintas et al. Citation2011).
The purity of hemoglobin desorbed from real blood sample was verified using UV-VIS Spectrophotometer. A broaden-shape spectrum was obtained due to the existence of other biomolecules than hemoglobin in the blood. As the number of species in sample decreases (means more homogeneous and pure), the spectrum gets narrower and sharper. As can be seen from the spectra, the hemoglobin desorbed from the real blood sample has exactly the same spectrum (same wavelength) with that obtained by standard hemoglobin solution (). There was no impurity observed for the hemoglobin desorbed from the microparticles, which adsorbed hemoglobin in a real blood sample.
Figure 9. The UV-Vis spectra of a blood sample, standard hemoglobin solution and hemoglobin desorbed from a blood sample. pH: 7.4; interaction time: 20 min; mmicroparticle: 10 mg.
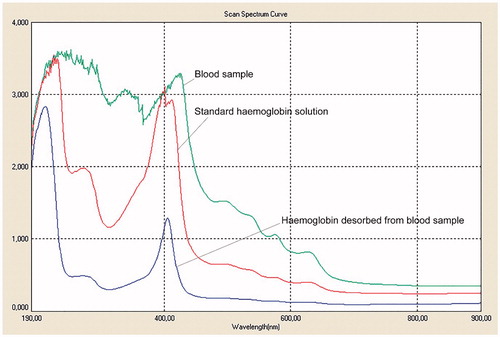
The adsorption of hemoglobin, lysozyme, and invertase molecules onto the microparticles were studied under pre-determined optimum adsorption conditions (pH 7.4, interaction time: 20 min., the concentration of these molecules is 3 mg/mL) to determine the selectivity of microparticles. The adsorption capacity obtained at the end of the study for hemoglobin, lysozyme and invertase are 1263.5, 302.1, and 218.3 mg/g, respectively. It can be said in the light of these results that the positive charges owned by hemoglobin (because pf Fe2 + ions and histidine amino acids) provides a selectivity towards acrylic acid groups in microparticles as regards to invertase and lysozyme molecules. Because, lysozyme and invertase are not a kind of metalloprotein. Moreover, these biological molecules have not histidin and other positively charged amino acids as much as hemoglobin has.
As can be seen from the experimental results, this study shows the applicability of net-poly(HEMA-co-AA) microparticles for in-vitro biological applications like dialysis method. Blood may be passed through a line and go into a container consisting the microparticles synthesized in this study, and treated in terms of high amount hemoglobin or Fe2 + content of blood and then exit from the container to go the body by means of a line. As to be noted, these microparticles are not necessary to be biocompatible because of the aimed in-vitro applications.
Concluding remarks
As mentioned before, hemoglobin is a very important molecule and the separation, purification, and removal of this molecule are significantly important in biological and medical applications. Because of this importance, there were several studies in the literature about the development of new and efficient techniques for the removal of large molecules especially hemoglobin, but most of them have some drawbacks or not enough performance. In this study, we try to overcome some disadvantages by means of the costly effective magnetic net-poly(HEMA-co-AA) microparticles due to its high adsorption performance depending on the high surface area with respect to cryogel etc. Moreover, the interaction time is significantly short, which is very important criteria for clinical applications. The electrostatic interaction was observed between ligand and target molecule and thus, adsorption was decreased with increasing ionic strength and temperature. The purity of hemoglobin obtained at the end of all process was nearly in the grade of stroma-free hemoglobin. However, there is still some points to be improved such as increasing surface area and thus adsorption capacity. These materials may be improved especially for in-vitro medical applications.
Acknowledgements
The authors thank to Hitit University, Assoc. Prof. Dr. Dursun Ali Köse and Prof. Dr. Adil Denizli for their valuable contributions and supporting facilities.
Disclosure statement
This study was supported by Hitit University, Scientific Research Projects Coordination Unit with a grant number as ODMYO19001.16.001.
References
- Altintas EB, Turkmen D, Karakoc V, Denizli A. 2011. Hemoglobin binding from human blood hemolysate with poly(glycidyl methacrylate) beads. Colloids Surf B. 85:235–240.
- Anagnostopoulos AK, Dimas KS, Papathanassiou C, Braoudaki M, Anastasiadou E, Vougas K, et al. 2011. Proteomics studies of childhood pilocytic astrocytoma. J Proteome Res. 10:2555–2565.
- Andrade CT, Barros LA, Lima MC, Azero EG. 2004. Purification and characterization of human hemoglobin: effect of the hemolysis conditions. Int J Biol Macromol. 34:233–240.
- Anirudhan TS, Rejeena SR. 2013. Selective adsorption of hemoglobin using polymer-grafted-magnetite nanocellulose composite. Carbohydr Polym. 93:518–527.
- Anirudhan TS, Senan P. 2011. Adsorptive potential of sulfonated poly(glycidylmethacrylate)-grafted cellulose for separation of lysozyme from aqueous phase: Mass transfer analysis, kinetic and equilibrium profiles. Colloids Surf A. 377:156–166.
- Armutcu C, Bereli N, Bayram E, Uzun L, Say R, Denizli A. 2014. Aspartic acid incorporated monolithic columns for affinity glycoprotein purification. Colloids Surf B. 114:67–74.
- Bain BJ, Amos RJ, Bareford D, Chapman C, Davies SC, Old JM, Wild BJ. 1998. The laboratory diagnosis of haemoglobinopathies. Br J Haematol. 101:783–792.
- Beeskow T, Kroner KH, Anspach FB. 1997. Nylon-based affinity membranes: impacts of surface modification on protein adsorption. J Colloid Interface Sci. 196:278–291.
- Bhattacharya D, Mukhopadhyay D, Chakrabarti A. 2007. Hemoglobin depletion from red blood cell cytosol reveals new proteins in 2-D gel-based proteomics study. Proteomics Clin Appl. 1:561–564.
- Derazshamshir A, Baydemir G, Andac M, Say R, Galaev IY, Denizli A. 2010. Molecularly imprinted PHEMA-based cryogel for depletion of hemoglobin from human blood. Macromol Chem Phys. 211:657–668.
- Dimino ML, Palmer AF. 2007. Purification of bovine hemoglobin via fast performance liquid chromatography. J Chromatogr B Analyt Technol Biomed Life Sci. 856:353–357.
- Ding C, Ma X, Yao X, Jia L. 2015. Facile synthesis of copper(II)-decorated magnetic particles for selective removal of hemoglobin from blood samples. J Chromatogr A. 1424:18–26.
- Ding SJ, Wang Y, Jacobs JM, Qian WJ, Yang F, Tolmachev AV, et al. 2008. Quantitative phosphoproteome analysis of lysophosphatidic acid induced chemotaxis applying dual-step (18)O labeling coupled with immobilized metal-ion affinity chromatography. J Proteome Res. 7:4215–4224.
- Erol K, Kose K, Kose DA, Sizir U, Satir IT, Uzun L. 2016. Adsorption of Victoria Blue R (VBR) dye on magnetic microparticles containing Fe(II)-Co(II) double salt. Desalin. Water Treat. 57:9307–9317.
- Fang KF, Chen ZJ, Liu M, Wu PS, Yu DZ. 2015. Blood pH in coronary artery microthrombosis of rats. Asian Pac J Trop Med. 8:864–869.
- Gao R, Cui X, Hao Y, He G, Zhang M, Tang Y. 2016. Preparation of Cu(2+)-mediated magnetic imprinted polymers for the selective sorption of bovine hemoglobin. Talanta. 150:46–53.
- Goh WW, Lee YH, Ramdzan ZM, Sergot MJ, Chung M, Wong L. 2012. Proteomics signature profiling (PSP): a novel contextualization approach for cancer proteomics. J Proteome Res. 11:1571–1581.
- Haney CR, Buehler PW, Gulati A. 2000. Purification and chemical modifications of hemoglobin in developing hemoglobin based oxygen carriers. Adv Drug Deliv Rev. 40:153–169.
- Hsia JC, Er SS. 1986. Purification of stroma-free haemoglobin by ATP-agarose affinity chromatography. J Chromatogr. 374:143–148.
- Idil N, Percin I, Karakoc V, Yavuz H, Aksoz N, Denizli A. 2015. Concanavalin A immobilized magnetic poly(glycidyl methacrylate) beads for prostate specific antigen binding. Colloids Surf B Biointerf. 134:461–468.
- Kan P, Lee C. 1994. Application of aqueous two-phase system in separation/purification of stroma free hemoglobin from animal blood. Artif Cells Blood Substitut Biotechnol. 22:641–649.
- Kosaka N, Iguchi H, Ochiya T. 2010. Circulating microRNA in body fluid: a new potential biomarker for cancer diagnosis and prognosis. Cancer Sci. 101:2087–2092.
- Kose K, Denizli A. 2013. Poly(hydroxyethyl methacrylate) based magnetic nanoparticles for lysozyme purification from chicken egg white. Artif Cells Nanomed Biotechnol. 41:13–20.
- Kose K, Erol K, Emniyet AA, Kose DA, Avci GA, Uzun L. 2015. Fe(II)-Co(II) double salt incorporated magnetic hydrophobic microparticles for invertase adsorption. Appl Biochem Biotechnol. 177:1025–1039.
- Köse K. 2016. Nucleotide incorporated magnetic microparticles for isolation of DNA. Process Biochem.: in press. doi:dx.doi.org/10.1016/j.procbio.2016.07.021.
- Levine J, Weickert M, Pagratis M, Etter J, Mathews A, Fattor T, Lippincott J, Apostol I. 1998. Identification of a nickel(II) binding site on hemoglobin which confers susceptibility to oxidative deamination and intramolecular cross-linking. J Biol Chem. 273:13037–13046.
- Lezcano NE, Odo N, Kutlar A, Brambilla D, Adams RJ. 2006. Regular transfusion lowers plasma free hemoglobin in children with sickle-cell disease at risk for stroke. Stroke. 37:1424–1426.
- Li W, Zhang D, Lin B, Su Z. 2000. Purification and identification of PEGlated hemoglobin, a potential blood substitute, by chromatography and capillary electrophoresis. Chromatographia. 52:451–454.
- Lu X, Zhao D, Ma G, Su Z. 2004a. Polyethylene glycol increases purification and recovery, alters retention behavior in flow-through chromatography of hemoglobin. J Chromatogr A. 1059:233–237.
- Lu XL, Zhao DX, Su ZG. 2004b. Purification of hemoglobin by ion exchange chromatography in flow-through mode with PEG as an escort. Artif Cells Blood Substitut Immobiliz Biotechnol. 32:209–227.
- Ma Z-Y, Liu X-Q, Guan Y-P, Liu H-Z. 2006. Synthesis of magnetic silica nanospheres with metal ligands and application in affinity separation of proteins. Colloids Surf A: Physicochem Eng Aspects. 275:87–91.
- Ma ZY, Guan YP, Liu HZ. 2005a. Synthesis of monodisperse nonporous crosslinked poly(glycidyl methacrylate) particles with metal affinity ligands for protein adsorption. Polym Int. 54:1502–1507.
- Ma ZY, Guan YP, Liu XQ, Liu HZ. 2005b. Synthesis of magnetic chelator for high-capacity immobilized metal affinity adsorption of protein by cerium initiated graft polymerization. Langmuir. 21:6987–6994.
- Min Z, Xiwen H, Langxing C, Yukui Z. 2011. Preparation and characterization of iminodiacetic acid-functionalized magnetic nanoparticles and its selective removal of bovine hemoglobin. Nanotechnology. 22:065705.
- Pieper JS, Hafmans T, Veerkamp JH, van Kuppevelt TH. 2000. Development of tailor-made collagen-glycosaminoglycan matrices: EDC/NHS crosslinking, and ultrastructural aspects. Biomaterials. 21:581–593.
- Ringrose JH, van Solinge WW, Mohammed S, O'Flaherty MC, van Wijk R, Heck AJ, Slijper M. 2008. Highly efficient depletion strategy for the two most abundant erythrocyte soluble proteins improves proteome coverage dramatically. J Proteome Res. 7:3060–3063.
- Sakai H, Takeoka S, Yokohama H, Seino Y, Nishide H, Tsuchida E. 1993. Purification of concentrated hemoglobin using organic solvent and heat treatment. Protein Expr Purif. 4:563–569.
- Samuel D, Bismuth A, Mathieu D, Arulnaden JL, Reynes M, Benhamou JP, Brechot C, Bismuth H. 1991. Passive immunoprophylaxis after liver transplantation in HBsAg-positive patients. Lancet. 337:813–815.
- Shen J-R, Sun X-M, Lei Z-L, Li B-H, Qin X-R. 2002. Separation and Purification of Hemoglobin by Using Liquid-Solid Extraction System of Cu (II) IDAPEG6000-Tween80-(NH∼ 4) ∼ 2SO∼ 4. Acta Chimica Sinica-Chinese Edition. 60:859–865.
- Shepard CW, Finelli L, Alter MJ. 2005. Global epidemiology of hepatitis C virus infection. Lancet Infect Dis. 5:558–567.
- Shi C, Deng C. 2015. Immobilized metal ion affinity chromatography ZipTip pipette tip with polydopamine modification and Ti(4)(+) immobilization for selective enrichment and isolation of phosphopeptides. Talanta. 143:464–468.
- Shukoor MI, Natalio F, Tahir MN, Ksenofontov V, Therese HA, Theato P, Schroder HC, Muller WE, Tremel W. 2007. Superparamagnetic gamma-Fe(2)O(3) nanoparticles with tailored functionality for protein separation. Chem Commun (Camb). 4677–4679.
- Sowemimo-Coker SO. 2002. Red blood cell hemolysis during processing. Transfus Med Rev. 16:46–60.
- Tetala KK, Skrzypek K, Levisson M, Stamatialis DF. 2013. A metal ion charged mixed matrix membrane for selective adsorption of hemoglobin. Sep Purif Technol. 115:20–26.
- Tüzmen N, Kalburcu T, Denizli A. 2012. α-Amylase immobilization onto dye attached magnetic beads: optimization and characterization. J Mol Catal B: Enzym. 78:16–23.
- Vozarova B, Stefan N, Lindsay RS, Saremi A, Pratley RE, Bogardus C, Tataranni PA. 2002. High alanine aminotransferase is associated with decreased hepatic insulin sensitivity and predicts the development of type 2 diabetes. Diabetes. 51:1889–1895.
- Williams LM, Fu Z, Dulloor P, Yen T, Barron-Casella E, Savage W, et al. 2010. Hemoglobin depletion from plasma: considerations for proteomic discovery in sickle cell disease and other hemolytic processes. Proteomics Clin Appl. 4:926–930.
- Zhang M, Cheng D, He X, Chen L, Zhang Y. 2010a. Magnetic silica-coated sub-microspheres with immobilized metal ions for the selective removal of bovine hemoglobin from bovine blood. Chem Asian J. 5:1332–1340.
- Zhang M, He XW, Chen LX, Zhang YK. 2010b. Preparation of IDA-Cu functionalized core-satellite Fe3O4/polydopamine/Au magnetic nanocomposites and their application for depletion of abundant protein in bovine blood. J Mater Chem. 20:10696–10704.