Abstract
Enamel demineralization is destructive, esthetically compromised, and costly complications for orthodontic patients. Nano-sized amorphous calcium phosphate (NACP) has been explored to address this challenge. The 20% NACP-loaded ortho-cement notably exhibited favorable behavior on reducing demineralization of enamel around brackets in a caries model designed to simulate the carious attack. The 20% NACP-loaded ortho-cement markedly promotes higher calcium and phosphate release at a low pH, and the mineral loss was almost two fold lower and carious lesion depth decreased the by 1/3. This novel approach is promising co-adjuvant route for prevention of dental caries dissemination in millions of patients under orthodontic treatment.
Introduction
As most common and relevant downside, enamel demineralization is a serious clinical problem during and after the orthodontic treatment (Enaia et al. Citation2011). Fixed orthodontic appliances such as brackets, metal ligature ties, arch wires, and elastomeric rings may lead to excessive growth in the number of cariogenic (acid-forming) bacteria-biofilm and consequently developing dental caries (Ahn et al. Citation2010, Papaioannou et al. Citation2007). Enamel demineralization represents the initial developing stage of a carious lesion. The substantial mineral loss is accredited to the prolonged accumulation of oral biofilm (dental plaque) on the enamel surfaces adjacent to the orthodontic appliances. The biofilm retention can be attributed to the difficulties in performing oral hygiene procedures, thereby leaving biofilms behind at the defenseless bracket–cement–enamel junctions (Julien et al. Citation2013). In cases of teeth with bonded brackets, the crucial surfaces which receive inadequate cleaning are areas on the mesial and distal sides of the bracket base.
Enamel demineralization begins initially as a surface-softened area; it then progresses to a subsurface lesion over time, which is clinically seen as a white-spot lesion and can occur in a short time frame of four weeks (Øgaard et al. Citation1988). Acid production by cariogenic bacteria is capable of decreasing the pH of the oral environment from a neutral pH slightly below 7 to an acidic pH of 4.5, which occurs to a greater extent in orthodontic patients than non-orthodontic patients (Lucchese and Gherlone Citation2013). This acid production is what demineralizes (removes calcium and phosphate ions) from enamel (Tanner et al. Citation2012). Following constant acidic environment promoted by bacterial acids, the continuous net mineral loss leads to the irreversible stage of cavitation of the demineralized area. The length of time spent in braces varies considerably, depending on the severity of the case. The average orthodontic treatment time for adults and adolescents is about 24 months, which is long enough to cause lesions (Tanner et al. Citation2012). Such lesions were reported to have an incidence and prevalence rate of 45.8 and 68.4%, respectively, with lesions covering the majority of the facial surface of teeth (Sundararaj et al. Citation2015). The problem of enamel demineralization can be detrimental to oral health with the potential to turn into dental cavities, impairing facial esthetics after orthodontic treatment. Recent studies identified enamel demineralization as an inherent complication of orthodontic treatment and a potential public health threat (Heymann and Grauer Citation2013, Ren et al. Citation2014). Over three million orthodontic patients in the USA alone develop enamel demineralization as a result of the treatment. Up to 7,50,000 of these patients require professional care after orthodontic treatment with an estimated cost of nearly US$500 million, involving 2–3 h per patient needed for invasive restorative treatments under local anesthesia. It is estimated that every year, around 1000 dentists have to work full time to treat demineralized areas after orthodontic treatment (Al Mulla et al. Citation2009). This illustrates the great risk of enamel during orthodontic treatment and needs to find approaches to prevent demineralization without relying on patient cooperation.
Dental enamel comprises the outer layer of the tooth and is the most densely calcified tissue in the body, approaching ∼96% carbonated hydroxyapatite, ∼3% of water and less than 1% of the organic matrix by weight (Duverger et al. Citation2016). It is composed primarily of hydroxyapatite [(Ca10(PO4)6(OH)2] having a rhombohedral shape with typical cross-sectional edge lengths of 25–50 nm. The concentration of calcium and phosphorus is significantly reduced in demineralized enamel, compared to healthy enamel.
An epicenter research area to deal with this problem is the development of engineering dental nanobiomaterials that goes beyond the physical and mechanical aspect to possess bioactivity (Chung et al. Citation2016, Melo et al. Citation2013). In the past decade, significant achievements have been witnessed in the field of dentistry, especially due to a crucial impulse of nanotechnology to the development of bioactive dental materials (Sirin et al. Citation2016). The bioactive materials can stabilize/restore the mineral content and contribute to local tissue regeneration (Besinis et al. Citation2015). In this context, orthodontic cement is in direct contact with the vulnerable enamel surface to perform their role as carrier for nanoparticles. They can release atoms or molecules from its surface into the surrounding tissues where the demineralization process happens.
Founded on high performance for ions release provided by a nanostructured compound, nanoparticles of nano-sized amorphous calcium phosphate (NACP) have led to new possibilities of combating enamel demineralization (Zhao et al. Citation2012). As a precursor of the final crystalline hydroxyapatite, amorphous calcium phosphate (Ca3[PO4]2) has been investigated as a suitable remineralizing agent (Xu et al. Citation2010). NACP could enhance the remineralizing capacity due to the greater surface area-to-volume ratio. NACP had a relatively high specific surface area of 17.76 m2/g, compared to about 0.5 m2/g of traditional CaP particles (Sun et al. Citation2010). The higher proportion of material exposed for the potential reaction requires lower filler level to reach the same outcome of amorphous calcium phosphate on the micro scale. Previous in vitro studies have evidenced the versatility and capacity of NACP embedded in certain types of polymer matrix phases for dental filling materials (Hannig and Hannig Citation2010). Significant levels of calcium (Ca) and phosphate (P) ions were released from these materials that were sustainable over long periods of time and with rechargeable capacity (Moreau et al. Citation2011). The supersaturation by high rates of Ca and PO4 ions released into the surrounding microenvironments under acidic attack plays a vital role in the precipitation of crystallites (Chow et al. Citation2004). It also may be used to overcome the challenges associated with the limited access of ions diffusion from another source of mineralizing agents as toothpaste and mouthwashes to the areas near to the base of brackets (Melo et al. Citation2014). The NACP-loaded ortho-cement would act as a reservoir to supply calcium and phosphate ions. Additionally, we may take advantage of pH-dependent ion release features of NACP to address the neutralization of the acidic biofilm microenvironment around the bracket–cement– enamel interface (Dickens et al. Citation2003). The pH-responsive NACP approach to dealing with the dissolution–diffusion process of dental caries is summarized in . Here, we develop a facile approach that enables enhanced ion release from resin-based orthodontic cement loaded with NACP and provide a great improvement in the mineral content response of human enamel.
Figure 1. Diagrams illustrating the pH-responsive NACP approach to dealing with the dissolution–diffusion process of dental caries: (A) The demineralization of enamel adjacent to orthodontics brackets are characterized by mineral loss with a white appearance. The initial demineralization is the precursor of frank enamel caries. If the enamel demineralization is not controlled, it will progress to irreversible stage of cavitated lesions (the arrows point out the cavited areas after the orthodontic treatment has finalized and the brackets removed); (B) Bacterial attachment to enamel and orthodontic appliances surfaces; acid is produced by the cariogenic biofilm; the subsurface demineralization of tooth requires diffusion of acid ions into, and solubilized mineral ions out of the lesion to form the surface-softened demineralization, that progress to a subsurface lesion over time with the “body of the lesion” consisting of partially demineralized enamel; (C) The bacterial acid reduces the pH, calcium, and phosphate ions are removed from tooth; (D) NACP in an ortho-cement releases calcium (Ca) and phosphate (P) ions. The ions will suppress or retard tooth demineralization as well as facilitate tooth remineralization.
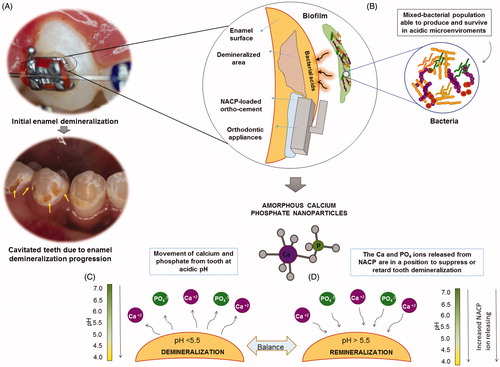
Methods
Synthesis of NACP. Calcium carbonate (CaCO3) and dicalcium phosphate anhydrous (CaHPO4) were provided, respectively, by Fisher (Fair Lawn, Bergen County, NJ) and Baker Chemical Co. (Phillipsburg, Warren County, NJ). Acetic acid was obtained from Sigma-Aldrich (St. Louis, MO). All chemicals were used as received without further purification. A spray-drying process was used to fabricate NACP with high purity as reported by Chow et al. Citation2004. Briefly, calcium carbonate (CaCO3) and dicalcium phosphate anhydrous (CaHPO4) were dissolved in an acetic acid solution to obtain final Ca and P ionic concentrations of 8 and 5.333 mmol/L, respectively. The resulting 1.5 ratio is the same Ca/P molar ratio of amorphous calcium phosphate on micro-scale. The atomizing/spraying of CaP suspension of microsized droplets is followed by a drying process in a heated chamber, resulting in the production of solid particles. The nanoparticles were trapped by an electrostatic precipitator (Air Quality, Minneapolis, MN).
Fabrication of NACP-loaded ortho-cement
For the preparation of NACP-loaded ortho-cement, four proportions of NACP were used: 10, 15, 20, and 30% by weight. A commercially available ortho-cement (Transbond XT, 3M Unitek, Monrovia, CA) was used as the parental material. This cement consisted of silane-treated quartz (70–80% by weight), bisphenol-A-diglycidyl ether dimethacrylate (10–20%), bisphenol-A-bis (2-hydroxyethyl) dimethacrylate (5–10%), silane-treated silica (<2%), and diphenyl iodonium hexafluorophosphate (<0.2%), according the manufacturer. The NACP powder was added and the mixture was homogenized to reach the four tested NACP-loaded ortho-cements.
Characterization techniques
The surface morphology of the NACP powder was investigated using transmission electron microscopy. The Brunauer, Emmett, and Teller (BET) surface area measurement yielded an equivalent particle diameter of 112 nm as shown in the previous report by Xu et al. Citation2010. Shear bond tests, mineral content analysis by cross-sectional microhardness (CSMH) testing, and ion releasing assays were performed as described below. A minimum of 12 samples of each material was used to produce reproducible and relevant data.
Test on bonding mechanical properties
To determine the optimal ratio of NACP to ortho-cement (denoted as OC + NACP), we prepared pastes with various ratios ranging from 10 to 30% wt to assess the bond strength to the enamel of metallic brackets when bonded with ortho-cement containing NACP. The institutional review board of the University of Maryland approved the collection and use of extracted human teeth. Sixty human permanent premolars were randomly allocated into five groups (n = 12). The inclusion criteria were premolars fully erupted and intact without white spot lesions or visible defects on their buccal surfaces. For bonding procedures, the buccal surfaces of each tooth were subjected to prophylaxis, washed, etched with phosphoric acid at 35% (Scotchbond, 3M ESPE, St. Paul, MN) for 20 s, then washed and dried. The NACP-loaded ortho-cement was used to bond conventional stainless brackets (Edgewise, Ormco, Glendora, CA) to the central area of the buccal surface. No additional surface pretreatment was performed. Both sides (mesial and distal) of the bracket were light-cured using a quartz-tungsten-halogen unit with peak emission wavelength of 460 nm and output intensity of 600 mW/cm2 (Optilux 501, Kerr, Danbury, CT) for 10 s. The tooth-bracket set was incubated in distilled water at 37 °C for 24 h.
A chisel with occluso-gingival load on a Universal Testing Machine (MTS, Eden Prairie, MN) was aligned to be parallel to the bracket-tooth interface and loaded at a displacement rate of 0.5 mm/min until the bond failed. The maximum load was recorded in Newtons (N) and then converted into Mpa using the following equation: shear stress (Mpa) = Debonding force (N)/Bracket surface area (mm2), where 1 Mpa = 1 N/mm2 and the bracket surface area is 11.35 mm2.
Ca and P ion release measurement
Ionic release for Ca and P was quantified by colorimetric, spectrophotometric method (DMS-80 UV–vis, Varian, Palo Alto, CA) using known standards and calibration curves. The influence of pH on Ca and P release was studied at pH values that simulate the transition from a neutral healthy condition to a cariogenic low pH condition. A sodium chloride solution (133 mmol/L) was buffered to three pH values: pH 4, 5.5, and 7 with 50 mmol/L HEPES using a combination pH electrode (Orion, Cambridge, MA).
For control and NACP-loaded ortho-cements, three specimens (2 × 2 ×12 mm) were immersed in 50 mL-containing solution plastic tubes to yield a specimen volume/solution of 2.9 mm/mL. The Ca and P ion concentrations released from the specimens were measured at 1, 3, 5, 7, 14, 21, and 28 days. For analysis, 500 μL aliquots were removed and replaced with fresh solution. The release was measured as the total accumulative ion concentration versus time.
Production of artificial enamel caries lesions model
Artificial caries lesions on enamel around orthodontic appliances were produced by pH-cycling, a dynamic model that simulates a clinical situation of enamel being subjected to a cariogenic environment. Thirty human permanent premolars were randomly allocated into two groups (n = 15). Based on the data from bonding strength test and Ca and P ion release, the NACP-loaded ortho-cement at 20% was selected to bond conventional stainless brackets to the central area of the buccal surface. An ortho-cement without NACP was used as a reference. The teeth to be demineralized were coated with acid-resistant nail varnish except for buccal area and subjected to 30 days-demineralization–remineralization cycles at 37 °C by immersion in pH-cycling solutions according to previously described protocol (Melo, Morais et al. Citation2014). Each cycle consisted of a 1-h immersion in 20 mL of demineralizing solution, followed by a 23-h immersion in 20 mL of remineralizing solution. The demineralizing solution contained 3.0 mmol/L CaCl2, 1.8 mmol/L K2HPO4, 0.1 mol/L-lactic acid, and 1% carboxymethylcellulose adjusted to a pH of 4. The remineralizing solution contained 1.2 mmol/L CaCl2, 0.72 mmol/L K2HPO4, 2.6 μmol/L F, and 50 mmol/L HEPES buffer (pH pH7).
Cross-sectional microhardness (CSMH) testing
The mineral contents around the studied ortho-cements were assessed via lesion depths and integrated demineralization. The tooth was longitudinally sectioned in the center of the bracket (IsoMet, Buehler, Lake Bluff, IL). Tooth section was embedded in self-curing acrylic resin (Lang Dental Manufacturing Co., Wheeling, IL). The surface was ground flat with wet 320-, 600-, and 1200-grit silicon carbide paper (Extec, Enfield, CT) and polished with a 1-μm diamond paste (Extec, Enfield, CT).
The CSMH analysis was performed using a Knoop diamond under a 25-g load for 5 s on a microhardness tester (FM7AMRS; Future Tech, Tokyo, Japan). Testing was performed with 30 indentations distributed in two rows of 15 indentations each. The first 10 indentations were spaced 10 μm from the previous one and the last ones at 20-μm intervals. The first row began at 20 μm from the enamel border that remained in contact with the ortho-cement, with the other row performed 100 μm from the ortho-cement border. The 80-μm distance between the lanes was established to minimize interactions between indentations marks. CSMH values, up to the 200-μm depth, were converted to integrated demineralization (ΔS). The integrated area of sound enamel (Δ1) and pH-cycled (Δ2) enamel was calculated, followed by calculating the mineral content [ΔS = (Δ2 – Δ1)]. Also, we obtained the hardness profile of each group by plotting hardness as a function of distance from enamel surface. Lesion depth was measured as the distance from the surface where the mineral content was 95% of the sound enamel level.
Statistical analysis was conducted using Sigma Stat statistical package version 3.5 (Systat, San Jose, CA). Data were tested for normality using Q Kolmogorov–Smirnov test. One-way analysis of variance (ANOVA) and Tukey’s HSD post hoc tests was applied for bond strength values at P = .05. Data from ion release, ΔS, lesion depth, and CSH were subjected to the unpaired t-test.
Results and discussion
Amorphous calcium phosphate has excellent biocompatibility and chemical similarity to human dental tissues and it was prepared here according to previously reported method (Langhorst et al. Citation2009). Other works report and explore NACP potential for dealing with enamel demineralization for restorative proposes (Zarschler et al. Citation2016). The effective inhibition of demineralization found in this study, associated to the acid neutralization previously reported (Moreau et al. Citation2011) indicates that the NACP-loaded ortho-cement is promising for caries inhibition around orthodontic brackets.
We monitored the ion release and showed its effect on mineral loss in a simulated model for orthodontic clinical application for the first time. During the very early stages of caries, the nanoscale-sized carbonated hydroxyapatite crystallites are only slightly altered in size and orientation (Olszta et al. Citation2003). The deposition of plaque calcium and phosphate ions from an amorphous disordered mineral precursor to preserve the crystalline phase pattern of the mineral is the hallmark of demineralization–remineralization homeostasis (Kwak et al. Citation2014). In this study, the final nanopowder as revealed by TEM was about 116 nm in average size as shown in . The characterization of NACP crystalline phases by X-ray diffraction, infrared spectra by FT-IR spectrometer, particle size distribution by dynamic light scattering, the surface area analysis by BET and microstructure, and the solubility properties were assessed in the previous report by Xu et al. Citation2011). It was also observed during the cement manipulation, better interface, and dispersion of amorphous calcium phosphate within the resin matrix related to the deagglomeration, common condition in the micro size of this compound.
Figure 2. (A) Typical TEM micrographs of nanoparticles of amorphous calcium phosphate (NACP). Arrows indicate examples of small particles (about 10 nm) and larger particles (about 100–300 nm). (B) The shear bond response of ortho-cement with a varied weight fraction of NACP. The lowest shear resistance is found at 30%. The band shows the range of clinically acceptable shear bond strength data expressed in the literature. *P ≤ .05 about all other groups; one-way ANOVA.
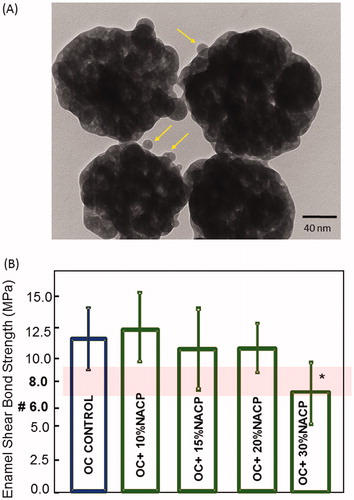
Screening of the optimal ratio via shear bond strength
To determine the optimal ratio of NACP to ortho-cement (denoted as OC + NACP), we prepared pastes with various ratios ranging from 10 to 30% wt to assess the bond strength to the enamel of metallic brackets when bonded with ortho-cement containing NACP. The optimization of the NACP-ortho-cement ratio was based on maximum incorporation while achieving effective shear bond strength able to withstand the forces applied during the orthodontic treatment. This mechanical response is critical for clinical applicability of the tested materials. The present simulation using human teeth provide a prediction that is much more consistent with the real intra-oral scenario (Finnema et al. Citation2010).
shows the enamel shear bond strength results for the ortho-cements with increased weight fraction to determine the rate-limiting fraction (mean ± sd; n = 12). A range of 6–8 Mpa (red band in ) has been reported previously as the adequate bond strength for orthodontic purposes. The shear bond response of ortho-cement varied with increasing weight fraction of NACP. The lowest shear resistance is found at a weight fraction of 30%. The changes in chemistry had a significant impact on the stiffening of the ortho-cement which can be explained by the high inorganic filler content (silane-treated quartz at 70–80%) in this type of material. The shear bond strengths ranged from 11.1 to 12.3 Mpa for weight fraction of up to 20% with no statistical differences (P = .093). The optimal ratio of NACP-ortho-cement was at 20% wt.
Ions release and mineral assessment
In the presence of sugars, the caries-related bacteria produce acids by bacterial metabolism; the biofilm fluid becomes under-saturated on the enamel mineral, and demineralization occurs (Cury and Tenuta Citation2009). In the control of caries process, it is crucial to maintaining the balance between demineralization (mineral loss) and remineralization (mineral gain). This involves shifting it in favor of remineralization where the pH of the saliva returns to normal (∼pH of 7) so that a period of remineralization (repair) occurs in non-cavitated caries lesions (ten Cate and Duijsters Citation1982). This process is facilitated if fluoride, calcium, and phosphate ions are locally present in supersaturated concentrations (ten Cate et al. Citation2008). This can drive the penetration of enamel surface to the subsurface lesion by ions of calcium, phosphate, and fluoride (Lynch Citation2006). The precipitation of calcium phosphate mineral from ionic clusters exists in amorphous calcium phosphate from both synthesized and biological origin. These clusters correspond to a local arrangement of calcium and phosphate ions existing in the structure of apatites and are analogous to that existing in several other crystalline phosphates, such as apatites, OCP, and b-tricalcium phosphate (TCP).
A recent trend in nanotechnology is investigating the interactions of nanoparticles with surrounding biological systems, known as nanobio interactions (Prabha et al. Citation2016). In the dental materials field, besides size effect, surface chemistry plays an important role on nanoparticle-surround environment interactions which has promoted substantial progress in the feasibility of this compound for remineralization.
The repair of early caries lesions may be enhanced by an exogenous supply of calcium and phosphate from the ortho-cement. NACP can present high levels of Ca and P ion release (Lynch Citation2006, Weir et al. Citation2012). illustrates the calcium and phosphate ion release from 20% NACP-loaded ortho-cement and control as a function of time and pH. The dependence of ion release on the acidity can be observed in calcium and phosphate concentration (). The lower the pH, the larger the amount of calcium and phosphate released by the 20% NACP-loaded ortho-cement. At pH 5.5, the calcium ion concentration is 55% greater than at pH 7; at pH 4, the values reached 78% higher than in neutral medium. The solution pH significantly affected the Ca and P ion release (P < .05). This suggests that in a more acidic medium, a condition where the enamel presents a deficit of calcium and phosphate (sub-saturated concentration), the strongly pH-dependent release of calcium and phosphate may drive the diffusion of ions into depleted mineral areas. These ions can form hydroxyapatite or fluorapatite in the presence of fluoride. As the mineral is formed, it is deposited on the margins of the lesion, resulting in a progressive reduction in size toward the center (ten Cate et al. Citation2008).
Figure 3. The extent of calcium and phosphate release from ortho-cement as a function of days in immersion time and pH. Time-cumulative release measurements of (A) calcium ions, and (B) phosphate ions. Each value is mean ± SD; n ;±3. Compared to pH 7, the ion release was slightly higher at pH 5.5, but substantially higher at pH 4. 20% NACP-loaded ortho-cement had a greater release in the solution of pH 4, which simulates a local cariogenic pH from biofilm acids.
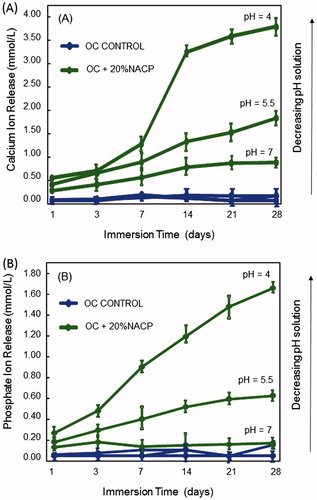
Next, we investigated the effect of ions from the ortho-cement on the inhibition of demineralization or enhancement of remineralization around ortho-cements. Mineral content can be clearly shown in vitro using pH-cycling models which simulate clinical situations of predominant demineralization (De > Re) or remineralization (Re > De). This model captures the observed sequence of oscillating pH events similar to those experienced in the mouth and is particularly suitable for studying a single process in isolation as the applicability for anticaries systems (Cury and Tenuta Citation2009). However, the demineralization process in vitro was somewhat more aggressive than what is typical in vivo. The reason for the use of an aggressive acid attack of long duration (30 d) was to simulate the accumulation of damaging effects of acid attacks during the timeframe of orthodontic treatment in vivo (∼2 years) (Weir et al. Citation2012).
It appears from the results shown in that the availability of calcium and phosphate ions has a relevant role in the non-invasive management of non-cavitated caries lesions. This implies in minerals deposition similar to those of enamel and at the same time that can diffuse through the inner (body) lesion. The microhardness values for enamel around the 20% NACP-loaded ortho-cement were higher than control at both distances from the ortho-cement interface, with a significant difference extended to the depth to ∼40 μm as highlighted in and 4B*. At 30-μm depth and for a 20-μm distance, the Knoop hardness value for 20% NACP-loaded ortho-cement was 175.3 versus 81.7 which shows an increase of 116% in relation to unmodified ortho-cement.
Figure 4. Mean ± standard deviation of Knoop hardness in enamel at 20 μm (A), and 100 μm (B), from the interface of enamel/ortho-cement. The effect of calcium and phosphate ion release on enamel hardness when De > Re (patient under caries activity) is reflected in increasing enamel hardness. *P ≤ .05. The dosh dot selected area (*) represents the area where the mineral content around the ortho-cements was markedly different.
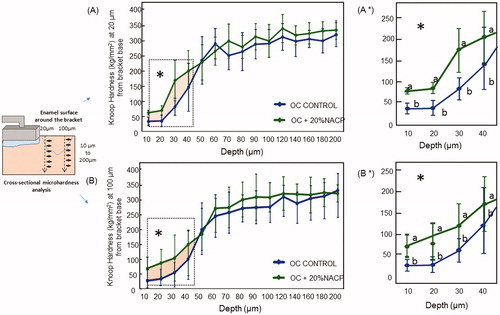
According to mineral content from , enamel demineralization was significantly inhibited to a certain degree in our study. The 20% NACP-loaded ortho-cement exhibited significantly higher mineral content compared to the control (). The average lesion depth was also significant lower around the 20% NACP-loaded ortho-cement (P ≤ .05), representing 69% of the control lesion depth. Nanotechnology can provide newer, promising a route to the development of a new generation of dental materials such as orthodontic cement and composites with a combination of load-bearing and remineralization capabilities. Amorphous calcium phosphate is particularly useful in its nanoparticle format, which has appeared to be a promising material with a great deal of interest over recent years.
Figure 5. Enamel mineral loss around 20% NACP-loaded ortho-cement and control ortho-cement versus the (A) 20 μm and (B) 100 μm-distance from the enamel–ortho-cement interface. At both distances, enamel mineral loss was much less around NACP-loaded ortho-cement than control; (C) The average lesion depth at 0–100 μm from the enamel–ortho-cement interface was calculated from the hardness profiles.
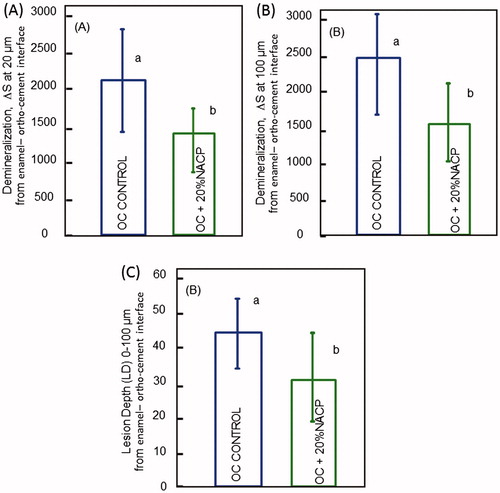
Conclusion and outlook
The use of calcium phosphate nanoparticles as a remineralizing tool is possible if they are stabilized in a polymeric resin matrix. We designed a bioactive ortho-cement loaded with NACP. NACP was incorporated into an orthodontic bracket cement, and the enamel bonding properties, as well as dental mineral content responses, were determined for the first time. We showed that at 20% NACP content, the ortho-cement performed similarly to commercially available ortho-cement regarding bonding strength. The in vitro tests support high releasing rates of Ca and PO4 and positive response on the preservation of mineral content under acidic condition. Capitalizing on the high surface area of the nanomaterials, this approach could enable the production of tailor-made ortho-cements, which could provide a promising tool to meet the challenge of contemporary orthodontic practice. New nanotechnology using calcium phosphate bioactive materials is promising to reduce the incidence of caries lesions in orthodontic patients without relying on patient compliance.
Acknowledgments
This study was supported by NIH R01 DE17974 (HX), a bridge fund from the Department of Endodontics, Prosthodontics and Operative Dentistry (HX), and a seed grant from the University of Maryland Baltimore (HX). The authors especially thank the Laboratory of School of Pharmacy, Dentistry, and Nursing of the Federal University of Ceará, Brazil for the use of their microhardness tester.
Disclosure statement
No conflict of interest.
Additional information
Funding
References
- Ahn SJ, Lim BS, Leec SJ. 2010. Surface characteristics of orthodontic adhesives and effects on streptococcal adhesion. Am J Orthod Dentofacial Orthop. 137:489–495.
- Al Mulla AH, Kharsa SA, Kjellberg H, Birkhed D. 2009. Caries risk profiles in orthodontic patients at follow-up using Cariogram. Angle Orthod. 79:323–330.
- Besinis A, De Peralta T, Tredwin CJ, Handy RD. 2015. Review of nanomaterials in dentistry: interactions with the oral microenvironment, clinical applications, hazards, and benefits. ACS Nano. 9:2255–2289.
- Chow LC, Sun L, Hockey B. 2004. Properties of nanostructured hydroxyapatite prepared by spray drying technique. J Res Natl Inst Stand Technol. 109:543–551.
- Chung J, Kim YKK-H, Kim Kwon T, Vaezmomen SZ, Samiei M, Aghazadeh M, et al. 2016. Synthesis, characterization, biocompatibility of hydroxyapatite–natural polymers nanocomposites for dentistry applications. Artif Cells Nanomed Biotechnol. 44:277–284.
- Cury JA, Tenuta L. 2009. Enamel remineralization: controlling the caries disease or treating early caries lesions?. Braz Oral Res. 23:23–30.
- Dickens SH, Flaim GM, Takagi S. 2003. Mechanical properties and biochemical activity of remineralizing resin-based Ca-PO4 cements. Dent Mater. 19:558–566.
- Duverger O, Beniash E, Morasso MI. 2016. Keratins as components of the enamel organic matrix. Matrix Biol. 52–54:260–265.
- Enaia M, Bock N, Ruf S. 2011. White-spot lesions during multibracket appliance treatment: a challenge for clinical excellence. Am J Orthod Dentofacial Orthop. 140:17–24.
- Finnema KJ, Ozcan M, Post WJ, Ren Y, Dijkstra PU. 2010. In-vitro orthodontic bond strength testing: a systematic review and meta-analysis. Am J Orthod Dentofacial Orthop. 137:615–622.
- Hannig M, Hannig C. 2010. Nanomaterials in preventive dentistry. Nat Nanotechnol. 5:565–569.
- Heymann GC, Grauer D. 2013. A contemporary review of white spot lesions in orthodontics. J Esthet Restor Dent. 25:85–95.
- Julien KC, Buschang PH, Campbell PM. 2013. Prevalence of white spot lesion formation during orthodontic treatment. Angle Orthodontist. 83:641–647.
- Kwak SY, Kim S, Yamakoshi Y, Simmer JP, Beniash E, Margolis HC. 2014. Regulation of calcium phosphate formation by native amelogenins in vitro. Connect Tissue Res. 55:21–24.
- Langhorst SE, O'Donnell JN, Skrtic D. 2009. In vitro remineralization of enamel by polymeric amorphous calcium phosphate composite: quantitative microradiographic study. Dent Mater. 25:884–891.
- Lucchese A, Gherlone E. 2013. Prevalence of white-spot lesions before and during orthodontic treatment with fixed appliances. Eur J Orthod. 35:664–668.
- Lynch RJ. 2006. Model parameters and their influence on the outcome of in vitro demineralisation and remineralisation studies. Monogr Oral Sci. 19:65–85.
- Melo MA, Guedes SF, Xu HH, Rodrigues LK. 2013. Nanotechnology-based restorative materials for dental caries management. Trends Biotechnol. 31:459–467.
- Melo MA, Morais WA, Passos VF, Lima JP, Rodrigues LK. 2014. Fluoride releasing and enamel demineralization around orthodontic brackets by fluoride-releasing composite containing nanoparticles. Clin Oral Investig. 18:1343–1350.
- Melo MA, Wu J, Weir MD, Xu HH. 2014. Novel antibacterial orthodontic cement containing quaternary ammonium monomer dimethylaminododecyl methacrylate. J Dent. 42:1193–1201.
- Moreau JL, Sun L, Chow LC, Xu HH. 2011. Mechanical and acid neutralizing properties and inhibition of bacterial growth of amorphous calcium phosphate dental nanocomposite. J Biomed Mater Res B Appl Biomater. 98:80–88.
- Øgaard B, Rolla G, Arends J. 1988. Orthodontic appliances and enamel demineralization. Part 1. Lesion development. Am J Orthod Dentofacial Orthop. 94:68–73.
- Olszta MJ, Odom DJ, Douglas EP, Gower LB. 2003. A new paradigm for biomineral formation: mineralization via an amorphous liquid-phase precursor. Connect Tissue Res. 44:326–334.
- Papaioannou W, Gizani S, Nassika M, Kontou E, Nakou M. 2007. Adhesion of Streptococcus mutans to different types of brackets. Angle Orthodontics. 77:1090–1095.
- Prabha S, Arya G, Chandra R, Ahmed B, Nimesh S. 2016. Effect of size on biological properties of nanoparticles employed in gene delivery. Artif Cells Nanomed Biotechnol. 44:83–91.
- Ren Y, Jongsma MA, Mei L, Van der Mei HC, Busscher HJ. 2014. Orthodontic treatment with fixed appliances and biofilm formation-a potential public health threat? formation–a potential public health threat?. Clin Oral Investig. 18:1711–1718.
- Sirin HT, Vargel I, Kutsal T, Korkusuz P, Piskin E. 2016. Ti implants with nanostructured and HA-coated surfaces for improved osseointegration. Artif Cells Nanomed Biotechnol. 44:1023–1030.
- Sun L, Chow LC, Frukhtbeyn SA, Bonevich JE. 2010. Preparation and properties of nanoparticles of calcium phosphates with various Ca/P ratios. J Res Natl Inst Stand Technol. 115:243–255.
- Sundararaj D, Venkatachalapathy S, Tandon A, Pereira A. 2015. Critical evaluation of incidence and prevalence of white spot lesions during fixed orthodontic appliance treatment: a meta-analysis. J Int Soc Prev Community Dent. 5:433–439.
- Tanner AC, Sonis AL, Lif Holgerson P, Starr JR, Nunez Y, Kressirer CA, Paster BJ, Johansson I. 2012. White-spot lesions and gingivitis microbiotas in orthodontic patients. J Dent Res. 91:853–858.
- ten Cate JM, Duijsters PP. 1982. Alternating demineralization and remineralization of artificial enamel lesions. Caries Res. 16:201–210.
- ten Cate JM, Larsen MJ, Pearce EIF, Fejerskov O. 2008. Chemical interactions between the tooth and oral fluids. In: Fejerskov O, Kidd E, Eds. Dental Caries – The Disease and Its Clinical Management, 2nd ed. Oxford: Blackwell Munksgaard, Chap. 12.
- Weir MD, Chow LC, Xu HH. 2012. Remineralization of demineralized enamel via calcium phosphate nanocomposite. J Dent Res. 91:979–984.
- Xu HH, Moreau JL, Sun L, Chow LC. 2011. Nanocomposite containing amorphous calcium phosphate nanoparticles for caries inhibition. Dent Mater. 27:762–769.
- Xu HH, Weir MD, Sun L, Moreau JL, Takagi S, Chow LC, Antonucci JM. 2010. Strong nanocomposites with Ca, PO(4), and F release for caries inhibition. J Dent Res. 89:19–28.
- Zarschler K, Rocks L, Licciardello N, Boselli L, Polo E, Garcia KP, et al. 2016. Ultrasmall inorganic nanoparticles: state-of-the-art and perspectives for biomedical applications. Nanomedicine. doi: 10.1016/j.nano.2016.02.019. [Epub ahead of print]
- Zhao J, Liu Y, Sun W, Yang X. 2012. First detection, characterization, and application of amorphous calcium phosphate in dentistry. J Dent Sci. 7:316–323.