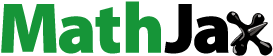
Abstract
Haemoglobin (Hb)-based oxygen carriers are under consideration as oxygen therapeutics. Their effect on apoptosis is critical, because the onset of pro-apoptotic pathways may lead to tissue damage. MP4OX, a polyethylene glycol-conjugated human Hb preserves the baseline level of neuron apoptosis with respect to sham. Here we develop a method for measuring Hb extravasation in brain. We exchange transfused rats by haemorrhaging 50% of their blood with simultaneous, isovolemic replacement with Hextend (negative control), MP4OX, or αα-cross-linked Hb. Animals were sacrificed 2 h after transfusion, brain tissue was harvested and processed for double-staining immunofluorescence, whereby Hb ? chain and NeuN (a neuron protein) were stained and quantitated. Whereas Hextend did not induce Hb extravasation, in both MP4OX and ??Hb brains Hb molecules were detected outside neurons. The level of extravasated Hb chains was > 3-fold higher in Hb compared to MP4OX. Western blot analysis revealed that the expression levels of protein related to redox imbalance (e.g., Nrf2, iNOS and ERK phosphorylation) were higher in ααHb than MP4OX. In conclusions, higher Hb extravasation in ααHb than MP4OX induces redox imbalance, which causes higher anti-oxidant response. Whereas Nrf2 response may be considered protective, iNOS response appears damaging.
Introduction
The search for resuscitation fluids to optimize recovery from hypovolemic shock involves development of crystalloids and hemoglobin (Hb)-based O2 carriers (HBOCs). Clinical results with Hextend, a non-O2 carrying crystalloid plasma expander (Hartog et al. Citation2011), or diaspirin cross-linked Hb (DCLHb) (Sloan et al. Citation1999) have questioned their safety (Mollan and Alayash Citation2013), and the need for improved solutions remains acute. Polyethylene glycol-conjugated human Hb, or MP4OX, a newly developed HBOC, has colloidal, molecular, and O2 transport properties (Svergun et al. Citation2008, Vandegriff et al. Citation2003, Vandegriff et al. Citation2004) particularly suitable for further experimentation. In the brain of rats exchange transfused with two different HBOCs (MP4OX vs. ααHb), the interaction of MP4OX with certain cellular signaling pathways reduced markedly neuron apoptosis compared to ααHb (Vandegriff et al. Citation2014). These results are despite of the high O2 affinity and presumably lowered O2 delivery by MP4OX (Samaja and Terraneo Citation2011). In fact, high O2 affinity of cell-free HBOCs has been demonstrated to be critical to prevent hyperoxygenation and vasoconstriction in pre-capillary arterioles (Cole et al. Citation2008, Tsai et al. Citation2003).
It is now evident that, in addition to its high O2 affinity, other physical and chemical properties of MP4OX, such as molecular radius (Cole et al. Citation2008, Svergun et al. Citation2008), and differences in oxidative potential (Vandegriff et al. Citation2006), may be key to understand the protective effects of this molecule. In this study, we tested whether the different degree in neuroprotection observed with MP4OX and ααHb is related to the extent of Hb extravasation into brain tissue. Because the oxidative potential may be linked to the ability of the Hb molecule to stay in the circulation (Baldwin Citation2004), we developed a novel technology to monitor Hb extravasation into the extracellular space. From these studies, we conclude that the cell responses to redox imbalance, which may trigger either protective or damaging pathways in relation to their nature, is strictly dependent on the degree of extravasation of Hb molecules, thereby emphasizing the ability of Hb to remain in the circulation as a primary target for therapeutic use of HBOCs.
Materials and methods
Animals
Sprague-Dawley rats, aged 14 weeks and weighing (perhaps) 311 ± 17 g were used. Animals were cared for in accordance to the Guide for the Care and Use of Laboratory Animals published by the National Institutes of Health (NIH Publication No. 85–23, revised 1996). Rats had free access to water and conventional laboratory diet until 24 h before sacrifice. A 12/12 h light/dark cycle was maintained.
Hemorrhage-hemodilution protocol
For the hemodilution experiments, rats were divided into four groups: Hextend, MP4OX, ααHb, and non-treated shams. Rats were hemorrhaged, withdrawing 50% of their blood volume (32 mL/kg) while simultaneously transfusing with an equal volume of Hextend, MP4OX or ααHb. The Hb concentrations of MP4OX and ααHb were matched at 4 g/dL to evaluate the cellular mechanisms without the variable of different Hb concentrations. Sham-operated animals served as control and underwent only surgery. Animals were euthanized at t = 2 h after exchange transfusion (n = 4–6 per group). The whole brain was flash frozen immediately in liquid nitrogen within 2.5 min from the time of sacrifice for subsequent analysis.
Measurement of extravasated Hb
Part of the frozen biopsies was embedded in Optimum Cutting Temperature Compound (Leica Instruments, Nussloch, Germany); serial 5-μm thick sections were obtained in a cryomicrotome (Leica CM1510) and placed on Superfrost Plus glass slides (Menzel Gmbh, Braunschweig, Germany). Two distinct sections were obtained from each biopsy. Care was taken to analyze the same brain area (cerebral cortex) in all sections. The sections were dried 2 min at room temperature, fixed in 4% buffered formalin (45 min), rinsed 3 times in PBS (each for 5 min), post-fixed with ethanol-acetic acid 2:1 (vol:vol) (5 min at −20 °C), rinsed twice in PBS (each for 5 min), boiled in 10 mM citrate at pH 6.0 (10 min), washed 3 times in PBS for 5 min each, then incubated with 0.5% Triton-X for 5 min and washed twice in PBS. After treatment with 10% normal goat serum + 0.1% Tween20 for 1 h with gentle agitation, the sections were incubated overnight at 4 °C with a rabbit anti-Hb α polyclonal antibody (Santa Cruz Biotechnology, Santa Cruz, CA), diluted 1:100 in 1.5% normal goat serum + 0.05% Tween20, washed in PBS and treated at room temperature for 45 min with a goat anti rabbit IgG rhodamine-conjugated secondary antibody (Santa Cruz Biotechnology) diluted 1:180 in 1.5% normal goat serum + 0.05% Tween20 and rinsed in PBS three times. The sections were double stained for 90 min with a mouse anti neuron-specific protein NeuN monoclonal antibody (Millipore, Temecula, CA), diluted 1:100 in 1.5% normal goat serum + 0.05% Tween20, rinsed three times in PBS and treated for 1 h with a goat anti mouse IgG fluorescein-conjugate secondary antibody, diluted 1:180 in 1.5% normal goat serum + 0.05% Tween20 and rinsed three times in PBS. A negative control was prepared for each section by replacing the primary antibody with 1.5% normal goat serum + 0.05% Tween20. Slides were mounted in a 9:1 glycerol/PBS medium, pH 8.5, containing 0.1% p-phenylenediamine as anti-quenching agent. The slides were examined at a magnification of 40X in an inverted fluorescence microscope (Axiovert 25 CFL; Zeiss, Göttingen, Germany), equipped with a filter for the detection of either rhodamine (filter set 15, excitation bandpass 546 ± 12 nm, emission low-pass 590 nm) or fluorescein (filter set 9, excitation bandpass 450–490 nm, and emission low-pass 515 nm). Five distinct images per section were acquired by a digital camera system for microscopy (DS-5M; Nikon, Tokyo, Japan) and stored in a computer.
For the quantification of the Hb signal, each image was analyzed by IPlab Software (Scanalytics, Fairfax, VA) and split into RGB channels. The red channel was used to calculate the color intensity as the sum of the pixel intensity values. Five random fields per section were selected for each slide; the red color intensity was averaged and subtracted of the signal detected in the negative controls. Hb extravasation was expressed as the sum of the red pixel intensity × 106/0.037 mm².
Cell signaling
Another part of the tissue biopsies was run for Western blots of the cytosolic extracts to assess certain cell signaling paths. To obtain the cytosolic extracts, frozen tissue (50–80 mg) was homogenized in a glass potter in a 1:3 ratio (w:v) with 10 mM HEPES, 1.5 mM MgCl2, 0.5 mM DTT, 0.2 mM PMSF, 10 mM KCl, and 10% Protease Inhibitor Cocktail (Complete Protease Inhibitor Cocktail Tables, EDTA-free, Roche Diagnostics GmbH, Mannheim, Germany), pH 7.9. The homogenate was centrifuged for 20 min at 14,000 rpm, the pellet was resuspended and centrifuged again for 10 min at 14,000 rpm. The cytosolic extract was obtained by pooling the supernatant fractions from both centrifugations. Given the large number of samples to be compared, a reference extract subdivided into several aliquots, frozen at −20 °C, thawed only once and run in duplicate in every blot run.
Extracts were separated by SDS-PAGE, 8% acrylamide gels. The protein concentration was measured by the Coomassie Plus Protein Assay reagent Kit (Pierce, Rockford, IL) and 50–70 μg protein was loaded per each lane. After separation, proteins were blotted onto a nitrocellulose membrane (Amersham Pharmacia Biotech., Little Chalfont, Buckinghamshire, UK) and blocked with 5% nonfat dry milk in TRIS-buffered saline containing 0.1% Tween (1 h, room temperature). Membranes were incubated overnight at 4 °C with the primary antibody, followed by incubation with horseradish peroxidase-conjugated secondary antibody (1 h, room temperature). The following primary antibodies were used: β-actin (Clone AC-74, Sigma Chemicals, mouse, 1:10,000), Nrf-2 (C20: sc-722, Santa Cruz Biotechnology, rabbit, 1:1000), iNOS (N20: sc651, Santa Cruz Biotechnology), ERK1 (C-16: Santa Cruz Biotechnology), and p-ERK (E-4: sc7383, Santa Cruz Biotechnology).
The secondary antibodies used for Western blot experiments were: peroxidase-conjugated AffiniPure Goat Anti-Rabbit IgG, code number: 111–035-003, lot number: 95,093/Jackson Immunoresearch, 1:10,000.
Chemiluminescence was detected by incubating the membrane with LiteAblot Chemiluminescent substrate (Lite Ablot, EuroClone, EMPO10004) followed by x-ray film exposure (Kodak X-Omat Blue XB-1 Film, Eastman Kodak Company, Rochester, NY). The resulting image was acquired and quantified by Gel Doc (Bio-Rad quantitation software Quantity One; Hercules, CA). After subtracting the background signal, densitometry data were normalized with respect to the reference extract, which represented a positive control to encompass intra- and inter-run variability due to factors, such as variations in antibodies concentrations, varying exposure times, etc. Protein levels are expressed as densitometry ratio with β-actin unless otherwise stated.
Results
Hb extravasation
To assess Hb extravasation in the extracellular space of brain tissue, we used a double-staining immunofluorescence procedure, whereby antibodies against the α chain of Hb and against NeuN (a neuron protein) were stained, respectively, by a red (rhodamine) and a green (fluorescein) dye. The latter was subsequently digitally converted into blue to favor visibility. The top panel in reports representative images of brain tissue from the three groups under study, taken 2 h following exchange transfusion. In shams and in Hextend-treated animals, the blue staining of neurons was free of red staining, indicating the absence of Hb α chains. In contrast, the red signal was observable in both MP4OX and ααHb brains. In both cases, lack of overlapping between the blue and red signals indicates that extravasated Hb molecules were outside of neurons. The level of Hb α chains staining was quantified (, bottom panel) from the sum of red pixels in all images. As expected, Hextend showed minimal α-globin staining (P = NS from sham). In contrast, ααHb-exposed brain tissue showed ∼3-fold higher staining (i.e., amount of extravasation) compared to MP4OX, Hextend and shams (P = 0.01, unpaired two-tailed Student t-test). Thus, even though MP4OX, like ααHb, is a freely circulating cell-free Hb, no difference was detected between MP4OX vs. shams (P = NS).
Figure 1. Hb extravasation. The top panel reports representative images from Hextend, MP4OX, ααHb-treated rats sacrificed at 2 h after exchange transfusion. Extravasated Hb was reacted with anti-Hb α chain and counterstained with rhodamine-labeled antibody (red). Neurons were stained with the anti-NeuN antibody and counterstained with fluorescein-labeled antibody, which was converted into blue to favor visibility. Lack of overlapping between the blue and red signals indicates that virtually all Hb α chains were outside the neurons. The bottom panel reports the red pixel intensity count obtained in five images/slide, two slides/sample, and 4–6 samples/group (n = 40–60/samples/group). The bars represent mean ± SD, ** P = 0.01 unpaired two-tailed Student t-test.
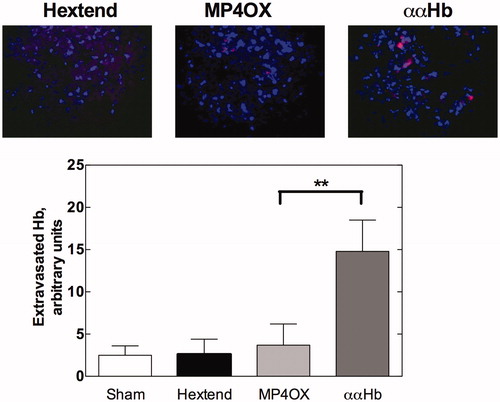
Signaling paths
The expression of Nrf2 and iNOS was higher in ααHb than in MP4OX-treated rat brain (, P = 0.05, unpaired two-tailed Student t-test). By contrast, the phosphorylation of ERK, assessed by the p-ERK/ERK ratio, was non-significantly different (P = 0.2) in ααHb vs. MP4OX ().
Figure 2. Expression of Nrf2, iNOS, and the phosphorylated ERK/total ERK ratio (from top to bottom). Nrf2 and iNOS are expressed in relation to β-actin as load control. The bars represent the average density (mean ± SD) of Western blots obtained using the primary antibody against the indicated proteins, and a secondary labeled antibody. *P = 0.05, unpaired two-tailed Student t-test.
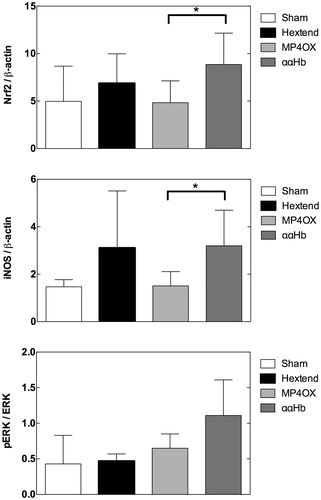
Discussion
Prevailing theories about cell-free Hb-induced toxicity involve the notion that Hb molecules come into close contact with the endothelium (Lancaster Citation1994) or extravasate across the endothelium barrier (Bucci et al. Citation2006). In this report, we measured, for the first time, HBOC extravasation in rat brain tissue. Since MP4OX molecules extravasate markedly less than ααHb molecules, this feature may be linked to the protective effects of MP4OX that are not evident with other HBOC types that may or may not be associated with O2 delivery and/or other differential molecular effects. This result further supports MP4OX as an affordable target for hemorrhagic shock and a variety of diseases.
Hb extravasation
To estimate Hb extravasation, we measured the anti-Hb α chain immunoreactivity in the extracellular space, which was greatly increased in ααHb with respect to MP4OX. The extent of the extravasation was constant over time after exchange transfusion up to 12 h (not shown), suggestive of early Hb extravasation of ααHb within 2 h of exchange transfusion. The slight immunoreactivity in shams and Hextend was attributed to cross-reactivity with neuron Hb (neuroglobin), an intracellular O2 storage specifically expressed in neurons (Schelshorn et al. Citation2009). By this method, it appears that the degree of Hb extravasation is greatly increased with ααHb, with respect to MP4OX. Further, the potential appearance of extravasated MP4OX by anti-Hb α chain immunoreactivity was not different from Hextend, suggesting either a very low degree of MP4OX extravasation or also simply background signal of neuroglobin as postulated with Hextend and shams (). Since ααHb is covalently cross-linked between α chains, ααHb does not dissociate into Hb dimers; thus, at least in the case of ααHb, the α-globin staining most likely represents intact ααHb tetramers.
The increased size (Baldwin Citation1999) and/or the presence of PEG molecules conjugated to the Hb molecule (Svergun et al. Citation2008) may have important impact on the extent of Hb extravasation. PEGylation has been shown to increase the dimensions of the Hb molecule. The highly flexible and hydrophilic nature of PEG-polymer-conjugates impedes crystallization of the Hb protein, and thus atomic-resolution structural dimensions could not be determined using X-ray crystallography. Instead, lower-resolution dimensions and shapes of Hb vs. MP4OX were determined using small angle X-ray scattering (SAXS) (Svergun et al. Citation2008). That study showed that PEGylation increases the maximum particle diameter of Hb from 70 ± 5 to 130 ± 10 Å for MP4OX. The non-PEGylated Hb used for SAXS studies was not ααHb, but the structure determined for the native Hb molecule matched that of crystallized Hb tetramers and thus should depict the size of the crosslinked ααHb tetramer. (In a separate study, we showed that the radius of gyration of ααHb matched that of unmodified Hb as well (Vandegriff et al. Citation1997)).
The SAXS results also demonstrated that surface shielding provided by PEGylation resulted in a high degree of intermolecular repulsion (Svergun et al. Citation2008). It was theorized that such PEG-induced intermolecular repulsion could significantly reduce the interaction of MP4OX with the surface glycocalyx of endothelial cells, which could contribute to the prolongation of intravascular retention (Svergun et al. Citation2008).
These results are consistent with the longer circulatory half-life of MP4OX, ∼12 h in rats (Vandegriff et al. Citation2006) compared to ∼5 h for ααHb (Keipert et al. Citation1994). These results are also consistent with the extravasation measurements described in the present report and with the lower degree of apoptosis reported in rat brain (Vandegriff et al. Citation2014). Molecular size alone cannot completely differentiate these results. For example, another study using a cell-free polymerized Hb similar in molecular size to MP4OX but with lower O2 affinity compared to either ααHb or MP4OX (Cole et al. Citation2008), showed compromised endothelial junctions in the blood-brain barrier and Hb extravasation that led to oxidative stress in brain (Butt et al. Citation2011). Interestingly, when a high-molecular weight polyhemoglobin cross-linked with superoxide dismutase and catalase was used in a model of transient global cerebral ischemia, the improved redox balance due to the concurrent presence of antioxidant enzymes considerably reduced damage to the blood-brain barrier to a much greater extent than polyhemoglobin alone even without appreciable changes in the Hb-O2 affinity (Powanda and Chang Citation2002). Thus, beyond Hb molecular size alone, oxygen affinity, and/or molecular repulsion of endothelial glycocalyx with PEGylated Hbs remain pertinent areas of HBOC research.
Anti-oxidant responses
While the mechanism(s) for the redox imbalance differences between MP4OX and ααHb have not been fully defined, extravasated Hb is expected to release iron and increment the redox imbalance via the Fenton reaction (Misra and Fridovich Citation1972):
(1)
(1)
As shown in reaction (1), Hb(Fe²+) reacts with H2O2, which may then release free oxidized iron (Fe³+). The oxidation of ααHb collapses the haem into a highly oxidizing rhombic geometric formation (Nagababu et al. Citation2002). The redox behaviors of MP4OX vs. ααHb are currently under investigation.
Hb extravasation is expected to result into increased redox imbalance due to the oxidant activity of Hb molecules (Baldwin Citation2004), and the greater extravasation of ααHb reported here is reflected by cell signaling mechanisms resulting in, for one, greater expression of the transcription factor Nrf2. Nrf2 represents the primary defense against redox imbalance (Shah et al. Citation2007) and intracerebral hemorrhage (Zhao et al. Citation2007). It mediates several adaptive pathways that include antioxidant enzymes (Sykiotis et al. Citation2011), HIF-1α accumulation (Kim et al. Citation2011), up-regulation of the HO-1 expression with reduction of the nuclear localization of CHOP (Ryter et al. Citation2006), a protein marker for autophagy and in general for the cell defense against stress, all activities that release carbon monoxide and raise expression of anti-apoptotic molecules (Stein et al. Citation2012).
One specific example is that Nrf2 induces transcription of the cytoprotective enzyme thioredoxin 1 (Trx1). Under non-stimulated conditions, cytosolic Nrf2 is bound to Keap1, an adaptor of the Cul3-based E3 ligase that mediates ubiquitination and proteasomal degradation of Nrf2. Thus, Keap1 acts as a sensor molecule for oxidative stress, under which the interaction between Keap1 and Nrf2 is disrupted. This results in the release of Nrf2, which translocates to the nucleus and induces target genes that contain an anti-oxidative-responsive element (ARE) in their regulatory DNA regions. Nrf2 is also known to detoxify the intracellular environment (Shelton and Jaiswal Citation2013) and to allow cells to escape from death (Mitsuishi et al. Citation2012). The connection between apoptosis (Vandegriff et al. Citation2014) and Hb extravasation is still to be determined, but the major injurious path recruited by ααHb is likely through Hb extravasation, which leads to greater redox imbalance and greater anti-oxidant response. This is consistent with data obtained using a cell-free polymerized Hb solution that showed compromised endothelial junctions in the blood-brain barrier and Hb extravasation that led to oxidative stress in brain (Butt et al. Citation2011). In further support to this conjecture, when another type of polyhemoglobin, i.e., bovine Hb cross-linked with superoxide dismutase and catalase, was injected during the reperfusion of cerebral tissue challenged with transient global ischemia, the blood-brain barrier appeared less vulnerable in contrast to solutions containing polyhemoglobin alone (Powanda and Chang Citation2002).
Another response to redox imbalance is represented by overexpression of calcium-insensitive iNOS, which produces the largest amounts of NO that may cause septic shock. iNOS produces large quantities of NO upon stimulation, such as by proinflammatory cytokines (Hall and Attwell Citation2008). Induction of the high-output iNOS usually occurs in an oxidative environment, and thus high levels of NO have the opportunity to react with superoxide leading to peroxynitrite formation and cell toxicity (Moro et al. Citation2004). The observation that ααHb induces the largest expression in iNOS is consistent with the hypothesis that the more severe redox imbalance due to greater Hb extravasation mediates larger ROS damage due to excess NO production with injurious effects. Additionally, the higher iNOS reported in Hextend-treated animals () may have contributed to our earlier report on degrees of apoptosis such that MP4OX < Hextend < ααHb rat brain (Vandegriff et al. Citation2014).
Finally, extracellular-signal-regulated kinases (ERKs) are classically involved in directing cellular responses to a diverse array of stimuli, such as mitogens, osmotic stress, heat-shock, and proinflammatory cytokines (Leevers and Marshall Citation1992). Their higher activation (i.e., phosphorylation) in ααHb-treated rat brain (although not statistically different from MP4OX) is a further indication of greater extracellular stimulation in this group.
The scheme in summarizes the findings described here and depicts the predicted secondary effects due to increased Hb-induced oxidant stress as the result of greater Hb extravasation and/or excess ROS. Whereas Nrf2 response may be considered protective, iNOS response may appear damaging. The end-effect of ERK activation is presently under consideration.
Figure 3. Scheme depicting the downstream effects observed in rats exchange-transfused with either MP4OX (low-Hb extravasation) or ααHb (high-Hb extravasation). Filled upwards arrows on the left and empty upwards arrows on the right indicate the effects driven by low and high Hb extravasation, respectively. ▪ indicates no response. Hb extravasation imbalances the redox status to a larger extent upon exchange-transfusion with ααHb than MP4OX, likely due to the Fenton reaction. The examined adaptive responses follow three arms. Antioxidant Nrf2, finally leading to protection, responds to a larger extent to ααHb than MP4OX. Pro-oxidant iNOS, main producer of NO, and major contributor to damaging oxidative stress, is also higher in ααHb than MP4OX. Likewise ERK1/2 is higher in ααHb than MP4OX, but the effects driven by this protein are controversial. As a final outcome, the redox imbalance appears shifted toward protection in MP4OX, and toward damage in ααHb as a direct consequence of Hb extravasation.
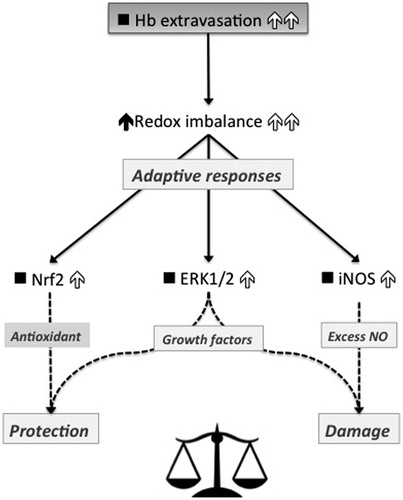
Conclusion
MP4OX is fundamentally different from other HBOCs because its relatively high O2 affinity helps targeting O2 delivery to capillaries. Furthermore, its ability to regenerate NO through enhanced nitrite reductase activity prevents vasoconstriction, concomitantly with NO release in vitro, which is under translational investigation as a mechanism to counteract Hb-NO scavenging in vivo (Olsen et al. Citation2012). As a result, MP4OX has been shown to be effective in O2 delivery, reversal of O2 debt and lactic acidosis, intravascular volume expansion and hemodynamic stabilization, compared with crystalloids, and other colloids (Tsai et al. Citation2003, Young et al. Citation2005, Young et al. Citation2007). Here, we show that an additional interesting feature is represented by reduced rate of extravasation, which reduces the redox imbalance and consequently the anti-oxidant response.
Disclosure statement
AM, GM, SNS, JL, and KDV were paid employees of Sangart Inc. during the course of this research. However, Sangart is no longer a business entity. The other authors declare that they have no conflicts of interest relevant to the manuscript submitted.
Additional information
Funding
References
- Baldwin AL. 1999. Modified hemoglobins produce venular interendothelial gaps and albumin leakage in the rat mesentery. Am J Physiol. 277:H650–H659.
- Baldwin AL. 2004. Blood substitutes and redox responses in the microcirculation. Antioxid Redox Signal. 6:1019–1030.
- Bucci E, Matheson B, Kwansa H, Koehler RC. 2006. Development of non-extravasating hemoglobin-based oxygen carriers. In: Winslow R, Ed. Blood Substitutes. London: Academic Press, pp. 488–497.
- Butt OI, Buehler PW, D’Agnillo F. 2011. Blood-brain barrier disruption and oxidative stress in guinea pig after systemic exposure to modified cell-free hemoglobin. Am J Pathol. 178:1316–1328.
- Cole R, Vandegriff K, Szeri A, Savas O, Winslow R. 2008. Targeted O2 delivery by blood substitutes: in vitro arteriolar simulations of first- and second-generation products. Microvasc Res. 76:169–179.
- Hall CN, Attwell D. 2008. Assessing the physiological concentration and targets of nitric oxide in brain tissue. J Physiol (Lond.). 586:3597–3615.
- Hartog CS, Kohl M, Reinhart K. 2011. A systematic review of third-generation hydroxyethyl starch (HES 130/0.4) in resuscitation: safety not adequately addressed. Anesth Analg. 112:635–645.
- Keipert PE, Gomez CL, Gonzales A, MacDonald VW, Hess JR, Winslow RM. 1994. Diaspirin cross-linked hemoglobin: tissue distribution and long-term excretion after exchange transfusion. J Lab Clin Med. 123:701–711.
- Kim TH, Hur EG, Kang SJ, Kim JA, Thapa D, Lee YM, et al. 2011. NRF2 blockade suppresses colon tumor angiogenesis by inhibiting hypoxia-induced activation of HIF-1alpha. Cancer Res. 71:2260–2275.
- Lancaster JR, Jr. 1994. Simulation of the diffusion and reaction of endogenously produced nitric oxide. Proc Natl Acad Sci USA. 91:8137–8141.
- Leevers SJ, Marshall CJ. 1992. Activation of extracellular signal-regulated kinase, ERK2, by p21ras oncoprotein. EMBO J. 11:569–574.
- Misra HP, Fridovich I. 1972. The generation of superoxide radical during the autoxidation of hemoglobin. J Biol Chem. 247:6960–6962.
- Mitsuishi Y, Motohashi H, Yamamoto M. 2012. The Keap1-Nrf2 system in cancers: stress response and anabolic metabolism. Front Oncol. 2:200.
- Mollan TL, Alayash AI. 2013. Redox reactions of hemoglobin: mechanisms of toxicity and control. Antioxid Redox Signal. 18:2251–2253.
- Moro MA, Cardenas A, Hurtado O, Leza JC, Lizasoain I. 2004. Role of nitric oxide after brain ischaemia. Cell Calcium. 36:265–275.
- Nagababu E, Ramasamy S, Rifkind JM, Jia Y, Alayash AI. 2002. Site-specific cross-linking of human and bovine hemoglobins differentially alters oxygen binding and redox side reactions producing rhombic heme and heme degradation. Biochemistry. 41:7407–7415.
- Olsen S, Malavalli A, Vandegriff K. 2012. Correlation between hemoglobin oxygen affinity and nitrite reductase activity. FASEB J. 26:573–574.
- Powanda DD, Chang TM. 2002. Cross-linked polyhemoglobin-superoxide dismutase-catalase supplies oxygen without causing blood-brain barrier disruption or brain edema in a rat model of transient global brain ischemia-reperfusion. Artif Cells Blood Substit Immobil Biotechnol. 30:23–37.
- Ryter SW, Alam J, Choi AM. 2006. Heme oxygenase-1/carbon monoxide: from basic science to therapeutic applications. Physiol Rev. 86:583–650.
- Samaja M, Terraneo L. 2011. Impact of hemoglobin concentration and affinity for oxygen on tissue oxygenation: the case of hemoglobin-based oxygen carriers. Artif Organs. 36:210–223.
- Schelshorn DW, Schneider A, Kuschinsky W, Weber D, Kruger C, Dittgen T, et al. 2009. Expression of hemoglobin in rodent neurons. J Cereb Blood Flow Metab. 29:585–595.
- Shah ZA, Li RC, Thimmulappa RK, Kensler TW, Yamamoto M, Biswal S, Dore S. 2007. Role of reactive oxygen species in modulation of Nrf2 following ischemic reperfusion injury. Neuroscience. 147:53–59.
- Shelton P, Jaiswal AK. 2013. The transcription factor NF-E2-related factor 2 (Nrf2): a protooncogene? FASEB J. 27:414–423.
- Sloan EP, Koenigsberg M, Gens D, Cipolle M, Runge J, Mallory MN, Rodman G, Jr. 1999. Diaspirin cross-linked hemoglobin (DCLHb) in the treatment of severe traumatic hemorrhagic shock: a randomized controlled efficacy trial. JAMA. 282:1857–1864.
- Stein AB, Bolli R, Dawn B, Sanganalmath SK, Zhu Y, Wang OL, et al. 2012. Carbon monoxide induces a late preconditioning-mimetic cardioprotective and antiapoptotic milieu in the myocardium. J Mol Cell Cardiol. 52:228–236.
- Svergun DI, Ekström F, Vandegriff KD, Malavalli A, Baker DA, Nilsson C, Winslow RM. 2008. Solution structure of poly(ethylene) glycol-conjugated hemoglobin revealed by small-angle X-ray scattering: implications for a new oxygen therapeutic. Biophys J. 94:173–181.
- Sykiotis GP, Habeos IG, Samuelson AV, Bohmann D. 2011. The role of the antioxidant and longevity-promoting Nrf2 pathway in metabolic regulation. Curr Opin Clin Nutr Metab Care. 14:41–48.
- Tsai AG, Vandegriff KD, Intaglietta M, Winslow RM. 2003. Targeted O2 delivery by low-P50 hemoglobin: a new basis for O2 therapeutics. Am J Physiol Heart Circ Physiol. 285:H1411–H1419.
- Vandegriff KD, Bellelli A, Samaja M, Malavalli A, Brunori M, Winslow RM. 2004. Kinetics of NO and O2 binding to a maleimide poly(ethylene glycol)-conjugated human haemoglobin. Biochem J. 382:183–189.
- Vandegriff KD, Malavalli A, Lohman J, Young MA, Terraneo L, Virgili E, et al. 2014. Impact of acellular hemoglobin-based oxygen carriers on brain apoptosis in rats. Transfusion. 54:2045–2054.
- Vandegriff KD, Malavalli A, Minn C, Jiang E, Lohman J, Young MA, Samaja M, Winslow RM. 2006. Oxidation and haem loss kinetics of poly(ethylene glycol)-conjugated haemoglobin (MP4): dissociation between in vitro and in vivo oxidation rates. Biochem J. 399:463–471.
- Vandegriff KD, Malavalli A, Wooldridge J, Lohman J, Winslow RM. 2003. MP4, a new nonvasoactive PEG-Hb conjugate. Transfusion. 43:509–516.
- Vandegriff KD, McCarthy M, Rohlfs RJ, Winslow RM. 1997. Colloid osmotic properties of modified hemoglobins: chemically cross-linked versus polyethylene glycol surface-conjugated. Biophys Chem. 69:23–30.
- Young MA, Lohman J, Malavalli A, Vandegriff KD, Winslow RM. 2009. Hemospan improves outcome in a model of perioperative hemodilution and blood loss in the rat: comparison with hydroxyethyl starch. J Cardiothorac Vasc Anesth. 23:339–347.
- Young MA, Riddez L, Kjellström BT, Bursell J, Winslow F, Lohman J, Winslow RM. 2005. MalPEG-hemoglobin (MP4) improves hemodynamics, acid-base status, and survival after uncontrolled hemorrhage in anesthetized swine. Crit Care Med. 33:1794–1804.
- Young MA, Riddez L, Kjellström BT, Winslow RM. 2007. Effect of maleimide-polyethylene glycol hemoglobin (MP4) on hemodynamics and acid-base status after uncontrolled hemorrhage in anesthetized swine: comparison with crystalloid and blood. J Trauma. 63:1234–1244.
- Zhao X, Sun G, Zhang J, Strong R, Dash PK, Kan YW, Grotta JC, Aronowski J. 2007. Transcription factor Nrf2 protects the brain from damage produced by intracerebral hemorrhage. Stroke. 38:3280–3286.