Abstract
Green synthesis of gold (CB-AuNps) and silver (CB-AgNps) nanoparticles using Cibotium barometz root extract was highlighted. CB-AuNps were synthesized almost instantly and CB-AgNps were formed after 25 min in a heated aqueous extract. The formation of CB-AuNps and CB-AgNps was detected at 548 and 412 nm; they were spherical with crystallite sizes of 6 nm and 23 nm, respectively. CB-AgNps were further investigated for their antimicrobial activity against Escherichia, Staphylococcus aureus, Salmonella enterica, and Pseudomonas aeruginosa. Furthermore, scavenging activity against 1,1-diphenyl-2-picrylhydrzyl (DPPH) free radicals was demonstrated. Lastly, the cytotoxic properties of CB-AuNps and CB-AgNps were explored in murine macrophages (RAW264.7) cells and MCF-7 breast cancer cells. This study demonstrated the prospective biomedical applications of CB-AuNps and CB-AgNps as antioxidant, antimicrobial agents, and drug-delivery agents.
Introduction
Metallic nanoparticles are increasingly becoming critical in biomedical, electronic, optical, and magnetic applications (Cui et al. Citation2009). Their uniform size dimensions, optical, and physicochemical properties continue to be of immense interest and increasing importance for further nanotechnological applications. Currently, there is a developing demand to promote one-step, rapid, economical, and environmentally friendly nanoparticles synthesis approaches that avoid the usage of toxic chemicals and excessive energy consumption in the synthesis protocols (Jain et al. Citation2009, Veerasamy et al. Citation2011). Therefore, green synthesis methodologies are crucial for the development of metal nanoparticles and especially for the benefit of human health (Raveendran et al. Citation2003). In our study, we report a rapid green synthesis of functional gold and silver nanoparticles by the aqueous extract of C. barometz (L.) J. Sm. root.
Chinese herbal medicines have been widely used in clinical treatment to various diseases for thousands of years; they will continue to be used as cost-efficient commercial pharmaceutical products by traditional users. C. barometz, which belongs to the fern family Dicksoniaceae, is popularly known as “Gouji” in Chinese herbal medicine. They are widely distributed and have been extensively collected in Southeast Asia. They are abundantly present in China, Vietnam, Japan, Thailand, Indonesia, and Malaysia. Cibotium barometz is well-known for its benefits in ancient medicine. Even today, the hairs attributes to the staple ingredient in ointments used in natural remedies on wound to stop bleeding (Wu and Yang Citation2009). Moreover, the rhizome of C. barometz has anti-inflammatory, anodyne, and kidney-tonifying efficacies and is used for the treatment of rheumatism, lumbago, sciatica, dysuria, polyuria, and leucorrhoea in the aged population. Based on the theories of traditional Chinese herbal medicine, it is responsible for strengthening bones and tendons. In this aspect, C. barometz is one of the most frequently used herbs in formulas that are prescribed for the treatment of osteoporosis in China (Zhao et al. Citation2011). The rhizomes mainly contain caffeic acids, protocatechuic acid (Cheng et al. Citation2003, Zhang and Wang Citation2000), D-glucose (Cheng et al. Citation2003), fatty acid (Xu et al. Citation2000), and flavonoids such as karmpferol and onychin (Xu et al. Citation2004). The rhizomes also consist of fatty acids such as oletic acid, palmitic acid, and octadecanoic acid (Xu et al. Citation2000), flavonoids such as kaempferol and onychin (Xu et al. Citation2004,) and phenolic acids such as caffeic acid and protocatechuic acid (Cheng et al. Citation2003). Earlier pharmacological studies revealed that many of these natural products possessed important health benefits. For example, caffeic and protocatechuic acids were the effective constituents for osteoarthritis and rheumatoid arthritis (Yuan et al. Citation1999), palmitic acid had anti-inflammatory properties, octadecaonic acid could reduce blood fat and kaempferol and onychin have antioxidant activity and anticancer effects (Kim et al. Citation2010, Yan et al. Citation2002, Zhang et al. Citation2008). In view of these physicochemical properties, C. barometz root extract is conducive to the development of green biosynthesis of multifunctional gold and silver nanoparticles.
Considering the appealing physiochemical and biological properties, metallic nanoparticles are under intensive study and able to be applied in the development of new technologies for pharmaceutical applications. Since gold nanoparticles (AuNps) have indicated potentials in biomedical applications, especially as scaffold for drug and gene delivery, they have emerged as cancer diagnosis tools and bio-labeling sensors (Lin et al. Citation2009). Otherwise, silver nanoparticles (AgNps) have been widely used in antimicrobial agents for a long time, especially in wound dressing (Pyun et al. Citation2015), medical devices (Prabhu and Poulose Citation2012). Due to their antibacterial, anti-inflammatory, anticancer, and biofilm inhibition efficacies, AgNps become the critical candidates on biomedical platform (Singh et al. Citation2016).
To the best our knowledge, this is the first study to report the synthesis of CB-AuNps and CB-AgNps by C. barometz root extract as a biological substrate with essential in vitro biological applications. CB-AuNps and CB-AgNps were characterized and analyzed for their scavenging activities against 2,2-diphenyl-1-picrylhydrazyl (DPPH) free radicals. Furthermore, CB-AgNps were evaluated for antimicrobial activity against pathogenic Escherichia coli, Staphylococcus aureus, Salmonella enterica, and Pseudomonas aeruginosa. Lastly, in vitro cytotoxicity assay of CB-AuNps and CB-AgNps were investigated in murine macrophage (RAW264.7) and breast cancer cells (MCF-7).
Materials and methods
Materials
Cibotium barometz roots were obtained from Serom, Republic of Korea. Silver nitrate (AgNO3), gold (III) chloride trihydrate (HAuCl4⋅3H2O), DPPH, and ascorbic acid (vitamin C) were purchased from Sigma-Aldrich Chemicals (St. Louis, MO, USA). All bacterial growth media were purchased from Difco, MB Cell, Seoul, Republic of Korea. The pathogenic bacterial strains E. coli [ATCC 10798], S. aureus [ATCC 6538], S. enterica [ATCC 13076], and P. aeruginosa [ATCC 10145] were used. Antibiotic discs were obtained from Oxoid Ltd., England. The bacterial strains were cultured on nutrient agar media at 37 °C for 24 h and preserved at 70 °C in glycerol stock vials for further study.
Preparation of root extract
Cibotium barometz root was cleaned and washed with double-distilled water several times to remove any dust or suspended particles (Singh et al. Citation2015b). Afterwards, the roots were dried, cut into small pieces and pulverized into fine powder. Five grams of ground power was added into 100 mL distilled water and boiled for 30 min at 100 °C to obtain aqueous root extract. The water-extraction was collected and filtered using Whatman filter paper (No. 2) followed by centrifugation at 10,000 rpm for 10 min to remove any suspended material. Lastly, a filtrate was obtained and the total volume was maintained at 100 mL with sterile double-distilled water. The root extract stock was stored at 4 °C for further study.
Green synthesis of CB-AuNps and CB-AgNps
Five mL from the 100 mL of stock filtrate was mixed with 25 mL of sterile distilled water. The precursor gold and silver salts were added to the reaction mixtures with a final concentration of 1 mM. The reaction mixture was then kept at 80 °C until color conversion was observed. The color change of the reaction mixture indicated the formation of CB-AuNps and CB-AgNps in the reaction mixture. After the formation of nanoparticles, CB-AuNps and CB-AgNps were collected by centrifugation at 2000 rpm for 10 min to remove any other unessential components. The formed nanoparticles were purified by high-speed centrifugation at 16,000 rpm for 20 min repeatedly following dispersion of the pellet in sterile water. The purified nanoparticles were finally air dried, kept overnight and obtained in powder form. The obtained product was used for further characterization and in vitro applications.
Characterization of CB-AuNps and CB-AgNps
The presence of CB-AuNps and CB-AgNps in the reaction mixtures were verified by UV-Vis spectrophotometer (2100 Pro, Amersham, Biosciences Corp., USA). 200 μL of nanoparticle solution were scanned in the range of 300–800 nm. The morphology and purity of the nanoparticles were analyzed by field emission transmission electron microscopy (FE-TEM), energy-dispersive X-ray spectroscopy (EDX), and elemental mapping using a JEM-2100F (JEOL) electron microscope operated at a voltage of 200 kV. Before analysis, the nanoparticles were placed on carbon-coated copper grids and dried at 60 °C to evaporate the remaining solvent.
The crystallinity analyses were performed on an X-ray diffractometer (D8 Advance, Bruker, Germany) operated at 40 kV, 40 mA, with CuK α radiation of 1.54 Å, scanning rate of 6°/min, and a step size of 0.02°. Samples were scanned over the 2θ range of 20–80°. The average crystal size diameter of the nanoparticles was calculated by Debye-Scherrer equation: D = 0.9λ/β cos θ, where D is the crystallite size in nm, λ is the wavelength of CuK α radiation in nm, β is the full width at half maximum (FWHM) in radians, and θ is the half of the Bragg angle in radians (Omidi et al. Citation2014).
The size distribution of the nanoparticles in water was examined using Dynamic Light Scattering (DLS-Photal, Otsuka Electronics, Japan) technique. The hydrodynamic (Z-average) diameter and polydispersity index (PDI) was determined at 25 °C. As a reference, a dispersive medium of pure water with a refractive index of 1.3328, viscosity of 0.8878, and dielectric constant of 78.3 was used.
CB-AuNps and CB-AgNps were further characterized by Fourier Transform Infrared (FTIR) spectroscopy for examining the nature of biomolecules responsible for stabilizing the nanoparticles. The PerkinElmer Spectrum One FTIR spectrometer was used in the range of 4000–450 cm−1 at a resolution of 4 cm−1.
Antimicrobial activity of CB-AgNps
The antimicrobial activity of CB-AgNps against pathogenic microorganisms E. coli [ATCC 10798], S. aureus [ATCC 6538], S. enterica [ATCC 13076], and P. aeruginosa [ATCC 10145] were evaluated on Muller-Hinton agar (MHA) plates using the disc-diffusion method (Wang et al. Citation2016a). In this assay, the MHA agar plates were spread evenly with 100 μL of an overnight log-culture of test pathogenic microorganism, and standard antibiotic disc of neomycin (30 μg/disc) was maintained as control. The sterile paper disc was further impregnated with different concentration of purified CB-AgNps solution (15, 30, and 45 μg/disc) and placed onto the MHA agar plates and then these plated were incubated at 37 °C for 24 h. After incubation, the zones of inhibition around sterile discs impregnated with CB-AgNps were measured and compared with the zone of inhibition of antibiotic discs (Naz et al. Citation2016, Singh et al. Citation2015a, Wang et al. Citation2016b).
In vitro DPPH radical scavenging assay
A modified DPPH scavenging method was used to perform the free-radical scavenging activity of CB-AgNps (Debnath et al. Citation2016). Briefly, 20 μL of different concentrations of CB-AgNps and CB-AuNps were added to 180 μL of methanolic solution of 2,2-diphenyl-1-picrylhydrazyl (0.1 mM DPPH) and incubated in darkness at room temperature for 30 min. Absorbance was recorded at 517 nm by UV-Vis spectrophotometer. The free-radical scavenging activity was calculated using the following formula: % scavenging = A517Control – A517Test/A517Control × 100
In vitro of MTT cell proliferation assay
Murine macrophage (RAW264.7) and breast cancer cells (MCF-7) (Korean Cell Line Bank, Seoul, Korea) cell line was cultured in Dulbecco’s Modified Eagles medium (DMEM) (Gibco-BRL, Grand Island, NY) supplemented with 10% fetal bovine serum (FBS) and 1% penicillin/streptomycin (P/S) (WelGENE Inc., Daegu, Korea) at 37 °C in a humidified atmosphere containing 5% CO2 and 95% air. Then, the cells were seeded at a density of 1 × 104 per well in a 96-well plate (Corning Costar, Lowell, NY) and treated with different concentrations of CB-AuNps and CB-AgNps (0, 0.01, 0.1, 1, and 10 μg/mL) at 37 °C for 48 h at 90% confluency. After 48 h of incubation, 10 μL of 3–(4,5-dimethyl-2-thiazolyl)-2,5-diphenyl-2H tetrazolium bromide (MTT) (Life Technologies, Eugene, OR) assay solution dissolved in 5 mg/mL phosphate buffer saline were added into each well and further incubated at 37 °C for 4 h. Subsequently, 100 μL of dimethyl sulfoxide (DMSO) were added to dissolve the formazan crystals. Finally, the absorbance of each well was measured at 570 nm using an Enzyme-Linked Immunosorbent Assay reader (Bio-Tek Instruments, Inc., Vinooski, VT). The optical density of formazan formed in untreated cells (negative control) represents 100% cell viability (Ahn et al. Citation2015, Citation2016).
Results and discussion
Various plants and their extracts have been successfully reported for the synthesis of metal nanoparticles and their biomedical applications (Arunachalam and Annamalai Citation2013, Elavazhagan and Arunachalam Citation2011, MubarakAli et al. Citation2011). To our best knowledge, this is the first report for the biosynthesis of CB-AuNps and CB-AgNps by extract of C. barometz (L.) J. Sm root without the addition of hazardous solvents and capping agents. The synthesis of nanoparticles was detected by the color change of the reaction mixtures consisting of aqueous extract of C. barometz and precursor salts after incubation at 80 °C. For CB-AuNps and CB-AgNps, the color of the reaction mixtures respectively changed into ruby red and orange-brown in 1 s and 25 min, and no further color change was observed after 50 min and 1 h, respectively ().
Figure 1. Time-dependent UV-Vis spectra of the reaction mixture for (A) CB-AuNps and (B) CB-AgNps, respectively.
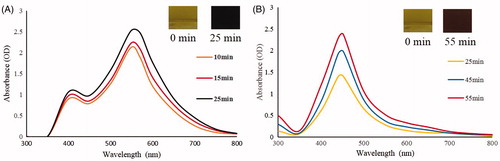
The results of FE-TEM analysis showed that the biosynthesized CB-AuNps and CB-AgNps were spherical in shape, with size ranging from 5–20 nm () and 5–40 nm (), respectively. Additionally, the elemental mapping results showed the distribution profiles of elemental gold and silver in their respective FE-TEM image (). Furthermore, the purity of nanoparticles was determined by EDX analysis. Maximum EDX peaks were determined at 2.3 keV and 3 keV, which are the characteristic peaks of gold and silver, respectively (). The characteristic peak observed at 8 keV corresponds to the copper grid used during the analysis.
Figure 2. FE-TEM images of spherical (A) CB-AuNps and (E) CB-AgNps, respectively. Elemental mapping results indicate the distribution of (B) element gold and (F) silver nanoparticle pellet solution, respectively; (C) gold (red) and (G) silver (green), respectively. EDX spectra of (D) CB-AuNps and (H) CB-AgNps, respectively.
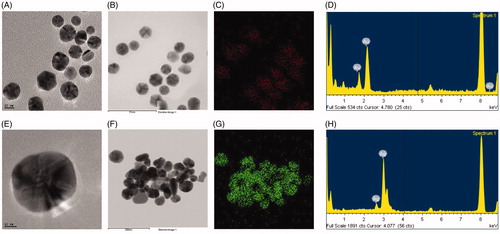
The crystal structure of the bio-synthesized CB-AuNps and CB-AgNps was analyzed by Selected Area Electron Diffraction (SAED) pattern () and XRD spectra (). The analysis was performed by scattering a beam of X-rays to diffract through atoms of nanoparticles crystals. The four diffraction peaks of gold measured at 38.19°, 44.34°, 64.48°, and 77.46° were assigned to diffraction peaks of (111), (200), (220), and (311) lattice plane of Bragg’s reflection. Similarly, the six diffraction peaks of silver measured at 27.79°, 32.21°, 38.02°, 46.21°, 54.74°, and 57.42° were assigned to diffraction peaks of (111), (200), (111), (220), (311), and (222) lattice plane of Bragg’s reflection. The biogenic CB-AuNps and CB-AgNps were found to have an average crystallite size of 6 nm and 23 nm, respectively.
Figure 3. SAED results indicate the crystallinity of biosynthesized nanoparticles of (A) CB-AuNps and (C) CB-AgNps, respectively. XRD results indicate the crystallinity of biosynthesized nanoparticles of (B) CB-AuNps and (D) CB-AgNps, respectively.
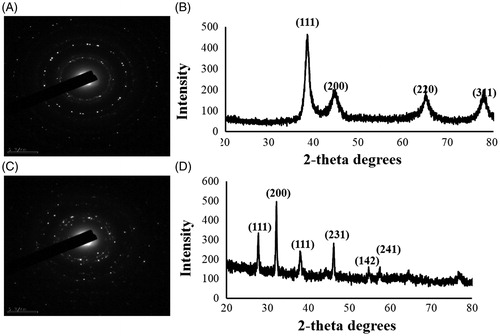
The hydrodynamic size distributions of CB-AuNps and CB-AgNps were detected by DLS analysis according to intensity, number, and volume of nanoparticles (). The results showed that the Z-average particle size of CB-AuNps was 116 nm with a PDI value of 0.240. Similarly, the Z-average particle size of CB-AgNps was 77.2 nm with a PDI value of 0.225. According to the PDI values, both nanoparticles were polydisperse in nature.
Figure 4. Particle size distribution of (A) CB-AuNps and (B) CB-AgNps with respect to number, volume, and intensity.
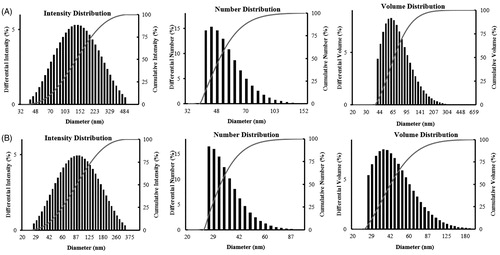
The results of FTIR spectra of CB-AuNps and CB-AgNps are represented in and compared with C. barometz root extract as positive control. The FTIR spectrum of the root extract showed sharp transmission peaks at 3429 cm−1, 2925 cm−1 1643 cm−1, and 1019 cm−1. Strong peaks at 3429 cm−1 represent O–H stretching vibration of hydroxyl group of flavonoids and phenolic acids, while the peak at 1019 cm−1 indicated the vibration of C–O stretching of esters. Otherwise, the medium peaks at 2929 cm−1 and 1643 cm−1 corresponded to C–H stretching of methyl groups and fatty acid, respectively (Chang Chien et al. Citation2007, Selvi et al. Citation2009). As the results showed, the characteristic peaks of CB-AuNps (3422 cm−1, 2918 cm−1, 1633 cm−1, and 1000 cm−1) and CB-AgNps (3422 cm−1, 2918 cm−1, 1633 cm−1, and 1000 cm−1) were transmitted in similar wavenumbers albeit with different intensities. In other words, the presence of flavonoids, phenolic acids and fatty acids contained in the root extract were also present on the nanoparticles. In conclusion, flavonoids, phenolic acids and fatty acid in C. barometz root extract are suggested to be responsible for the reduction and capping of green biosynthesized CB-AuNps and CB-AgNps.
Many studies have shown the antimicrobial activity of silver nanoparticles against pathogenic microorganisms (Dar et al. Citation2013, Geethalakshmi and Sarada Citation2012). In our study, the antimicrobial activity of the synthesized CB-AgNps was examined by standard disc diffusion assay and compared to standard antibiotics ( and ). 30 μL of purified CB-AgNps with three different concentrations of CB-AgNps (15, 30, 45 μg/disc) were used to test the antimicrobial activity against E. coli, S. aureus, S. enterica, and P. aeruginosa compared to Neomycin (30 μg/dis). Zones of inhibition began to form around paper discs after 24 h incubated at 37 °C. As shown in , the mean diameters of the zones were increased along with the increasing concentrations of CB-AgNps. The biosynthesized CB-AgNps revealed more effective zones of inhibition against S. aureus at concentration of 15 μg and 45 μg compared with inhibition against E. coli, S. enterica, and P. aeruginosa. The results indicated the antimicrobial activity of CB-AgNps is comparable to that of commercial Neomycin and that increasing the amount of CB-AgNps resulted in higher antimicrobial activity against human pathogenic microorganisms. The antimicrobial mechanism of silver nanoparticles has yet to be elucidated, however, it is suggested that the positively charged increase of silver ions accumulated around the nanoparticles at the bacterial membrane may result in transmembrane movement and cellular damage against cell membranes, which ultimately cause loss of permeability and cell death (Singh et al. Citation2016).
Figure 6. Antibacterial activity of CB-AgNps against (A) E. coli, (B) S. aureus, (C) S. enterica , and (D) P. aeruginosa.
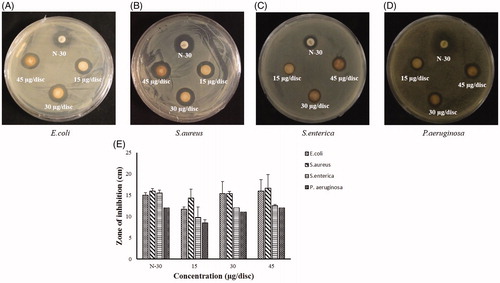
The free radical scavenging ability of CB-AuNps and CB-AgNps was evaluated by DPPH assay. The results were tested at 517 nm by UV-Vis spectrophotometer according to the color change from purple to yellow. The percentage inhibition of CB-AuNps and CB-AgNps are shown in . CB-AgNps (65.05% ± 3.28) was found to exert slightly higher inhibition than CB-AuNps (58.30% ± 3.31). The IC50 values were recorded at 1.40 and 1.22 mg/mL for CB-AuNps and CB-AgNps, respectively. These results suggest that the biosynthesized CB-AuNps and CB-AgNps show promising prospects for the development of novel antioxidant agents. Furthermore, the content of caffeic acids, protocatechuic acid fatty acid flavonoids played an important role in the enhanced free radical scavenging activities of CB-AuNps and CB-AgNps. This is the first report on the CB-AuNps and CB-AgNps synthesized by the aqueous extract of C. barometz root extract, which is ecofriendly, economical, and without any toxic agents.
Figure 7. DPPH radical scavenging activity of CB-AuNps and CB-AgNps using ascorbic acid as positive control.
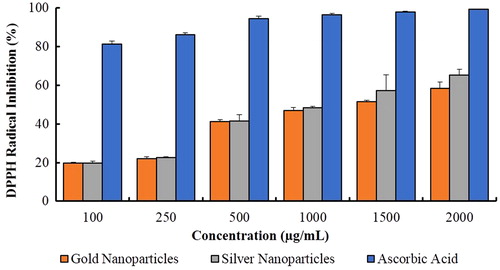
In the present study, the cytotoxicity of CB-AuNps and CB-AgNps were determined in RAW264.7 and MCF-7 as models. In vitro cytotoxicity of CB-AuNps and CB-AgNps was evaluated at concentration ranging from 0.1–10 μg/mL by MTT. CB-AuNps didn’t exhibit cell death of RAW264.7 cells and MCF-7 at a concentration of 0.1–10 μg/mL (). However, CB-AgNps showed cytotoxicity to RAW264.7 cells at concentration of ≥10 μg/mL (). Moreover, the CB-AgNPS exhibited significant cytotoxicity toward MCF-7 at concentrations more than 10 μg/mL. Based on the results, the CB-AuNps and CB-AgNps could be considered as non-toxic therapeutics delivery agents to the cancer diagnostics.
Conclusions
In the present study, we demonstrated a rapid, efficient, and eco-friendly method for synthesis of CB-AuNps and CB-AgNps for the first time by C. barometz root extract. In addition, the biosynthesized CB-AuNps and CB-AgNps were spherical with crystallite sizes of 6 nm and 23 nm respectively. Moreover, CB-AgNps showed effective antimicrobial activity against E.coli, S.aureus, S. enterica, and P. aeruginosa. The cytotoxicity of CB-AuNps and CB-AgNps was evaluated in RAW264.7 and MCF-7 cell line. CB-AuNps did not show cytotoxicity to both cells. And, CB-AgNps showed significant cytotoxicity in MCF-7 cells at concentrations more than 10 μg/mL. Thus, the combinational antimicrobial and antioxidant properties demonstrate the utility of biogenic gold and silver nanoparticles in a wide range of biomedical applications.
Acknowledgments
This research was supported by the Korea Institute of Planning & Evaluation for Technology in Food, Agriculture, Forestry & Fisheries, Republic of Korea (KIPET NO: 313038–03-2-SB010) and the Next-Generation BioGreen 21 Program (SSAC, grant#: PJ0210342016), Rural Development Administration, Republic of Korea.
Disclosure statement
The authors report no declarations of interest.
Additional information
Funding
References
- Ahn S, Singh P, Castro-Aceituno V, Yesmin Simu S, Kim YJ, Mathiyalagan R, Yang DC. 2016. Gold nanoparticles synthesized using Panax ginseng leaves suppress inflammatory - mediators production via blockade of NF-κB activation in macrophages. Artif Cells, Nanomed Biotechnol. 9:1–7.
- Ahn S, Siddiqi MH, Aceituno VC, Simu SY, Zhang J, Jimenez Perez ZE, Kim YJ, Yang DC. 2015. Ginsenoside Rg5: Rk1 attenuates TNF-α/IFN-γ-induced production of thymus-and activation-regulated chemokine (TARC/CCL17) and LPS-induced NO production via downregulation of NF-κB/p38 MAPK/STAT1 signaling in human keratinocytes and macrophages. In Vitro Cell Dev Biol Anim. 52:287–295.
- Arunachalam KD, Annamalai SK. 2013. Chrysopogon zizanioides aqueous extract mediated synthesis, characterization of crystalline silver and gold nanoparticles for biomedical applications. Int J Nanomedicine. 8:2375–2384.
- Chien SW, WANG MC, Huang CC, Seshaiah K. 2007. Characterization of humic substances derived from swine manure-based compost and correlation of their characteristics with reactivities with heavy metals. J Agric Food Chem. 55:4820–4827.
- Cheng QH, Yang ZL, Hu YM. 2003. Studies on the chemical constituents of Rhizoma Cibotii. Prog Pharm Sci. 27:298–299.
- Cui H, Feng Y, Ren W, Zeng T, Lv H, Pan Y. 2009. Strategies of large scale synthesis of monodisperse nanoparticles. Recent Pat Nanotechnol. 3:32–41.
- Dar MA, Ingle A, Rai M. 2013. Enhanced antimicrobial activity of silver nanoparticles synthesized by Cryphonectria sp. evaluated singly and in combination with antibiotics. Nanomedicine. 9:105–110.
- Debnath R, Purkayastha DD, Hazra S, Ghosh NN, Bhattacharjee CR, Rout J. 2016. Biogenic synthesis of antioxidant, shape selective gold nanomaterials mediated by high altitude lichens. Mater Letters. 169:58–61.
- Elavazhagan T, Arunachalam KD. 2011. Memecylon edule leaf extract mediated green synthesis of silver and gold nanoparticles. Int J Nanomedicine. 6:1265–1278.
- Geethalakshmi R, Sarada DV. 2012. Gold and silver nanoparticles from Trianthema decandra: synthesis, characterization, and antimicrobial properties. Int J Nanomedicine. 7:5375–5384.
- Jain D, Daima HK, Kachhwala S, Kothari S. 2009. Synthesis of plant-mediated silver nanoparticles using papaya fruit extract and evaluation of their anti microbial activities. Dig J Nanomate Biostruct. 4:557–563.
- Kim JK, Choi SJ, Cho HY, Hwang HJ, Kim YJ, Lim ST, et al. 2010. Protective effects of kaempferol (3,4',5,7-tetrahydroxyflavone) against amyloid beta peptide (Abeta)-induced neurotoxicity in ICR mice. Biosci Biotechnol Biochem. 74:397–401.
- Lin CA, Yang TY, Lee CH, Huang SH, Sperling RA, Zanella M, et al. 2009. Synthesis, characterization, and bioconjugation of fluorescent gold nanoclusters toward biological labeling applications. ACS Nano. 3:395–401.
- MubarakAli D, Thajuddin N, Jeganathan K, Gunasekaran M. 2011. Plant extract mediated synthesis of silver and gold nanoparticles and its antibacterial activity against clinically isolated pathogens. Colloids Surf B Biointerfaces. 85:360–365.
- Naz F, Koul V, Srivastava A, Gupta YK, Dinda AK. 2016. Biokinetics of ultrafine gold nanoparticles (AuNPs) relating to redistribution and urinary excretion: a long-term in vivo study. J Drug Target. 24:720–729.
- Omidi B, Hashemi SJ, Bayat M, Larijani K. 2014. Biosynthesis of Silver Nanoparticles by Lactobacillus fermentum. Bull Env Pharmacol Life Sci. 3:186–192.
- Prabhu S, Poulose EK. 2012. Silver nanoparticles: mechanism of antimicrobial action, synthesis, medical applications, and toxicity effects. Int Nano Lett. 2:32.
- Pyun DG, Yoon HS, Chung HY, Choi HJ, Thambi T, Kim BS, Lee DS. 2015. Evaluation of AgHAP-containing polyurethane foam dressing for wound healing: synthesis, characterization, in vitro and in vivo studies. J Mater Chem B. 3:7752–7763.
- Raveendran P, Fu J, Wallen SL. 2003. Completely "green" synthesis and stabilization of metal nanoparticles. J Am Chem Soc. 125:13940–13941.
- Selvi JA, Rajendran S, Sri VG, Amalraj AJ, Narayanasamy B. 2009. Corrosion inhibition by beet root extract. Portugaliae Electrochimica Acta. 27:1–11.
- Singh P, Kim YJ, Wang C, Mathiyalagan R, EL-Agamy Farh M, Yang DC. 2015a. Biogenic silver and gold nanoparticles synthesized using red ginseng root extract, and their applications. Artif Cells, Nanomed Biotechnol. 44:811–816.
- Singh P, Kim YJ, Wang C, Mathiyalagan R, Yang DC. 2015b. The development of a green approach for the biosynthesis of silver and gold nanoparticles by using Panax ginseng root extract, and their biological applications. Artif Cells, Nanomed Biotechnol. 44:1150–1157.
- Singh P, Singh H, Kim YJ, Mathiyalagan R, Wang C, Yang DC. 2016. Extracellular synthesis of silver and gold nanoparticles by Sporosarcina koreensis DC4 and their biological applications. Enzyme Microb Technol. 86:75–83.
- Veerasamy R, Xin TZ, Gunasagaran S, Xiang TFW, Yang EFC, Jeyakumar N, Dhanaraj SA. 2011. Biosynthesis of silver nanoparticles using mangosteen leaf extract and evaluation of their antimicrobial activities. J Saudi Chem Soc. 15:113–120.
- Wang C, Kim YJ, Singh P, Mathiyalagan R, Jin Y, Yang DC. 2016a. Green synthesis of silver nanoparticles by Bacillus methylotrophicus, and their antimicrobial activity. Artif Cells, Nanomed Biotechnol. 44:1127–1132.
- Wang C, Singh P, Kim YJ, Mathiyalagan R, Myagmariav D, Wang D, Jin CG, Yang DC. 2016b. Characterization and antimicrobial application of biosynthesized gold and silver nanoparticles by using Microbacterium resistens. Artif Cells, Nanomed Biotechnol. 44:1714–1721.
- Wu Q, Yang XW. 2009. The constituents of Cibotium barometz and their permeability in the human Caco-2 monolayer cell model. J Ethnopharmacol. 125:417–422.
- Xu Z, Yan Y, Chen ZD, Chen ZL, Zhang K. 2000. Studies on the chemical constituents of cibotium barometz (II). Pharm J Chin PLA. 16:65–68.
- Yan FX, Zhu BY, Zheng X, Hu JF, Ge XL, Huang HL, Liao DF. 2002. Protective effect of onychin on lipid peroxidation in experimental liver damage of mice. J Nanhua University (Medical Edition). 30:4–6.
- Yuan Z, Yu JT, Su SW. 1999. Determination of the contents of protocatechuic acid and caffeic acid in Rhizoma Cibotii and Rhizoma Pteris by TLCs. J Shenyang Pharm University. 17:338–340.
- Zhang CL, Wang ZX. 2000. Studies on the constituents of Cibotium barometz (L) J. Sm. rhizome. J Chin Med Chem. 11:279–280.
- Zhang J, Zhao CC, Han WN, Liu FZ. 2008. Effects of flavonoids on myocardial apoptosis. Chin Pharm Bull. 24:635–639.
- Zhao X, Wu ZX, Zhang Y, Yan YB, He Q, Cao PC, Lei W. 2011. Anti-osteoporosis activity of Cibotium barometz extract on ovariectomy-induced bone loss in rats. J Ethnopharmacol. 137:1083–1088.
- Xu ZH, YAN Y, Chen ZD, Chen ZL, Zhang K. 2004. Studies on the Chemical Constituents of Cibotium Barometz (III). [J]. J Pharm Chin PLA. 20:337–339.