Abstract
The aim of this study is to investigate the usability of cryogel columns for the purification of invertase from Saccharomyces cerevisiae. Poly(2-hydroxyethyl methacrylate) monolithic columns were produced via cryogelation. Ester groups of the poly(2-hydroxyethyl methacrylate) structure were then converted to imine groups by the reaction with poly(ethylene imine) in the presence of NaHCO3. Transition metal ions, Cu(II), Co(II), and Ni(II), were chelated on the PEI-modified cryogel columns. Purification of invertase from natural source namely S. cerevisiae was also studied, and the purification fold values were obtained as 41.350, 44.714, and 30.302 for Cu(II)-chelated, Co(II)-chelated, and Ni(II)-chelated PHEMA/PEI columns, respectively.
Introduction
Invertase or officially named β-fructofuranoside fructohydrolase (EC 3.2.1.26) is an enzyme used to manufacture invert sugar. It catalyzes the hydrolysis of sucrose into equimolar mixture of D-glucose and D-fructose (Kim et al. Citation2011). Hydrolysis of sucrose by invertase rather than acid has some preferences such as colorless product, higher yield, and no salt content (Andjelković et al. Citation2015) Because invert sugar is less prone to crystallization and sweeter than sucrose itself, invertase is widely used in food industry (Veana et al. Citation2014). It is also used for bee feeding, production of certain organic acids, alcohol, and plasticizing agents (Andjelković et al. Citation2015). Furthermore, invertase acts as a transfructoslating enzyme to produce fructooligosaccharides (FOS) known as neo-sugars (Kim et al. Citation2011). Since FOS are nondigestible carbohydrates and reduce cholesterol levels, they are used in low-calorie foods for consumption by especially diabetics (Rustiguel et al. Citation2011). In nature, invertase exists in plants, certain animal tissues, and various microorganisms (Yücekan and Önal Citation2011). Among microorganisms, Saccharomyces cerevisiae is a good candidate as a source of invertase since it is nonpathogenic and nontoxicogenic (Andjelković et al. Citation2010).
Hydrogels produced under semi-frozen conditions, i.e., cryogels, have attracted great interest in bioseparation applications (Derazshamshir et al. Citation2016, Soomro et al. Citation2016). Cryogels have supermacropores with diameter up to 100 μm and flow channels which provide convective transport of solution (Baydemir and Denizli Citation2015, Kumar and Srivastava Citation2010). It leads efficient mass transfer, low pressure drop, and short process time (Ersöz et al. Citation2014). Immobilized metal affinity chromatography (IMAC), first presented by Porath et al. in Citation1975, is a technique based on the interactions between support materials and biomolecules via metal ion coordination. IMAC presents effective results in the purification of a variety of proteins and peptides (Bereli et al. Citation2010, Block et al. Citation2009, Zhang et al. Citation2005).
Different strategies, including ammonium sulfate precipitation (Nadeem at al. Citation2009, Nguyen et al. Citation2005, Sivakumar et al. Citation2013) and a wide range of chromatographic techniques, have been used for the purification of enzymes including invertase from different natural sources (Akkaya et al. Citation2009, Bhatti et al. Citation2006, Guimarães et al. Citation2007, Kayirhan and Çelebi Citation1999, L’Hocine et al. Citation2000, Uygun et al. Citation2014). Pomegranate peel waste was used as substrate in order to produce invertase from Cladosporium cladosporioides by submerged fermentation (Uma et al. Citation2012). Invertase was also purified from Semillon wine, and physicochemical and enzymatic properties of the enzyme were compared with the one from Semillon grape juice (Nakanishi et al. Citation1991). Three-phase partitioning method was used to purify invertase from both Baker’s yeast (S. cerevisiae) (Akardere et al. Citation2010) and tomato (Lycopersicon esculentum) (Özer et al. Citation2010) in a single step. In a different approach, concanavalin A immobilized affinity cryogel system was carried out to investigate the adsorption of invertase from aqueous solutions (Uygun et al. Citation2012).
In this study, PHEMA monolithic cryogel columns were prepared by radical polymerization of 2-hydroxyethyl methacrylate (HEMA) as functional monomer and N-N′-methylene-bisacrylamide (MBAAm) as cross-linker. Because cryogel has relatively large pores, it has low surface area causing lower adsorption capacities (Asliyuce et al. Citation2012). Poly(ethylene imine) (PEI) was covalently attached on the PHEMA cryogel to form a tentacle-type structure in order to increase adsorption capacity of the cryogel matrix (Müller Citation1990, Özgür et al. Citation2011). In tentacle-type structure, polymer brushes reach away from the support surface, allowing analyte molecules can bind the multiple layers of the tentacle polymer. Therefore, tentacle-type supports increase the adsorption capacity of analyte molecules, particularly the macromolecules such as proteins (Müller Citation1990, Türkmen et al. Citation2006). Tentacle-type structures also provide decrease in mass transfer resistance due to lower nonspecific interactions between the analyte and the matrix (Müller Citation1990). Then, Cu(II), Co(II), and Ni(II) were chelated onto the PHEMA/PEI cryogel columns. Effects of invertase concentration, medium pH, and temperature on the invertase adsorption from aqueous solutions were studied. Adsorption kinetics and isotherm studies were conducted to evaluate the adsorption capacity of invertase onto metal ions-chelated PHEMA/PEI cryogel columns. Purification of invertase from natural source, i.e., fresh Baker’s yeast, was also studied.
Experimental
Materials
Fresh Baker’s yeast (S. cerevisiae) was obtained from grocery store. HEMA and N-N′-methylene-bisacrylamide (MBAAm), N,N,N′,N′-tetramethyl ethylene diamine (TEMED), ammonium persulfate (APS), and PEI [50% (w/v) in H2O] were purchased from Sigma-Aldrich Corp. (St Louis, MO). All other chemicals are of reagent grade and were purchased from Merck AG (Darmstadt, Germany).
Preparation of PHEMA cryogel columns
The preparation of the PHEMA cryogel has been described elsewhere (Bereli et al. Citation2008). First, the cross-linker MBAAm (0.3 g) was dissolved in 12.0 mL of purified water, and the main monomer HEMA (1.3 mL) was added into this solution. Then, the mixture was degassed and kept in an ice bath for 10 min. The cryogel columns were prepared via free radical polymerization initiated by TEMED and APS. APS (20 mg, 1% (w/v) of the total monomers) was added into the reaction mixture, it was cooled in an ice bath for 2–3 min. After adding TEMED (25 μL, 1% (w/v) of the total monomers), the solution was stirred for 1 min. Subsequently, this homogenous mixture was poured into a plastic syringe (2 mL) with a closed outlet at the bottom. The polymerization mixture in the syringe was frozen at −16 °C for 24 h and then thawed to room temperature (RT). After washing with 200 mL of water, the cryogel was stored in buffer containing 0.02% sodium azide (NaN3) at 4 °C until use.
Characterization of PHEMA cryogel columns
The specific surface area of dried cryogel columns was detected by using multipoint Brunauer–Emmett–Teller (BET) apparatus (NOVA 2000, Quantachrome, USA). Cryogel columns were weighed and put in a sample holder. After degassing with nitrogen gas at 150 °C for 1 h, the cryogels were weighed again. Adsorption of the nitrogen was carried out at −210 °C, and desorption was applied at room temperature. Surface area of the samples was determined by the data procured from desorption part.
Swelling degree (S) of cryogel columns was obtained as follows: Each sample was first dried and weighed (Wo, g). Then dried cryogels were plunged in pure water at 25 ± 0.5 °C for 2 h. Then, the swollen cryogel columns were pulled out of the water, wiped carefully by a filter paper, and then weighed (Ws, g). The equilibrium mass swelling degree was calculated by the EquationEquation (1)(1) :
(1)
The total volume of macro-pores in the swelled cryogel structure was roughly estimated by the EquationEquation (2)(2) :
(2)
where, W1 and W2 are fully swelled gel and squeezed gel weights (g), respectively.
SEM was used to investigate the surface morphologies of the cryogel columns. After lyophilization process, cryogels were mounted on a SEM sample holder and were subjected to gold film coating for 2 min. The surface of cryogels was scanned and SEM images of the cryogels at desired magnification were captured (Jeol Ltd., JSM 5600, Tokyo, Japan).
PEI attachment
The following procedure discussed in our early study was applied (Özgür et al. Citation2011). For activation process, PHEMA cryogel column was washed with pure water for around 24 h and kept in 0.5 M NaCl solution and then pure water. About 15 mL of Na2CO3–NaHCO3 buffer (0.5 M, pH 10.5) was recirculated through the cryogel column at 1.0 mL/min at RT. For attachment of PEI process, PEI solution (10% (w/w), pH 10.6) was recirculated through the column at 1.0 mL/min at 55 °C for 6 h. The PHEMA/PEI cryogel was extensively washed with pure water to get rid of any physical adsorbed PEI from the cryogel matrix. In order to determine the amount of PEI attached onto the PHEMA cryogel matrix, both PEI attached and nonattached PHEMA cryogel columns were dried and ground into powder. The PEI content was calculated by the difference of nitrogen amount of these two columns with elemental analysis using an elemental analyzer (Thermo Scientific, Flash 2000, Italy).
Chelation of metal ions
The chelation of metal ions, including Cu(II), Co(II), and Ni(II), from the single-metal-ion solutions was investigated in a recirculating system. An aqueous metal-ion solution (15 mL) was treated with the PHEMA/PEI cryogel columns for 2 h (equilibrium adsorption time) at RT. The initial concentrations of the metal ions were 100 ppm (mg/L) with the medium pH 5.0. Standard metal-ion solutions were prepared from the nitrate salts of the metals. After the desired treatment periods, the concentrations of the metal ions in the aqueous phase were determined by using a graphite furnace atomic absorption spectrophotometer (Carl Zeiss Technology, AAS 5EA, Jena Zeiss Analytical Systems, Germany). The instrument responses were periodically checked with the known metal solution standards.
Invertase adsorption and elution studies
Invertase adsorption onto metal ion-chelated PHEMA/PEI cryogel columns experiments were applied at various pH values, in sodium acetate buffer (0.1 M, pH 4.0–5.5) and in sodium phosphate buffer (0.1 M, pH 6.0–8.0) with the 1.5 mg/mL initial invertase concentration. The adsorption studies were conducted for 2 h at 25 °C in a continuous system. At the end of this period, the columns were washed with the same buffer. The adsorption was followed by monitoring the decrease in UV absorbance at 280 nm. Effects of concentration of invertase (0.1–3.0 mg/mL) and temperature (4 to 45 °C) on the adsorption amount were examined. All the adsorption studies were applied in replicates of three, and standard statistical methods were used to find the mean values and relative SDs for each set of given data. Confidence intervals of 95% were calculated for each set of samples so as to find out the margin of error. The amount of invertase adsorption per unit mass of the cryogel sample was calculated using the mass balance.
Elution of invertase from metal ion-chelated PHEMA/PEI cryogel columns was performed as follows: 50 mL of 1 M NaCl solution as elution agent was pumped through the cryogel column at a flow rate of 1.0 mL/min for 2 h at RT. The final concentration of invertase in the elution medium was determined spectroscopically. When elution was completed, the monolithic cryogel was washed with 0.05 M, pH 5.0 acetate buffer. After the elution of invertase, metal-ion leakage from the PHEMA/PEI cryogel was also monitored continuously. Invertase adsorption–elution cycles were repeated 10 times using the same cryogel columns in order to evaluate the reusability of the metal ion-chelated PHEMA/PEI cryogel columns.
Extraction of crude invertase from Baker’s yeast
Fresh Baker’s yeast (i.e., S. cerevisiae) was obtained from grocery store. 84 g of fresh Baker’s yeast was suspended in 300 mL 0.10 M NaHCO3 and incubated at 35 °C for 15 h. After the incubation period was completed, the suspension was centrifuged at 9000 rpm for 30 min at 4 °C and supernatant was collected. The supernatant was labeled as crude invertase extract and used for affinity experiments.
Activity assay of invertase
Activity of invertase was determined by spectrophotometric measurement of glucose which is the product of sucrose hydrolysis (Goldstein and Lampen Citation1975); 3,5-dinitrosalicilic acid method was used for the spectrophotometric measurement of glucose (Nelson Citation1944). Activity assay was achieved in three steps; first, 0.6 mL acetate buffer (pH 5.0), 0.2 mL of 0.5 M sucrose, and 0.2 mL enzyme source were incubated at 37 °C for 30 min. Dinitrosalicylic acid reagent was prepared as follows: 1 g of dinitrosalicylic was dissolved in 50 mL of pure water and 30 g of sodium potassium tartrate (Rochelle salt) was added to this solution. Then, 20 mL of 2 M NaOH was added into this solution. Final volume of the solution was fixed at 100 mL with distilled water (Coughlan and Moloney Citation1988, Miller Citation1959). After adding of dinitrosalicylic reagent, the samples were heated in a boiling water for 10 min and then were cooled to room temperature. Finally, absorbance of the samples was measured spectrophotometrically at 546 nm. One unit of invertase activity was defined as the amount of enzyme that hydrolysis sucrose to yield 1 μmol glucose per minute at 37 °C and pH 5.0.
Total protein determination
Total protein concentration of the crude invertase sample was determined by Bradford method by using BSA as a standard (Bradford Citation1976). Specific activity was calculated as the amount of enzyme unit per 1 mg of total protein.
SDS-PAGE of yeast extract fractions
Yeast extract fractions were applied to SDS-PAGE. Stacking (5%) and separating gels (10%) were prepared in a gel size of 8 × 10 cm. The samples were prepared under nondenaturating conditions and applied to Mini Protean Tetra Cell gel electrophoresis system (Bio-Rad, CA). Running of the samples were performed at 100 V for 2 h. Gel was stained with silver stain, and the image was obtained using an image analyzer (ImageQuant 300, GE Healthcare, USA).
Results and discussion
Characterization studies
Megaporous monolithic columns were produced by the cryogelation of the monomer, HEMA with MBAAm as a cross-linker in the presence of APS/TEMED as initiator/activator pair. In cryogelation, while ice crystals acting as porogen grows in the monomer solution, polymerization progresses in nonfrozen or moderately frozen aqueous phase of the solution. The SEM images of the pore structure of the cryogel matrix are displayed in . also shows some physical properties of PHEMA/PEI cryogel columns. The architecture of cryogel structure exhibits supermacropores as flow channels which are much larger than the size of the macromolecules such as proteins. Hence, this novel structure of cryogel allows the macromolecules which can pass easily through the column with low back pressures. Beside this, flow of mobile phase is mainly provided with convective transport in cryogels unlike it is primarily diffusion in hydrogels (Jain and Kumar Citation2013). Therefore, the mass transfer resistance is practically negligible. shows the hypothetical model of the metal ions-chelated PHEMA/PEI monolithic cryogel columns. Ester groups of the PHEMA reacted with PEI in the presence of NaHCO3 and thus PHEMA structure had imine groups (Özgür et al. Citation2011). The presence of PEI on the PHEMA cryogel was investigated by the elemental analysis. The amount of PEI attached onto the PHEMA cryogel was found about 78.2 mg PEI/g polymer using nitrogen stoichiometry. The digital images of the cryogel columns are shown in with the characteristic colors of metal ions chelated onto the cryogel matrix.
Figure 1. (a) Digital image (b) and hypothetical model of the anticipated metal ions-chelated PHEMA/PEI monolithic cryogel columns. (c) SEM images of the cryogel matrix.
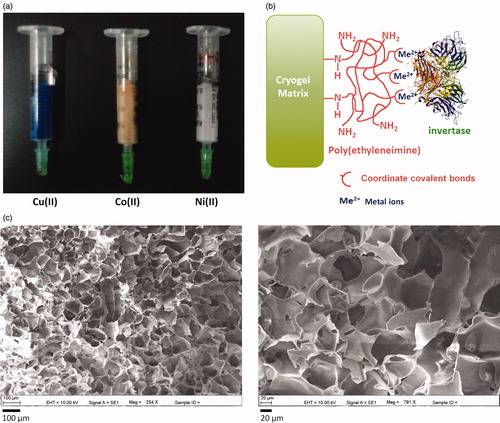
Table 1. Physical properties of PHEMA/PEI cryogel columnsTable Footnotea.
Invertase purification studies
Effect of the metal type
The metal loading values were 324.48 μmol/g polymer, 241.52 μmol/g polymer, and 236.14 μmol/g polymer for Cu(II), Co(II), Ni(II) ions-chelated cryogel columns, respectively. The initial concentrations of the metal ions were 100 ppm (mg/L) with the medium pH 5.0. At the given concentration and pH value, metal ions are predominantly in Cu(II), Co(II), and Ni(II) ions forms in the aqueous solution (Cuppett et al. Citation2006, Shobana et al. Citation2013). Cu(II) has a much stronger chelation ability to PEI than Ni(II) and Co(II) have (Wen et al. Citation2013). presents the effects of metal-ion type on the invertase adsorption from aqueous solutions. Invertase adsorption capacities on metal-chelated PHEMA/PEI monolithic cryogel columns were 16.79 mg/g for Cu(II), 15.60 mg/g for Ni(II), and 14.40 mg/g polymer for Co(II) ions under the same conditions. Cu(II), Ni(II), and Co(II) which are the 1st-row transition metal ions act as soft or borderline Lewis acids due to easily polarized nature of their d-electron shells because of orbital vacancies (Pearson Citation1968). Therefore, these metals have attractions to the nonbonding lone pair electrons of nitrogen atoms in both aromatic and aliphatic amino-containing ligands (Osman et al. Citation2005). Amino acid residues such as histidine and tryptophan of protein and α-amino group at the N-terminus are attracted by these borderline metal ions to form coordinate bonds (Chaouk and Hearn Citation1999). Sulkowski reported that the strength of adsorption between immobilized metals ions and proteins with accessible histidines has the following order: Cu(II) > Ni(II) > Co(II) (Sulkowski Citation1989, Vançan et al. Citation2002). As it is seen in the results, the same order for the invertase adsorption capacity on PHEMA/PEI cryogel columns was observed. The result is also consisted with the literature (Belew et al. Citation1987, Özkara et al. Citation2003, Ueda et al. Citation2003).
Effect of pH change
Since IMAC is mainly based on coordinate bonds, the medium pH is one of the most important factor effecting protein adsorption by the adsorbent. Because hydroxides of metals as a precipitate forms at higher values of pH 8.5 (Shobana et al. Citation2013), effect of pH on invertase adsorption by PHEMA/PEI cryogel columns was studied with the varying pH values in the range of 3.0–8.0 as indicated in . The reason of that lower adsorption capacity values were observed below pH 5.0 might be due to the fact that proton in the medium acts as a competitor ion with the metal ions to bind the ligand site of the invertase. The maximum invertase adsorption was observed at pH 5.0, which is the isoelectric point of invertase. Net charge of proteins is zero at the isoelectric point, and thus the maximum protein adsorption is usually observed at this pH values (Uygun et al. Citation2012). At higher pH values, invertase adsorption capacity also decreases. The same result reported in the literature and described as it was probably because of the preferential interactions between invertase molecules and metal ions incorporated polymeric matrix at neutral pH values (Uzun et al. Citation2011). Moreover, the decrease in the protein adsorption capacity in more acidic and more alkaline regions also might be due to the electrostatic repulsion effects between the opposite charged groups (Öztürk et al. Citation2012).
Effect of equilibrium concentration of invertase
The effects of equilibrium concentration of invertase on the adsorption capacity of metal-chelated PHEMA/PEI cryogel columns are shown in . The amount of adsorbed invertase increased with increasing of concentration of invertase because of high driving force, which is the invertase concentration difference between the liquid (i.e., the protein solution) and the solid (i.e., the cryogel matrix) phases, in the case of high invertase concentration. In addition, a saturation value was achieved at the invertase concentration of 1.5 mg/mL which represents saturation of the accessible binding sites of the metal ions on the PHEMA/PEI monolithic cryogel columns. The maximum adsorption capacities were 16.79 mg/g polymer, 14.40 mg/g polymer, and 15.60 mg/g polymer for Cu(II), Co(II), Ni(II) ions-chelated cryogel columns, respectively.
Effect of temperature
demonstrates the effect of temperature on invertase adsorption capacity of metal-chelated PHEMA/PEI cryogel columns in the range of 4–45 °C. The maximum adsorption was procured at 4 °C, and it was decreased along with increasing the temperature. Decrease percentages from 4 °C to 45 °C were determined as around 38%, 54%, and 49% for Cu(II), Co(II), Ni(II) ions-chelated cryogel columns, respectively. The enthalpy of adsorption (ΔH) for the adsorption of invertase onto the metal-chelated PHEMA/PEI cryogel columns was calculated as described in an earlier article (Kalburcu et al. Citation2014). First, the distribution coefficient Kd was defined as (Jung et al. Citation2008):
(3)
Figure 5. Effect of the temperature on invertase adsorption. pH 5.0; invertase concentration: 1.5 mg/mL.
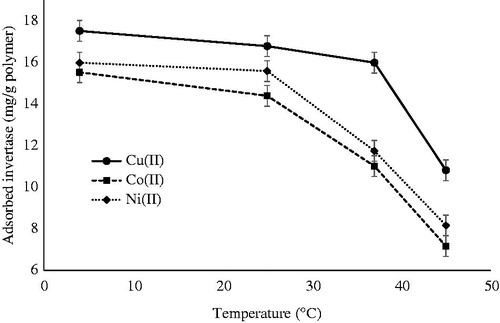
Qeq is the mass of adsorbed invertase per unit mass of adsorbent. Ceq is the equilibrium concentration of invertase (mg/mL). The enthalpy of adsorption ΔH for the adsorption of invertase can be evaluated according to Van’t Hoff equation:
(4)
This equation can be integrated and rearranged as:
(5)
The enthalpy changes of the process were obtained as −10.26 kJ/mol, −11.36 kJ/mol, and −16.69 kJ/mol for Cu(II), Co(II), and Ni(II) ions-chelated cryogel columns, respectively (Jing-Tian et al. Citation1999a, Jing-Tian et al. Citation1999b). These data show that the adsorption process of invertase onto the metal-chelated PHEMA/PEI cryogel columns has an exothermic nature.
Effect of adsorption time of invertase
presents the time dependence of the adsorption values of invertase on metal-chelated PHEMA/PEI monolithic cryogel columns. Higher adsorption values were observed at the beginning of the adsorption, and the adsorption rate was decreased by time due to fact that decrease of invertase concentration in the solution with time owing to adsorption. Saturation values were then gradually reached in about 120 min. As expected, according as the invertase concentration in the mobile phase (i.e., the protein solution) decreases which also corresponds to an increase in the stationary phase (i.e., the cryogel matrix), the driving force (i.e., the concentration difference between the two respective phases) decreases which in turn results in a drop in the adsorption rate (Denizli et al. Citation1999).
Adsorption isotherms
The modeling of the equilibrium data was performed by Langmuir and Freundlich isotherms which are represented by the EquationEqs. (6)(6) and (Equation7
(7) ), respectively (Finette et al. Citation1997, LeVan and Vermeulen Citation1981):
(6)
(7)
where, Qe is the adsorbed amount of invertase (mg/g), b (mL/mg) is the Langmuir isotherm constant, Qmax is the maximum adsorption capacity (mg/g), Ce is the equilibrium invertase concentration (mg/mL). KF and n are the Freundlich constants related to the adsorption capacity and adsorption intensity of the adsorbent, respectively. The calculated isotherm parameters were shown in . When the data given in are investigated, it is obviously seen that a high correlation coefficient values (R2) denote that Langmuir adsorption isotherm is more suitable for experimental data than the Freundlich isotherm. It can be concluded that the adsorption of invertase onto metal-chelated PHEMA/PEI cryogel is a monolayer adsorption.
Table 2. Langmuir and Freundlich adsorption isotherm constants.
Adsorption dynamics
Adsorption mechanism can be effected by the factors including mass transfer and the binding itself. The kinetic models, i.e., pseudo-first- and second-order equations can be applied to inquire these factors. The first-order rate expression of Lagergren which is used for the adsorption of solute from a liquid solution is defined by the EquationEq. (8)(8) (Cheung et al. Citation2001, Lagergren and Svenska Citation1898):
(8)
where, Qe is the experimental amount of invertase adsorbed at equilibrium (mg/g); Qt is the amount of invertase adsorbed at time t (mg/g); Q1cal is the adsorption capacity calculated by the pseudo-first-order model (mg/g); and k1 is the equilibrium rate constant of first-order adsorption (min−1).
The pseudo-second order kinetic model can be expressed with the EquationEq. (9)(9) (Ho and McKay Citation1999):
(9)
where, k2 is the equilibrium rate constant of pseudo-second-order adsorption (g/mg min); Q2cal is the adsorption capacity calculated by the pseudo-second-order kinetic model (mg/g). The results obtained from the first-order and the second-order kinetic models are given in . Since R2 values are higher in second order mechanism, this mechanism is more applicable and predominant for our system (Karataş et al. Citation2007). Hence, it can be deduced that chemisorption might be the rate-limiting step which means that it controls the adsorption process.
Table 3. The first- and second-order kinetic constants.
Reusability of the cryogel columns
In order to show the stability and reusability of the metal ion-chelated PHEMA/PEI cryogel columns, the adsorption–elution cycles were repeated 10 times using the same cryogel columns in a continuous experimental set-up. As seen in the , there were no remarkable decreases in the invertase adsorption capacities at the end of 10 adsorption–elution cycles. It indicates that metal ion-chelated cryogel columns are very stable, and maintain their adsorption capacities at almost constant values of 96.8%, 95.6%, 96.3% for Cu(II), Co(II), and Ni(II) ions-chelated cryogel columns, respectively.
Purification of invertase from Saccharomyces cerevisiae
summarizes the results of purification steps for invertase from S. cerevisiae. According to the results, yield of 92.69%, 88.76%, and 93.09% were obtained for the purification of invertase from S. cerevisiae using Cu(II)-chelated, Co(II)-chelated, and Ni(II)-chelated PHEMA/PEI cryogel columns, respectively. Purification fold values were obtained as 41.35, 44.71, and 30.30 for Cu(II)-chelated, Co(II)-chelated, and Ni(II)-chelated PHEMA/PEI cryogel columns, respectively. The yield and the purification fold were higher for Co(II)-chelated PHEMA/PEI column than the other columns. As the purification fold value indicates the purity of the enzyme, it can be concluded that purification of invertase using Co(II)-chelated PHEMA/PEI column is the most effective for the purification of invertase. In literature, it is expressed that the highest protein retention does not necessarily mean the best protein separation, because very high retention may also cause an increase in adsorption of impurities (Ueda et al. Citation2003). High purification fold values shown in indicate the effective binding of invertase on metal-chelated columns.
Table 4. Purification table for the isolation of invertase from Saccharomyces cerevisiae.
SDS-PAGE results were presented in . The molecular weight of the yeast invertase is 270 kDa. Invertase is a glycoprotein and contains 50% carbohydrate (Neumann and Lampen Citation1967). Therefore, invertase bands on SDS-PAGE image gives “smeared” appearance and can be distinguished from other protein bands. As clearly seen in lane 5, commercial invertase contains some other protein bands in addition to invertase. Silver stain is a sensitive method and can detect proteins even the amount of the proteins is in “ng” scale. Therefore, appearance of the other bands could be dependent on staining method. As the best results obtained from Co(II)-chelated PHEMA/PEI cryogel column, we have applied the fractions from Co(II)-chelated PHEMA/PEI column to SDS-PAGE. The single band on lane 2 shows the desorbed fraction from Co(II)-chelated PHEMA/PEI cryogel column and indicates the success of invertase purification from S. cerevisiae.
Figure 8. SDS-PAGE analysis of yeast fractions. Lane 1. Molecular weight marker. Lane 2. Desorbed fraction from Co(II)-chelated PHEMA/PEI cryogel column. 3. Fraction obtained after adsorption on Co(II)-chelated PHEMA/PEI cryogel column. 4. Yeast extract. 5. Commercial invertase.
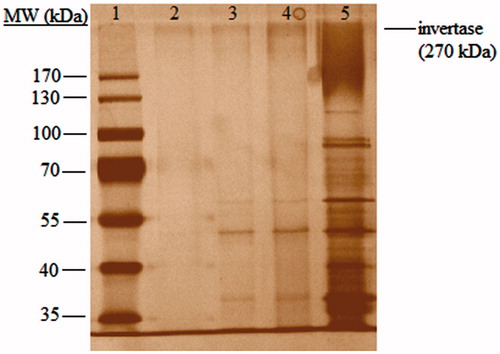
Consequently, we aimed to improve a novel reusable and cost effective tentacle-type cryogel columns for the purification of invertase from Baker’s yeast by using IMAC technique. For the purification of biomacromolecules including enzymes and the other protein types, cryogel architecture offers various advantages with not only their novel megaporous structure but also low cost of manufacture (Andaç and Denizli Citation2014, Yavuz and Denizli Citation2015). PEI which has a tentacle structure was used as chelating agent attached on PHEMA cryogel structure. Invertase adsorption capacities of PHEMA/PEI columns with different metals were investigated by changing the medium conditions such as equilibrium concentration of invertase, solution pH, and temperature. The adsorption capacities of metal ions-chelated PHEMA/PEI cryogel columns followed this order: Cu(II) > Ni(II) > Co(II). Activity assay of invertase was also studied, and Co(II)-chelated PHEMA/PEI cryogel column presented the best purification of invertase. The adsorption capacity of the tentacle-type matrix is not limited by the surface area of the matrix since adsorption of invertase is accomplished along the multilayers of tentacle structure of PEI. Because cryogels have megapores up to 100 μm, they are unique structures for grafting of tentacle-type polymers, allowing fast mass transfer without any clogging. These tentacle-type, megaporous cryogel matrices can be used for invertase adsorption under mild conditions. Because IMAC cryogels are stable and reusable products, they may provide economic advantages for large-scale applications.
Acknowledgements
Kemal Çetin thanks The Scientific and Technological Research Council of Turkey (TUBITAK) for the support by “TUBITAK-BIDEB 2211-National Ph.D. Scholarship Programme”.
Disclosure statement
The authors report no conflicts of interest. The authors alone are responsible for the content and writing of this article.
References
- Akardere E, Özer B, Çelem EB, Önal S. 2010. Three-phase partitioning of invertase from Baker’s yeast. Sep Purif Technol. 72:335–339.
- Akkaya B, Uzun L, Altintaş EB, Candan F, Denizli A. 2009. Concanavalin A immobilized monosize and magnetic poly(glycidyl methacrylate) beads for use in yeast invertase adsorption. J Macromol Sci Pure Appl Chem. 46:232–239.
- Andaç M, Denizli A. 2014. Affinity recognition based polymeric cryogels for protein depletion studies. RSC Adv. 4:31130–31141.
- Andjelković U, Milutinović-Nikolić A, Jović-Jovičić N, Banković P, Bajt T, Mojović Z, et al. 2015. Efficient stabilization of Saccharomyces cerevisiae external invertase by immobilisation on modified beidellite nanoclays. Food Chem. 168:262–269.
- Andjelković U, Pićurić S, Vujčić Z. 2010. Purification and characterisation of Saccharomyces cerevisiae external invertase isoforms. Food Chem. 120:799–804.
- Asliyuce S, Uzun L, Rad AY, Unal S, Say R, Denizli A. 2012. Molecular imprinting based composite cryogel membranes for purification of anti-hepatitis B surface antibody by fast protein liquid chromatography. J Chromatogr B. 889:95–102.
- Baydemir G, Denizli A. 2015. Heparin removal from human plasma using molecular imprinted cryogels. Artif Cells Nanomed Biotechnol. 43:403–412.
- Belew M, Yip TT, Andersson L, Ehrnström R. 1987. High-performance analytical applications of immobilized metal ion affinity chromatography. Anal Biochem. 164:457–465.
- Bereli N, Andaç M, Baydemir G, Say R, Galaev IY, Denizli A. 2008. Protein recognition via ion-coordinated molecularly imprinted supermacroporous cryogels. J Chromatogr A. 1190:18–26.
- Bereli N, Şener G, Altintaş EB, Yavuz H, Denizli A. 2010. Poly(glycidyl methacrylate) beads embedded cryogels for pseudo-specific affinity depletion of albumin and immunoglobulin G. Mater Sci Eng C. 30:323–329.
- Bhatti HN, Asgher M, Abbas A, Nawaz R, Sheikh MA. 2006. Studies on kinetics and thermostability of a novel acid invertase from Fusarium solani. J Agric Food Chem. 54:4617–4623.
- Block H, Maertens B, Spriestersbach A, Brinker N, Kubicek J, Fabis R, et al. 2009. Immobilized-metal affinity chromatography (IMAC): a review. Meth Enzymol. 463:439–473.
- Bradford MM. 1976. A rapid and sensitive method for the quantitation of microgram quantities of protein utilizing the principle of protein-dye binding. Anal Biochem. 72:248–254.
- Chaouk H, Hearn MT. 1999. Examination of the protein binding behaviour of immobilised copper (II)-2,6-diaminomethylpyridine and its application in the immobilised metal ion affinity chromatographic separation of several human serum proteins. J Biochem Biophys Meth. 39:161–177.
- Cheung CW, Porter JF, McKay G. 2001. Sorption kinetic analysis for the removal of cadmium ions from effluents using bone char. Water Res. 35:605–612.
- Coughlan MP, Moloney AP. 1988. Isolation of 1, 4-β-d-glucan 4-glucanohydrolases of Talaromyces emersonii. Meth Enzymol. 160:363–368.
- Cuppett JD, Duncan SE, Dietrich AM. 2006. Evaluation of copper speciation and water quality factors that affect aqueous copper tasting response. Chem Senses. 31:689–697.
- Denizli A, Köktürk G, Yavuz H, Pişkin E. 1999. Albumin adsorption from aqueous solutions and human plasma in a packed-bed column with Cibacron Blue F3GA-Zn(II) attached poly(EGDMA-HEMA) microbeads. React Funct Polym. 40:195–203.
- Derazshamshir A, Baydemir G, Yilmaz F, Bereli N, Denizli A. 2016. Preparation of cryogel columns for depletion of hemoglobin from human blood. Artif Cells Nanomed Biotechnol. 44:792–799.
- Ersöz A, Ünlüer ÖB, Dönmez G, Hür D, Say R. 2014. Developing column material for the separation of serum amyloid P and C reactive protein from biological sources. Biomed Chrom. 28:1345–1351.
- Finette GM, Mao QM, Hearn MT. 1997. Comparative studies on the isothermal characteristics of proteins adsorbed under batch equilibrium conditions to ion-exchange, immobilised metal ion affinity and dye affinity matrices with different ionic strength and temperature conditions. J Chromatogr A. 763:71–90.
- Goldstein A, Lampen JO. 1975. Beta-D-fructofuranoside fructohydrolase from yeast. Meth Enzymol. 42:504–511.
- Guimarães LHS, Terenzi HF, de Moraes MDLT, Jorge JA. 2007. Production and characterization of a thermostable extracellular β-D-fructofuranosidase produced by Aspergillus ochraceus with agroindustrial residues as carbon sources. Enzyme Microb Technol. 42:52–57.
- Ho YS, McKay G. 1999. Pseudo-second order model for sorption processes. Process Biochem. 34:451–465.
- Jain E, Kumar A. 2013. Disposable polymeric cryogel bioreactor matrix for therapeutic protein production. Nat Protoc. 8:821–835.
- Jing-Tian H, Guo-Xin S, Hua S, Shu-Feng C, Bo-Rong B. 1999(a). Phenyl-N, N-dibutylcarbamoylmethyl sulfoxide as a new bifunctional extractant for extraction of uranium (VI) in toluene. J Radioanal Nucl Chem. 242:821–824.
- Jing-Tian H, Guo-Xin S, Jian-Hui F, Bo-Rong B. 1999(b). Solvent extraction of uranium (VI) by N-octanoylpyrrolidine in toluene. J Radioanal Nucl Chem. 241:215–217.
- Jung Y, Kim S, Park SJ, Kim JM. 2008. Application of polymer-modified nanoporous silica to adsorbents of uranyl ions. Colloids Surf A. 313:162–166.
- Kalburcu T, Öztürk N, Tüzmen N, Akgöl S, Denizli A. 2014. Cholesterol removal onto the different hydrophobic nanospheres: a comparison study. J Ind Eng Chem. 20:153–159.
- Karataş M, Akgöl S, Yavuz H, Say R, Denizli A. 2007. Immunoglobulin G depletion from human serum with metal-chelated beads under magnetic field. Int J Biol Macromol. 40:254–260.
- Kayirhan F, Çelebi SS. 1999. Increase in the enzymatic hydrolysis rate of triacetin using polyethylene particles packed in a column reactor. Biochem Eng J. 1:153–158.
- Kim D, Lee G, Chang M, Park J, Chung Y, Lee S, Lee TK. 2011. Purification and biochemical characterization of insoluble acid invertase (INAC-INV) from pea seedlings. J Agric Food Chem. 59:11228–11233.
- Kumar A, Srivastava A. 2010. Cell separation using cryogel-based affinity chromatography. Nat Protoc. 5:1737–1747.
- Lagergren S, Svenska BK. 1898. On the theory of so-called adsorption of materials. R Swed Acad Sci Doc Band. 24:1–13.
- LeVan MD, Vermeulen T. 1981. Binary Langmuir and Freundlich isotherms for ideal adsorbed solutions. J Phys Chem. 85:3247–3250.
- L’Hocine L, Wang Z, Jiang BO, Xu S. 2000. Purification and partial characterization of fructosyltransferase and invertase from Aspergillus niger AS0023. J Biotechnol. 81:73–84.
- Miller GL. 1959. Use of dinitrosalicylic acid reagent for determination of reducing sugar. Anal Chem. 31:426–428.
- Müller W. 1990. New ion exchangers for the chromatography of biopolymers. J. Chromatogr A. 1990, 510:133–140.
- Nadeem H, Rashid MH, Riaz M, Asma B, Javed MR, Perveen R. 2009. Invertase from hyper producer strain of Aspergillus niger: physiochemical properties, thermodynamics and active site residues heat of ionization. Protein Pept Lett. 16:1098–1105.
- Nakanishi K, Wu W, Yokotsuka K. 1991. Purification and some properties of thermostable invertase from wine. J Ferment Bioeng. 71:66–68.
- Nelson NA. 1944. A photometric adaptation of the Somogyi method for the determination of glucose. J Biol Chem. 153:375–380.
- Neumann NP, Lampen JO. 1967. Purification and properties of yeast invertase. Biochemistry. 6:468–475.
- Nguyen QD, Rezessy-Szabó JM, Bhat MK, Hoschke Á. 2005. Purification and some properties of β-fructofuranosidase from Aspergillus niger IMI303386. Process Biochem. 40:2461–2466.
- Osman B, Kara A, Uzun L, Beşirli N, Denizli A. 2005. Vinyl imidazole carrying metal-chelated beads for reversible use in yeast invertase adsorption. J Mol Catal B Enzym. 37:88–94.
- Özer B, Akardere E, Çelem EB, Önal S. 2010. Three-phase partitioning as a rapid and efficient method for purification of invertase from tomato. Biochem Eng J. 50:110–115.
- Özgür E, Bereli N, Türkmen D, Ünal S, Denizli A. 2011. PHEMA cryogel for in-vitro removal of anti-dsDNA antibodies from SLE plasma. Mater Sci Eng C. 31:915–920.
- Özkara S, Yavuz H, Denizli A. 2003. Purification of immunoglobulin G from human plasma by metal-chelate affinity chromatography. J Appl Polym Sci. 89:1567–1572.
- Öztürk N, Şenay RH, Avcibaşi N, Akgöl S, Denizli A. 2012. Reversible lysozyme immobilization onto N,N′-bis-(3-(4-morpholino)-propyl)-3,4,9,10-perylenetetracarboxylic acid dimide (MPPDI) attached polymeric nanospheres. Process Biochem. 47:816–821.
- Pearson RG. 1968. Hard and soft acids and bases, HSAB, part 1: fundamental principles. J Chem Educ. 45:581.
- Porath J, Carlsson JAN, Olsson I, Belfrage G. 1975. Metal chelate affinity chromatography, a new approach to protein fractionation. Nature. 258:598–599.
- Rustiguel CB, Oliveira AHCD, Terenzi HF, Jorge JA, Guimarães LHS. 2011. Biochemical properties of an extracellular β-D-fructofuranosidase II produced by Aspergillus phoenicis under solid-sate fermentation using soy bran as substrate. Electron J Biotechnol. 14:2–2.
- Shobana S, Dharmaraja J, Selvaraj S. 2013. Mixed ligand complexation of some transition metal ions in solution and solid state: spectral characterization, antimicrobial, antioxidant, DNA cleavage activities and molecular modeling. Spectrochim Acta A Mol Biomol Spectrosc. 107:117–132.
- Sivakumar T, Ravikumar M, Prakash M, Shanmugaraju V. 2013. Production of extracelluar invertase from Saccharomyces cerevisiae strain isolated from grapes. Int J Curr Res Acd Rev 1:72–83.
- Soomro R, Perin I, Memon N, Bhanger MI, Denizli A. 2016. Gelatin-loaded p(HEMA-GMA) cryogel for high-capacity immobilization of horseradish peroxidase. Artif Cells Nanomed Biotechnol. 44:1708–1713.
- Sulkowski E. 1989. The saga of IMAC and MIT. BioEssays. 10:170–175.
- Türkmen D, Yavuz H, Denizli A. 2006. Synthesis of tentacle-type magnetic beads as immobilized metal-chelate affinity support for cytochrome c adsorption. Int J Biol Macromol. 38:126–133.
- Ueda EKM, Gout PW, Morganti L. 2003. Current and prospective applications of metal ion-protein binding. J Chromatogr A. 988:1–23.
- Uma C, Gomathi D, Ravikumar G, Kalaiselvi M, Palaniswamy M. 2012. Production and properties of invertase from a Cladosporium cladosporioides in SmF using pomegranate peel waste as substrate. Asian Pac J Trop Biomed. 2:S605–S611.
- Uygun M, Karagözler AA, Denizli A. 2014. Molecularly imprinted cryogels for carbonic anhydrase purification from bovine erythrocyte. Artif Cells Nanomed Biotechnol. 42:128–137.
- Uygun M, Uygun DA, Özçalişkan E, Akgöl S, Denizli A. 2012. Concanavalin A immobilized poly(ethylene glycol dimethacrylate) based affinity cryogel matrix and usability of invertase immobilization. J Chromatogr B. 887:73–78.
- Uzun K, Çevik E, Şenel M. 2011. Invertase immobilization on a metal chelated triazole-functionalized Eupergit® C. Am J Chem. 1:16–21.
- Vançan S, Miranda EA, Bueno SMA. 2002. IMAC of human IgG: studies with IDA-immobilized copper, nickel, zinc, and cobalt ions and different buffer systems. Process Biochem. 37:573–579.
- Veana F, Fuentes-Garibay JA, Aguilar CN, Rodríguez-Herrera R, Guerrero-Olazarán M, Viader-Salvadó JM. 2014. Gene encoding a novel invertase from a xerophilic Aspergillus niger strain and production of the enzyme in Pichia pastoris. Enzyme Microb Technol. 63:28–33.
- Wen T, Qu F, Li NB, Luo HQ. 2013. A facile, sensitive, and rapid spectrophotometric method for copper (II) ion detection in aqueous media using polyethyleneimine. Arabian J Chem. doi:10.1016/j.arabjc.2013.06.013.
- Yavuz H, Denizli A. 2015. A new affinity separation medium: supermacroporous cryogels, reference module in chemistry. Mol Sci Chem Eng. doi:10.1016/B978-0-12-409547-2.10733-4.
- Yücekan İ, Önal S. 2011. Partitioning of invertase from tomato in poly(ethylene glycol)/sodium sulfate aqueous two-phase systems. Process Biochem. 46:226–232.
- Zhang L, Zhang L, Zhang W, Zhang Y. 2005. On-line concentration of peptides and proteins with the hyphenation of polymer monolithic immobilized metal affinity chromatography and capillary electrophoresis. Electrophoresis. 26:2172–2178.