Abstract
Background: Prior to heart transplantation, static storage of donor hearts is currently limited to 4–5 h, despite profound hypothermia (4–8 °C). Because heart transplantation is an emergency procedure, improved protection to extend safe storage duration would be advantageous. We investigated whether the naturally respiratory pigment HEMO2life®, which is effective at hypothermia for the passive release of oxygen via oxygen gradient, could improve long-term preservation.
Methods: Isolated Langendorff-perfused rat hearts (n = 12/group) were equilibrated (20 min) and function (left ventricular developed pressure: LVDP) measured by intraventricular balloon before arrest with cold (7.5 °C) Celsior® solution, either alone (control) or with the addition of HEMO2life® (Hemarina SA, Morlaix, France) at 1 g/L. Cold storage lasted 8 h prior to reperfusion (60 min) and recovery (as % of pre-ischemic function) was assessed. Hearts (minced and homogenized) were also assessed by TTC staining as a measure of viability and two hearts from each group were sliced and assessed by TTC staining for infarct size. Values are expressed as mean ± standard error of mean and analyzed by Student’s t-test.
Results: Hearts recovered rapidly in both groups to a plateau by 20 min of reperfusion; control and HEMO2life® final recovery (60 min) was 45 ± 2% and 57 ± 1% (P < 0.05) respectively. Left ventricular end-diastolic pressure recovered to a similar extent in both groups (between 31 to 35 mmHg), as did heart rate (final recovery between 84 to 89% pre-ischemic value); however, coronary flow was significantly (P < 0.05) higher in HEMO2life® group (7.5 ± 0.7 ml/min) compared to control (5.4 ± 0.4 ml/min). Viability and infarct size measurements were similar between groups.
Conclusion: The addition of the natural oxygen releasing pigment HEMO2life® to Celsior® preservation solution significantly improved post-ischemic recovery of heart function. This additive may have major therapeutic potential for clinical heart transplantation.
Introduction
Currently, static storage of donor hearts prior to heart transplantation is limited to ∼4–5 h. A number of solutions, such as the St Thomas’ Hospital solution, Custodiol® (HTK), Viaspan® (UW) and Celsior®, are used clinically for long-term preservation storage of donor hearts, with each having their protagonists among transplant surgeons. All these solutions are used with profound hypothermia (between 4 and 8 °C) and act by rapidly arresting the heart, slowing metabolism and maintaining levels of high-energy phosphate compounds within the myocardium prior to reperfusion. These solutions have also been used for preservation of other organs, such as kidney, liver and spleen, where longer static storage durations, of up to 24–48 h, are common (probably because the requirement to function almost optimally very rapidly is less important). The relatively short storage duration for hearts (with a maximum of 4–6 h) have meant that heart transplantation is more of an emergency procedure. There is some evidence that constant perfusion of hearts during storage improves preservation, but this remains fraught with alternative problems associated with development of edema or hemolysis during blood perfusion.
We have conducted studies investigating the concept of using “polarized” arrest, induced by sodium-channel blockade. These studies (Snabaitis et al. Citation1997, Citation1999a, Citation1999b) conducted in the isolated “working” heart preparation, have shown that it is possible to extend the static storage duration to ∼8 h with good recovery of function. There have been many studies comparing various storage solutions; a new “polarizing” solution [adenosine–lidocaine (Adenocaine) solution] developed by Rudd and Dobson (Citation2009) showed improved post-storage recovery to Celsior® after 6 h of storage. In vivo heart transplantation studies using hearts stored for 6 or 18 h prior to heterotopic transplantation (Lee et al. Citation2010, Citation2011) showed superior protection with HTK solution compared with Celsior®. In contrast, Celsior® was shown to be associated with improved post-transplant recovery (cardiac output) together with lower vasculopathy and chronic rejection than in hearts preserved with Custodiol or Viaspan (Garlicki, Citation2003). This was supported by a porcine study demonstrating reduced endothelial dysfunction when compared to crystalloid or blood cardioplegia (Perrault et al. Citation2005), indicating the possibility of reduced vasculopathy and rejection using Celsior® storage.
Recently, a natural biopolymer, known as M101 and derived from a lugworm (Arenicola marina) has been developed by HEMARINA SA (Morlaix, France) and formulated as a manufactured product designated as HEMO2life®. M101 is a respiratory pigment that acts as a giant oxygen carrier (3600 KDa) corresponding to extracellular hexagonal-bilayer hemoglobin. M101 is composed of 156 globins and 44 non-globin linker chains and presents a large oxygen binding capacity, carrying up to 156 O2 molecules when saturated. M101 acts to release oxygen molecules passively in an oxygen gradient without requiring any allosteric effector, providing the environment with the right amount of O2. It has intrinsic Cu/Zn-superoxide dismutase (SOD) activity preventing both the occurrence of potentially harmful heme–protein-associated free radical species and the release of Hb degradation products that could induce endothelial and surrounding tissue damages and acts effectively at hypothermic temperatures. M101 is a non-glycosylated protein composed of globin chains with a high homology with vertebrate globin that could explain its not immunogenic and allergenic responses upon i.v. injection in BP/2 strain mice which are well known to be hyper-responsive and easily produce antibodies (Rousselot et al. Citation2006).
Recent studies (Thuillier et al. Citation2011) have shown that the addition of HEMO2life® to either UW solution or HTK (the most commonly used solutions for prolonged static storage of kidneys prior to transplantation) significantly improves early graft survival and recovery compared to these solutions alone; at 3 months, these attributes were still evident. Based on this evidence, we hypothesized that the addition of HEMO2life® to Celsior® solution would enhance its efficacy during long-term preservation of the heart.
Methods
Isolated Langendorff rat heart perfusion
Adult male Wistar rats (250–300 g body weight) were used. Rats were anesthetised with 0.8–1.0 mL of 30% phenobarbitone (diluted in 0.9% saline) intraperitoneally, and heparinised with 0.1 mL of 1000 U/mL of heparin injected into the femoral vein. Following a clamshell incision across the chest and division of the diaphragm to expose the mediastinum, hearts were rapidly excised and immersed in cold Kreb–Henseleit solution. The aorta was then cannulated and secured using 3/0 silk suture within 30 s of excision, and immediately perfused retrogradely with normothermic (37 °C) Kreb–Henseleit bicarbonate buffer solution (composition in mM: NaCl, 118.5; NaHCO3, 25.0; KCl, 4.75; MgSO4, 1.19; KH2PO4, 1.18; Glucose, 11.0 and CaCl2 1.4) at 100 cm H2O at that was continually gassed with a mixture of 95% O2:5% CO2. The cannulated heart was also immersed in warm perfusion solution to maintain the temperature at 37 °C.
An incision was made to the left atrium and an ultrathin intraventricular water-filled balloon (made from clingfilm to fit the left ventricle) was inserted through the mitral valve into the left ventricle. The balloon was connected to a pressure transducer, which allows real-time measurement of the mechanical function of the heart, and was inflated to give stable left ventricular end-diastolic pressures (LVEDP) of 5–10 mmHg. The heart rate (beats/min), left ventricular systolic pressure (LVSP: mmHg) and LVEDP (mmHg) was monitored and acquired continuously using PowerLab (ADInstruments, Oxford, UK) and stored using LabChart 7 (ADInstruments), and coronary flow (CF) (mL/min) was also measured. The left ventricular developed pressure (LVDP) was determined by LVSP minus LVEDP. The heart was allowed to stabilise for 20 min, when control pre-ischemic function was measured, before starting the experimental protocol. Exclusion criteria were applied during this equilibration period; any hearts that did not achieve a LVDP of ≥100 mmHg, or if the CF was ≥20 mL/min (implying a leak from the cannulation) or ≤6 mL/min (implying a perfusion problem) were excluded.
A second reservoir (cooled to maintain the temperature between 4 and 8 °C) attached to a side-arm of the perfusion cannula was used to infuse the cardioplegic solutions.
Perfusion protocol
Hearts were randomised to two groups: (C) Celsior® solution alone or (C + M101) Celsior® solution with the addition of 1 g/L HEMO2life® (12 hearts per group). All hearts were arrested with a 2 min infusion of either Celsior® alone, or Celsior® plus 1 g/L HEMO2life®. Arrest was followed by 8 h of cold (4–8 °C) global ischemia [during which time the hearts were immersed in cold (4–8 °C) cardioplegia solution used to arrest the hearts] before 60 min of warm (37 °C) reperfusion with Krebs–Henseleit bicarbonate buffer. Recovery of function (expressed as % of pre-ischemic baseline function) was measured throughout reperfusion to determine recovery profile of LVDP. At the end of reperfusion, 10 hearts from each group were frozen in liquid nitrogen and stored for further analysis of viability by a 2,3,5-triphenyl tetrazolium chloride (TTC) staining method (modified from (Ferrera et al. Citation1993)), and two hearts from each group were used to measure infarct size using a previously defined method (Maruyama & Chambers, Citation2012).
TTC staining for viability
TTC staining measures tissue viability used to evaluate infarct size.
At the end of reperfusion, hearts were immediately frozen in liquid nitrogen and stored for later analysis. For analysis, the hearts were placed in a conical tube, minced into small pieces in 20 ml of phosphate buffer containing TTC (1 mM at final concentration) and incubated for 90 min at 37 °C. After 90 min, the buffer was decanted and the heart tissue homogenized in 5 ml of dimethyl sulfoxide and was agitated at a shaking table for a further 90 min. The homogenate was then centrifuged at 10,000 rpm for 1 min. The absorbance of the supernatant was measured at 480 nm, and the results were expressed as arbitrary units per gram heart wet weight.
Additionally, at the end of the isolated Langendorff perfusion protocol, 1% w/v TTC was infused into two hearts from each group at 0.5 mL/min. After ∼15 min of infusion, the hearts were removed from the cannula and stored at −20 °C for 1–2 h before slicing into 2 mm thickness sections. The heart sections were stored in 4% formaldehyde overnight at 4 °C. The following day, the heart sections were scanned using an Epson Scanner. Infarcted myocardium appeared pale yellow and viable myocardium stained brick red.
Statistical analysis
Statistical analyses were carried out using Prism version 5 of software (GraphPad Software Inc., San Diego, CA). All values were expressed as mean ± standard error of mean. Comparisons of means of data sets between two groups were carried out using unpaired Student’s t-test. A value of P < 0.05 was considered statistically significant.
Results
Recovery of function
Baseline (pre-ischemic) function and % recovery during reperfusion are shown in ; the recovery profile of LVDP in Groups C and C + M101 is shown in ; recovery was similar in both groups over the initial 20 min of reperfusion but subsequently a significant improved recovery was shown for C+M101 compared to C alone.
Figure 1. Study protocol for isolated Langendorff heart perfusion groups. Hearts were subjected to an initial equilibration period of 20 min (when pre-ischemic function was measured), arrested with a 2-min infusion (at 4–8 °C) of the cardioplegic solution prior to 8 h of cold (4–8 °C) storage in Celsior supplemented (treated group, n = 12) or not (control group, n = 12) with 1 g/L of HEMO2life® (M101) and then reperfused for 60 min with warm (37 °C) Krebs–Henseleit bicarbonate buffer. Recovery of function (expressed as a % of pre-ischemic function) was assessed throughout reperfusion.

Figure 2. Recovery of LVDP (% pre-ischemic function) over 60 min reperfusion with warm (37 °C) Krebs–Henseleit bicarbonate buffer in C and C + M101 groups. *P < 0.0001 versus Celsior.
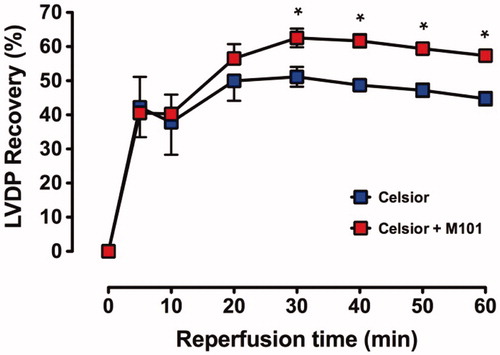
Table 1. Celsior versus Celsior + M101: baseline data for each function parameter (LVDP, LVEDP, HR and CF), and recovery data during 60 min of reperfusion.
The final recovery () after 60 min of reperfusion shows a significantly (P < 0.0001) improved recovery of C + M101 compared to C alone (57 ± 1% versus 45 ± 2%, respectively).
The recovery of CF () was significantly higher for C + M101 hearts at 30–60 min of reperfusion than C hearts; the final recovery at 60 min of reperfusion in C + M101 was 7.5 ± 0.7 mL/min compared to 5.4 ± 0.4 mL/min in C hearts (P < 0.02).
Figure 3. Recovery of CF in C and C + M101 groups. CF was measured from t = 20 min. *P < 0.02 versus Celsior.
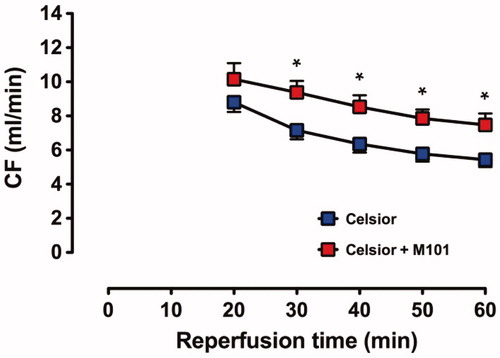
There were no differences in the recovery of LVEDP and HR between C and C + M101 (.
Myocardial viability and infarct size measurement with TTC staining
Myocardial viability is shown in . There were no significant differences in the amount of viable tissue between Celsior (C) and Celsior + M101 (C + M101) (P = 0.88). These data confirm the function data, with good recovery and only relatively small differences.
Figure 5. Myocardial viability (TTC staining) for C and C + M101 (A) and Representative transverse heart sections (2 mm) from C (a), C + M101 hearts (b), stained with TTC at the end of the isolated heart perfusion protocol. Very patchy areas of pale yellow in the myocardium are visible, representing non-viable tissues (B).
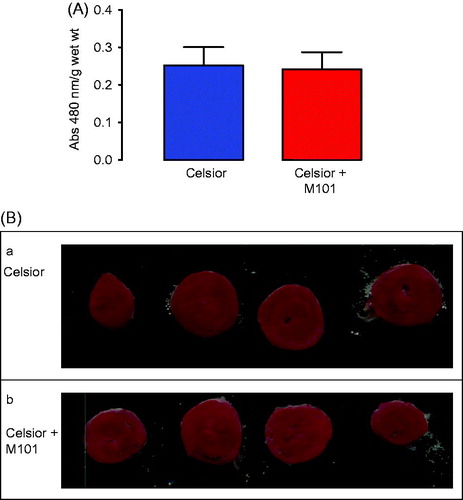
In the hearts stained with TTC and sliced to demonstrate infarct size, there were only very small and patchy areas of non-viable tissue. Representative heart slices from each group are shown in . Formal quantification or comparison of non-viable areas between the groups was not carried out due to the small number of hearts used in this method. This was performed mainly for demonstration purposes only.
Discussion
Celsior® solution was developed by Menasché et al. (Citation1994) as a long-term preservation solution specifically for myocardial tissue. Celsior® has subsequently been shown to provide effective clinical heart preservation during transplantation (De Santo et al. Citation2006), as well as other thoracic and abdominal organs (Karam et al. Citation2005). Despite its efficacy, it remains a clinical reality that ischemic storage of donor hearts is limited to a maximum duration of ∼4 h (Michel et al. Citation2002); current preservation solutions are generally used at profound hypothermia (4–8 °C), and transplantation is usually conducted as an emergency procedure outside conventional working hours. A long-time aim of many research studies has been to extend the “safe” storage period of donor hearts to enable heart transplantation to be conducted as an elective procedure; one way that this may be achieved is to use additives that enhance the protective capabilities of the solution, and there are a number of examples where this has been done in experimental studies (Koike et al. Citation2004); however, these additives have not been used clinically.
A variety of pathophysiological pathways are activated during hypothermia, such as oxidative stress, mitochondrial uncoupling and inflammation (Kosieradzki & Rowinski, Citation2008; Simmons et al. Citation2008). Moreover, injury is worsened by the sudden reperfusion of the organ with oxygenated blood and generation of deleterious free radicals. Ischemia–reperfusion injury (IRI) thus plays a central role in determining organ quality. It is thus of paramount importance to design strategies to better preserve organs in order to limit IRI and improve graft quality and outcome.
The heart is an aerobic organ that is dependent on a continuous supply of oxygen to generate the high-energy phosphate ATP for mechanical function; there is virtually no intracellular store of high-energy phosphate compounds (Doenst et al. Citation2008). Periods of severe ischemia (such as the global ischemia experienced by the donor heart subjected to long-term storage) rapidly result in the development of irreversible ischemic injury from which the heart will not recover. The use of “preservation” solutions at profound hypothermia delays the onset of irreversible injury by limiting the cellular energy metabolism (of high-energy phosphate compounds, ATP and creatinin phosphate) that is maintained during the ischemic storage (Doenst et al. Citation2008); however, the absence of oxygen in these preservation solutions means that, ultimately, high-energy phosphate compounds will be utilised during the cell’s attempts at maintenance of ionic equilibration and membrane potential.
Hypothermic preservation of donor grafts is imperative to ameliorate ischemia-related cellular damage prior to organ transplantation. The principle of hypothermic preservation is to reduce the temperature at 4 °C to slow metabolism and requirement for oxygen. During organ preservation, the need for oxygen persists because the metabolic rate remains at levels estimated ∼10%; therefore hypoxia remains a principal source of lesions induced in the context of preservation. Oxygen prevents ischemia, anaerobic metabolism and reperfusion injury.
Several approaches have been investigated to prevent the lack of oxygen. The mechanism underlying the benefit obtained with oxygen supply is the increased production of ATP during the hypothermic phase. Providing oxygen may support mitochondrial resynthesis of ATP and delay the injury process.
HEMO2life® acts to release oxygen molecules from air atmosphere passively in an oxygen gradient without requiring any allosteric effector, providing the environment with the right amount of O2. Oxygen binding molecules are usually compared by means of their oxygen dissociation curve, which gives access to characteristic parameters such as the O2 affinity (P50, the partial pressure of oxygen necessary to saturate half of the oxygen binding sites and consequently the higher the P50, the weaker the oxygen is bound), cooperativity (n50), Bohr effect and temperature effect (ΔH). Although the affinity of this hemoglobin is high under Arenicola marina physiological conditions (P50 = 2.6 mmHg), it decreases when diluted under human physiological ones (P50 = 7 mmHg). The Bohr coefficient (ΔlogP50/ΔpH) of Arenicola marina blood is normal (negative) relatively high (0.96 at 15 °C). It decreases when AmHb is diluted under human physiological conditions (0.5 at 37 °C). This decrease is explained by the increase of the temperature (Rousselot et al. Citation2006). When the PO2 is below the P50, oxygen is released passively from the hemoglobin and consumed by tissues. It corresponds to a dynamic equilibrium process dependent on cellular needs: when a O2 site becomes free, it will be reused by a new O2 molecule from air atmosphere. This molecule presents also a Cu/Zn-SOD activity that is determinant in the context of IRI (McCord et al. Citation1985, Rousselot et al. Citation2006).
This study has demonstrated a beneficial cardioprotective effect of HEMO2life®, when used as an additive to Celsior® solution during long-term preservation of hearts prior to transplantation. HEMO2life® is a natural biopolymer (M101) derived from the lugworm Arenicola marina. It is a respiratory pigment that acts as a giant oxygen carrier corresponding to extracellular hexagonal-bilayer hemoglobin at hypothermic temperatures as would be found in the lugworm (Rousselot et al. Citation2006). M101 acts to release oxygen molecules captured from air atmosphere passively in an oxygen gradient without requiring any allosteric effector, providing the environment with the right amount of O2. It provides oxygen in a controlled-release fashion to the ischemic organ that is perfused with the preservation solution. Moreover, protective effect is also due to its intrinsic Cu/Zn-SOD activity that permits to reduce cell damages by acting as a scavenger of reactive oxygen species. The absence of antibody response after one and two intravenous administrations of clinically relevant doses of M101 may be explained by the fact that M101 is not glycosylated and presents a high homology of sequence with vertebrate globin. It is well-known that globin structure is well-conserved in animal kingdom, that explains that you can inject intravenously bovine globin to human or vertebrate in general without any immune effects.
It has been shown to be effective as an additive to either UW solution or HTK solution in kidney preservation, when used in experimental pig studies in which the kidney was stored for 24 h (Thuillier et al. Citation2011). The kidney recovered to a significantly higher extent than kidneys stored in preservation solution alone, and these benefits were observed for up to 3 months post-storage.
On these evidences of safety and performance, French Regulatory Authority gave authorization for a clinical trial to evaluate HEMO2life® within hypothermic kidney graft preservation before transplantation.
Organs such as liver and kidney have an increased tolerance to cold storage when compared to the heart, and this is probably associated with the possibility to recover relatively slowly; in contrast, the heart has to function immediately at something approaching optimum capability to ensure the survival of the recipient. Hence, the significantly shorter safe storage duration, and the dependence on good maintenance of high-energy phosphate stores throughout the storage. These studies have demonstrated a significantly improved function after 8 h cold storage when HEMO2life® was added to Celsior® solution compared to Celsior® solution alone. This suggests the possibility that HEMO2life® may have the potential for a major therapeutic role in clinical heart transplantation.
Disclosure statement
F.Z. is founder and hold stock in HEMARINA SA, which produces the substance being investigated. V.P. is an employee of HEMARINA SA and does not hold stock. All other authors report no conflicts of interest. The authors alone are responsible for the content and writing of this article.
References
- De Santo LS, Amarelli C, Romano G, Della Corte A, Maiello C, Giannolo B, et al. 2006. High-risk heart grafts: effective preservation with Celsior solution. Heart Vessels. 21:89–94.
- Doenst T, Bugger H, Schwarzer M, Faerber G, Borger MA, Mohr FW. 2008. Three good reasons for heart surgeons to understand cardiac metabolism. Eur J Cardiothorac Surg. 33:862–871.
- Ferrera R, Larese A, Berthod F, Guidollet J, Rodriguez C, Dureau G, et al. 1993. Quantitative reduction of MTT by hearts biopsies in vitro is an index of viability. J Mol Cell Cardiol. 25:1091.
- Garlicki M. 2003. May preservation solution affect the incidence of graft vasculopathy in transplanted heart? Ann Transplant. 8:19–24.
- Karam G, Compagnon P, Hourmant M, Despins P, Duveau D, Noury D, et al. 2005. A single solution for multiple organ procurement and preservation. Transpl Int. 18:657–663.
- Koike N, Takeyoshi I, Ohki S, Tokumine M, Matsumoto K, Morishita Y. 2004. Effects of adding P38 mitogen-activated protein-kinase inhibitor to celsior solution in canine heart transplantation from non-heart-beating donors. Transplantation. 77:286–292.
- Kosieradzki M, Rowinski W. 2008. Ischemia/reperfusion injury in kidney transplantation: mechanisms and prevention. Transplant Proc. 40:3279–3288.
- Lee S, Huang CS, Kawamura T, Shigemura N, Billiar TR, Nakao A, et al. 2011. Histidine-tryptophan-ketoglutarate or celsior: which is more suitable for cold preservation for cardiac grafts from older donors? Ann Thorac Surg. 91:755–763.
- Lee S, Huang CS, Kawamura T, Shigemura N, Stolz DB, Billiar TR, et al. 2010. Superior myocardial preservation with HTK solution over Celsior in rat hearts with prolonged cold ischemia. Surgery. 148:463–473.
- Maruyama Y, Chambers DJ. 2012. Ischaemic postconditioning: does cardioplegia influence protection? Eur J Cardiothorac Surg. 42:530–539.
- McCord JM. 1985. Oxygen-derived free radicals in postischemic tissue injury. N Engl J Med. 312:159–163.
- Menasché P, Termignon JL, Pradier F, Grousset C, Mouas C, Alberici G, et al. 1994. Experimental evaluation of Celsior, a new heart preservation solution. Eur J Cardiothorac Surg. 8:207–213.
- Michel P, Vial R, Rodriguez C, Ferrera R. 2002. A comparative study of the most widely used solutions for cardiac graft preservation during hypothermia. J Heart Lung Transplant. 21:1030–1039.
- Perrault LP, El-Hamamsy I, Dumont E, Malo O, Carrier M. 2005. Effects of crystalloid, blood and Celsior solutions on porcine coronary endothelial function after heart transplantation. J Heart Lung Transplant. 24:912–920.
- Rousselot M, Delpy E, Drieu La Rochelle C, Lagente V, Pirow R. 2006. Arenicola marina extracellular hemoglobin: a new promising blood substitute. Biotechnol J. 1:333–345.
- Rudd DM, Dobson GP. 2009. Toward a new cold and warm nondepolarizing, normokalemic arrest paradigm for orthotopic heart transplantation. J Thorac Cardiovasc Surg. 137:198–207.
- Simmons MN, Schreiber MJ, Gill IS. 2008. Surgical renal ischemia: a contemporary overview. J Urol. 180:19–30.
- Snabaitis AK, Chambers DJ. 1999a. Long-term myocardial preservation: beneficial and additive effects of polarized arrest (Na+-channel blockade), Na+/H+-exchange inhibition, and Na+/K+/2Cl- -cotransport inhibition combined with calcium desensitization. Transplantation. 68:1444–1453.
- Snabaitis AK, Shattock MJ, Chambers DJ. 1999b. Long-term myocardial preservation: effects of hyperkalemia, sodium channel, and Na/K/2Cl cotransport inhibition on extracellular potassium accumulation during hypothermic storage. J Thorac Cardiovasc Surg. 118:123–134.
- Snabaitis AK, Shattock MJ, Chambers DJ. 1997. Comparison of polarized and depolarized arrest in the isolated rat heart for long-term preservation. Circulation. 96:3148–3156.
- Thuillier R, Dutheil D, Trieu MT, Mallet V, Allain G, Rousselot M, et al. 2011. Supplementation with a new therapeutic oxygen carrier reduces chronic fibrosis and organ dysfunction in kidney static preservation. Am J Transplant. 11:1845–1860.