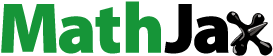
Abstract
Nanoparticle-based targeted drug delivery has the potential for rendering silibinin specifically at the favorite site using an external magnetic field. Also, it can circumvent the pitfalls of poor solubility. For this purpose, silibinin-loaded magnetic nanoparticles are fabricated, characterized and evaluated cytotoxicity and hTERT gene expression in A549 lung cancer cell line. silibinin-loaded PLGA-PEG-Fe3O4 had dose- and time-dependent cytotoxicity than pure silibinin. Additionally, hTERT expression is more efficiently reduced with increasing concentrations of nanosilibinin than pure silibinin. The present study indicates that PLGA-PEG-Fe3O4 nanoparticles, as an effective targeted carrier, can make a promising horizon in targeted lung cancer therapy.
Introduction
Cancer is a main public health problem in the world. According to WHO evaluates for 2011, cancer now causes more deaths than all coronary heart disease or all stroke and lung cancer is the most usual cancer in men and one of the four main cancers that happens in women (Ferlay et al. Citation2015). Incidence and mortality has risen steadily in lung cancer since the 1930s (Siegel et al. Citation2012). Most lung cancers could be prohibited, because they are associated with smoking (or second-hand smoke), or less often to exposure to radon or other environmental factors, but some lung cancers occur in people without any known risk factors for the disease (Mellatyar et al. Citation2014). Studies have revealed that immortality and uncontrolled proliferation of cancer cells is dependent on telomerase enzyme activity. Telomerase enzyme, a DNA polymerase, helps to retain telomere length in human stem cells, reproductive cells and cancer cells by attaching TTAGGG repeats onto the telomere sequences (Kim et al. Citation1994). So, cancer cells want a system to preserve telomerase if they are going to divide unlimitedly, and telomerase resolves this problem. Telomerase activity has been observed in almost all human tumor cells but not in adjacent normal cells (Kazemi-Lomedasht et al. Citation2013, Shay and Bacchetti Citation1997). The human telomerase RNP consists of both a catalytic protein component (hTERT) and a 451 bp integral RNA (hTR) that are essential for telomerase activity (Bodnar et al. Citation1997). After the preceding birth period, hTERT only expressed in cancer cells and immortal stem cells, and the level of telomerase activity is commensurate with expression level of its hTERT subunit (Alibakhshi et al. Citation2016). The telomerase RNP and telomere composite present various potential targets for the model of new anticancer plans. Although telomerase inhibitor agents should have great specificity, it is hoped they will also demonstrate low toxicity, low danger, and few side effects. Therefore, telomerase activity could be as a proper target for cancer therapy.
Silibinin (C25H22O10), a natural antioxidant, is extracts from the medicinal plant Silybum marianum (milk thistle) and have conventionally been used for the treatment of cancer. Silibinin has an extensive range of pharmacologic effects such as anticarcinogenic, hepato-protective, immunostimulatory, antioxidant, cell-cycle arrest, and induction of apoptosis in different cell lines including fibroblasts and lung cancer cells (Mateen et al. Citation2010, Nejati-Koshki et al. Citation2012, Surai Citation2015). Some of in vitro and in vivo works have been displayed that silibinin can arrest cancer in three stages; progression, angiogenesis, and tumor growth (Liang et al. Citation2014, Singh et al. Citation2006). Numerous molecules and signaling pathways are involved in the anticancer effects of silibinin containing VEGF, VEGF receptors, inducible nitric oxide synthase, STAT, PI3K/Akt, b-catenin, IGF-IGFBP3, NF-kB, adenosine monophosphate-activated protein kinase (AMPK), and MAPK (Deep and Agarwal Citation2010, Li et al. Citation2016).
Recent studies show that silibinin has decrease the expression of catalytic subunit of telomerase (hTERT). Also, the results of preclinical and clinical oral administration of silibinin has shown very low bioavailability of silibinin (Škottová et al. Citation2001). These obstacles can be abolished using advanced drug delivery systems, and thus, we need sensitive methods to discover the modulation of silibinin bioavailability. In order to decrease mortality due to lung cancer, it is important to improve new therapeutic methods. Nowadays, the growth of novel drug delivery systems for targeted delivery of anticancer drugs to the wanted location, decreasing side effects and increasing the efficiency of these drugs on the cancerous cells is one of the most important issues (Akbarzadeh et al. Citation2014). Recent advances have been shown that silibinin encapsulated in nano carriers, such as nano-liposome, galactosylated liposomes, and mixed micelles, improve the bioavailability of silibinin and cellular uptake, reduce the dissolution of the drug in physiological conditions, and subsequently increase drug cytotoxicity and apoptosis in cancer cells (Ardebili et al. Citation2015, Dube et al. Citation2010).
The major disadvantage of chemotherapeutic factors in cancer therapy is that they act as general and nonspecific. Nonspecific nature of chemotherapeutic agents causes dangerous side effects and harms healthy tissue. Magnetic nanoparticles can be used to overcome the issues (Dobson Citation2006). The magnetic nanoparticles encapsulated with the drug can be arrived to the target position using an external magnetic field and then initiated release of the drug at the proper location (Ebrahimnezhad et al. Citation2013). Therefore, the goal of this study was to synthesize silibinin-loaded PLGA-PEG-Fe3O4 nanoparticles and compared the effects of the pure silibinin and the silibinin–nanomagnetic encapsulated in PLGA-PEG on cell cytotoxicity and hTERT gene expression in A549 lung cancer cell line.
Materials and methods
Materials
RPMI1640 and fetal bovine serum (FBS) were provided from Gibco (Invitrogen, UK). Penicillin G, streptomycin, DL-lactide (LA), glycolide (GA), polyethylene glycol (PEG, molecular weight: 4000) stannous octoate [Sn(Oct)2], polyvinyl alcohol (PVA), dichloromethane (DCM), MTT [3–(4,5-dimethylthiazol-2-yl)-2,5-diphenyltetrazolium bromide], and dimethyl sulfoxide were obtained by Sigma-Aldrich (St Louis, MO). Ferric chloride hexahydrate (FeCl3⋅6H2O), ferrous chloride tetrahydrate (FeCl2⋅4H2O), and ammonium hydroxide (25 wt%) were purchased from Fluka (Buchs, Switzerland). RNX-plus kit was obtained from CinnaGen, Iran. First strand cDNA synthesis Kit and SYBR Green PCR Master Mix were purchased from Fermentas (Vilnius, Lithuania).
Cell culture
The human lung adenocarcinoma cell line, A549 was purchased from Pasteur Institute cell bank of Iran (code: C137) and cultured under standard cell culture conditions in RPMI-1640 media (Gibco, Invitrogen, UK) supplemented with 10% FBS and 1% penicillin/streptomycin at a temperature of 37 °C in a humidified incubator with a 5% CO2 atmosphere.
Cell viability assay
To measure the effect of pure silibinin and silibinin-loaded Fe3O4 magnetic nanoparticles modified with PLGA-PEG copolymers on cell growth, MTT assay was used with lung adenocarcinoma cancer A549 cell lines. For this assay, 6.5 × 103 cells/well were seeded in a 96-well plates and cultured. The cells were treated with different concentrations (5–150 μM) of free silibinin after 24 h and equivalent doses of nano-silibinin for different time intervals (24–72 h). The cells grown in media containing equivalent amount of ethanol without silibinin served as control. After the treatment, media containing silibinin and nano-silibinin were removed carefully and 50 μL of 2 mg/mL MTT dissolved in phosphate-buffered solution was added to each well, and the plate was covered with aluminum foil and incubated for 4 h. Then, contents of all wells were removed and 200 μL of pure DMSO was added to the wells followed by adding 25 μL Sorensen’s glycine buffer to each well. All experiments were set up in triplicates. Finally, the optical density (OD) was measured at 570 nm ELISA-reader with a reference wavelength of 630 nm. Results were expressed as mean ± SD IC50 (inhibitory concentration at 50%) was determined by Graph Pad 6 (Prism) software. Then, 5 × 105 cells were treated with serial concentrations of pure silibinin and silibinin-loaded PLGA-PEG-Fe3O4 nanoparticles (5–150 μM). The same volume of 10% DMSO without Fe3O4-PLGA-PEG4000-silibinin was added to the flask containing control cells. The cells were then incubated at a temperature of 37 °C in a humidified incubator with a 5% CO2 atmosphere for a 24 h.
Synthesis of magnetic nanoparticles
Fe3O4 magnetic nanoparticles were prepared using an improved chemical co-precipitation method (Kedar et al. Citation1997). According to this method, 3.1736 g of FeCl2⋅4H2O (0.016 mol) and 7.5684 g of FeCl3⋅6H2O (0.028 mol) were dissolved in 320 mL of bidistilled water, such that Fe2+/Fe3+ = 1/1.75. The mixed solution was stirred vigorously under nitrogen at 80 °C for 1 h. Then, NH3⋅H2O 40 mL was injected into the mixture rapidly, stirred under nitrogen for another hour, and then cooled to room temperature. The precipitated particles were separated by centrifugation and washed several time with hot water and separated by magnetic decantation. Finally, the magnetic nanoparticles were dried in vacuums oven at 70 °C.
Nanoparticle characterization
The size and shape of the nanoparticles was measured by SEM. The magnetization curves of the samples were measured with a vibrating sample magnetometer (VSM; Lakeshore Cryotronics, Westerville, OH) at room temperature. The Fourier transform infrared (FTIR) spectrum (500–4000 cm−1) from the magnetic nanoparticles and KBr were pressed to form a tablet, recorded on a FTIR spectrometer (Perkin Elmer series).
Preparation of PLGA-PEG copolymer
PLGA-PEG was provided by a melt polymerization process under nitrogen, using lactic acid and glycolic acid monomers in the presence of polyethylene glycol in the molten state and in the absence of solvent with Sn(Oct)2 as catalyst (Beletsi et al. Citation2005).
Glycolide (0.285 g), D, L-lactide (1.441 g), and PEG4000 (0.8 g) 45% w/w, were heated in a bottleneck flask to 140 °C under nitrogen atmosphere for complete melting. Then, 0.05% (w/w) Sn(Oct)2 was added and the temperature of the reaction mixture was elevated to 180 °C for 5 h. This reaction is performed by ring opening polymerization under vacuum. The copolymer was dissolved by dichloromethane and precipitated in ice-cold diethyl ether.
Encapsulation of silibinin-magnetic nanoparticles into PLGA-PEG copolymer
Silibinin-loaded Fe3O4 magnetic nanoparticles encapsulation into PLGA-PEG copolymer were obtained using the double emulsion method (w/o/w) with slight modifications (Kobayashi et al. Citation2013). First, 20 mg of silibinin was dissolved in 5 mL ethanol. Then, 250 mg of PLGA-PEG was dissolved in 4 mL dichloromethane. In the following, silibinin dissolved and 10 mg of magnetic nanoparticles added on PLGA-PEG solution and homogenized at 20,000 rpm for three minute. This w/o emulsion was transferred to a 15 mL aqueous solution of polyvinyl alcohol 1% and the mixture was probe-homogenized (or sonicated) at 72,000 rpm for one minute. Then, dichloromethane was vaporized using a rotary evaporator and the nanoparticles were purified by applying three cycles of centrifugation (10,000 rpm for 15 min in a Biofuge 28 RS, Heraeus centrifuge). The supernatant was isolated and used for compare with the total amount of silibinin to determine silibinin encapsulation efficiency of the nanoparticles using an ultraviolet 2550 spectrophotometer (Shimadzu) at 288 nm. The percent of silibinin encapsulated on the nanoparticles was determined by the difference between the total amount (OD1) used to obtain the nanoparticles and the amount of silibinin remained in the supernatant (OD2), using the following formula:
In vitro drug release
The in vitro drug release experiments of the synthesized silibinin-loaded Fe3O4 magnetic nanoparticles modified with PLGA-PEG copolymers were performed as follows: 3 mg of drug-loaded nanoparticles were dispersed in 30 mL PBS (pH =7.4). The samples were set into a dialysis tube and then kept in a shaker incubator at 37 °C and stirring at 60 rpm. At specific time interval, a 3 mL of sample was removed and same volume was replaced by adding 3 mL of PBS to each sample. The amount of the released silibinin was determined by UV spectrophotometer at 288 nm. Then, the concentration of the released silibinin was estimated using a formerly prepared standard curve of silibinin in ethanol.
Real-time PCR assay
After 24 h, total RNA was extracted from A549 cell line that was treated with different concentrations of pure silibinin and silibinin-loaded PLGA-PEG-Fe3O4 nanoparticles using Trizol reagent (Invitrogen, Carlsbad, CA). Then, purity and concentration of total RNA were measured by nanodrop ND1000 and at 260–280 nm purity of RNAs were assessed. The integrity of total RNA was validated by electrophoresis of the individual samples on a 1% agarose gel.
cDNA was synthesized using First Strand cDNA synthesis Kit (Fermentas) according to protocol. For cDNA synthesis, 2 μg of pure RNA was used as template.
hTERT gene expression study was carried out in total volume of 12 mL per reaction using a Rotor-Gene 6000 (Corbett, Sydney, Australia). Real-time PCR mixture contained 1 μL template cDNA (2000 ng), 4 μL (10 picomolar) of primers mixture (), 6 μL of SYBR green master mix, 1 μL nuclease-free water.
Table 1. hTERT mRNA level in Real-time PCR measurement.
The following sequences of the sense and antisense primers of hTERT (Genbank accession: NM_198255, bp 2165–2362) were used: forward 5′–CCGCCTGAGCTGTACTTTGT-3′ and reverse 5′-CAGGTGAGCCACGAACTGT-3′. For the internal reference gene, b-actin was chosen and its expression was detected by the following primers (GenBank accession: NM_ 001101, bp 787–917): forward 5′-ACCGTGAAAAGATGACCCAG-3′ and reverse 5′- CCATACCCAAGAAGGAAGGC-3′. β-actin was used as endogenous control. All reactions were done in triple repeats. The reaction mixture was incubated under the following conditions: 95 °C, 5 min, 1 cycle (holding step); 95 °C, 10 s, 40 cycle (denaturation); 60 °C, 30 s, 40 cycles (annealing); 72 °C, 20 s, 40 cycles (extension); 65–95 °C, 1 cycle (melting).
Statistical analysis
Graph Pad 6 (Prism) was used for statistical analysis. The differences in mRNA levels of hTERT between control and treated cells were assessed by analysis of variance (ANOVA) and Tukey’s method and P values <0.05 was considered as significant difference. Results were expressed as mean ± SD
Results and discussion
Measurement and characterization of nanoparticles
poly lactic-co-glycolic acid (PLGA) of synthetic polymeric nanoparticles is one of the best candidates that can be used for targeted drug delivery and tissue engineering applications to cancer cells because of high-quality biocompatibility and good biodegradability features (Mohammadian et al. Citation2016a, Mohammadian et al. Citation2016b). Polyethylene glycol (PEG), a nonionic hydrophilic polyether, is the most used polymers for drug delivery methods and the first PEGylated product is previously on the market for over 20 years. PEG is attached to the surface of the PLGA nanoparticles for charging the surface of the polymer and intensifying its hydrophobicity, which cause easier spread of the nanoparticles in the blood (Mohammadian et al. Citation2014, Mohammadian et al. Citation2016). Furthermore, the presence of the PEG on the surface of the PLGA nanoparticles can maintain their arrestment by phagocytes (Li et al. Citation2001). Fe3O4 is one of the most important kinds of magnetic nanoparticles which is greatly used to enable targeted drug delivery because of intrinsic magnetism (Cao et al. Citation2008). PLGA-PEG-Fe3O4 nanoparticles are constructed in the studying with the aim of improving the therapeutic effect of silibinin. The silibinin and the pharmaceutical drugs can be physically joined to PLGA-PEG-Fe3O4 surface and could be transmitted into the target site due to its exterior localized magnetic-field gradient (Avgoustakis et al. Citation2002).
The encapsulation efficiency of silibinin was indirectly measured by determining silibinin content in supernatant and the results revealed that 93% of silibinin was encapsulated at PLGA-PEG polymer. The method used for encapsulation resulted in efficient enclosure of silibinin, and the process was found to be highly reproducible content. Recently, for combinational therapy of cancer based on nanosized drug delivery system (NDDS), silibinin loaded with photothermal agent indocyanine green (ICG) into PCL lipid nanoparticles (SIPNs) showed higher efficiency of encapsulation (97.5%) (Sun et al. Citation2016). Also, Pooja et al. encapsulated silibinin into chitosan-tripolyphosphate and it has been found that more than 95% of silibinin was encapsulated at high polymer content (Pooja et al. Citation2014). These findings showed that as the silibinin content was increased in formulation, the encapsulation efficiency was decreased and particle size was increased dramatically. The decrease in encapsulation efficiency was explained by the presence of insufficient amount of polymer to encapsulate silibinin. Indeed, some parameters can be affect and control the efficiency of the encapsulation including choice of the solvent, type of polymer, weight percentage of drug, the concentration of the polymer and the drug in the solution, as well as the ratio of organic solvent to aqueous solution.
The silibinin-loaded PLGA-PEG-Fe3O4 nanoparticles were characterized using SEM, FTIR, and VSM. The size and surface morphology of the nanoparticles were measured by SEM. The nanographs of pure Fe3O4 nanoparticles and silibinin-loaded PLGA-PEG-Fe3O4 nanoparticles are shown in . The size of the Fe3O4 nanoparticles and silibinin-loaded PLGA-PEG-Fe3O4 nanoparticles are about 50–60 nm and 130–155 nm, respectively. Dispersion of the nanoparticles was greatly progressed. Due to the nanosize of the Fe3O4 of about 50–60 nm, it can be observed that the nanoparticles were well aggregated. After encapsulation and modification of the silibinin-loaded Fe3O4 magnetic nanoparticles with PLGA-PEG copolymers, the size of nanoparticles altered to 130–150 nm and dispersion of the nanoparticles was considerably improved (). It can be clarified by the electrostatic repulsion force and steric hindrance between the copolymer chains on the encapsulated Fe3O4 nanoparticles. The size of nanoparticles is a chief feature as it can influence the physical stability, biological distribution, cellular uptake, and release of the drug from the nanoparticles.
Figure 1. Scanning electron microscopy of (A) Fe3O4 magnetic nanoparticles, and (B) silibinin-loaded Fe3O4 magnetic nanoparticles modified with PLGA-PEG copolymers.
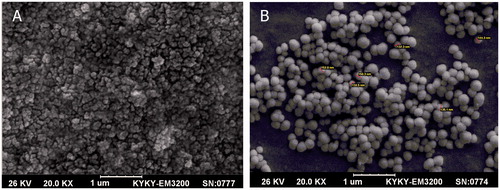
FTIR spectroscopy was applied to indicate the chemical structure of Fe3O4, PLGA-PEG copolymer and silibinin-loaded PLGA-PEG-Fe3O4 nanoparticles. From the infrared spectra shown in , the absorption peaks at 720 cm−1 is assigned to the stretching vibration mode of Fe–O bonds in Fe3O4. Furthermore, the absorption band at 3509.9 cm−1 is belonged to terminal hydroxyl groups in the copolymer which PEG homopolymer has been removed from. The bands at 2885 cm−1 due to C–H stretch of CH, and 3010 cm−1 and 2955 cm−1 are due to C–H stretch of CH. A sharp band at 1630 cm−1 is due to C = O stretch. Absorption at 1186–1089.6 cm−1 is assigned to C–O stretch. Absorption peaks recognized in the figures show that the nanoparticles were carried out correctly.
Figure 2. FTIR plot of (A) PLGA-PEG copolymer nanoparticles, (B) pure Fe3O4 nanoparticles and (C) loading silibinin in nanoparticle PLGA-PEG-Fe3O4.
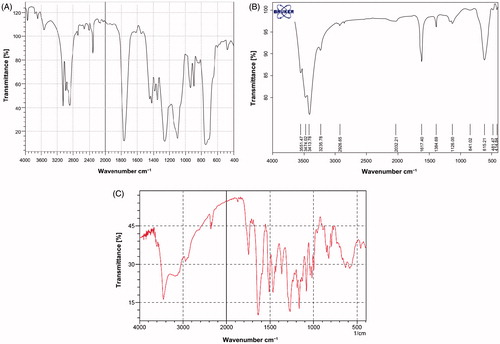
The basic chemical structure of PLGA-PEG copolymer was characterized by 1H NMR spectra that were recorded at real-time with a Brucker DRX 300 spectrometer running at 400 MHz. Tetramethylsilane (TMS) as an internal reference was used for measuring chemical shift (δ) in ppm. As shown in , a strong peak at 3.65 ppm is compliance with the methylene groups of the PEG. Overlapping doublets at 1.55 ppm were assigned to the methyl groups of the D-lactic acid and L-lactic acid repeat units. The multiples at 4.8 and 5.2 ppm is attributed to the glycolic acid CH and the lactic acid CH, respectively. The high complexity of the two peaks are resulted from different L-lactic, D-lactic and glycolic acid sequences in the polymer backbone.
Vibrating sample magnetometry (VSM) was applied to show the magnetic activities of the Fe3O4 and silibinin-loaded PLGA-PEG-Fe3O4 nanoparticles at room temperature. The saturation magnetization of the Fe3O4 nanoparticles in the hysteresis curve presented in was obtained to be 57 emu/g. This value is higher in compared to the saturation magnetization of the silibinin-loaded PLGA-PEG-Fe3O4 nanoparticles introduced in (8 emu/g). This difference recommends that the encapsulation of Fe3O4 and silibinin within the PLGA-PEG copolymers is appropriately done. Because of no hysteresis in the magnetization with both remanence and coercivity being zero, these magnetic nanoparticles are superparamagnetic. The Fe3O4 nanoparticles could be well dispersed by gentle shaking when the external magnetic field was removed. These magnetic activities are crucial for use in the biomedical and bioengineering fields.
In vitro release experiment
The in vitro silibinin release profiles were achieved by representing the percentage of silibinin release with respect to the amount of silibinin encapsulated. For the nanoparticle, silibinin release appeared in two phases: an initial burst release with a major amount of drug released within 12 h, 32.4% for Fe3O4 magnetic nanoparticles modified with PLGA-PEG nanoparticles, and after 12 h, the silibinin release profiles exhibited a sustained release pattern. The cumulative amount of silibinin release over 2 days was 80.4%. It is usually supposed that a drug is released by numerous manners, including diffusion via the polymer matrix, releasing from polymer degradation, and solubilization and diffusion via microchannels that are in the polymer matrix or are created by erosion. The magnetic copolymers planned in the present work are triblock copolymers composed of hydrophobic blocks (lactide-co-glycolide) and hydrophilic blocks (PEG). These copolymers are not soluble in water, but display reverse thermal and pH-dependent gelation properties (Oborná et al. Citation2016). Hydrolysis of the ester linkage in these polymers will produce the swelling to enhance with time as hydrolysis continues. The gel becomes progressively pH-sensitive as hydrolysis continues, and carboxylic acid groups are caused in the structure.
In vitro cell cytotoxicity
Cell viability was measured by MTT assay through exposing A549 cell line to various concentrations of free silibinin and silibinin-loaded PLGA-PEG-Fe3O4 (5–150 μM) during 24, 48, 72 h (). Data analysis of the cytotoxicity assay showed that IC50s of effect of pure silibinin and silibinin-loaded PLGA-PEG-Fe3O4 on A549 lung cancer cell line is 99.36, 73.37 μM for 24 h, 77.87, 60.87 μM for 48 h and 42.80, 34.24 μM for 72 h MTT assays, respectively.
Figure 5. Cytotoxic effect of different concentrations of silibinin on A549 lung cancer cell line during 24 (A), 48 (B), and 72(C) h.
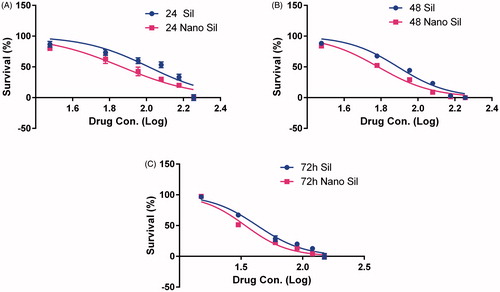
These findings displayed that by increasing the drug dose and time, the toxicity effect is increased, which points to the fact that these drugs influence the lung cancer A549 cell line in a dose-dependent and time-dependent manner. Furthermore, the IC50s indicated that cytotoxic effect of the nondrug on cells is more than that of pure drug in this cell line. It suggests that PLGA-PEG-Fe3O4 could improve the delivery of silibinin to the cells via enhancing its bioavailability and solubility.
Real-time PCR analysis
Effect of silibinin and silibinin-loaded PLGA-PEG-Fe3O4 nanoparticles on the levels of hTERT genes expressions were determined by real-time PCR. To evaluate the changes level of hTERT expression between the control and treated A549 cells were normalized to β-actin mRNA levels and then calculated by the 2-ΔΔct method. Analysis real-time PCR data showed that with increasing concentrations of silibinin, the hTERT gene expression is reduced, and the changes in hTERT gene expression in A549 lung cancer cell line treated with silibinin-loaded PLGA-PEG-Fe3O4 nanoparticles is more intense (). It can be expected that downregulation of the hTERT and inhibition of telomerase activity triggers telomere shortening and the cell reaching a critical circumstance, finally cell death by apoptosis.
Conclusions
Silibinin is a natural compound that has strong anticancer effects and few side effects. Silibinin was encapsulated into PLGA-PEG copolymer along with magnetic nanoparticles to develop efficient targeting drug delivery system for this natural anticancer. The results of the current study revealed that silibinin-loaded PLGA-PEG-Fe3O4 nanoparticles can be an inhibitory effect on the growth of A549 lung cancer cell line and hTERT gene expression in comparison to pure Therefore, silibinin incorporating with Fe3O4 loaded into PLGA-PEG co-polymer could establish an effective targeted chemotherapeutic system and a promising horizon in lung cancer therapy. Future in vitro and in vivo works will required to investigate targeting capability and effectiveness of these nanoparticles in the treatment of lung cancer.
Acknowledgements
This work was supported by grant from the Department of Medical Biotechnology, Faculty of Advanced Medical Sciences, Tabriz University of Medical Sciences (Project Number: 91/4–3/2). The authors gratefully acknowledge these research departments for their sincere support offered during the project.
Disclosure statement
The authors declare that they have no competing interests.
References
- Akbarzadeh A, Badrzadeh F, Zarghami N, Yamchi MR, Zeighamian V, Tabatabae FS, et al. 2014. Comparison between effects of free curcumin and curcumin loaded NIPAAm-MAA nanoparticles on telomerase and PinX1 gene expression in lung cancer cells. Asian Pac J Cancer Prev. 15:8931–8936.
- Alibakhshi A, Ranjbari J, Pilehvar-Soltanahmadi Y, Nasiri M, Mollazade M, Zarghami N. 2016. An update on phytochemicals substances in molecular target therapy of cancer: potential inhibitory effect on telomerase activity. Curr Med Chem. 23:2380–2393.
- Ardebili MO, Amoabediny G, Rezayat S, Akbarzadeh A, Ebrahimi B. 2015. Design and preparation of encapsulated nano-liposome controlled release including silibinin anti-cancer herbal drug (nano phytosome). SSU_J. 23:2000–2012.
- Avgoustakis K, Beletsi A, Panagi Z, Klepetsanis P, Karydas A, Ithakissios D. 2002. PLGA–mPEG nanoparticles of cisplatin: in vitro nanoparticle degradation, in vitro drug release and in vivo drug residence in blood properties. J Control Release. 79:1:123–135.
- Beletsi A, Panagi Z, Avgoustakis K. 2005. Biodistribution properties of nanoparticles based on mixtures of PLGA with PLGA-PEG diblock copolymers. Int J Pharm. 298:233–241.
- Bodnar A, Lichtsteiner S, Kim N, Trager J. 1997. Reconstitution of human telomerase with the template RNA component hTR and the catalytic protein subunit hTRT. Nat Genet. 17:502–498.
- Cao SW, Zhu YJ, Ma MY, Li L, Zhang L. 2008. Hierarchically nanostructured magnetic hollow spheres of Fe3O4 and γ-Fe2O3: preparation and potential application in drug delivery. J Phys Chem C 112:1851–1856.
- Deep G, Agarwal R. 2010. Antimetastatic efficacy of silibinin: molecular mechanisms and therapeutic potential against cancer. Cancer Metastasis Rev. 29:47–63.
- Dobson J. 2006. Magnetic nanoparticles for drug delivery. Drug Develop Res. 67:55–60.
- Dube D, Khatri K, Goyal AK, Mishra N, Vyas SP. 2010. Preparation and evaluation of galactosylated vesicular carrier for hepatic targeting of silibinin. Drug Develop Ind Pharm. 36:547–555.
- Ebrahimnezhad Z, Zarghami N, Keyhani M, Amirsaadat M, Akbarzadeh A, Rahmati M, et al. 2013. Inhibition of hTERT gene expression by silibinin-loaded PLGA-PEG-Fe3O4 in T47D breast cancer cell line. Bioimpacts. 3:67–74.
- Ferlay J, Soerjomataram I, Dikshit R, Eser S, Mathers C, Rebelo M, et al. 2015. Cancer incidence and mortality worldwide: sources, methods and major patterns in GLOBOCAN 2012. Int J Cancer. 136:E359–EE86.
- Kazemi-Lomedasht F, Rami A, Zarghami N. 2013. Comparison of inhibitory effect of curcumin nanoparticles and free curcumin in human telomerase reverse transcriptase gene expression in breast cancer. Adv Pharm Bull. 3:127–130.
- Kedar E, Palgi O, Golod G, Babai I, Barenholz Y. 1997. Delivery of cytokines by liposomes. III. Liposome-encapsulated GM-CSF and TNF-[alpha] show improved pharmacokinetics and biological activity and reduced toxicity in mice. J Immunother. 20:180–181.
- Kim NW, Piatyszek MA, Prowse KR, Harley CB. 1994. Specific association of human telomerase activity with immortal cells and cancer. Science. 266:2011.
- Kobayashi Y, Inose H, Nakagawa T, Kubota Y, Gonda K, Ohuchi N. 2013. X-ray imaging technique using colloid solution of Au/silica core-shell nanoparticles. J Nanostruct Chem. 3:1–6.
- Li J, Li B, Xu WW, Chan KW, Guan XY, Qin YR, et al. 2016. Role of AMPK signaling in mediating the anticancer effects of silibinin in esophageal squamous cell carcinoma. Expert Opin Ther Targets. 20:7–18.
- Li YP, Pei YY, Zhang XY, Gu ZH, Zhou ZH, Yuan WF, et al. 2001. PEGylated PLGA nanoparticles as protein carriers: synthesis, preparation and biodistribution in rats. J Control Release. 71:203–211.
- Liang Z, Yang Y, Wang H, Yi W, Yan X, Yan J, et al. 2014. Inhibition of SIRT1 signaling sensitizes the antitumor activity of silybin against human lung adenocarcinoma cells in vitro and in vivo. Mol Cancer Ther. 13:1860–1872.
- Mateen S, Tyagi A, Agarwal C, Singh RP, Agarwal R. 2010. Silibinin inhibits human nonsmall cell lung cancer cell growth through cell‐cycle arrest by modulating expression and function of key cell‐cycle regulators. Mol Carcinog. 49:58–247.
- Mellatyar H, Akbarzadeh A, Rahmati M, Ghalhar MG, Etemadi A, Nejati-Koshki K, et al. 2014. Comparison of inhibitory effect of 17-DMAG nanoparticles and free 17-DMAG in HSP90 gene expression in lung cancer. Asian Pac J Cancer Prev. 15:8693–8698.
- Mohammadian F, Abhari A, Dariushnejad H, Nikanfar A, Pilehvar-Soltanahmadi Y, Zarghami N. 2016a. Effects of chrysin-PLGA-PEG nanoparticles on proliferation and gene expression of miRNAs in gastric cancer cell line. Iran J Cancer Prev. 9:e4190. DOI:10.17795/ijcp-4190.
- Mohammadian F, Abhari A, Dariushnejad H, Zarghami F, Nikanfar A, Pilehvar-Soltanahmadi Y, et al. 2014. Upregulation of Mir-34a in AGS gastric cancer cells by a PLGA-PEG-PLGA chrysin nano formulation. Asian Pac J Cancer Prev. 16:8259–8263.
- Mohammadian F, Pilehvar-Soltanahmadi Y, Mofarrah M, Dastani-Habashi M, Zarghami N. 2016b. Down regulation of miR-18a, miR-21 and miR-221 genes in gastric cancer cell line by chrysin-loaded PLGA-PEG nanoparticles. Artif Cells Nanomed Biotechnol. 44:1972–1978.
- Nejati-Koshki K, Zarghami N, Pourhassan-Moghaddam M, Rahmati-Yamchi M, Mollazade M, Nasiri M, et al. 2012. Inhibition of leptin gene expression and secretion by silibinin: possible role of estrogen receptors. Cytotechnology. 64:719–726.
- Oborná J, Mravcová L, Michlovská L, Vojtová L, Vávrová M. 2016. The effect of PLGA-PEG-PLGA modification on the sol-gel transition and degradation properties. Express Polym Lett. 10:361–372.
- Pooja D, Bikkina DJB, Kulhari H, Nikhila N, Chinde S, Raghavendra Y, et al. 2014. Fabrication, characterization and bioevaluation of silibinin loaded chitosan nanoparticles. Int J Biol Macromol. 69:267–273.
- Shay J, Bacchetti S. 1997. A survey of telomerase activity in human cancer. Eur J Cancer. 33:787–791.
- Siegel R, Naishadham D, Jemal A. 2012. Cancer statistics, 2012. CA Cancer J Clin. 62:10–29.
- Singh RP, Deep G, Chittezhath M, Kaur M, Dwyer-Nield LD, Malkinson AM, et al. 2006. Effect of silibinin on the growth and progression of primary lung tumors in mice. J Natl Cancer Inst. 98:846–855.
- Škottová N, ŠVagera Z, Večeřa R, Urbánek K, Jegorov A, Šimánek V. 2001. Pharmacokinetic study of iodine-labeled silibinins in rat. Pharmacol Res. 44:247–253.
- Sun HP, Su JH, Meng QS, Yin Q, Zhang ZW, Yu HJ, et al. 2016. Silibinin and indocyanine green-loaded nanoparticles inhibit the growth and metastasis of mammalian breast cancer cells in vitro. Acta Pharmacologica Sinica. 37:941–949.
- Surai PF 2015. Silymarin as a natural antioxidant: an overview of the current evidence and perspectives. Antioxidants (Basel). 4:204–247.