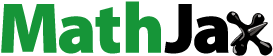
Abstract
Zinc oxide nanoparticles (ZnO NPs) were synthesized using Ziziphus nummularia leaf extract. The characterization was done by various spectral analysis and antifungal (anti-candidal) activity against multidrug resistant clinical isolates and their cytotoxic potential was evaluated. The ZnO NPs were 17.33 nm in size and were spherical/irregular in shape. The antifungal activity of ZnO NPs was better than four standard azole antibiotics and they also showed potent cytotoxic effect against HeLa cancer cell line. The results strongly suggest the applicability of green synthesized ZnO NPs as antifungal agent and also its use in cancer diagnosis and treatment.
Introduction
Nanotechnology is a young and modern discipline of science which is one of the fastest emerging research fields. Nanotechnology is all about construction of novel nanostructures, discovering and understanding their novel properties and learning how to organize these new nanostructures into larger and more complex functional structures and devices. Nanoparticles are produced from many metals like silver, gold, zinc, copper, iron, titanium, etc., by various physical and chemical methods. However, synthesis of nanoparticles from noble metals is more popular since nanoparticles of noble metals are extensively used in numerous fields such as biomedical sciences, electronics, optics, chemical industry, drug-gene delivery, environment cosmetics, energy saving, catalysis, etc. (Castro et al. Citation2014, Kathiravan et al. Citation2014). This extensive usage is because of their physical and chemical properties different from bulk materials of which they are made of.
Although synthesis can be attempted by any method, green synthesis is gaining much importance over physical and chemical synthetic methods, since the former method possess many advantages such as requires mild reaction conditions, does not involve any toxic chemicals, cost effective and ecofriendly while later methods are expensive with many disadvantages such as requirement of high temperature and pressure, costly, toxic chemicals are evolved into the environment making it harmful, etc. (Atarod et al. Citation2016, Govarthanan et al. Citation2016, Parashar et al. Citation2009). Hence, green synthesis is very desirable. Phytochemicals present in plant extracts serves as reducing, stabilizing and capping agents for the synthesis of nanoparticles (Park et al. Citation2011).
Zinc is an essential element for microorganisms and higher organisms as it is involved in many vital cellular reactions. It acts as cofactor for many catalytic and structural activities. It possesses antimicrobial properties and can act as antibacterial or antifungal agent. Zinc oxide nanoparticles (ZnO NPs) have unique optical properties which make them good candidates for various medical applications like drug delivery, biosensors and imaging techniques (Zhang et al. Citation2013). They have excellent interaction with electro-magnetic waves and possess electronic properties (Ko et al. Citation2013). Another interesting property of ZnO NPs is their antimicrobial activity. They exhibit antimicrobial activity against many microorganisms as reported by several researchers (Applerot et al. Citation2012, Dwivedi et al. Citation2014, Sangani et al. Citation2015, Velmurugan et al. Citation2016b).
Candida spp. is the most common opportunistic fungal pathogen of humans; they occur very frequently as a hospital-acquired infection and are the fourth leading cause of nosocomial infection (Richards et al. Citation2008). Candida albicans is the predominant cause of virtually all types of Candidiasis (Filler and Sheppard Citation2006). Non-albican species include C. tropicalis, C. glabrata, C. parapsilosis. C. glabrata is the second or third most common cause of Candidiasis after C. albicans. Fungal resistance to existing antibiotics is a major concern of people now-a-days. Fungal infections which were earlier very effectively cured by antifungal agents are becoming futile as the fungal pathogens are becoming resistant which necessitates discovering new ways or new substances to treat them. Cancer is characterized by invasive and uncontrolled cell division and the spread of abnormal cells (Gan et al. Citation2003). Every year thousands of people worldwide die from different types of cancer. Chemotherapy and radiotherapy are most common treatments of cancer but they evoke many serious side effects (De Santis et al. Citation2014). The cytotoxic agents or cancer drugs are very few and are not very efficient (Kim et al. Citation2007). Alternative treatment options are very less. Medicinal plants have always been used as natural remedy for many ailments and they have been a boon for many diseases and disorders. Nanoparticles synthesizing using medicinal plants extracts further enhances their efficacy as natural drugs many folds. In the present work, we report for the first time synthesis of ZnO NPs from leaves of Ziziphus nummularia. Synthesis of ZnO NPs from various plant parts is reported for e.g. Awwad et al. (Citation2014) reported from Olea europea leaf extract, Anbukkarasi et al. (Citation2015) reported from Emblica officinalis fruit extract, Mishra and Sharma (Citation2015) reported from peel extract of Punica granatum, Nagajyothi et al. (Citation2015) reported from Polygala tenuifolia root extract, Velmurugan et al. (Citation2016a) reported from Pinus densiflora young cones extract, etc.
Ziziphus nummularia (family: Rhamnaceae) is a thorny small shrub, grows abundantly in arid areas of India. The decoction of leaves of Z. nummularia is used for the treatment of cough cold and typhoid. Paste of leaves is used for healing of cuts and cutaneous disease (Meena and Yadav Citation2010). Ziziphus nummularia is known to possess various pharmacological activities like antipyretic, antinociceptive, anti-inflammation (Rauf et al. Citation2016), analgesic and anti-inflammatory (Goyal et al. Citation2013), antioxidant activity (Dureja and Dhiman Citation2012), etc.
Considering the aforesaid, in the present work an attempt was made to synthesize ZnO NPs using Ziziphus nummularia leaf extract. The characterization was done using various spectral analyses. The antifungal activity of ZnO NPs against three standard fungal strains was evaluated by minimum inhibitory concentration and time kill assay. The antifungal activity was also evaluated against clinical isolate of Candida spp. The cytotoxic effect was evaluated by MTT assay against HeLa cancer cell line.
Materials and methods
Plant material and chemicals
Fresh leaves of Ziziphus nummularia (Burm.f.) Wight & Arn were collected from botanical garden of Saurashtra university, Rajkot, Gujarat, India. All the chemicals were obtained from Hi Media Laboratories Pvt. Limited, Mumbai, India. Ultra purified water was used for all the experiments.
Preparation of the plant extract
Fresh leaves of Ziziphus nummularia were thoroughly washed with tap water followed by Ultra purified water and dried under shade. The dried plants were homogenized to fine powder and stored in air tight bottles. Five grams of leaf powder was taken in a 250 ml glass beaker along with 100 ml ultra-purified water and placed it on a magnetic stirrer heater at a temperature of 80 °C for 1 h. The extract was cooled to room temperature and filtered through Whatman filter paper No. 1. The filtered extract was used for the synthesis of ZnO NPs.
Synthesis of zinc oxide nanoparticles
Fifty milliliters of leaf extract was taken in a beaker and heated at 80 °C on a magnetic stirrer heater. Five grams of zinc nitrate was added to the leaf extract when the temperatures reached 80 °C. The mixture was heated until it was reduced to a deep yellow color paste. The paste was collected in a ceramic crucible and heated in a Muffle Furnace at 400 °C for 2 h. A light yellow colored powder of ZnO NPs was obtained which was carefully collected and stored at 4 °C for further analysis.
Characterization of the synthesized zinc oxide nanoparticles
The UV-Vis spectra of synthesized nanoparticles were recorded on spectrophotometer (Shimadzu UV-1601) in 300–700 nm range operated at a resolution of 20 nm. The thermal stability of nanoparticles was determined by Thermogravimetric-Differential thermal analysis (TGA-DTA). TGA measurement was made on DTG-60H (Shimadzu). TGA spectra were recorded at heating rate of 10 °C/min under nitrogen atmosphere. For investigation of nanoparticles associated molecules, Fourier Transform Infra-Red spectroscopy measurements were done. Nanoparticles were mixed with potassium bromide (KBr) and pressed into a pellet. The pellet was placed into the sample holder and spectra were recorded in the range of 400–4000 cm−1 Nicolet IS10 (Thermo Scientific, USA). The various modes of vibrations were identified and assigned to determine the different functional groups present in the Ziziphus nummularia leaf extract. The structure composition and nature of synthesized nanoparticles was analyzed by X-Ray Diffraction (XRD). X-ray powder diffraction is a rapid analytical technique used for phase identification of crystalline material. The X-Ray diffractometer (PAN analytical BV) operated at a voltage of 40 kV and a current of 30 mA with Cu Kα radiation in θ- 2θ configurations. The crystallite domain size was calculated from the width of the XRD peaks, assuming that they are free from non-uniform strains, using the Scherrer formula. D= 0.94 λ/β Cos θ, where D is the average crystallite domain size perpendicular to the reflecting planes, λ is the X-ray wavelength, β is the full width at half maximum and θ is the diffraction angle. Transmission Electron Microscopy (TEM) analysis was done to visualize the shape as well as to measure the diameter of the nanoparticles. The sample was dispersed in double distilled water. A drop of thin dispersion was placed on a “staining mat”. Carbon coated copper grid was inserted into the drop with the coated side upwards. After about ten minutes, the grid was removed and air dried. Then screened in JEOL JEM 2100 Transmission Electron Microscope.
Antifungal activity
Antifungal activity was evaluated against three standard fungal strains (Candida albicans ATCC2091, Candida glabrata NCIM3448, Cryptococcus neoformans ATCC34664) by minimum inhibitory concentration and time kill assay. The standard fungal strains were obtained from National Chemical Laboratory, Pune, India and were maintained at 4 °C.
Determination of minimum inhibitory concentration (MIC)
Antifungal activity of ZnO NPs and leaf extract was tested by Resazurin microtiter (REMA) plate method with some modifications (Palomino et al. Citation2002) against three standard fungal strains. The inoculum of the test fungi was prepared using the colony suspension method (EUCAST Citation2003). Ninety-six-well culture plates (Tarsons Products Pvt. Ltd.) were used and two-fold serial dilutions of the ZnO NPs and leaf extract (10–0.019 mg/ml) were dispensed into the plate wells. Two-fold dilutions of standard antibiotic Amphotericin B (10–0.019 mg/ml) were used as a positive control. The volume of dispensing nanoparticles was 20 μl per well along with 150 μl of Sabouraud Dextrose Broth (SDB). Thirty microliters of fungal culture at a density of 6 × 105 CFU/ml was added to the wells. Three control wells were maintained for each test batch; the positive control (antibiotic, SDB and test organism) and sterility control (SDB and water) and negative control (SDB, test organism and water). The plates were incubated at 28 °C for 48 h. The antifungal activity in the test wells was detected by adding 50 μl of 0.01% w/v of Resazurin (Himedia, India) solution dissolved in sterile distilled water to each well. The plates were incubated for further 2 h and estimated visually for any change in blue to pink color indicating reduction of the dye due to fungal growth. The lowest concentration (highest dilution) of the nanoparticles required to inhibit visible growth of the tested fungi was designated as the MIC value.
Determination of the time kill curve
Time kill assay was performed as described by Ernst et al. (Citation2002) with some modifications. Flask containing 15 ml of Sabouraud Dextrose Broth were inoculated with equal volumes of the diluted inoculums of each test fungal strain having optical density 0.2 at 600 nm, which is equivalent to 6 × 108 CFU ml−1 (EUCAST Citation2003) and were exposed to 1 × the MIC of the ZnO NPs and standard antibiotic Amphotericin B. Control experiment without antimicrobial agent (i.e. a fungal growth curve) was conducted simultaneously with the time kill studies. The flasks were incubated at 28 °C in an orbital shaking incubator at 150 rpm for 48 h. Aliquots were removed at 0, 4, 8, 24, 28, 32, 36 and 48 h and optical density was measured at 600 nm. A graph of time verse absorbance (OD at 600 nm) was plotted for the growth curve and the effect of ZnO NPs on fungal growth was recorded at 0, 4, 8, 24, 28, 32, 36 and 48 h.
Antifungal activity against clinical isolates of Candida spp
The antifungal activity was also evaluated against fifteen clinical isolates of Candida spp. (C1–C15) by agar well diffusion method. The clinical strains of were collected from various microbiological laboratories of Rajkot, Gujarat, India. Most of the strains were isolated from patients with skin infections and some from urine sample. The isolates were cultured on Sabouraud dextrose agar medium with chloramphenicol under aerobic conditions at 28 °C. The isolates were identified as Candida species on the basis of some biochemical tests like Blastospore/Chlamydospore formation, color of colony on HiChrome Candida differential agar, carbohydrate assimilation test (sucrose, mannose, lactose, malate,) and negative absorption (urea and nitrate).
In vitro antifungal activity of ZnO NPs was determined by agar well diffusion assay against 15 clinical strains of Candida spp. by the method as described earlier (Perez et al. Citation1990). Five different concentrations of ZnO NPs (25, 50, 75, 100 and 125 mg/ml) in 100% DMSO was used for evaluating their antifungal potential.
Antibiotics used in the study
The antibiotics Clotrimazole (10 mcg/disc), Fluconazole (10 mcg/disc), Itraconazole (30 mcg/disc) and Ketoconazole (30 mcg/disc) were used antifungal susceptibility test determined by disc diffusion method (Rakholiya and Chanda Citation2012). All the antibiotics were purchased from Hi Media Laboratories Pvt. Limited, Mumbai, India.
In vitro cytotoxic activity
Cancer cell viability of the ZnO NPs and leaf extract was evaluated by the MTT (3-[4,5-dimethylthiazol-2-yl]-2,5-diphenyltetrazolium bromide) assay (Labieniec and Gabryelak Citation2003). 150 μl of HeLa cells were seeded in 96-well plates (Becton Dickinson (BD), USA) at the density of 4 × 104 viable cells/well (HeLa) and incubated 24 h to allow cell attachment. Following attachment, the medium was replaced with complete medium (150 μL/well) containing the ZnO NPs and leaf extract concentrations ranging from 2–200 μg/ml for 72 h. Following treatment, the cells were washed with PBS and incubated with 100 μl/well fresh medium containing 0.5 mg/ml MTT. The MTT-containing medium was removed after 3 h incubation in dark condition. The MTT formazan was dissolved in 100 μl/well. DMSO and optical density was determined at 570 nm using an ELISA plate reader (Bio-Tek, USA). Cell viability was calculated by the following equation:
where, As is the absorbance of the cells incubated with ZnO NPs and leaf extract, and Acontrol is the absorbance of the cells incubated with the culture medium only.
Statistical analysis
All the experiments were done in triplicate. Results are reported as Mean ± SEM (Standard Error of Mean).
Results and discussion
Characterization of ZnO NPs
UV-Vis spectroscopy
The optical property of nanoparticles is one of the important aspects for the characterization of its structure and properties. The UV–Visible absorption spectrum of the synthesized ZnO NPs is given in . The maximum and narrow absorption peak of ZnO NPs was observed at 370 nm. Zinc oxide is known to exhibit absorption in the range of 360–370 nm. Jayaseelan et al. (Citation2012) also reported the absorption peak of ZnO NPs at 374 nm due to surface plasmon resonance. The results confirmed the synthesis of ZnO NPs from the leaf extract of Z. nummularia.
Thermal gravimetric analysis (TGA)
TGA was used to assess the relative composition of the organic capping agents present on the surface of nanoparticles. TGA curve of synthesized ZnO NPs is given in . TGA curve showed steadily weight loss in temperature range 0–800 °C. The TGA analyses of ZnO NPs revealed 2.69% weight loss at about 100 °C which is attributed to desorption or removal of moisture and 21.15% weight loss of its total weight at 700 °C indicating a thermal decomposition of plant bioorganic molecules present on the surface of ZnO NPs. Similar, thermal decomposition of ZnO NPs was reported by Prashanth et al. (Citation2015) and Khalil et al. (Citation2014). At temperatures above 800 °C there was no weight loss in the TGA curve, indicating that the ZnO NPs were stable within this temperature range.
Fourier transform infra-red spectroscopy (FTIR)
FTIR spectroscopy is used to identify the functional groups based on the peak value in the infra-red region. FTIR spectroscopy were carried out to identify the potential functional groups of the biomolecules present in the Z. nummularia leaf extract which is responsible for the reduction and stabilization of ZnO NPs. FTIR spectra showed intense peak at 3331.07, 1537.27, 1367.53, 1055.06, 1026.13 and 804.32 cm−1 (). The broad and intense peak observed at 3331 cm−1 exhibited the presence of O–H stretching of alcohol or phenol group. Similar band pattern has been reported by Sharma (Citation2016) for the ZnO nano-flowers synthesized by Carica papaya latex. The absorption band at 1537 cm−1 and 1367 cm−1 could be attributed to bending vibrations of N–H in secondary amines of proteins and C–H stretching of alkynes, respectively. The sharp narrow peak observed at 1055 and 1026 cm−1 exhibited the presence of C–N stretching of aliphatic amines. The peaks at 549 and 497 cm−1 are corresponding to ZnO NPs stretching and deformation, respectively, which confirmed the presence of metal oxygen and peak representing crystalline ZnO NPs around 437 cm−1 (Markova-Deneva Citation2010).
FTIR peak indicate the involvement of polyphenol, flavonoid, terpenoids and proteins having functional groups of secondary amines, phenol, aliphatic amines and alcohols in reduction reactions. Our results are in accordance to the observations of Elumalai and Velmurugan (Citation2015), who reported the role of phenolic acids, flavonoids, terpenoids and protein in synthesis and stability of ZnO NPs synthesized from Azadirachta indica. The FTIR result suggested that the phytoconstituents present in Z. nummularia leaf extract acted as capping and stabilizing agent and also prevented the aggregation of ZnO NPs.
X-ray diffraction analysis (XRD)
The phase identification of crystalline structure of the ZnO NPs was characterized by X-ray diffraction. X-ray diffraction pattern of ZnO NPs is given in . The ZnO NPs showed strong diffraction peak with 2Ø values of 31.66°, 34.34°, 36.16°, 47.40°, 56.46°, 62.78°, 66.42°, and 69.02° corresponding to the crystal planes of (100), (002), (101), (102) (110), (103), (200), (112) and (201), respectively. All the diffraction peaks were indexed to hexagonal wurtzite structure of ZnO NPs by comparison with the data from JCPDS card No.36–1451 (Chen Citation2000). Ali et al. (Citation2016) reported that the peaks were recognized as (1 0 0), (0 0 2), (1 0 1), (1 0 2), (1 1 0), (1 0 3), (2 0 0), (1 1 2) and (2 0 1) reflections, in ZnO NPs synthesized using leaf extract of Aloe vera. The sharp, strong and narrow diffraction peaks in the XRD pattern indicated that the synthesized ZnO NPs were crystalline in nature.
Transmission electron microscopy analysis (TEM)
The size and morphology of the ZnO NPs characterized by TEM analysis at differences magnification is given in . The TEM image revealed the size of ZnO NPs to be in the range of 12.47 nm to 26.97 nm and average size of nanoparticle was 17.33 nm. ZnO NPs were predominantly spherical and irregular in shape with well-defined morphology. The size of synthesized ZnO NPs was smaller than previously reported ZnO NPs synthesized by Aloe barbadensis and Citrus aurantifolia extracts; size was in the range of 25 to 40 nm and 50 to 200 nm respectively (Samat and Nor Citation2013, Sangeetha et al. Citation2011). The Selected Area Electron Diffraction (SAED) pattern of ZnO NPs is given in . The ring-like diffraction pattern in SAED image indicates that particles are purely crystalline in nature with symmetrical orientation of ZnO NPs. Similar diffraction pattern in SAED image of ZnO NPs was reported by Khalil et al. (Citation2014).
Antifungal activity
Antifungal activity of Z. nummularia leaf extract, synthesized ZnO NPs and standard antibiotic Amphotericin B was evaluated by minimum inhibitory concentration and time kill assay against three fungi viz. C. albicans, C. glabrata and C. neoformans. The MIC values of leaf extract was >10 mg/ml for all the three fungi while that of standard antibiotic Amphotericin B was 5 mg/ml. In comparison to these values, the MIC values of ZnO NPs was very much less viz. the MIC values of C. albicans and C. glabrata was 1.25 mg/ml while that of C. neoformans was 10 mg/ml (. Thus, it can be seen that ZnO NPs exhibited very good antifungal activity, even better than standard antibiotic Amphotericin B and leaf extract.
The effect of ZnO NPs and the standard antibiotic on the growth of three fungi C. albicans, C. glabrata and C. neoformans at 1 × MIC concentration was investigated. The growth curve pattern is depicted in . All the three fungal strains treated with ZnO NPs showed alterations in their growth curve pattern; lag phase was extended and distinct growth reduction was observed on treatment with ZnO NPs as compared to growth control and antibiotic Amphotericin B. This difference was observed till 48 h and the difference was maximum at 48 h. ZnO NPs showed distinct enhanced fungicidal effect as compared to standard antibiotics Amphotericin B against fungi.
The increased fungicidal activity of ZnO NPs in the present study may be attributed to the small size of synthesized ZnO NPs. Size-dependent antifungal activity of ZnO NPs is also reported by Rajiv et al. (Citation2013) against plant fungal pathogens. Correlation between size of the particles and antimicrobial activity is reported by other researchers also (Jeong et al. Citation2014, Raghupathi et al. Citation2011). Another reason for the antifungal activity of ZnO NPs may be the formation of reactive oxygen species which changes membrane permeability which leads to the release of membrane lipids and proteins, eventually leading to microbial cell death (Brayner et al. Citation2006, Hameed et al. Citation2015).
Antifungal activity against clinical isolates of Candida spp
The present study demonstrated that ZnO NPs induce significant growth inhibition of standard fungal strains C. albicans, C. glabrata and C. neoformans. Thus, we further tested antifungal activity of ZnO NPs against clinical isolates of Candida spp. The antifungal activity was evaluated with 5 different concentrations of ZnO NPs (25, 50, 75, 100 and 125 mg/ml) and four azole drugs viz. Fluconazole, Ketoconazole, Clotrimazole and Itraconazole against clinical isolates of Candida spp. None of the four standard antibiotics inhibited any of the 15 clinical isolates (hence data not shown). On the other hand, all the 15 isolates were inhibited by one or the other concentration of ZnO NPs. The susceptibility of isolates to ZnO NPs concentration varied (). Out of 15 isolates, 7 isolates (C3, C7, C9, C10, C11, C14 and C15) were inhibited by all the five concentrations of ZnO NPs; while the other eight isolates were inhibited by three or four concentrations of ZnO NPs. Increase in antifungal activity with increase in ZnO NPs concentration against Candida albicans is also reported by Sharma and Ghose (Citation2015). All the isolates though resistant to azole drugs were inhibited by ZnO NPs indicating the potential of ZnO NPs to treat the multi drug resistant Candida infections. Infections caused by Candida spp. are difficult to treat and are associated with a high mortality rate in immuno-compromised patients. The rapid global spread of resistance in clinical isolates of Candida spp. compels a dire need to find out other strategies for development of effective antifungal therapy. Thus it appears that treatment with ZnO NPs may be a novel, natural alternative approach to treat Candidiasis.
Lipovsky et al. (Citation2011) observed the effect of particle size of ZnO NPs on viability of Candida albicans, smaller particles had better antifungal activity as compared to larger particles. The anticandidal activity of ZnO NPs was due to the intracellular production of many free radicals like hydroxyl radicals, singlet oxygen, superoxide radical, nitric oxide radical which may cross the nuclear membrane and cause DNA damage which may lead to irreversible chromosome damage or cell death (Hwang et al. Citation2012, Shoeb et al. Citation2013). It is proposed that the fungicidal activity is due to the formation of insoluble compounds by inactivation of sulfhydryl groups in the fungal cell wall and finally disruption of membrane bound enzymes and lipids which results in cell death (Jaidev and Narasimha Citation2010). The results suggested that ZnO NPs may provide a novel family of fungicidal compounds to treat the Candidiasis.
In vitro cytotoxic activity
The cell-viability assay is one of the significant parameter for toxicology study, it can give information about cell death, survival and metabolic activities and cellular responses to toxic materials (Asharani et al. Citation2009). The cell viability of the synthesized ZnO NPs and Z. nummularia leaf extract was evaluated in vitro against the HeLa cancer cell line at different concentrations (.
Ziziphus nummularia leaf extract decreased the cell viability of HeLa cancer cells (96% to 60%) with increase in the concentration of leaf extract from 2 to 200 μg/ml. On the other hand, ZnO NPs showed dose-dependent cytotoxic effect, i.e. when the concentration of the ZnO NPs increased the cell viability decreased drastically. The synthesized ZnO NPs decreased the cell viability from 89% to 39%. This clearly indicated that ZnO NPs exhibited significant higher cytotoxic effect than the leaf extract. The results suggested that higher concentrations of ZnO NPs between 50 and 200 μg/ml had significantly more impact on cell viability than of 2–30 μg/ml concentrations (. Similar dose-dependent cytotoxic effect of ZnO NPs on human liver carcinoma cells and sliver nanoparticles on HeLa cancer cells is reported by Chung et al. (Citation2015) and Moteriya and Chanda (Citation2016), respectively. In the present study, ZnO NPs showed 52% cell viability at 50 μg/ml concentrations which is significantly better than Manivasagan et al. (Citation2013) who reported 50% cell viability at 200 μg/ml concentrations for HeLa cell line. Nanoparticles-treated cells showed decreased cellular metabolic activity and membrane integrity, which depends on the nature of cell types and the size of nanoparticles (Park et al. Citation2011). Gurunathan et al. (Citation2013) suggested that nanoparticles induce ROS generation, activation of caspase 3, DNA fragmentation, membrane leakage which resulted in the cell death. Prashanth et al. (Citation2015) reported the green synthesized ZnO nanopowder showed better cytotoxic effect as compared to commercial ZnO nanopowder against breast cancer cell line MCF-7. However, the action of nanoparticles depends on size, shape, type of cells and also dose and time dependence.
Conclusion
The present study successfully demonstrated a safe, simple, eco-friendly method for the synthesis of stable ZnO NPs by Z. nummularia leaf extract. The spectroscopic characterizations using various analytical techniques confirmed the formation of ZnO NPs. UV–Vis spectrum showed the peak at 370 nm indicated the characteristic absorption peak of ZnO NPs. XRD results confirmed the crystalline nature of ZnO NPs. TEM analysis revealed the shape of ZnO NPs as spherical and irregular and size was 17.33 nm. Synthesized ZnO NPs showed potent antifungal activity against clinical isolate of Candida spp. in comparison to even conventional antibiotics. ZnO NPs displayed a pronounce cytotoxic activity against HeLa cancer cell lines. This study further proves the significance of green technology for ZnO NPs production and applications in control of various human diseases.
Acknowledgements
The authors thank Department of Biosciences (UGC-CAS) for providing excellent research facilities.
Disclosure statement
The authors report no conflicts of interest. The authors alone are responsible for the content and writing of this article.
Funding
One of the authors Ms. Hemali Padalia is thankful to UGC-CAS, New Delhi, for providing Junior Research Fellowship.
References
- Ali K, Dwivedi S, Azam A, Saquib Q, Al-Said MS, Alkhedhairy AA, Musarrat J. 2016. Aloe vera extract functionalized zinc oxide nanoparticles as nanoantibiotics against multi-drug resistant clinical bacterial isolates. J Colloid Inter Sci. 472:145–156.
- Anbukkarasi V, Srinivasan R, Elangovan N. 2015. Antimicrobial activity of green synthesized zinc oxide nanoparticles from Emblica officinalis. Int J Pharmaceu Sci Rev Res. 33:110–115.
- Applerot G, Lellouche J, Perkas N, Nitzan Y, Gedanken A, Banin E. 2012. ZnO nanoparticle coated surfaces inhibit bacterial biofilm formation and increase antibiotic susceptibility. RSC Adv. 2:2314–2321.
- Asharani PV, Mun GLK, Hande MP, Valiyaveettil S. 2009. Cytotoxicity and genotoxicity of silver nanoparticles in human cells. ACS Nano. 3:279–290.
- Atarod M, Nasrollahzadeh M, Sajadi SM. 2016. Euphorbia heterophylla leaf extract mediated green synthesis of Ag/TiO2 nanocomposite and investigation of its excellent catalytic activity for reduction of variety of dyes in water. J Colloid Inter Sci. 462:272–279.
- Awwad AM, Albiss B, Ahmad SL. 2014. Green synthesis characterization and optical properties of zinc oxide nanosheets using Olea europea leaf extract. Adv Mater Lett. 5:520–524.
- Brayner R, Ferrari-Iliou R, Brivois N, Djediat S, Benedetti MF, Fievet F. 2006. Toxicological impact studies based on Escherichia coli bacteria in ultrafine ZnO nanoparticles colloidal medium. Nano Lett. 6:866–870.
- Castro L, Blazquez ML, Munoz JA, Gonzalez FG, Ballester A. 2014. Mechanism and applications of metal nanoparticles prepared by bio-mediated process. Rev Adv Sci Eng. 3:199–216.
- Chen C. 2000. Joint committee on powder diffraction standards. Diffr Data File. 36–45.
- Chung I-M, Rahuman AA, Marimuthu S, Kirthi AV, Anbarasan K, Rajakumar G. 2015. An investigation of the cytotoxicity and caspase-mediated apoptotic effect of green synthesized zinc oxide nanoparticles using Eclipta prostrata on human liver carcinoma cells. Nanomaterials. 5:1317–1330.
- De Santis CE, Lin CC, Mariotto AB, Siegel RL, Stein KD, Kramer JL, et al. 2014. Cancer treatment and survivorship statistics, 2014. CA Cancer J Clin. 64:252–271.
- Dureja AG, Dhiman K. 2012. Free radical scavenging potential and total phenolic and flavonoid content of Ziziphus mauritiana and Ziziphus nummularia fruit extracts. Int J Green Pharm. 6:187–192.
- Dwivedi S, Wahab R, Khan F, Mishra YK, Musarrat J, Al-Khedhairy A. 2014. A reactive oxygen species mediated bacterial biofilm inhibition via zinc oxide nanoparticles and their statistical determination. Plos One. 9:1–9.
- Elumalai K, Velmurugan S. 2015. Green synthesis, characterization and antimicrobial activities of zincoxide nanoparticles from the leaf extract of Azadirachta indica (L.). Appl Surf Sci. 345:329–336.
- Ernst EJ, Roling EE, Petzold CR, Keele DJ, Kleper ME. 2002. In vitro activity of micafungin (FK-463) against Candida spp.: microdilution, time-kill, and postantifungal-effect studies. Antimicrob. Agents Chemother. 46:3846–3853.
- European Committee for Antimicrobial Susceptibility Testing (EUCAST). 2003. Determination of minimum inhibitory concentrations (MICs) of antibacterial agents by broth dilution. Clin Microbiol Infec. 9:1–7.
- Filler SG, Sheppard DC. 2006. Fungal invasion of normally non-phagocytic host cells. Plos Pathogens. 2:1099–1105.
- Gan DD, Macaluso M, Cinti C, Khalili K, Giordano A. 2003. How does a normal human cell become a cancer cell? J Exp Clin Cancer Res. 22:509–516.
- Govarthanan M, Seo YS, Lee KJ, Jung IB, Ju HJ, Kim JS, et al. 2016. Low-cost and eco-friendly synthesis of silver nanoparticles using coconut (Cocos nucifera) oil cake extract and its antibacterial activity. Artif Cells Nanomed Biotechnol. 44:1878–1882.
- Goyal M, Ghosh M, Nagori BP, Sasmal D. 2013. Analgesic and anti-inflammatory studies of cyclopeptide alkaloid fraction of leaves of Ziziyphus nummularia. Saudi J Biol Sci. 20:365–371.
- Gurunathan S, Raman J, Malek SNA, John PA, Vikineswary S. 2013. Green synthesis of silver nanoparticles using Ganoderma neo-japonicum Imazeki: a potential cytotoxic agent against breast cancer cells. Int J Nanomed. 8:4399–4413.
- Hameed ASH, Karthikeyan C, Kumar VS, Kumaresan S, Sasikumara S. 2015. Effect of Mg2+, Ca2+, Sr2+ and Ba2+ metal ions on the antifungal activity of ZnO nanoparticles tested against Candida albicans. Mater Sci Eng C. 52:171–177. (
- Hwang IS, Lee J, Hwang JH, Kim KJ, Lee DG. 2012. Silver nanoparticles induce apoptotic cell death in Candida albicans through the increase of hydroxyl radicals. FEBS J. 279:1327–1338.
- Jaidev LR, Narasimha G. 2010. Fungal mediated biosynthesis of silver nanoparticles, characterization and antimicrobial activity. Colloids Sur B. 81:430–433.
- Jayaseelan C, Rahuman AA, Kirthi AV, Marimuthu S, Santhoshkumar T, Bagavan A, et al. 2012. Novel microbial route to synthesize ZnO nanoparticles using Aeromonas hydrophila and their activity against pathogenic bacteria and fungi. Spectrochim Acta A Mol Biomol Spectro. 90:78–84.
- Jeong Y, Lim DW, Choi J. 2014. Assessment of size-dependent antimicrobial and cytotoxic properties of silver nanoparticles. Adv Mater Sci Eng. 2014:1–6.
- Kathiravan V, Ravi S, Ashokkumar S. 2014. Synthesis of silver nanoparticles from Melia dubia leaf extract and their in vitro anticancer activity. Spectrochim Acta A Mol Biomol Spectrosc. 130:116–121.
- Khalil MI, Al-Qunaibit MM, Al-zahem AM, Labis JP. 2014. Synthesis and characterization of ZnO nanoparticles by thermal decomposition of a curcumin zinc complex. Arab J Chem. 7:1178–1184.
- Kim JS, Kuk E, Yu KN, Kim JH, Park SJ, Lee HJ. 2007. Antimicrobial effects of silver nanoparticles. Nanomedicine. 3:95–101.
- Ko W, Jung N, Lee M, Yun M, Jeon S. 2013. Electronic nose based on multipatterns of ZnO nanorods on a quartz resonator with remote electrodes. ACS Nano. 7:6685–6690.
- Labieniec M, Gabryelak T. 2003. Effects of tannins on Chinese hamster cell line B14. Mutat Res. 539:127–135.
- Lipovsky A, Nitzan Y, Gedanken A, Lubart R. 2011. Antifungal activity of ZnO nanoparticles: the role of ROS mediated cell injury. Nanotechnology. 22:1–5.
- Manivasagan P, Venkatesan J, Senthilkumar K, Sivakumar K, Kim SK. 2013. Biosynthesis, antimicrobial and cytotoxic effect of silver nanoparticles using a novel Nocardiopsis sp. MBRC-1. BioMed Res Int. 2013:1–9.
- Markova-Deneva L. 2010. Infrared spectroscopy investigation of metallic nanoparticles based on copper, cobalt, and nickel synthesized through borohydride reduction method (Review). J Uni Chemical Technol Metallurgy. 45:351–378.
- Meena KL, Yadav BL. 2010. Some traditional ethnomedicinal plants of southern Rajasthan. Ind J Trad Knowledge. 9:471–474.
- Mishra V, Sharma R. 2015. Green synthesis of zinc oxide nanoparticles using fresh peels extract of Punica granatum and its antimicrobial activities. Int J Pharma Res Health Sci. 3:694–699.
- Moteriya P, Chanda S. 2016. Synthesis and characterization of silver nanoparticles using Caesalpinia pulcherrima flower extract and assessment of their in vitro antimicrobial, antioxidant, cytotoxic, and genotoxic activities. Artif Cells Nanomed Biotechnol. (In press). doi: 10.1080/21691401.2016.1261871
- Nagajyothi PC, Cha SJ, Yang IJ, Sreekanth TVM, Kim KJ, Shin HM. 2015. Antioxidant and anti-inflammatory activities of zinc oxide nanoparticles synthesized using Polygala tenuifolia root extract. J Photochem Photobiol B Biol. 146:10–17.
- Palomino JC, martin A, Camacho M, Guerra H, Swings J, Portaels F. 2002. Resazurin microtiter assay plate: simple and inexpensive method for detection of drug resistance in Mycobacterium tuberculosis. Antimicrob Agents Chemother. 46:2720–2722.
- Parashar UK, Saxena PS, Srivastava A. 2009. Bioinspired synthesis of silver nanoparticles. Dig J Nanomater Biostruct. 4:159–166.
- Park MVDZ, Neigh AM, Vermeulen JP, Fonteyne LJJ, Verharen HW, Briede JJ, Loverena HV, Jong WH. 2011. The effect of particle size on the cytotoxicity, inflammation, developmental toxicity and genotoxicity of silver nanoparticles. Biomaterials. 32:9810–9817.
- Park Y, Hong YN, Weyers A, Kim YM, Linhardt RJ. 2011. Polysaccharides and phytochemicals: a natural reservoir for the green synthesis of gold and silver nanoparticles. IET Nanobiotechnol. 5:69–78.
- Perez C, Paul M, Bazerque P. 1990. An antibiotic assay by the agar well diffusion method. Acta Biol Et Med Exp. 15:113–115.
- Prashanth GK, Prashanth PA, Bora U, Gadewar M, Nagabhushana BM, Ananda S, Krishnaiah GM, Sathyananda HM. 2015. In vitro antibacterial and cytotoxicity studies of ZnO nanopowders prepared by combustion assisted facile green synthesis. Karbala Int J Modern Sci. 1:67–77.
- Raghupathi KR, Koodali RT, Manna AC. 2011. Size-dependent bacterial growth inhibition and mechanism of antibacterial activity of zinc oxide nanoparticles. Langmuir. 27:4020–4028.
- Rajiv P, Rajeshwari S, Venckatesh R. 2013. Bio-Fabrication of zinc oxide nanoparticles using leaf extract of Parthenium hysterophorus L. and its size-dependent antifungal activity against plant fungal pathogens. Spectrochimica Acta A Mol Biomol Spectro. 112:384–387.
- Rakholiya K, Chanda S. 2012. In vitro interaction of certain antimicrobial agents in combination with plant extracts against some pathogenic bacterial strains. Asian Pac J Trop Biomed. 2:S876–S880.
- Rauf A, Ali J, Khan H, Mubarak MS, Patel S. 2016. Emerging CAM Ziziphus nummularia with in vivo sedative hypnotic, antipyretic and analgesic attributes. 3 Biotech. 6:1–10.
- Richards TS, Oliver BG, White TC. 2008. Micafungin activity against Candida albicans with diverse azole resistance phenotypes. J Antimicrobial Chemothera. 62:349–355.
- Samat NA, Nor RM. 2013. Sol–gel synthesis of zinc oxide nanoparticles using Citrus aurantifolia extracts. Ceramics Int. 39:545–548.
- Sangani MH, Moghaddam MN, Forghanifard MM. 2015. Inhibitory effect of zinc oxide nanoparticles on Pseudomonas aeruginosa biofilm formation. Nanomed J. 2:121–128.
- Sangeetha G, Rajeshwari S, Venckatesh R. 2011. Green synthesis of zinc oxide nanoparticles by Aloe barbadensis miller leaf extract: structure and optical properties. Mater Res Bul. 46:2560–2566.
- Sharma RK, Ghose R. 2015. Synthesis of zinc oxide nanoparticles by homogeneous precipitation method and its application in antifungal activity against Candida albicans. Ceramics Int. 41:967–975.
- Sharma SC. 2016. ZnO nano-flowers from Carica papaya milk: Degradation of alizarin red-s dye and antibacterial activity against Pseudomonas aeruginosa and Staphylococcus aureus. Optik. 127:6498–6512.
- Shoeb M, Singh BR, Khan JA, Khan W, Singh BN, Singh HB, Naqvi AH. 2013. ROS-dependent anticandidal activity of zinc oxide nanoparticles synthesized by using egg albumen as a biotemplate. Adv Nat Sci: Nanosci Nanotechnol. 4:1–11.
- Velmurugan P, Park JH, Lee SM, Jang JS, Yi YJ, Han SS. 2016a. Phytofabrication of bioinspired zinc oxide nanocrystals for biomedical application. Artif Cells Nanomed Biotechnol. 44:1529–1536.
- Velmurugan P, Park JH, Lee SM, Yi YJ, Cho M, Jang JS. 2016b. Eco-friendly approach towards green synthesis of zinc oxide nanocrystals and its potential applications. Artif Cells Nanomed Biotechnol. 44:1537–1543.
- Zhang Y, Nayak TR, Hong H, Cai W. 2013. Biomedical applications of zinc oxide nanomaterials. Curr Mol Med. 13:1633–1645.