Abstract
Gold (FA-AuNps) and silver (FA-AgNps) nanoparticles were synthesized at room temperature by aqueous extract of dried fruits of Amomum villosum, also known as Fructus Amomi (cardamom), in order to confer antioxidant, catalytic, antimicrobial activities and treatment effect against breast cancer cells. Fruit extracts served as both reducing agents and stabilizers in lieu of chemical agents. Ultra-violet visible (UV-Vis) spectroscopy, field emission transmission electron microscopy (FE-TEM), energy-dispersive X-ray (EDX) spectroscopy, elemental mapping, X-ray powder diffraction (XRD), selected area electron diffraction (SAED), dynamic light scattering (DLS) and Fourier transform infrared (FTIR) spectroscopy were employed to characterize the biosynthesized nanoparticles. Both FA-AuNps and FA-AgNps exhibited free radical scavenging activity against 2,2-diphenyl-1-picrylhydrzyl (DPPH). Additionally, biosynthesized nanoparticles successfully reduced methylene blue, a well-known redox indicator. FA-AgNps showed zones of inhibition against pathogenic Staphylococcus aureus and Escherichia coli. Finally, the biological activities and cytotoxicity of nanoparticles were subsequently investigated in vitro. FA-AuNps demonstrated a potential cytotoxic agent against breast cancer cells as evaluated by MTT assay. The study highlights a rapid synthesis of FA-AuNps and FA-AgNps by dried Fructus Amomi aqueous extract and evaluates their potential biological applications on medical platforms.
Graphical Abstract
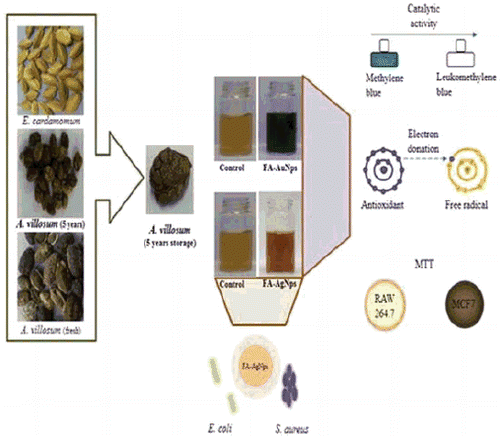
Introduction
Nanotechnology has become one of the most auspicious technologies incorporated in a wide range of scientific disciplines to impart cutting edge features. The combination of biotechnology and material science has gained pervasive attention due to huge number of applications in the biomedical and physiochemical fields. Lately, the biogenic synthesis of metal nanoparticles has been thoroughly studied and has been widely used because of its eco-friendly mechanism [Citation1–3]. Two of the most utilized metal nanoparticles are gold nanoparticles (AuNps) and silver nanoparticles (AgNps) as they present captivating aspects over multiple fields including electronics, materials science, medical and their applications to catalysis and biology [Citation4]. Moreover, silver nanoparticles (AgNps) are distinguished for their antibacterial, antifungal and anti-inflammatory activities [Citation5,Citation6].
Conventional synthetic methods such as chemical and physical have several disadvantages such as utilization of toxic reactive (hydrazine and sodium borohydride), excessive power consumption, high cost and sophisticated apparatus. Green synthesis, on the contrary, offers a more eco-friendly and safer approach due to the implementation of green resources. At present, there have been various studies which exploited plants and bacteria to synthesize metal nanoparticles. For instances, Panax ginseng [Citation7], Eleutherococcuss enticosus [Citation8] and Bacillus methylotrophicus [Citation9] have been reported to synthesize AuNps and AgNps. In this study, we portray a very facile method of green synthesis of metal nanoparticles using aqueous extract dried fruits of cardamom, also known as Fructus Amomi.
Wild cardamom such as Amomum villosum and true cardamom such as Elettaria cardamomum is an important non-timber forest product that has a very high value. However, as a result of lack of scientific research, principally in taxonomy, the scientific knowledge is poor [Citation10]. Amomum villosum is a perennial herb; 60–70% of total yield is produced in Xishuangbanna in Yunnan Province, China. The fruits of these plants have been used as spices in Asian cuisines and as herbal medicines to treat stomachache, anorexia and dysentery in humans. In Traditional Chinese Medicine (TCM), these fruits are commonly known as Shan Ren [Citation11]. Similarly, E. cardamomum has been cultivated and naturalized in South Eastern countries, Sri Lanka, India and Central America [Citation12,Citation13]. In virtue of these pharmacological activities, we aim to synthesize Cardamom fruits functionalized gold (FA-AuNps) and silver nanoparticles (FA-AgNps) with positive biological applications.
The biosynthesized nanoparticles were characterized by ultra-violet visible (UV-Vis) spectroscopy, field emission transmission electron microscopy (FE-TEM), energy-dispersive X-ray (EDX) spectroscopy, elemental mapping, X-ray powder diffraction (XRD), selected area electron diffraction (SAED), dynamic light scattering (DLS) and Fourier transform-infrared (FT-IR) spectroscopy. The biosynthesized nanoparticles were investigated for antioxidant and catalytic activity against 2,2-diphenyl-1-picrylhydrzyl (DPPH) free radical and methylene blue (MB), respectively. Additionally, FA-AgNps were tested for their antimicrobial properties against bacterial pathogens Staphylococcus aureus and Escherichia coli. Lastly, the in vitro cytotoxicity in murine macrophage (RAW264.7) and human breast cancer (MCF7) cell lines were evaluated.
Materials and methods
Materials
The fruits of A. villosum and E. cardamomum were obtained from the Ginseng bank, Kyung Hee University, South Korea. Gold (III) chloride trihydrate (HAuCl4·3H2O) and silver nitrate (AgNO3) was purchased from Sigma-Aldrich Chemicals, USA. Methylene blue (C16H18CIN3S·nH2O) was purchased from Kanto Chemical Co., Inc., Tokyo, Japan. DPPH and L-ascorbic acid were purchased from Sigma-Aldrich Chemicals, USA. All media used were purchased from Difco, MB cell, Seoul, Korea. The pathogenic bacterial strains S. aureus (ATCC 6538) and E. coli (BL21) were used. Methyl alcohol (MeOH) was procured from Samchun Pure Chemical Co. Ltd., Gyeonggi-do, Korea and further diluted by distilled water to reach 80% concentration for experimental work.
Synthesis of nanoparticles
Three distinct Cardamom fruits were used to synthesize nanoparticles: 5-year old A. villosum, fresh A. villosum and fresh E. cardamomum. 10 g of each fruit were grinded thoroughly and autoclaved for 1 h at 100 °C in 100 ml of sterile water to obtain aqueous fruit extracts. After boiling, the collected extracts were filtered with Whatman filter papers. Filtered extracts were further diluted to reach a concentration of 70% and stored at 4 °C for further use. To synthesize nanoparticles, 1 mM of HAuCl4·3H2O and AgNO3 solution were added into diluted extracts at room temperature. A colour change in the reaction mixtures indicated the formation of nanoparticles. After the synthesis, the nanoparticles were collected by centrifugation at 16,000 rpm for 10 min, washed thoroughly with sterile water, and finally washed with 80% MeOH. The washed nanoparticles of the chosen extract were kept overnight for air-drying and obtained in pellet form for XRD and FTIR characterization.
Characterization of nanoparticles
UV-vis spectra analysis
To confirm the reduction of gold and silver salts after visual observations, nanoparticle suspension was scanned by UV-Vis spectrophotometer (2100 Pro; Amersham Biosciences Corp., Piscataway, NJ) in the range of 300–800 nm. Spectra were obtained from 200 μL of test volumes with a 1 cm path length quartz cuvette.
FE-TEM analysis
A multifunctional 200 kV-operated JEM-2100 F (JEOL) electron microscope was used to analyze the shape, size, purity, crystallinity and distribution of metallic elements. Droplets from the nanoparticle suspensions in water were placed onto a carbon-coated copper grid. Prepared samples were dried in an oven at 60 °C for 15 min and transferred to electron microscope for analysis. FE-TEM was used to define the morphology of the nanoparticles. EDX spectrometer measured the purity of metallic nanoparticles. Elemental mapping showed the relative location and the distribution of the target element (i.e. Au and Ag). Lastly, SAED was used to identify the crystallite structure of the nanosized particles.
XRD analysis
XRD analysis was executed on an energy dispersive X-ray spectrometer (D8 Advance, Bruker, Germany). XRD depends on the double wave/particle essence of X-rays to receive data about the structure of crystalline elements. The working conditions for the instrument were 40 mA and 40 kV. Cross-section of nanoparticles in powder form was taken and based on a quartz plate to impact Cu Kα radiation of wavelength λ = 1.5406 Å. The samples were run over the 2θ range of 20–80° at 6°/min with sampling intervals of 0.02° in room temperature. Based on received results, the average crystal size diameter of gold and silver/silver chloride nanoparticles could be calculated using Scherrer equation: D = Kλ/β cos θ, where D is the crystallite size in nm, K is dimensionless shape factor (0.9), λ is the X-ray wavelength of Cu-Kα radiation in nm, β is the full width at half maximum (FWHM) in radians and θ is the half of the Bragg angle in radians.
Nanoparticle size analysis by DLS technique
DLS is a technique that is used to define the size distribution structure of nanoparticles in suspension. DLS data were performed at 23 °C with zeta potential and particle size analyzer ELSZ-2000 series (Otsuka Electronics Photal, Osaka, Japan).
FT-IR spectroscopy analysis
The surface capping of metal nanoparticles was analyzed by Perkin Elmer Spectrum One FT-IR spectrometer with the range of 4000–450 cm−1. The spectra recorded were plotted as transmittance (%) versus wavenumber (cm−1).
In vitro biological studies of nanoparticles
DPPH radical scavenging assay
In vitro antioxidant activity of both FA-AuNps and FA-AgNps was calculated using a modified method based on a previous DPPH assay [Citation14]. Methanolic nanoparticle suspension of 20 μL was mixed with 180 μL of 1 mM methanolic DPPH solution. To obtain IC50, different concentrations of FA-AuNps and FA-AgNps (100, 250, 500, 1000 and 1500 μg/mL) were adopted. The radical scavenging activity was measured as percentage inhibition: [(Acontrol − Asample)/Acontrol] × 100, where Asample is the absorbance of the DPPH solution with nanoparticles and Acontrol is the absorbance of the DPPH solution without nanoparticles. L-ascorbic acid (vitamin C) was used as positive control. The reaction mixture was incubated at room temperature in dark condition for 30 min and the colour change was measured by an ELISA reader at 517 nm. The experiments were executed in triplicates with mean ± standard error calculated [Citation14].
Catalytic activity of nanoparticles on reduction of methylene blue
Methylene blue (MB), also known as methylthioninium chloride, was implemented as a redox indicator in order to measure the catalytic activity of the metal nanoparticles. The following three reaction mixtures were monitored using 1 cm path length quartz cuvette and absorbance values were assessed by UV–Vis spectrophotometer. In the first reaction, 1 mL of MB (1 ×1 0−4 M), 0.2 mL of aqueous Fructus Amomi extract, and 1.8 mL of distilled water was mixed. After 30 min of incubation in the dark, the absorbance was measured. In second and third reactions, the same amount of MB (1 mL, 1 × 10−4 M) was mixed with 0.2 mL of fruit extract and 1.8 mL of synthesized FA-AuNps and FA-AgNps (10 μL of 1000 μg/mL stock) accordingly. These reaction mixtures were monitored after 30 min incubation. The obtained data of absorption maxima were compared with that of MB’s absorbance (1 mL of 1 × 10−4 M of MB and 2 mL of distilled water) [Citation15]. The experiments were executed in triplicates with mean ± standard error calculated.
Antimicrobial activity of FA-AgNps
The antimicrobial activity of metal nanoparticles studied were examined on Mueller-Hinton agar (MHA) plates against pathogenic bacteria, S. aureus and E. coli, using the disc diffusion method. 100 μL of overnight cultured bacteria was added into MHA plates and spread equally. Furthermore, the sterile autoclaved paper discs were placed on MHA plates and permeated by 30 μL (100 mg/L) freshly prepared nanoparticles suspensions. As a control, the standard antibiotic disc of Neomycin was maintained on each plate. After incubation at 37 °C for 24 h, the average diameter of each concentration’s zone of inhibition was measured and compared. The experiments were executed in triplicates with mean ± standard error calculated
In vitro cell cytotoxicity assay
The cytotoxicity of FA-AuNps and FA-AgNps in RAW264.7 cells and MCF7 cells were evaluated using 3-(4,5-dimethyl-2-thiazolyl)-2,5-diphenyl-2 H tetrazolium bromide (MTT) (Life Technologies, Eugene, OR) assay. All cell lines were cultured in Dulbecco’s Modified Eagles Medium (DMEM) (Gibco-BRL, Grand Island, NY) supplemented with 10% foetal bovine serum (FBS) and 1% penicillin/streptomycin (P/S) (WelGENE Inc., Daegu, Korea) at 37 °C in a humidified atmosphere containing 5% CO2 and 95% air and were seeded at a density of 1 × 104 per well in a 96-well plate (Corning Costar, Lowell, NY). The wells were treated with different concentrations of FA-AuNps and FA-AgNps (0, 1, 25, 50 and 100 μg/mL) at 37 °C for 48 h at 90% confluency. After incubation for 48 h, 10 μL of MTT (5 mg/mL) in PBS solution was added to each well. The wells were further incubated at 37 °C for 4 h. Next, 100 μl of dimethyl sulfoxide (DMSO) was added to dissolve the insoluble formazan crystals into the coloured solution. An enzyme-linked immunosorbent assay (ELISA) reader (Bio-Tek Instruments, Inc., Vinooski, VT) was used to measure the absorbance of each reaction mixture. The optical density of formazan formed in untreated cells (negative control) represents 100% cell viability. The experiment was done in triplicates with mean ± standard error calculated.
Results and discussion
Synthesis of nanoparticles
Three different Cardamom fruits were employed to synthesize AuNps and AgNps with different capabilities as observed by UV-Vis spectrophotometer. As mentioned previously, 70% fruit extracts were employed for the synthesis of nanoparticles. Biosynthesized nanoparticles by fresh and 5-year old fruits of A. villosum demonstrated major absorbance peaks in the region of characteristic peaks of AuNps in . The formation of FA-AuNps was observed within 3 s of reaction at room temperature, as the reaction mixture turned to a complete purple colour almost instantaneously which was attributed due to the collective oscillations of electrons in the mixture upon interactions with light (surface plasmon resonance (SPR)) [Citation16]. The change in reaction mixture indicates the formation of FA-AuNps and the efficient reduction of Au3+to Au0 (). However, no colour change and SPR peak spectra were observed in the reaction mixture consisting of aqueous extract of fresh E. cardamomum; the spectrum was flat with no apparent peak. The sharpest and the highest absorbance peak was obtained for 5-year old A. villosum.
Figure 1. UV-Vis spectra of (a) FA-AuNps, (b) FA-AgNps and (c) aqueous plant extracts. Insets show the colour conversion of reaction mixtures into FA-AuNps (purple) and FA-AgNps (brown).
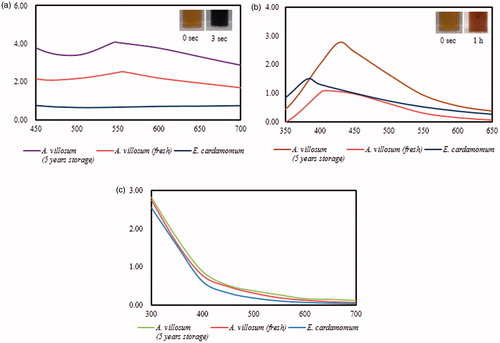
On the other hand, the reaction mixture containing silver salt changed to dark brown colour at 80 °C () in 1 h for all fruit extracts albeit with different absorbance values and SPR wavelengths (λmax). The presence of FA-AgNps was indicated by the dark brown colour due to SPR in the reaction mixture. Similarly, the sharpest and the highest absorbance peak was obtained for 5-year old A. villosum. Smaller absorbance values, significant shifts of λmax, and broader peaks (i.e. polydispersity) were observed in for AgNps synthesized by fresh A. villosum and E. cardamom; smaller absorbance values indicate the reduced concentration of AgNps in the reaction mixtures while λmax shifts to a lower wavelength and broader peaks may indicate the presence of small and polydisperse nanoparticles in the reaction mixtures. Narrow and sharp peaks can indicate a narrow size distribution and are desirable for bio-imaging applications due to their increased sensitivity as bio-sensors [Citation17]. The aqueous fruit extract solutions were maintained as negative controls; they remained the same colour in room temperature and at 80 °C. Spectra of plant extract solutions () indicated that the extracts did not contribute to the SPR peaks observed in the presence of FA-AuNps and FA-AgNps. For further experiments, 5-year old A. villosum functionalized nanoparticles were characterized and explored for their potential biological applications. Biosynthesis using plant extract is comparatively fast in comparison with bacteria and fungi, where the synthesis can take up to 48 h [Citation18,Citation19].
Characterization of nanoparticles
UV-vis spectra analysis
UV–Vis spectroscopy is a significant technique to define the existence, formation and stability of metal nanoparticle in suspension. The reduction of these metal ions was verified by UV-Vis spectroscopy analysis which indicated a highest peak at 550 nm and 428 nm for gold and silver mixtures, respectively, by 5-year old A. villosum. The recorded peaks are in a typical range of AuNps and AgNps peaks () [Citation20,Citation21].
FE-TEM analysis
FE-TEM measurements have been utilized to demonstrate various shapes and sizes of metal nanoparticles. Thus, in the present study FA-AuNps and FA-AgNps were analyzed by FE-TEM, which clearly showed the spherical shape of FA-AuNps (] and FA-AgNps () with varying sizes of 5–10 nm and 5–15 nm, respectively
EDX is a very supportive instrument to characterize nanoparticles as it provides elemental analysis or chemical characterization of a suspension sample. Chemical composition of FA-AuNps and FA-AgNps synthesized by Fructus Amomi extract was analyzed by measuring the energy and intensity of X-rays [Citation22]. The presence of elemental gold and silver in the reaction mixture was confirmed by EDX analysis observing strong signals from the gold and silver atoms in the nanoparticles at 2.3 keV and 3 keV accordingly (), which are characteristic peaks of metallic gold and silver nanocrystals. The results were coincident with previous results of EDX spectra of biosynthesized AuNps and AgNps [Citation14,Citation16]. Other signals originated from carbon and copper due to TEM grid.
The distribution of nanoparticles analyzed by elemental mapping was clearly visible in the electron image and was found to be the predominant element in the nanoparticles (). The SAED pattern of purified spherical FA-AuNps corresponded to the [111], [200], [220], and [311] lattice plane of Bragg’s reflections (). Correspondingly, the SAED pattern for FA-AgNps showed [111], [200], [111] and [220] lattice plane of Bragg’s reflections ().
XRD analysis
The crystallinity of FA-AuNps and FA-AgNps were analyzed by XRD. The resulting XRD spectra exhibited four intense peaks for FA-AuNps and seven peaks for FA-AgNps [Citation23]. The four characteristic peaks of FA-AuNps were indexed to [111], [200], [220], [311] and [222] lattice planes in the whole spectrum of 2θ value ranging from 20 to 80 (). The diffraction peaks at 2θ = 38.49° [111], 44.44° [200], 65.07° [220] and 77.82° [311] obtained were similar to those reported for the standard gold metal (Joint Committee on Powder Diffraction Standards-JCPDS, USA). Hence, these patterns suggested that the structure of FA-AuNps was face-centred cubic and crystalline in nature. The average size of FA-AuNps was calculated by Scherrer’s equation and determined to be 3.63 nm, as shown in Supplementary Table 1. The intensity ratio between the [200] and the [111] diffractions (I[200]/I[111]) (0.50) for the prepared sample was smaller than the conventional bulk intensity ratio (∼0.53), suggesting that the [111] plane was the predominant orientation. Earlier similar study about biologically synthesized AuNps showed identical XRD patterns, such as Hibiscus rosasinensis and olive leaf [Citation24,Citation25].
Interestingly, the XRD pattern of FA-AgNps confirmed the existence of both silver and silver chloride nanocrystals as evidenced by the peaks at 2θ values (). Silver nanocrystal peak corresponds to 38.20° [111] with the average size of 4.77 nm. Silver chloride nanocrystal peaks correspond to 27.89° [111], 32.33° [200], 46.27° [220], 55.00° [311], 57.40° [222] and 76.80° [420] with the average size of 10.62 nm, as demonstrated in Supplementary Table 2 [Citation26].
Particle size distribution profile analysis
The distribution profile of the biosynthesized FA-AuNps and FA-AgNps was studied by particle size analyzer using DLS pattern detecting intensity, number and volume. The DLS particle size analysis revealed that the z-average hydrodynamic diameters of FA-AuNps and FA-AgNps were approximately 269.9 and 256.7 nm, respectively. Intensity (size) distribution histogram revealed a wide range of gold particle size distribution varying from 80 to 1100 nm with PDI 0.237 (). The FA-AgNps intensity histogram in its turn, revealed the size in diapason of 85–850 nm with PDI 0.246 (). In consonance with TEM images, XRD pattern and DLS analysis, nanoparticles synthesized by Fructus Amomi were not entirely monodisperse. The discrepancy in the average diameter obtained from the Scherrer’s equation and z-average hydrodynamic diameter from the DLS analysis particle size analysis could be featured to the fact that the hydrodynamic diameter comprising of plant biomolecule coatings on the nanoparticle surface in aqueous suspension, whereas the crystallite diameter measured by XRD is solely its core diameter in dry form [Citation8,Citation14].
Fourier transform-infrared (FT-IR) spectroscopy profile
FTIR spectroscopy studies investigated the qualitative analysis of organic molecules present in the A. villosum fruit aqueous extract. FTIR spectrum was recorded from 500 to 4500 cm−1 ()). Correlating the FTIR spectrum of extract with FA-AuNps and FA-AgNps before and after synthesis, the wave numbers were shown at 3375.45, 2928.54, 1608.89, 1022.17 cm−1 are due to the stretching bonds of primary –OH alcohol functional group, aliphatic –CH, C = C and alcohol C–O groups, respectively [Citation27]. Strong characteristic peaks of primary alcohol groups, aliphatic bonds and carbonyl stretches suggest that phenols (bonded to a hydroxyl group − OH), tannins (bound to and precipitated proteins and alkaloids) and terpenoids (the combination of several isoprene units) are responsible for the formation of capping layers on the metal nanoparticles and preventing their agglomeration. Similar characteristic peaks of FT-IR spectra were also observed in the previously published reports [Citation27].
Applications of nanoparticles
DPPH radical scavenging assay
Free radicals are atoms or groups of atoms with unpaired number of electrons and can be formed if oxygen interrelates with certain molecules and it is highly reactive. Thus, free radicals always rush to form stable bond, by losing or gaining of an unpaired electron. Since, AuNps and AgNps preponderantly exist in two oxidation states such as Au+1, Au+3 and Ag+1 and Ag+2, they ready to accept or donate electrons or hydrogen to create a strong bond based on existing oxidation state. DPPH reducing ability of FA-AuNps and FA-AgNps was determined as colour changed from black to colourless within 30 min and recorded at 517 nm by ELISA reader. The scavenging activities increased as the dose became higher and showed compatible results with ascorbic acid. The percentages of scavenging ability recorded for lowest concentration of nanoparticles (100 μg/mL) were 15.41 ± 0.97 and 15.85 ± 0.18 for FA-AuNps and FA-AgNps, respectively (). When concentration of FA-AgNps was increased to 500 μg/mL, DPPH radical percentage inhibition increased to 67.89 ± 1.47. The IC50 value was indicated graphically and determined to be 1172.24 μg/mL and 311.53 μg/mL for FA-AuNps and FA-AgNps, respectively (). The percentage inhibition was higher in FA-AgNps compared with FA-AuNps, which could relate to the fact that silver acts as a good oxidant and can lose electrons easier than gold. Therefore, this research claims that the biosynthesized FA-AuNps and FA-AgNps can be acknowledged as novel antioxidant agents.
Catalytic activity of nanoparticles on reduction of methylene blue
One of the potential applications of AuNps and AgNps is the catalysis of some reactions that would not occur otherwise. Thus, the catalytic activity of biosynthesized FA-AuNps and FA-AgNps were tested using methylene blue. Methylene blue (MB) is broadly used as a redox (reduction–oxidation reaction) indicator in analytical chemistry. Fluids of this substance are deep blue in an oxidizing environment, but it will become colourless if it is added to a reducing agent. Hence, the present study examined the reduction of MB by the natural green aqueous fruit extract of Fructus Amomi containing FA-AuNps and FA-AgNps. After 30 min the absorbance value of pure MB was measured using UV-Vis spectrophotometer and registered a peak at λmax 664 nm. Also, after 30 min the reactions of plant extract mixing into MB, plant extract and FA-AuNps/FA-AgNps mixing into MB were measured. The absorbance peak of plant extract and MB solution was visibly decreased by 35% and shifted to lower wavelength. The reaction containing MB, extract and FA-AgNps, showed a decrease in absorbance peak after 30 min by 40%. In , it was recorded that the most remarkable decrease by 44% was observed in the mixture of MB, plant extract and FA-AuNps. This decrease in absorbance peak demonstrates that the metal nanoparticles have the potential to reduce MB. This shows that nanoparticles can act as an electron transfer mediator, as it can accept electrons from extract and donate electrons for MB by acting as an effective redox catalyst, which is also known as electron relay effect [Citation15,Citation28].
Antimicrobial activity of FA-AgNps
As shown from the results, FA-AgNps exhibited antimicrobial activity against E. coli and S. aureus as demonstrated by visible zones of inhibition on (). Triplicates were conducted and their averages and standard errors were calculated as shown in . FA-AgNps showed dose-dependent activity as concentration of nanoparticles was increased from 15 to 45 μg/disc. Nanoparticles were conjointly compared against commercial Neomycin at a concentration of 30 μg/disc. Biosynthesized FA-AgNps demonstrated larger zones of inhibition at equal concentrations of 30 μg (S. aureus and E. coli: 1.5 ± 0.11 mm and 1.33 ± 0.33 mm wider than Neomycin, respectively). This activity clearly indicated that the FA-AgNps endowed cell inhibition against both Gram-positive and Gram-negative bacteria. In other words, AgNps were more effective in inhibiting the growth of pathogenic bacteria than commercial antibiotics.
Figure 6. Antimicrobial activity of FA-AgNps against (a) Staphylococcus aureus and (b) Escherichia coli.
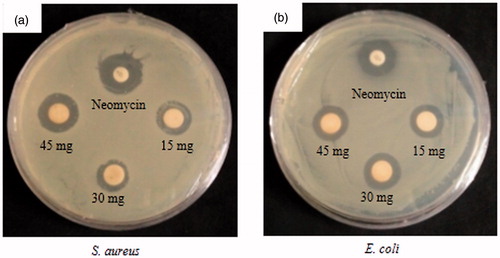
Table 1. Diameter of zone of inhibition (mm) of samples (30 μL) containing purified FA-AgNps.
Multiple reports have effectively reported the antimicrobial activity of silver against various pathogens [Citation9,Citation29]. Here, we demonstrated that our nanoparticles exhibited more potent antibactericidal effect than Neomycin. The mechanism as to how AgNps can effectively inhibit the growth of bacteria is not yet elucidated. However, few studies have shed some lights with hypotheses: (i) all silver nanoparticles which have the diameter in the range of ∼1–10 nm attach to the bacteria’s cell membrane surface and intervene in its function; (ii) to interact with bacteria they should be spherical shape and (iii) nanoparticles are able to invade into the bacteria and trigger damage by probably collaborating with sulphur- and phosphorus-containing compounds [Citation30].
In vitro cytotoxicity of nanoparticles
The in vitro cytotoxicity of FA-AuNps and FA-AgNps were examined in murine macrophage (RAW264.7) and human breast cancer cell lines (MCF7) by MTT assay as shown in . RAW264.7and MCF7 cell lines were treated with increasing concentration of nanoparticles for 48 h. As shown by in vitro MTT assays, FA-AuNps treated RAW264.7 cells did not show significant cytotoxicity at concentration less than 50 μg/mL where the cell viability was recorded at 80%, meanwhile they were toxic to MCF7 cells at a dosage of 25 μg/mL. FA-AuNps inhibited the viability of breast cancer cell line in a dose-dependent manner until 25 μg/mL. However, no further inhibition was observed at 50 μg/mL. FA-AuNps can be effectively utilized as drug-delivery agents to inhibit the growth of breast cancer cells at 25 μg/mL where it is non-cytotoxic to normal cell line (RAW264.7). Non-cytotoxicity can be attributed to the protective capping layer by A. villosum. Moreover, FA-AgNps demonstrated significant cytotoxicity against RAW264.7 and MCF7 cells at 100 μg/mL and at a lower dosage of 50 μg/mL, respectively. When the concentration of FA-AgNps was increased to 100 μg/mL, no additional cytotoxicity was significantly observed in RAW264.7 cells. Higher resistance to FA-AgNps mediated cell inhibition was observed in breast cancer cell line. Cell inhibition began to be observed starting at 50 μg/mL. Based on the MTT results, FA-AuNps demonstrated better potential than FA-AgNps against breast cancer cells.
Conclusions
FA-AuNps and FA-AgNps have been conveniently synthesized by a rapid and green method using aqueous extract of Fructus Amomi. The methodology of the green synthesis was innocuous, ecologically benign, and inexpensive. The resulting nanoparticles were thoroughly characterized and analyzed in vitro for antioxidant, catalytic, antimicrobial and cytotoxicity activities. Both FA-AuNps and FA-AgNps demonstrated free radical scavenging activity against DPPH. Additionally, nanoparticles synthesized by Fructus Amomi successfully reduced methylene blue. FA-AgNps showed larger zones of inhibition against pathogenic E. coli in comparison with S. aureus. Finally, FA-AuNps demonstrated better potential than FA-AgNps against breast cancer cells as evaluated by MTT assays. In conclusion, FA-AuNps and FA-AgNps have the potential as novel antioxidant, catalytic, and antimicrobial agents due to the surface capping provided by aqueous extract of Fructus Amomi. In addition, these nanoparticles inherently possess the ability to inhibit the proliferation of breast cancer cells without significantly repressing the growth of healthy cells. Due to these unique biological activities and a more environmentally friendly synthesis, FA-AuNps and FA-AgNps offer a better alternative than the existing AuNps and AgNps manufactured by physical and chemical methods for human applications.
Veronika_Soshnikova_et_al_supplementary_content.docx
Download MS Word (15 KB)Acknowledgements
This research was supported by a grant from the Next-Generation BioGreen 21 Program (SSAC, grant#: PJ0120342016), Rural Development Administration, and also supported by the Korea Institute of Planning and Evaluation for Technology in Food, Agriculture, Forestry and Fisheries, Republic of Korea (KIPET NO: 316065–3).
Disclosure statement
The authors report no declarations of interest. The authors alone are responsible for the content and writing of the paper.
Additional information
Funding
References
- Singh P, Kim Y-J, Zhang D, et al. Biological synthesis of nanoparticles from plants and microorganisms. Trends Biotechnol. 2016;34:588–599.
- Paulkumar K, Rajeshkumar S, Gnanajobitha G, et al. Eco-friendly synthesis of silver chloride nanoparticles using Klebsiella planticola (MTCC 2277). Int J Green Chem Bioprocess. 2013;3:12–16.
- Wang C, Singh P, Kim Y-J, et al. Characterization and antimicrobial application of biosynthesized gold and silver nanoparticles by using Microbacterium resistens. Artif Cells Nanomed Biotechnol. 2016;44:1714–1721.
- Daniel M-C, Astruc D. Gold nanoparticles: assembly, supramolecular chemistry, quantum-size-related properties, and applications toward biology, catalysis, and nanotechnology. Chem Rev. 2004;104:293–346.
- Ahmed S, Ahmad M, Swami BL, et al. A review on plants extract mediated synthesis of silver nanoparticles for antimicrobial applications: a green expertise. J Adv Res. 2016;7:17–28.
- Singh P, Kim Y-J, Wang C, et al. Weissella oryzae DC6-facilitated green synthesis of silver nanoparticles and their antimicrobial potential. Artif. Cells Nanomed. Biotechnol. 2016;44:1569–1575.
- Singh P, Kim Y-J, Wang C, et al. The development of a green approach for the biosynthesis of silver and gold nanoparticles by using Panax ginseng root extract, and their biological applications. Artif Cells Nanomed Biotechnol. 2015;44:1150–1157.
- Abbai R, Mathiyalagan R, Markus J, et al. Green synthesis of multifunctional silver and gold nanoparticles from the oriental herbal adaptogen: Siberian ginseng. Int J Nanomedicine. 2016;11:3131
- Wang C, Kim YJ, Singh P, et al. Green synthesis of silver nanoparticles by Bacillus methylotrophicus, and their antimicrobial activity. Artif Cells Nanomed Biotechnol. 2015;44:1127–1132.
- Lamxay V, Newman M. A revision of Amomum (Zingiberaceae) in Cambodia, Laos and Vietnam. Edinb J Bot. 2012;69:99–206.
- Li G, Chen A, Chen X, et al. First report of Amomum villosum (cardamom) leaf lesion caused by Pyricularia costina in China. New Dis Rep. 2010;22:2.
- Beristain C, Garcıa H, Vernon-Carter E. Spray-dried encapsulation of cardamom (Elettaria cardamomum) essential oil with mesquite (Prosopis juliflora) gum. LWT-Food Sci Technol. 2001;34:398–401.
- Jamal A, Javed K, Aslam M, et al. Gastroprotective effect of cardamom, Elettaria cardamomum Maton. fruits in rats. J Ethnopharmacol. 2006;103:149–153.
- Markus J, Mathiyalagan R, Kim Y-J, et al. Intracellular synthesis of gold nanoparticles with antioxidant activity by probiotic Lactobacillus kimchicus DCY51T isolated from Korean kimchi. Enzyme Microb Tech. 2016;95:85–93.
- Edison TJI, Sethuraman M. Instant green synthesis of silver nanoparticles using Terminalia chebula fruit extract and evaluation of their catalytic activity on reduction of methylene blue. Process Biochem. 2012;47:1351–1357.
- Gnanajobitha G, Annadurai G, Kannan C. Green synthesis of silver nanoparticle using Elettaria cardamomom and assesment of its antimicrobial activity. Int J Pharma Sci Res. 2012;3:323–330.
- Cobley CM, Skrabalak SE, Campbell DJ, et al. Shape-controlled synthesis of silver nanoparticles for plasmonic and sensing applications. Plasmonics. 2009;4:171–179.
- He S, Guo Z, Zhang Y, et al. Biosynthesis of gold nanoparticles using the bacteria Rhodopseudomonas capsulata. Mater Lett. 2007;61:3984–3987.
- Sastry M, Ahmad A, Khan MI, et al. Biosynthesis of metal nanoparticles using fungi and actinomycete. Curr Sci. 2003;85:162–170.
- Vigneshwaran N, Nachane R, Balasubramanya R, et al. A novel one-pot ‘green’synthesis of stable silver nanoparticles using soluble starch. Carbohydr Res. 2006;341:2012–2018.
- Kumar V, Yadav SK. Plant‐mediated synthesis of silver and gold nanoparticles and their applications. J Chem Technol Biotechnol. 2009;84:151–157.
- Ramamurthy C, Padma M, Mareeswaran R, et al. The extra cellular synthesis of gold and silver nanoparticles and their free radical scavenging and antibacterial properties. Colloids Surf B Biointerfaces. 2013;102:808–815.
- Bhainsa KC, D'souza S. Extracellular biosynthesis of silver nanoparticles using the fungus Aspergillus fumigatus. Colloids Surf B Biointerfaces. 2006;47:160–164.
- Philip D. Green synthesis of gold and silver nanoparticles using Hibiscus rosa sinensis. Physica E Low Dimens. Syst Nanostruct. 2010;42:1417–1424.
- Khalil MM, Ismail EH, El-Magdoub F. Biosynthesis of Au nanoparticles using olive leaf extract: 1st nano updates. Arabian J Chem. 2012;5:431–437.
- Choi M, Shin K-H, Jang J. Plasmonic photocatalytic system using silver chloride/silver nanostructures under visible light. J Colloid Interface Sci. 2010;341:83–87.
- Sukandar D, Hermanto S, Amelia ER, et al. Antibacterial activity of Amomum compactum Sol. Ex Maton extract. Indonesian J Appl Chem. 2015;17:119–129.
- Tripathi R, Kumar N, Shrivastav A, et al. Catalytic activity of biogenic silver nanoparticles synthesized by Ficus panda leaf extract. J Mol Catal B Enzym. 2013;96:75–80.
- Singh P, Kim Y-J, Singh H, et al. Biosynthesis of anisotropic silver nanoparticles by Bhargavaea indica and their synergistic effect with antibiotics against pathogenic microorganisms. J. Nanomater. 2015;2015:4.
- Morones JR, Elechiguerra JL, Camacho A, et al. The bactericidal effect of silver nanoparticles. Nanotechnology. 2005;16:2346