Abstract
Hydrophobic modification of low molecular weight polyethylenimine (PEI 2 kDa) by cholic acid (ChA) was done to obtain PEI2-ChA. The nanoplexes of PEI2-ChA with gWIZ-GFP demonstrated increase transfection efficiency (∼27%) in NT8e cell lines. The cell-cycle analysis of NT8e cells (p53 mutant) treated with transferrin containing nanoplexes showed increased apoptosis of cells. In vitro protein expression revealed expression of exogenous p53 protein. In vivo imaging of mice showed localized signal for GFP protein in brain region. The tumors of mice treated with transferrin containing nanoplexes of PEI2-ChA were ∼5 times smaller in size than the tumor of untreated animals.
Introduction
Gene delivery to the target cells still remains to be a major hurdle in the success of gene therapy. Being relatively safer than viral vectors, non-viral vectors have been extensively investigated for gene delivery. Among polymers, polyethylenimine (PEI) and its variants are the most studied cationic polymers for delivery of nucleic acids. PEI has a high-charge density at reduced pH values due to presence of nitrogen atom at every third position along the polymer which imparts PEI the ability to promote in vitro and in vivo gene transfection (Kircheis et al. Citation1999). PEI-based polyplexes have been reported for both in vitro and in vivo delivery of pDNA, siRNA, and mRNA. PEI has been shown to have highest transfection efficiencies both in vitro and in vivo and, therefore, has been considered as a “gold standard” in non-viral gene delivery (Lemkine and Demeneix Citation2001). Polyethylenimine forms a complex with pDNA which has shown high gene expression during in vitro and in vivo gene delivery. However, on one hand, the high molecular weight polyethylenimine (PEI) has not been clinically employed due to its non-specific cytotoxicity displayed against different cell types. The branched PEI-pDNA complex binds non-specifically to negatively charged proteoglycans on cell membranes and agglutinates with erythrocytes and serum albumins. Such agglutination of the complex with cellular and blood components often lead to its rapid elimination and adverse effects, such as embolism and inflammatory reaction. On the other hand, low molecular weight PEIs are much less cytotoxic, but they are not efficient transfection agents due to limitations of DNA delivery into the cytoplasm. In order to reduce the cytotoxicity and agglutination with blood components and modify the complex surface, several novel polymers of PEI have been developed by covalently binding to hydrophilic polymers; have been developed to modify the complex surface (Lian et al. Citation2014). In case of hydrophobic modification, the anionic polymers can bind to the cationic PEI electrostatically to modify the complex surface. Such hydrophobic modification of low molecular weight PEI (2 kDa) using cholic acid resulting in its enhanced transfection efficiency has been previously reported (Dube et al. Citation2013). During cellular uptake, aggregated PEI-pDNA complexes (having large size) are internalized with great difficulty but complexes with mean diameter less than 200 nm are easily internalized as the average diameter of endosomes is ∼100–200 nm (Murk et al. Citation2003). Even in case of complexes with particle size less than 200 nm, the complexes must pass through the epithelial tissue of the blood vessels and enter the target tissue after administration which may be limited due to their various non-specific interactions of complex with cellular and blood components. Interactions of positively charged DNA/polycation complexes with the extracellular matrix or the serum proteins has been an proven obstacles to the in vivo gene delivery using non-viral vectors for gene therapy (Pandey and Sawant Citation2016). The main pre-requisite for enhancing targeting specificity of complexes is to modify them in a manner that would help them identify specific cell types to avoid any non-specific interaction/toxicity and to ensure safety. Various surface modifications of PEI-pDNA complexes have been reported with the aim of decreasing non-specific interaction and enhancing cell specific targeting. Poly(ethylene glycol) derivatives having carboxylic acid-side chains (PEG-C) were explored for suppressing non-specific interaction by using it as coating material for DNA/polycation complexes (Pandey et al. Citation2014). The obtained results suggested that introduction of the ligand into the side chains of the polymer will allow the receptor-mediated cell-specific and enhanced gene transfection. PEI-based nanoparticles conjugated to succinic anhydride have been reported previously for delivery of interleukin 12 gene into hepatocytes (Khalvati et al. Citation2016). The results revealed that the conjugated PEI derivatives enhanced the transfection efficiency by up to three-fold in comparison to unmodified PEI. Apart from PEI, various other nanoparticles based systems have been explored for immunotherapy and drug delivery to brain tumor (Chen et al. Citation2016, Geldenhuys et al. Citation2011, HosseiniHaji-Fatahaliha et al. Citation2016, Kutlu et al. Citation2014, Tripathi et al. Citation2015, Wankhede et al. Citation2012). The use of surface modifying agents or targeting agents further enhances the efficacy of such systems
The addition of other selective ligands such as transferrin (Tf) or folate has also been reported to enhance cell-specific targeting along with cellular internalization via endocytosis (Jing et al. Citation2014, Yoshiyuki Tomoko et al. Citation2003). Transferrin receptor (TfR), a membrane glycoprotein which mediates cellular uptake of iron from a plasma glycoprotein transferrin, is known to be over-expressed on various tumor cell surfaces. Transferrin (Tf), a natural iron-binding blood plasma glycoprotein, has been reported as an effecting ligand to coat DNA/polycation complexes without decomposition. It can improve cellular internalization and avoid any non-specific interactions by shielding the nanoplexes. A Tf-conjugated liposome complex loaded with a Bcl-2-specific oligo-deoxyribonucleotide (G3139) showed improved in vitro and in vivo targeting and internalization into K562 cancer cells (Chiu et al. Citation2006). TfR is upregulated on the surface of various cancer types and is internalized efficiently via endocytsis, hence provides a means of targeting anticancer drugs and nucleic acid medicines to tumor cells (Klausner et al. Citation1984).
The present investigation deals with development of novel non-viral vector based nanoplexes for gene delivery using cholic acid modified PEI aimed at targeting them to brain tumor with enhanced transfection and targeting efficiency. This work aims at exploring transferrin (Tf) as a ligand for enhancing endocytosis and targeting efficiency of nanoplexes using polyelectrolyte complexation method. Tf incorporated nanoplexes were evaluated for brain targeting ability and in vivo performance.
Materials and methods
Polyethylenimine 2 kDa (PEI-2), polyethylenimine 25 kDa (PEI-25), cholic acid (ChA), deuterated water (D2O), Hank’s balanced salt solution (HBSS), trypsin/EDTA, N-hydroxysuccinimide (NHS), N,N'-dicyclohexyl-carbodiimide (DCC), 30% acrylamide/bis solution 37.5:1, and sodium chloride were purchased from Sigma-Aldrich (Mumbai, India). All other chemicals and reagents used were of analytical grade.
Experimental
Preparation of nanoplexes
The nanoplexes (with or without transferrin) were prepared by the polyelectrolyte complexation technique owing to the charge differences among polymer, pDNA, and transfer (Nakase et al. Citation2005). The nanoplexes of unmodified polymer (or polymer conjugates) and pDNA (incorporated with or without transferrin) were prepared according to the method previously described in Joshee et al. i.e., by simultaneous gentle mixing of cationic species (polymer or its conjugate), with the anionic pDNA followed by short incubation (for transferrin conjugated nanoplexes) (Joshee et al. Citation2002). Appropriate amounts of polymer and pDNA were each dissolved in HEPES buffered 150 mM saline to prepare the nanoplexes. The polymer solution was added to pDNA solution in a low-binding tube, followed by brief vortexing and incubation for 30 min at 37 °C (Vader et al. Citation2012). The formed nanoplexes were separated by centrifugation at 15,000 rpm for 30 min (Remi, Mumbai, India). Appropriate quantity of unmodified polymer or polymer conjugate stock solution (1 mg/ml) was added to pDNA (0.8 μg) in 150 mM saline and the final volume was brought to 60 μl. The nanoplexes were formed at different polymer:pDNA weight ratios (ranging from 3.75 to 30). To obtain transferrin incorporated nanoplexes, two different Tf to pDNA weight ratios (5 and 10) were mixed prior to nanoplex formation.
Characterization of nanoplexes
Particle size and zeta potential analysis
The hydrodynamic diameters of the nanoplexes were determined by dynamic light scattering using zetasizer (NanoBrook 90Plus, Brookhaven Instruments Corporation, Brookhaven, NY). Zeta-potential measurements were carried out using a zetasizer (NanoBrook 90Plus, Brookhaven Instruments Corporation, Brookhaven, NY) at 25 °C using fold capillary cell (Clements et al. Citation2009, Zheng et al. Citation2012).
GFP expression in U87MG cells
The U87MG cells were treated with nanoplexes prepared with PEI (PEI25) or modified PEI (PEI2-ChA) (with or without transferrin) at two polymer to pDNA weight ratios (5:1 and 12:1) and two different Tf to pDNA weight ratios (5:1 and 10:1). Appropriate quantity of polymer or polymer conjugate stock solution (1 mg/ml) was added to gWIZ-GFP (1.8 μg) in 150 mM saline with a final volume of 60 μl, vortexed gently for 5 s and incubated for 30 min at room temperature. The U87MG cells were seeded in 24-well tissue culture plates with 10% FCS DMEM. Cells achieved ∼70% confluency within 24 h, after which they were transfected with prepared nanoplexes by adding 20 μl of prepared nanoplexes to each well in triplicate. The nanoplexes prepared using PEI25 and PEI25-ChA were used as positive control. The cells were allowed to incubate at 37 °C in CO2 incubator and, after 48 h, the media was removed and cells were trypsinized and fixed in 3.7% formaldehyde in HBSS (250 μl) so as to prepare samples for analysis by flow cytometry.
GFP expression in NT8e cells
The NT8e cells were seeded in 24-well tissue culture plates with 10% FBS DMEM and incubated till cells achieved ∼70% confluency. The cells were then transfected with transferring-containing nanoplexes (prepared at two different polymer conjugate to pDNA weight ratios 10 and 12 and at Tf to pDNA weight ratio of 10). The nanoplexes were prepared using appropriate quantity of polymer conjugate stock solution (1 mg/ml) with 1.8 μg of pDNA (gWIZ-GFP) in 150 mM saline with a final volume of 60 μl and 20 μl of nanoplexes added to each well in triplicate. The cells were allowed to incubate at 37 °C in CO2 incubator and, after 48 h, the media was removed and cells were trypsinized and fixed in 3.7% formaldehyde in HBSS (250 μl) so as to prepare samples for analysis by flow cytometry.
Stability of nanoplexes
To assess the stability of nanoplexes, experiments were carried out in 293T cells using two types of nanoplexes, first being the nanoplexes after preparation without further incubation and were termed as the “no incubation” group. The nanoplexes which were incubated at 37 °C for 24 h after preparation were termed as the “24 h incubation” group. The transferrin containing nanoplexes of PEI2-ChA conjugate with pDNA (gWIZ-GFP) were prepared (at polymer conjugate to pDNA weight ratio of 10 and Tf to pDNA weight ratio of 10) in 150 mM saline solution. The nanoplexes of lipofectamine (commercially available standard transfecting agent) and PEI25 were also prepared with pDNA (gWIZ-GFP) at polymer to pDNA weight ratio of 10 in 150 mM saline solution. About 20 μl of the prepared nanoplexes were added to the 293T in three different wells (triplicate) and 72 h post-nanoplexes addition, cells were harvested using routine procedure of trypsinization and cells were fixed in 3.7% formaldehyde in HBSS (250 μl) so as to prepare samples for analysis by flow cytometry.
BMP-2 production in hBMSC cells
The human BMP-2 production assay was performed in hBMSC cells using DuoSet ELISA Development Kit (#DY355, Remi, Mumbai, India). The nanoplexes were prepared (at the polymers and plasmid BMP-2 with weight ratios of 2, 4, and 8) and added to the 70% confluent hBMSC cells in 24-well plates (in triplicate). The complexes were removed after 24 h by centrifugation at 4000 rpm and the cells were incubated with fresh medium for 6 days. The supernatant were recovered by separating the upper layer after centrifugation for BMP-2 production. For assessment of the BMP-2 concentration, 96-well microplates were coated with a diluted capture antibody (100 μl) in PBS and incubated overnight at RT. Each well was washed with wash buffer (3×) and the remaining sites were blocked by incubating for 1 h at RT with 1% BSA solution (300 μl). The supernatants (100 μl) of the transfected cells or standards in 1% BSA solution were added to the wells and incubated for 2 h at room temperature. The wells were then washed and incubated with a biotinylated detection antibody (100 μl) for 2 h, followed by the addition of horseradish peroxidase-conjugated streptavidin solution (100 μl) for 30 min in the dark. After washing, 100 μl of substrate solution was added to the wells and the reaction was stopped after 20 min with 50 μl of 2N H2SO4. Immediately, the absorbance in individual wells was measured at 450 nm using a microplate reader and converted into BMP-2 concentration using a calibration curve generated using the manufacturer supplied BMP-2 standard.
Cell-cycle analysis in NT8e cells
NT8e cells were treated with transferrin containing nanoplexes of polymer or polymer conjugates with pDNA at different polymers to pDNA weight ratios and incubated for 24 h. Cells were harvested, washed twice with PBS and fixed in chilled 70% ethanol. The fixed cell pellet was washed with PBS and re-suspended in 200 μl of PBS. Cells were treated with 10 μl RNAse solution (0.5 mg/ml concentration) for 15 min at 37 °C. The volume was made up to 1 ml and 50 μl propidium Iodide (50 μg/ml concentration) was added to it. Cells were incubated for 10 min at room temperature and the data for 10,000 events were acquired on FACSCalibur (BD Biosciences, Singapore, Singapore) and analyzed using Modfit software (BD Biosciences, Singapore, Singapore).
In vitro protein expression studies
It is necessary to calculate total amount of protein present in the cell lysate before evaluation of actual protein expression (LiuCong et al. Citation1999). Twenty-four hours after the initial addition of nanoplexes, cells were harvested by trypsinization and lysed in 1% nonidetP40 containing 1 mg/ml aprotinin (Sigma, Chicago, IL), 1 mg/ml leupeptin (Sigma, Chicago, IL), and 1 mg/ml pepstatin A (Sigma, Chicago, IL). The cell lysates were separated by 8%-16% sodium dodecyl sulfate polyacrylamide gel electrophoresis (SDS-PAGE) and transferred to a nitrocellulose membrane at 200 mA, 4 °C for 45 min and β-actin was used as an internal loading control. The membrane was blotted with 5% non-fat dry milk in PBS containing 0.1% Tween 20 for 1 h at room temperature. Following the application of the primary mouse monoclonal IgG1antibody p53 (F-8) (1:1000 diluted, sc-374087) for 1 h, the blot was incubated with secondary antibody goat anti-mouse IgG horseradish peroxidase (HRP)-conjugated (1:5000 diluted, #sc-2005) for 1 h and developed using chemiluminescent substrates.
Biodistribution studies
The animals (Swiss bare mice) were divided into three groups. Group A was administered with 100 μl of either pDNA in saline or nanoplexes without transferrin injected in animals through tail vein. Animals in group B received 100 μl of either pDNA in saline or nanoplexes with transferrin through tail vein. Control group (group C) received 100 μl of nanoplexes containing 15 μg of pDNA with or without transferrin (polymer:plasmid weight ratio 10:1) or naked plasmid DNA (15 μg) in saline injected intravenously into the tail vein of Swiss bare mice. The animals were provided water and food ad libitum. After 48 h of injection, mice were anesthetized with an i.p. injection of mixture of ketamine (50 mg/ml) and xylazine (2 mg/ml) at 150 mg/kg of body weight (Caysa et al. Citation2012). After imaging, the mice were euthanized humanely and major organs namely brain, lungs, liver, and spleen were removed for direct measurement of fluorescence.
Tumor regression studies
NT8e xenograft tumors were developed in 6 to 8-week-old either sex nude mice (5 mice per group) by implanting a cell suspension of 5 × 106 NT8e cells (Xue et al. Citation2007). Animals were divided into two groups (five animals in each group): (1) control (injection of plasmid p53 in saline) and (2) PEI2-ChA-p53-Tf nanoplexes in saline (polymer:plasmid weight ratio 10:1 and plasmid:transferrin weight ratio 4:1). As soon as the tumors developed ∼100 mm3 in size (∼after 35 d), transferrin containing nanoplexes (PEI2-ChA and plasmid p53 incorporated with Tf) in 100 μl of saline were injected intratumorally to investigate the tumor regression ability. The quantity of plasmid used was 8 μg (at polymer:plasmid weight ratio of 10:1 and weight ratio of 4:1 of plasmid:transferrin). The animals were injected with either saline (control) or polyplexes (treatment) again after day 3 and 7 of first injection. Tumor volume was monitored for next 48 days. Finally, mice were sacrificed on the 48th day and photographs were taken. The results were plotted as tumor volume versus days post-tumor transplantation.
Results and discussion
Preparation of nanoplexes
Effective condensation of pDNA by PEI-ChA conjugates into nanoplexes, more so with the transferrin incorporated system, is critical for its successful application in gene delivery. Condensation protects pDNA from enzymatic degradation and facilitates uptake of complexes by cells. The cell-binding ligand transferrin was also incorporated into the complexes to combine the high gene transfer efficacy of nanoplexes of PEI-ChA and pDNA with the target-specific mechanism of receptor mediated uptake. Binding of the Tf shielded pDNA nanoplexes to the Tf receptor on the target cells, followed by endocytosis into vesicles, escape of the DNA from the endosomal compartment, and nuclear entry are critical steps for efficient transfection. In the present investigation, condensed nanoplexes were obtained which were further characterized. The formed nanoplexes were highly condensed which was visible from the particles size.
Characterization of nanoplexes
Dynamic light scattering (DLS) studies
The particle sizes of nanoplexes prepared with polymer or polymer conjugates with plasmid DNA were recorded (Brookhaven 90Plus, Brookhaven Instruments Corporation, Brookhaven, NY). The dynamic light scattering analysis showed that the hydrodynamic diameter of nanoplexes was within the range of 100–230 nm. The particle size distribution pattern indicated that all particles were in nanometer range with polydispersity index of less than 0.45 and devoid of any aggregates.
It can be observed from that the particle size of polymer conjugates (PEI2-ChA) was smaller in comparison with their native counterparts similar to the observation reported by Zheng et al. in which it was demonstrated that reduction in particle size was observed after conjugation of lipoic acid with PEI-1.8 via dicyclohexylcarbodiimide (DCC)/N-hydroxysuccinimide (NHS) coupling (Zheng et al. Citation2012). The polymer conjugates of PEI2 and PEI25 (substitution ratio: 4) were able to condense plasmid DNA to form complexes with smaller particles size (90–100 nm) which was attributed to increased hydrophobic interactions leading to more efficient particle assembly (Grzelczak et al. Citation2010). However, the incorporation of transferrin to the nanoplexes system of polymer or polymer conjugate with pDNA showed an increase in particle size ∼80 nm which was attributed to bulky nature of the ligand.
Zeta potential
In addition to small size, a cationic surface charge is also desired as it helps in electrostatic interaction between nanoplexes and anionic proteoglycans, such as syndecan on cell surfaces, resulting in increased endocytosis (Gratton et al. Citation2008, Kopatz et al. Citation2004). The obtained value of zeta potential (ζ) (17–30 mV) indicated that all particles had positive zeta potential with lower standard deviation, which means that the nanoplexes would help in binding to cell surface. The zeta potential of transferrin containing nanoplexes was also positive, but the overall positive charge for transferrin containing nanoplexes decreased significantly owing to its negative charge. The reduction in resulting surface charge may reduce the non-specific interaction with anionic proteins that occurs in blood circulation in vivo and may act as shield against opsonization. This in turn may enhance transferrin-mediated uptake of nanoplexes in the brain cells by transferrin receptor (TfR) mediated endocytosis (Ogris et al. Citation1998). The zeta potential measurements showed that nanoplexes formed by PEI2-ChA and PEI25-ChA at higher cholic acid substitution levels had higher cationic charge (∼25–30 mV) than unmodified PEI2 and PEI25 (∼17 mV) () which may be attributed to lipid–lipid interactions in complexes which are likely to facilitate more stable assembly of polymers on soluble pDNA, hence increasing the overall zeta potential. A similar phenomenon was also reported in DNA complexes formed with lipid-modified low molecular weight PEI (Bahadur et al. Citation2011, Clements et al. Citation2009). shows the typical zeta potential (ζ) graph showing zeta potential (ζ) in mV for nanoplexes (with and without Tf) prepared in 150 mM saline at polymer to plasmid ratio of 10:1. This higher (∼10) overall positive zeta value of nanoplexes will impart stability in solution (Neu et al. Citation2006) and may help them avoid charge-charge interaction among each other.
GFP expression in U87MG cells
In pursuit of demonstrating the GFP expression in a brain derived cell line, the transfection efficiency experiment was estimated in U87MG cell line. Transferrin receptor (TfR) is one of the most attractive targets to overcome the blood–brain barrier. U87MG cells have been shown to overexpress TfR (Xu et al. Citation2011). Cellular iron uptake is mediated by this ubiquitously expressed receptor for transferrin and it also plays a key role in the control of the rate of cellular iron uptake, tuning the amount of iron delivered to the cells to the metabolic needs (Calzolari et al. Citation2010). Therefore, U87MG cell line was chosen to evaluate transfection efficiency of transferrin containing nanoplexes. shows treatment groups with corresponding mean %GFP positive cells after normalization.
Table 1. Treatment groups of nanoplexes with corresponding mean %GFP positive cells after normalization in U87MG cells.
It was observed that the cellular exposure of placebo nanoplexes to U87MG did not yield significant autofluorescence which indicated relatively little toxic effect of nanoplexes. As shown in , the transfection efficiency of PEI2-ChA conjugates at lower polymer:plasmid weight ratio of 5 yield less GFP (34.72%) in the U87MG cell population as compared with PEI2-ChA with higher polymer:plasmid weight ratio (12) which demonstrated 79.41% GFP positive cells (almost double the amount for lower weight ratio). Similar transfection results were also observed in 293T cells and CB-MSCs for nanoplexes of PEI2 and PEI25 series conjugates (for nanoplexes without transferrin). Moreover, the cells with treatment groups of nanoplexes with conjugates of both the polymer series (PEI2 and PEI25) containing transferrin produced more GFP as compared with the nanoplexes without transferrin. A similar trend was observed for nanoplexes prepared with unmodified PEI25 polymer. shows GFP positive and negative cells (shift from gated region) corresponding to different treatment groups with and without transferrin.
Figure 3. The % GFP positive U87MG cells (after normalization) corresponding to different treatment groups.
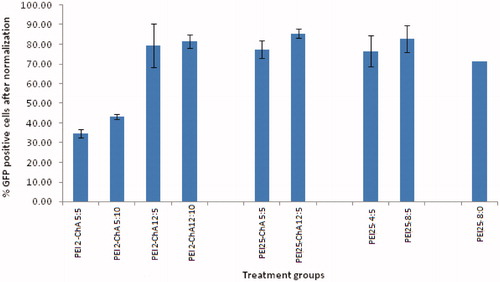
Figure 4. Flow cytogram showing GFP positive and negative cells (shift from gated region) corresponding to different treatment groups with and without transferrin.
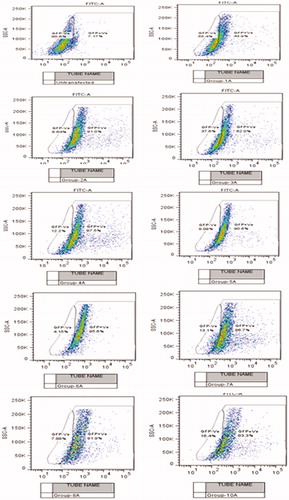
It can be also seen that the nanoplexes prepared with unmodified PEI25 polymer but without transferrin showed less GFP expression as compared with the nanoplexes with transferrin. These results were in line with the earlier work of Joshee et al. who showed that their standard formulation, which was prepared by adding DNA to a mixture of transferrin and lipofectin, yielded higher transfection efficiency than the formulation without transferrin (Chiu et al. Citation2006). An increased transfection efficiency of the nanoplexes can be attributed to the overexpression of TfR on U87MG cells (Sang-SooAntonina et al. Citation2014).
GFP expression in NT8e cells
The nanoplexes of PEI2-ChA with and without transferrin were evaluated for transfection efficiency using NT8e cells (p53 mutant). The NT8e cell line was established from human head and neck cancer by group of our research collaborator (Dr. Rita Mulherkar) at Cancer Research Institute, Mumbai and was maintained in the cell medium (Mulherkar et al. Citation1997). The cell line grows as a monolayer in vitro and as a transplantable tumor in immune-compromised mice in vivo, and has been used as a model for preclinical studies (Mulherkar Citation2010). The NT8e cell line is also one of the cell lines which are hard to transfect and are more sensitive to the toxicity of polymers. shows the typical gating parameters set for the normal NT8e cells based on their volume. As seen in , the transferrin-containing nanoplexes showed transfection of ∼25% and ∼30% for polymer:plasmid weight ratio of 10 and 12, respectively. The control cells were normalized to ∼1% for transfected cells (M2).
Figure 5. GFP expression for nanoplexes prepared in 150 mM saline with polymer to plasmid weight ratio of 10 (B) and 12 (C) in NT8e cells after 48 h by fluorescence microscopy; (A) is cells treated only with 150 mM saline.
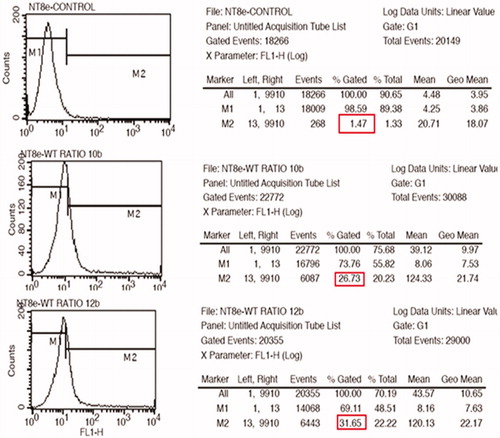
Figure 6. GFP expression for nanoplexes prepared in 150 mM saline with polymer to plasmid weight ratio of 10 (A) and 12 (B) in NT8e cells after 48 h by fluorescence microscopy; (A) is cells treated only with 150 mM saline.
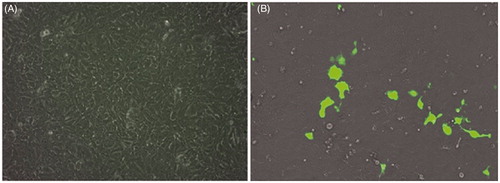
The fluorescent microscopic image () revealed a similar trend of transfection as observed by flow cytometric analysis for the transferrin-containing nanoplexes prepared at two different weight ratios of polymer:pDNA (i.e., 10 and 12) using GFP as a reporter gene. shows the qualitative transfection efficiency for cell treated with the nanoplexes prepared at polymer to pDNA weight ratios of 12.
The fluorescent microscopic imaging revealed lower transfection for the transferrin-containing nanoplexes prepared at two different weight ratios of polymer:pDNA (i.e., 10 and 12) using GFP as a reporter gene. Although microscopy is a qualitative analysis, it implies that the cell line was hard to transfect. However, it appears that qualitative evaluation showed significant difference in transfection efficiency at two different polymer:pDNA weight ratios. In other words, it can be stated that higher weight ratios of polymer:pDNA might produce higher transfection in these cells.
Stability of nanoplexes
After the formation of the nanoplexes, the utility time frame for transfection is limited as the nanoplexes are slightly unstable in the solution and are prone to form large aggregates over time. Aggregation can arise in vivo because of interaction with serum protein and/or interaction with other PEI complexes as a result of hydrophobic shielding. One of the reasons for vast difference in extent of in vitro and in vivo transfection efficiencies can be attributed to variable complex stabilities. Systemic administration exposes polyplexes to physiological ionic strengths and anionically charged proteins, which can induce aggregation and destabilization by non-specific interactions (Petersen et al. Citation2002). For in vitro experiments, the nanoplexes are incubated for 30 min before addition to the cells. But for in vivo application, the complexes need to remain stable and should be able to transfect the cells for considerably longer period of time.
Although the overall charge of the complex is positive, particles can exist as amphiphatic molecules with pockets of hydrophobic regions (Ikonen et al. Citation2008); thus, particles may spontaneously bind to each other to shield these hydrophobic pockets from the aqueous surrounding, forming aggregates. The large aggregates are not desirable due to their lower cellular uptake efficiency. Also, such large particles are not as readily dissociated and can lead to increased toxicity, resulting in dramatic reduction in transfection efficiency (CharlieMing and Hasan Citation2012). Because of this time sensitivity, complexes are typically incubated with cells for a limited time frame (i.e., <24 h, typically about 2 h).
The transfection efficiency of these two treatment groups was assessed at two different weight ratios. The nanoplexes were prepared and immediately added to the cells in the treatment group one (no incubation) whereas, for group 2 (24 h incubation), the nanoplexes were prepared 24 h before and added to 293T cells as shown in . It should be noted that both groups of nanoplexes were added to the cells at the same time.
Figure 7. Mean fluorescence intensity of FL1 + cells for treatment groups with nanoplexes with “no incubation” after preparation and “24 h incubation” after preparation (incubation at 37 °C).
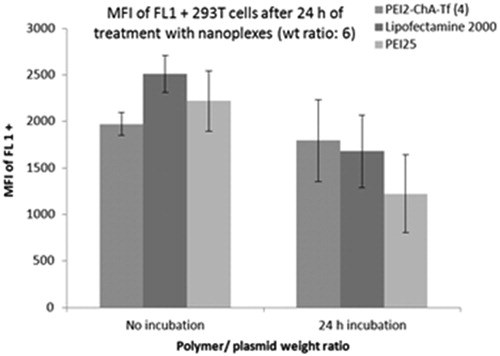
It was observed that transfection efficiency of nanoplexes with lipofectamine 2000 (commercially available standard transfecting agent) and PEI25 was decreased after 24 h incubation, whereas no significant loss of transfection efficiency was observed with nanoplexes prepared using PEI2-ChA. These results may be attributed to the fact that transferrin plays role in shielding the hydrophobic pockets (formed in the nanoplexes) from the aqueous surrounding as reported earlier (Ikonen et al. Citation2008) ().
BMP-2 production in hBMSC cells
For further verification of enhanced transfection efficiency of the human bone, morphogenetic protein (BMP-2) production study was performed with polymer conjugates and plasmid BMP-2. It is a very sensitive assay and may give insights about synthesized polymer conjugate’s ability of transfecting hard to transfect cells like hBMSC cells (Wang et al. Citation2013). It was clearly visible from that the nanoplexes with PEI2-ChA at polymer/plasmid (BMP-2) weight ratio of 4:1 and 16:1 ratio led to increased BMP-2 production compared with the nanoplexes prepared with PEI25 at 4:1 or 2:1 weight ratio. PEI25 showed higher toxicity even at the lowest concentration (2:1) whereas much less cytotoxicity was observed at highest ratio (16:1) of PEI2-ChA. The hBMSC cells are more sensitive and hence PEI25 causes more toxicity to it even at lower weight ratios.
Cell-cycle analysis in NT8e cells
Apoptosis is crucial in eliminating cells with unrepaired DNA damage and preventing carcinogenesis. It is characterized by a p53-dependent induction of pro-apoptotic proteins, leading to permeabilization of the outer mitochondrial membrane, release of apoptogenic factors into the cytoplasm, activation of caspases, and subsequent cleavage of various cellular proteins (Fragkos and Beard Citation2011). Apoptogenic effects include chromatin condensation and exposure of phosphatidylserine on membrane surfaces of the cell (Meier and Vousden Citation2007). It has been suggested that p53-independent mechanisms of killing tumor cells may not involve apoptosis and could be a result of induced mechanical damage, rather than programmed cell death (Finkel Citation1999). Cell-cycle analysis was conducted to understand the effect of nanoplexes containing p53 on the cell cycle. This method requires propidium iodide (PI) and employs flow cytometry for the cell-cycle analysis. Before analysis, the cells were permeabilized and treated with PI (a fluorescent dye that stains DNA quantitatively). As the DNA content of cells varies in different phases, the relative amount of cells in the different phases of cell cycle can be determined based on the quantity of DNA. The transferrin incorporated nanoplexes of PEI2-ChA with plasmid p53 showed increased apoptosis in NT8e cells (). The increased apoptosis clearly suggested restored p53 function. Also, it can be seen that polymer conjugates (PEI2-ChA and PEI25-ChA) exhibited low apoptosis values indicating that they did not contribute to the apoptosis. In other words, apoptosis is solely due to delivery of plasmid p53 into the cell which consequently restores the p53 protein.
In vitro protein expression studies
The p53 tumor suppressor protein is involved in several central cellular processes that are critical for maintaining cellular homeostasis, including gene transcription, DNA repair, cell cycling, senescence, and apoptosis (Levine Citation1997, Vogelstein and Kinzler Citation1992). But compared with the vast information and knowledge available regarding the role of p53 protein in apoptosis, the function of p53 in cell differentiation is not well understood. It was imperative to analyze the p53 gene expression before proceeding for in vivo animal studies. Before evaluating the transferrin-containing nanoplexes of polymer conjugates in vivo, an in vitro protein expression study was carried out to demonstrate the ability of prepared nanoplexes to synthesize the desired protein, p53. For in vitro protein expression studies the NT8e cell line was selected which is a p53 mutant cell line.
It was clearly seen from that p53 was expressed significantly more in NT8e cells in comparison with the p53 expression in SKOV-3 cells. NT8e is a p53 mutant cell line which contains endogenous mutant p53. Therefore, this cell shows two bands for p53, i.e., one for endogenous p53 and another for exogenous p53 protein. The relatively less expression in lane 3 was of PEI25 which was used as positive control. The naked plasmid p53 (lane 2) and only saline solution, i.e., no treatment were used as negative controls. It should be noted that an equal loading of protein samples was clearly seen from β tubulin expression in both the cell lines.
Biodistribution studies
The fluorescence imaging uses an external light source to excite a variety of fluorescent compounds, the most common of which is green fluorescent protein (GFP). Structurally, GFP is a beta barrel with a centrally located tripeptide sequence undergoing cyclization reactions in the presence of oxygen to serve as the chromophore (Yang et al. Citation1996). The chromophore emits green light at a wavelength of 509 nm when exposed to an excitation peak of 395 nm (blue light). Imaging with GFP has previously been used to study the gene expression in hESCs (Vallier et al. Citation2004). Although bioluminescence is favored for gene expression over fluorescence as far as organ depth is concerned, using longer wavelength fluorescent proteins may improve tissue penetration depth.
Results of in vivo imaging of mice treated with nanoplexes of PEI2-ChA without transferrin () and with transferrin () are shown in . Control mice () were treated only with 150 mM saline. The epi-fluorescene scale showed the highest fluorescent intensity signal appears as yellow color in all the images. The red color is either autofluorescence or weak GFP signal. It can be clearly seen that animals injected intravenously with transferrin containing nanoplexes prepared with PEI2 conjugates (PEI2-ChA-Tf) showed localized yellow colored signal (highest GFP intensity) in brain region (). It should be noted that the GFP signal was spotted only in brain region which implied that it was a GFP signal and not an autofluorescence. Moreover, autofluorescence was associated with red color which was not the case observed in the brain. The animals injected with the nanoplexes without transferrin prepared with PEI2 conjugates (PEI2-ChA-Tf) showed strong GFP expression in lung region (), but weak GFP signals in lung area (). Systemic administration of non-shielded luciferase reporter gene complexes into tumor-bearing mice resulted in high gene expression in the lungs, lower expression in other organs, such as the heart and liver, but was often accompanied by considerable toxicity. Particularly, when high molecular weight PEI800/DNA complexes were used, approximately half of the animals died with signs of lung embolism (Kircheis et al. Citation1999). Complexes using the lower molecular weight PEIs (e.g., 25 kDa or22kDa) showed generally lower toxicity, particularly when the linear PEI22 was used (Goula et al. Citation1998, Wightman et al. Citation2001). With all PEIs, lung expression was prominent with varying expression levels in other major organs or the tumor. In contrast, transferrin-containing nanoplexes (shielded by high density Tf) resulted in preferable reporter gene expression in brain region.
Figure 11. In vivo imaging of mice treated with nanoplexes of PEI2-ChA without transferrin (A, B, and C) and with transferrin (D, E, and F). Control mice (G) were treated only with 150 mM saline. H and I are images for organs isolated from animals treated with and without transferring, respectively.
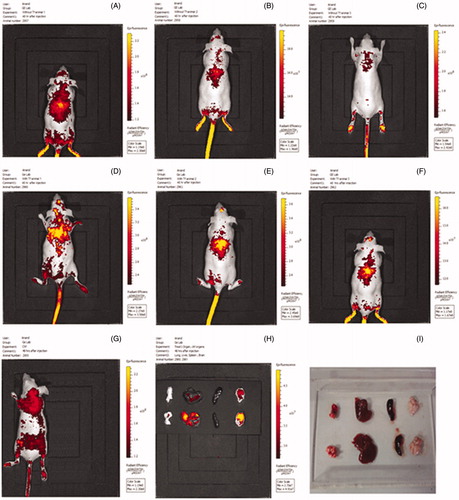
Figure 12. Treatment with wild-type plasmid p53 induces regression of ectopic solid tumors. Pictures showing tumor volume of untreated/control mice (B) and mice treated with transferring-containing nanoplexes of PEI2-ChA with plasmid p53 (A). The numbers in the pictures denotes in-house animal numbers for identification and are unique for each animal.
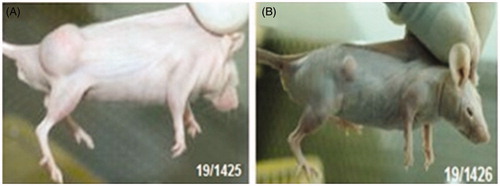
In addition to in vivo imaging, ex vivo imaging for organs isolated from animals treated with and without transferrin was also carried out as shown in , while shows the images of the same organs in plain bright light. The organs from the animals injected with transferrin containing nanoplexes clearly showed a strong signal for GFP in brain, whereas only weak signal was seen in brain and lung for the nanoplexes without transferrin. Thus, it can be concluded that transferrin-containing nanoplexes have the ability to carry therapeutic gene to brain region and thereby in brain tumors.
Tumor regression studies
Tumor regression is an improvement or cure from a disease that appears to be progressing in its severity. The tumor regression could occur by apoptosis (programmed cell death) and/or by anti-angiogenic effects (growth of new blood vessels) of therapeutic agents. Treatment with wild-type plasmid p53 induces regression of ectopic solid tumors. shows tumor volume of untreated/control mice () and mice treated with transferrin-containing nanoplexes of PEI2-ChA with plasmid p53 (). The numbers in the pictures denote in-house animal numbers for identification and are unique for each animal. At 48 days post-tumor induction, p53-treated tumors were significantly smaller compared with control animals as shown in . The tumors of untreated mice were ∼5 times bigger in size than the tumor of animals treated with transferring-containing nanoplexes of PEI2-ChA ( and ).
Figure 13. Tumor size of two groups (termed as control and treated) after tumor induction (A) and after treatment (B); tumor volume expressed in cm3.
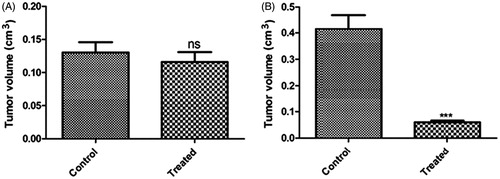
Table 2. Tumor size of control group at tumor induction and post-tumor induction (cm3).
Table 3. Tumor size of treated group before and after treatment (cm3).
shows the tumor size of two groups (termed as control and treated) after tumor induction (cm3) and shows tumor size of control and treated group after treatment (p < 0.001). It was shown in the cell-cycle analysis that the cell kill was majorly due to apoptosis of cancerous cells, which contributed to the regression of tumors. Thus, it can be concluded that the cholic acid modified PEI2 conjugates (i.e., PEI2-ChA) can be effectively used towards regression of brain tumors in humans after exhaustive preclinical studies are carried out.
Conclusions
The modified PEIs demonstrated better stability and transfection efficiency as compared with their unmodified counterparts. This paves the path for further research on PEI-based non-viral gene delivery system with the aim of decreasing inherent toxicity of PEI along with enhanced gene transfection efficiency. The use of transferrin as targeting ligand could further localize the nanoplexes inside brain leading to better therapeutic efficacy in brain tumors. The ability of Tf incorporated nanoplexes of PEI2-ChA to express the gene of interest localized in the brain region and their ability of tumor regression with p53 in xenograft model of nude mice makes them promising delivery system for gene delivery to the brain warranting exhaustive animal studies in order to optimize their therapeutic potential.
Acknowledgements
The authors acknowledge Faculty of Pharmacy, The M.S. University of Baroda and ACTREC, Mumbai for providing the facilities to carry out the research work.
Disclosure statement
No conflict of interest.
References
- Bahadur K, Landry CB, Aliabadi H, Lavasanifar M, Uludag AH. 2011. Lipid substitution on low molecular weight (0.6–2.0 kDa) polyethylenimine leads to a higher zeta potential of plasmid DNA and enhances transgene expression. Acta Biomater. 7:2209–2217.
- Calzolari A, Luigi ML, Robert P. 2010. Transferrin receptor 2 is frequently and highly expressed in glioblastomas. Transl Onclol. 3:123–134.
- Caysa H, Hoffmann S, Luetzkendorf J, Mueller LP, Unverzagt S, Mäder K, Mueller T. 2012. Monitoring of xenograft tumor growth and response to chemotherapy by non-invasive in vivo multispectral fluorescence imaging. PLoS One. 7:e47927.
- CharlieMing Y, Hasan HU. 2012. Nucleic-acid based gene therapeutics: delivery challenges and modular design of nonviral gene carriers and expression cassettes to overcome intracellular barriers for sustained targeted expression. J Drug Target. 20:301–328.
- Chen H, Nan W, Wei X, Wang Y, Lv F, Tang H, et al. 2016. Toxicity, pharmacokinetics, and in vivo efficacy of biotinylated chitosan surface-modified PLGA nanoparticles for tumor therapy. Artif Cells Nanomed Biotechnol. [Epub ahead of print].
- Chiu SJ, Liu S, Perrotti D. 2006. Efficient delivery of a Bcl-2-specific antisense oligodeoxyribonucleotide (G3139) via transferrin receptor-targeted liposomes. J Control Rel. 112:199–207.
- Clements BA, Hsu CY, Kucharski C, Lin X, Rose L, Uludağ H. 2009. Nonviral delivery of basic fibroblast growth factor gene to bone marrow stromal cells. Clin Orthop Relat Res. 467:3129–3137.
- Dube B, Rose L, Sawant K, Uludag H. 2013. Cholic acid modified 2 kDa polyethylenimine as efficient transfection agent. Biotechnol Prog. 29:1337–1341.
- Finkel E. 1999. Does cancer therapy trigger cell suicide. Science. 286:2256–2258.
- Fragkos M, Beard P. 2011. Mitotic catastrophe occurs in the absence of apoptosis in p53-null cells with a defective G1 checkpoint. PLoS One. 6:e22946.
- Geldenhuys W, Mbimba T, Bui T, Harrison K, Sutariya V. 2011. Brain-targeted delivery of paclitaxel using glutathione-coated nanoparticles for brain cancers. J Drug Target. 19:837–845.
- Goula D, Benoist C, Mantero S, Merlo G, Levi G, Demeneix BA. 1998. polyethylenimine-based intravenous delivery of transgenes to mouse lung. Gene Ther. 5:1291–1295.
- Gratton SA, Ropp PA, Pohlhaus PD, Luft JC, Madden VJ, Napier ME. 2008. The effect of particle design on cellular internalization pathways. Proc Natl Acad Sci USA. 105:11613–11618.
- Grzelczak M, Vermant J, Furst EM, Liz-Marzan LM. 2010. Directed self-assembly of nanoparticles. ACS Nano. 4:3591–3605.
- HosseiniHaji-Fatahaliha M, Jadidi-Niaragh M, Majidi F, Yousefi JM. 2016. The use of nanoparticles as a promising therapeutic approach in cancer immunotherapy. Artif Cells Nanomed Biotechnol. 44:1051–1061.
- Ikonen M, Murtomäki L, Kontturi K. 2008. Controlled complexation of plasmid DNA with cationic polymers: effect of surfactant on the complexation and stability of the complexes. Colloids Surf B Biointerfaces. 66:77–83.
- Jing F, Li D, Xu W, Liu Y, Wang K, Sui Z. 2014. Transferrin- and folate-modified, double-targeted nanocarriers for gene delivery. Pharm Biol. 52:570–574.
- Joshee N, Bastola DR, Cheng PW. 2002. Transferrin-facilitated lipofection gene delivery strategy: characterization of the transfection complexes and intracellular trafficking. Gene Ther. 13:1991–2004.
- Khalvati B, Sheikhsaran F, Sharifzadeh S, Kalantari T, Behbahani AB, Jamshidzadeh A, Dehshahri A. 2016. Delivery of plasmid encoding interleukin-12 gene into hepatocytes by conjugated polyethylenimine-based nanoparticles. Artif Cells Nanomed Biotechnol. 1–9. DOI: 10.1080/21691401.2016.1202256
- Kircheis R, Schüller S, Brunner S, Ogris M, Heider KH, Zauner W, Wagner E. 1999. Polycation-based DNA complexes for tumor-targeted gene delivery in vivo. J Gene Med. 1:111–120.
- Klausner RD, Harford J, Van RJ. 1984. Rapid internalization of the transferrin receptor in K562 cells is triggered by ligand binding or treatment with a Phorbol ester. Proc Natl Acad Sci USA. 81:3005–3009.
- Kopatz I, Remy JS, Behr JP. 2004. A model for non-viral gene delivery: through syndecan adhesion molecules and powered by actin. J Gene Med. 6:769–776.
- Kutlu C, Çakmak AS, Gümüşderelioğlu M. 2014. Double-effective chitosan scaffold-PLGA nanoparticle system for brain tumour therapy: in vitro study. J Microencapsul. 31:700–707.
- Lemkine GF, Demeneix BA. 2001. Polyethylenimines for in vivo gene delivery. Curr Opin Mol Ther. 3:178–182.
- Levine AJ. 1997. P53, the cellular gatekeeper for growth and division. Cell. 88:323–331.
- Lian J, Xin Z, Ming L, Yan D, Nongyue H. 2014. Current progress in gene delivery technology based on chemical methods and nano-carriers. Theranostics. 4:240–255.
- LiuCong J, Thomas LE, Ahlborn TE, Michael J, Lou SM, Linda MB. 1999. The expression of p53 tumor suppressor gene in breast cancer cells is down-regulated by cytokine oncostatin M1. Cell Growth Differ. 10:677–683.
- Meier P, Vousden KH. 2007. Lucifer's Labyrinth – ten years of path finding in cell death. Mol Cell. 28:746–754.
- Mulherkar R, Goud AP, Wagle AS, Naresh KN, Mahimkar MB, Thomas SM. 1997. Establishment of a human squamous cell carcinoma cell line of the upper aero-digestive tract. Cancer Lett. 118:115–121.
- Mulherkar R. 2010. Gene and cell therapy in India. Curr Sci. 99:1542–1548.
- Murk JLAN, Humbel BM, Ziese U, Griffith JM, Posthuma G, Slot JW, et al. 2003. Endosomal compartmentalization in three dimensions: implications for membrane fusion. PNAS. 100:13332–13337.
- Nakase M, Madoka I, Kenya O, Takahiko K, Shinnosuke N, Toshiro T. 2005. p53 gene therapy of human osteosarcoma using a transferrin-modified cationic liposome. Mol Cancer Ther. 4:625–631.
- Neu M, Sitterberg J, Bakowsky U, Kissel T. 2006. Stabilized nanocarriers for plasmids based upon cross-linked poly(ethyleneimine). Biomacromolecules. 7:3428–3438.
- Ogris M, Steinlein P, Kursa M, Mechtler K, Kircheis R, Wagner E. 1998. The size of DNA/transferrin-PEI complexes is an important factor for gene expression in cultured cells. Gene Therapy. 5:1425–1433.
- Pandey AP, Girase NM, Patil MD, Patil PO, Patil DA, Deshmukh PK. 2014. Nanoarchitectonics in cancer therapy and imaging diagnosis. J Nanosci Nanotechnol. 14:828–840.
- Pandey AP, Sawant KK. 2016. Polyethylenimine: a versatile, multifunctional non-viral vector for nucleic acid delivery. Mater Sci Eng C Mater Biol Appl. 68:904–918.
- Petersen H, Fechner PM, Martin AL, Kunath K, Stolnik S, Roberts CJ, et al. 2002. Polyethylenimine-graft-poly(ethylene glycol) copolymers: influence of copolymer block structure on DNA complexation and biological activities as gene delivery system. Bioconjug Chem. 13:845–854.
- Sang-SooAntonina K, Farwah RR, Abhi KR, Michael CK, Kathleen F, Shangzi PW, et al. 2014. The clinical potential of targeted nanomedicine: delivering to cancer stem-like cells. Mol Therapy. 22:278–291.
- Tripathi RM, Shrivastav A, Shrivastav BR. 2015. Biogenic gold nanoparticles: as a potential candidate for brain tumor directed drug delivery. Artif Cells Nanomed Biotechnol. 43:311–317.
- Vader P, Leonardus JA, Schiffelers RM. 2012. Physicochemical and biological evaluation of siRNA polyplexes based on PEGylated poly(amido amine)s. Pharm Res. 29:352–361.
- Vallier L, Rugg-Gunn PJ, BouhonI A, Andersson FK, Sadler AJ, Pedersen RA. 2004. Enhancing and diminishing gene function in human embryonic stem cells. Stem Cells. 22:2–11.
- Vogelstein B, Kinzler KW. 1992. p53 function and dysfunction. Cell. 70:523–526.
- Wang Y, Mostafa NZ, Hsu CM, Rose L, Kucharki C, Yan J, et al. 2013. Modification of human BMSC with nanoparticles of polymeric biomaterials and plasmid DNA for BMP-2 secretion. J Surg Res. 183:8–17.
- Wankhede M, Bouras A, Kaluzova M, Hadjipanayis CG. 2012. Magnetic nanoparticles: an emerging technology for malignant brain tumor imaging and therapy. Expert Rev Clin Pharmacol. 5:173–186.
- Wightman L, Kircheis R, Roessler V, Carotta S, Ruzicka R, Kursa M, Wagner E. 2001. Different behavior of branched and linear polyethylenimine for gene delivery in vitro and in vivo. J Gene Med. 3:362–372.
- Xu G, Wen X, Hong Y, Du H, Zhang X, Song J, et al. 2011. An anti-transferrin receptor antibody enhanced the growth inhibitory effects of chemotherapeutic drugs on human glioma cells. Int Immuno Pharmacol. 11:1844–1849.
- Xue W, Zender L, Miething C, Dickins RA, Hernando Krizhanovsky E, Lowe SW. 2007. Senescence and tumour clearance is triggered by p53 restoration in murine liver carcinomas. Nature. 445:656–660.
- Yang F, Moss LG, Phillips GN Jr, 1996. The molecular structure of green fluorescent protein. Nat Biotechnol. 14:1246–1251.
- Yoshiyuki Tomoko K, Hidetoshi I, Akihiko M, Tatsuya T, Nobuaki O, Haruhiko Y, Takuro AN. 2003. Novel poly(ethylene glycol) derivatives with carboxylic acid pendant groups: synthesis and their protection and enhancing effect on non-viral gene transfection systems. J Biomater Sci Polym Edn. 14:515–531.
- Zheng M, Zhong Y, Meng F, Peng R, Zhong Z. 2012. Lipoic acid modified low molecular weight polyethylenimine mediates nontoxic and highly potent in vitro gene transfection. Mol Pharm. 8:2434–2443.