Abstract
Monodisperse silica microspheres with bimodal pore-size distribution were proposed as a high performance sorbent for DNA isolation in batch fashion under equilibrium conditions. The proposed sorbent including both macroporous and mesoporous compartments was synthesized 5.1 μm in-size, by a “staged shape templated hydrolysis and condensation method”. Hydrophilic polymer based sorbents were also obtained in the form of monodisperse-macroporous microspheres ca 5.5 μm in size, with different functionalities, by a developed “multi-stage microsuspension copolymerization” technique. The batch DNA isolation performance of proposed material was comparatively investigated using polymer based sorbents with similar morphologies. Among all sorbents tried, the best DNA isolation performance was achieved with the monodisperse silica microspheres with bimodal pore size distribution. The collocation of interconnected mesoporous and macroporous compartments within the monodisperse silica microspheres provided a high surface area and reduced the intraparticular mass transfer resistance and made easier both the adsorption and desorption of DNA. Among the polymer based sorbents, higher DNA isolation yields were achieved with the monodisperse-macroporous polymer microspheres carrying trimethoxysilyl and quaternary ammonium functionalities. However, batch DNA isolation performances of polymer based sorbents were significantly lower with respect to the silica microspheres.
Introduction
DNA isolation has been widely used for nucleic acid-based clinical and forensic analysis of biological samples. The most suitable method is based on the selective adsorption of DNA onto a stationary phase induced by chaotropic salt and desorption by using an appropriate buffer [Citation1,Citation2].
The sorbents with polar functional groups in the hydrophilic form mostly provide DNA isolation with high efficiency. Silica based sorbent has a great importance in DNA isolation studies from different sources. These sorbents include silica-based neutral or cationic particles and monoliths (i.e. bare silica gel, or silica gel with aminopropyl or quaternary ammonium groups) [Citation3–9].
In the past few years, various new sorbents were proposed to isolate DNA from different biological sources. DNA isolation was also performed with high efficiency by using amino-modified silica-coated magnetic nanoparticles at high concentrations of phosphate buffer [Citation10]. DNA isolation was performed by using polyethyleneimine (PEI) functionalized FePO4 nanoparticles with high efficiency in a very short time via electrostatic interactions [Citation11]. Ionic liquid-modified Fe3O4 nanoparticles were also used as a sorbent to investigate the effects of DNA concentration, pH of the sample solution, ionic strength, temperature and contact time in DNA isolation [Citation12]. Wang et al. developed haemoglobin modified magnetic nanocomposite providing efficient and easy DNA isolation [Citation13].
Polyacrylate/methacrylate-based sorbents have been widely used for DNA isolation due to their high specific surface area, suitable hydrophilicity and functional groups that can create effective reversible interaction with DNA [Citation14–19]. Methacrylate based monolith was prepared by bearing diethylaminoethyl (DEAE) and butyl groups and used as a sorbent for selective extraction of DNA [Citation20]. Matos et al. used a chromatography medium (resin) to isolate plasmid DNA [Citation21]. The magnetic hybrid nanocomposites were synthesized to perform simple and fast DNA separation [Citation22]. Hydrophilic non-porous magnetic microspheres containing carboxyl groups were tried for the isolation of DNA from different food matrices and the highest isolation was achieved with the microspheres containing high content of carboxyl groups within the medium containing polyethylene glycol and NaCl [Citation23]. The hydrophobic sorbents or the sorbents with anionic groups lead to the formation of a strong connection between the sorbent and DNA by strong electrostatic attraction or hydrophobic interactions. This case causes a decrease in the DNA isolation yield. Thus, DNA desorption can be performed with low efficiency [Citation24–26].
Both the surface chemistry and the porous properties of the sorbent can be considered as the most effective factors controlling the reversible adsorption/desorption behaviour and then the isolation yield of DNA. The porous properties (i.e. mean pore size, porosity, pore size distribution and specific surface area) of polymer microspheres can be adjusted by controlling the synthesis conditions. The surface chemistry of the sorbent dominantly depends on both the type of base material and the ligand used for the derivatization of the base material. Recently, we focused on the synthesis of silica and polymer based sorbents in the form of monodisperse-porous microspheres with different chemistries and porous properties. A new synthesis protocol for “monodisperse-porous silica microspheres containing both mesoporous and macroporous compartments” was developed. In our previous study, DNA isolation behaviour of monodisperse-porous silica microspheres with bimodal pore-size distribution was comparatively investigated under “non-equilibrium (dynamic) conditions in a microfluidic separation system”, using mesoporous silica and macroporous polymer microspheres as the potential sorbents for comparison [Citation27]. In this study, DNA adsorption and desorption characteristics of monodisperse-porous silica microspheres were comparatively investigated using the silica and polymer based sorbents, under “equilibrium conditions in batch fashion”. In the batch DNA isolation runs, the effects of DNA feed concentration, pH and sorbent concentration on the DNA isolation yield and desorption yield under equilibrium conditions were determined.
Experimental methods
Materials
The functional monomers, 2-[(methacryloylox)ethyl] trimethylammonium chloride (METMA-Cl) glycerol 1,3-diglycerolate diacrylate (GDGDA), 3-trimethoxysilylpropyl methacrylate (TMSPM) and crosslinking agents, glycerol dimethacrylate (GDMA) and ethylene dimethacrylate (EDMA) were supplied from Aldrich Chem. Co. (Sheboygan Falls, WI) and used without further purification. Tetraethyl orthosilicate (TEOS) was also supplied from Aldrich (Sheboygan Falls, WI). 1-(3-sulphopropyl)-2-vinylpyridiniumbetaine (SVP) were obtained from Across Organics (London, UK). Deoxyribonucleic acid sodium salt from calf thymus (D1501) was obtained from Sigma Chem. Co. (St. Louis, MO).
Synthesis and characterization of monodisperse-porous silica and polymer based microspheres
The synthesis of monodisperse silica microspheres with bimodal pore-size distribution was performed by a newly developed staged-shape templated hydrolysis and condensation protocol according to the procedure described elsewhere [Citation27]. Polydisperse-mesoporous silica microspheres were obtained by the hydrolysis and condensation of TEOS in the presence of an acid catalyst within distilled water [Citation28]. Monodisperse-porous polymer microspheres with five different chemistries were obtained by a “multi-stage microsuspension polymerization” using different functional monomers and GDMA or EDMA as the crosslinking agent [Citation27].
DNA adsorption and desorption experiments under equilibrium conditions in batch fashion
The adsorption medium was prepared by dissolving DNA with a prescribed concentration in 1× TE buffer including 6 M Gu–HCl at pH 6.0. The absorbance corresponding to the initial DNA concentration in the adsorption medium was then measured with a micro UV-spectrophotometer at 260 nm (Nanodrop, ND, Thermoscientific, Waltham, MA). The particulate sorbent used in the batch adsorption run (10 mg) was washed three times with elution buffer (1 mL, 1× TE buffer at pH 8.0) and three times with the adsorption buffer (1 mL, 1× TE buffer including 6 M Gu–HCl at pH 6.0) by a protocol containing successive centrifuging and decantation steps. The sorbent (10 mg) was then dispersed by vortexing within the adsorption medium containing DNA with a certain concentration. For adsorption of DNA onto the sorbent, the adsorption medium was stirred magnetically at 200 rpm for 2 h at room temperature. At the end of the adsorption period, the sorbent was separated from the adsorption medium by centrifugation and the liquid part was analysed for the DNA remained in the liquid compartment after the adsorption. DNA concentration in the supernatant was determined by measuring the absorbance at 260 nm (Af). The sorbent isolated by centrifugation was then redispersed within the elution medium (1 mL, 1× TE buffer at pH 8.5). The elution medium was stirred at 200 rpm for 1 h, at room temperature. The resulting DNA concentration in the elution medium (Ce) was determined by measuring the absorbance of the desorption medium at 260 nm (Ae). The DNA concentrations determined by micro-UV spectrophotometer were also checked using Qubit florescence assay (Qubit 2.0 Fluorometer, Q32866-Invitrogen, Thermo Fisher Scientific, Waltham, MA).
DNA adsorbed at equilibrium (Q, ng DNA/mg dry particles) was calculated based on the following expression (EquationEquation (1)(1) ).
(1) where Co: initial DNA concentration (ng DNA/μL medium); VA: volume of the adsorption buffer (μL); Mm: amount of sorbent (mg).
The desorption yield was calculated as the ratio of amount of DNA desorbed (mg) to the amount of DNA adsorbed on the particles (mg) and calculated based on the following expression (EquationEquation (2)(2) ).
(2)
The isolation yield was determined as the ratio of amount of DNA desorbed (mg) to the DNA loaded into the adsorption medium (mg) (EquationEquation (3)(3) ).
(3)
Results and discussion
Characterization of sorbents
In this study, the DNA isolation behaviour of a new sorbent, the so-called “monodisperse-porous silica microspheres with bimodal pore size distribution”, obtained by a developed “staged-shape templated hydrolysis and condensation” protocol was comparatively investigated using both mesoporous silica and macroporous polymer microspheres in the monodisperse form, in batch-fashion. The physical and chemical characterizations of silica and polymer based microspheres were performed in our previous study and some important properties are given in [Citation27]. Monodisperse silica microspheres with mesoporous and macroporous compartments were obtained with mean particle size of 5.1 μm and a mean pore size of 12.5 nm [Citation27]. Polydisperse-mesoporous silica microspheres were synthesized by a modified form of hydrolysis-condensation protocol, with a particle size of 4.2 μm and a mean pore size of 3.5 nm [Citation28]. As polymer based sorbents, the monodisperse-porous polymer microspheres were synthesized by multistage microsuspension copolymerization of hydrophilic, methacrylate based functional monomers (i.e. GDGDA, TMSPM, METMA-Cl and SVP) with hydrophilic methacrylate based crosslinking agents (i.e. GDMA and EDMA) (). In these runs, monodisperse poly(TMSPM-co-EDMA), poly(GDGDA-co-GDMA), poly(METMA-Cl-co-GDMA) and poly(SVP-co-GDMA) microspheres with the mean size values ranging between 4 and 6 μm were obtained with a porous structure containing only macroporous compartment () [Citation27]. As expected, silica based microspheres, both including the mesoporous compartment had higher SSA values with respect to the macroporous polymer microspheres ().
Table 1. The types and properties of particulate stationary phases used for DNA microextraction in microfluidic system [Citation27].
Equilibrium DNA isolation behaviours of silica and polymer based microspheres
The effect of pH on the equilibrium DNA adsorption onto the polymer and silica based microspheres is given in . As seen here, the highest DNA adsorption was observed at pH 6 for most of the microspheres studied. By considering this finding, the effects of other conditions on the equilibrium DNA adsorption onto the polymer and silica based microspheres were investigated at pH 6. This value was also used as the most appropriate pH in various studies aiming the isolation of DNA from different sources [Citation3,Citation5,Citation7,Citation9].
Figure 1. The effect of pH on the equilibrium DNA adsorption onto the silica and polymer based microspheres. Initial DNA concentration: 400 ng/μL, sorbent concentration: 10 mg/mL. Adsorption time: 2 h, room temperature, stirring rate: 250 rpm.
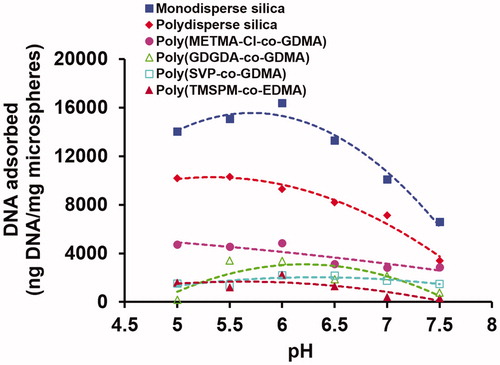
The effect of initial DNA concentration on the equilibrium DNA adsorption onto the polymer and silica based microspheres is given in . For a certain initial DNA concentration, the highest equilibrium DNA adsorption was obtained with the monodisperse silica microspheres containing both mesoporous and macroporous compartments. Although SSA of polydisperse-mesoporous silica was about 2.0 times higher than that of the monodisperse one (), the equilibrium DNA adsorption obtained with the polydisperse mesoporous silica microspheres was lower with respect to that obtained with the monodisperse silica microspheres. This behaviour was observed for all DNA initial concentrations studied. Note that most of the parking area necessary for DNA adsorption onto the sorbent should be provided by the mesopores. In the pore size distribution measurements performed by nitrogen adsorption/desorption method, the mean pore size was measured as 3.5 and 12.5 nm for polydisperse silica and monodisperse silica samples, respectively (). Hence higher DNA adsorption obtained with the monodisperse silica should be attributed to the higher usability of larger pores by large DNA molecules. Although the total mesoporous surface area was higher for polydisperse silica, some part of surface area could not be efficiently used by large DNA molecules due to the higher internal mass transfer resistance arising from smaller mesopores. The presence of macroporous compartment in the monodisperse silica should be considered as a factor making easier the intraparticular transport of DNA within the microsphere. The presence of macropores probably reduces the intraparticular diffusion resistance against the DNA transport within the microsphere and particularly larger mesopores can be more available for parking of DNA onto the surface. On the other hand, the lower equilibrium DNA adsorptions obtained with the macroporous polymer microspheres in can be explained by the lower SSA values of these sorbents with respect to the silica based ones.
Figure 2. The effect of initial DNA concentration on the equilibrium DNA adsorption onto the silica and polymer based microspheres. pH: 6.0, sorbent concentration: 10 mg/mL. Adsorption time: 2 h, room temperature, stirring rate: 250 rpm.
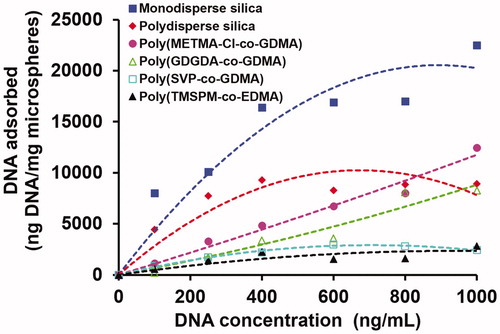
The genomic DNA adsorption behaviours of both silica and polymer based microspheres were investigated using different adsorption models including Langmuir, Freundlich and Temkin adsorption isotherms [Citation29,Citation30]. For this purpose, the equilibrium adsorption data reported in are used for performing curve-fittings for the selected adsorption isotherms. The related constants and the correlation coefficient values determined with the selected models are given in . In this table, the constants are described as follows:
Table 2. The constants and correlation coefficients determined with different adsorption isotherms using silica and polymer based microspheres as the sorbents for human genomic DNA adsorption.
Langmuir isotherm:
(4) where Ce = the equilibrium concentration of adsorbate (mg/L); qe = the amount of DNA adsorbed per gram of the adsorbent at equilibrium (mg/g); qm = maximum monolayer coverage capacity (mg/g); KL = Langmuir isotherm constant (L/mg).
Freundlich isotherm:
(5) where KF = Freundlich isotherm constant (mg/g); n = adsorption intensity; Ce = the equilibrium concentration of adsorbate (mg/L); qe = the amount of DNA adsorbed per gram of the adsorbent at equilibrium (mg/g).
Temkin isotherm:
(6) where A = Temkin isotherm equilibrium binding constant (L/g); R = universal gas constant (8.314 J/mol/K); T = temperature at 298 K; b = Temkin isotherm constant; B (RT/b) = constant related to heat of sorption (J/mol).
As seen in , physically meaningless (i.e. negative) qm values were found for poly(GDGDA-co-GDMA) and poly(METMA-co-GDMA) microspheres using the Langmuir model. The adsorption behaviour was more adequately described by the Freundlich model for these microspheres with relatively higher correlation coefficients. On the other hand, relatively higher correlation coefficients were found for most of the microspheres (i.e. polydisperse-mesoporous silica, poly(TMSPM-co-GDMA) and poly(SVP-co-GDMA) using the Langmuir model. In addition to this, although the correlation coefficient determined with Langmuir model was the lowest for monodisperse silica microspheres, the highest qm value indicating an excellent equilibrium adsorption capacity of the sorbent was obtained with this material. However, the adsorption behaviour was more adequately described by the Temkin model for monodisperse silica microspheres with relatively higher correlation coefficient and the highest “B” value.
The effect of sorbent concentration on the equilibrium DNA adsorption onto the polymer and silica based microspheres is shown in . As anticipated, the equilibrium adsorption of DNA decreased with the increasing sorbent concentration for all sorbent types. For relatively lower sorbent concentrations, the highest DNA adsorptions were observed with monodisperse silica. However, the DNA adsorption onto polydisperse silica was more insensitive to the sorbent concentration. For this reason, the DNA adsorptions with high sorbent concentrations were very close for polydisperse and monodisperse silica sorbents.
Figure 3. The effect of sorbent concentration on the equilibrium DNA adsorption onto the silica and polymer based microspheres. Medium pH: 6.0, initial DNA concentration: 400 ng/μL. Adsorption time: 2 h, room temperature, stirring rate: 250 rpm.
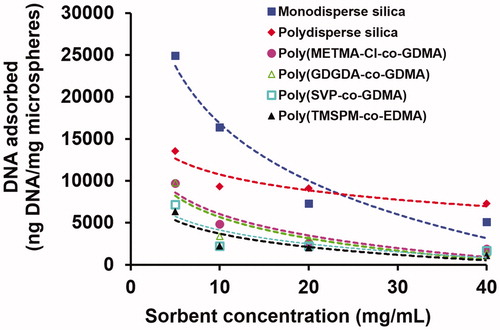
In the DNA desorption runs, the desorption medium commonly used in the previous studies, pH 8.5 TE buffer was used. The variation of DNA desorption yield with the sorbent type under equilibrium conditions is shown in . As seen here, the highest desorption yields were obtained with both types of silica microspheres. Among the polymer based ones, desorption yields close to the silica based sorbents were achieved with the neutral polymeric sorbent, poly(TMSPM-co-EDMA) microspheres carrying both trimethoxysilyl and hydroxyl groups formed by the hydrolysis of trimethoxysilyl residues and the cationic polymeric sorbent poly(METMA-Cl-co-GDMA) microspheres carrying anion-exchanger quaternary ammonium group. This behaviour showed that the non-specific interactions between DNA and sorbent surface were relatively weaker for both types of silica, poly(TMSPM-co-EDMA) and poly(METMA-Cl-co-GDMA) microspheres at slightly basic pH used for desorption. The lowest desorption yields were obtained with neutral poly(GDGDA-co-GDMA) microspheres with hydroxyl functionality and zwitterionic poly(SVP-co-GDMA) microspheres with sulphopyridinium betaine functionality.
Figure 4. The variation of DNA (A) desorption yield, (B) isolation yield and (C) amount of DNA isolated with the sorbent type. Desorption medium: Tris (10 mM)–EDTA (1 mM) buffer at pH 8.5. Adsorption conditions: initial DNA concentration: 400 ng/μL. Medium pH: 6.0, sorbent concentration: 10 mg/mL. Adsorption time: 2 h, room temperature, stirring rate: 250 rpm. Sorbent type: (1) monodisperse silica, (2) polydisperse silica, (3) poly(TMSPM-co-EDMA), (4) poly(METMA-Cl-co-GDMA), (5) poly(GDGDA-co-GDMA) and (6) poly(SVP-co-GDMA).
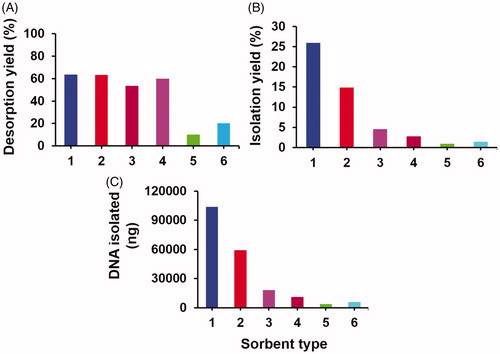
The variation of isolation yield and the isolated amount of DNA with the sorbent type are given in , respectively. The amount of DNA isolated and the isolation yield are clear indicators showing the performance of a DNA isolation sorbent. Among all sorbents, the highest amount of DNA isolated and the highest isolation yields were obtained with the monodisperse-porous silica microspheres with biomodal pore-size distribution. The isolation yield and the amount of DNA isolated were almost two times higher when the monodisperse-porous silica was used as sorbent with respect to the commonly used one, mesoporous silica. Hence this study showed that newly produced monodisperse silica microspheres having both mesoporous and macroporous compartments were a new type of efficient sorbent for DNA isolation in bath fashion. Relatively larger mean pore size and the presence of macropores should probably reduce the intraparticular mass transfer resistance (i.e. by lowering intraparticular diffusion limitation) during the desorption and make easier the removal adsorbed DNA from the sorbent. Higher intraparticular mass transfer resistance probably originated from very low mean pore size should be probably the reason for the lower desorption yield obtained with the mesoporous silica microspheres (). On the other hand, both the amounts of DNA isolated and the desorption yields were significantly lower with the polymer based sorbents with respect to the silica based sorbents. Although the desorption yields obtained with the poly(TMSPM-co-EDMA) and poly(METMA-Cl-co-GDMA) microspheres were relatively higher (), the insufficient surface area of these microspheres providing low DNA adsorption and stronger non-specific interactions between the polymer surface and DNA should be probably the factors causing low DNA isolation yields with these sorbents (). These effects should be probably much stronger for the poly(SVP-co-GDMA) and poly(GDGDA-co-GDMA) microspheres since very low isolation yields could be obtained by these sorbents.
The effects of sorbent concentration on both desorption yield and isolation yield of DNA are given in , respectively. As seen in , the desorption yields were higher for both types of silica, poly (TMSPM-co-EDMA) and poly(METMA-Cl-co-GDMA) microspheres for all sorbent concentrations. This behaviour was reasonably consistent with the behaviour given in observed with the sorbent concentration of 10 mg/mL. Note that the desorption yields obtained with poly(GDGDA-co-GDMA) and poly(METMA-Cl-co-GDMA) microspheres were close to the other sorbents when the sorbent concentration was kept at the highest level (i.e. 100 mg/mL). The behaviour observed in was very clear. The highest isolation yields were obtained with the monodisperse-porous silica microspheres. Although a marked difference (i.e. almost 80%) between the isolation yields of monodisperse silica microspheres and mesoporous silica microspheres was observed with the lowest sorbent concentration (i.e. 10 mg/mL), this difference became unimportant with the increasing sorbent concentration. However, this plot clearly showed the superiority of silica based sorbents with respect to the polymer based ones for DNA isolation under equilibrium conditions in batch fashion. As seen in , the isolation yields achieved with the polymer based sorbents were reasonably lower with respect to the silica based ones. Even the sorbent concentration was extremely increased, it was not possible to reach to the isolation yields achieved with the silica based ones under equilibrium conditions by using polymer based sorbents with neutral, cationic and zwitterionic functionalities. Very low equilibrium DNA adsorption mostly depending upon insufficient surface area should be probably the main limitation of the macroporous polymeric sorbents used for DNA isolation.
Figure 5. The variation of DNA (A) desorption yield and (B) isolation yield with the sorbent concentration. Desorption medium: Tris (10 mM)–EDTA (1 mM) buffer at pH 8.5. Sorbent concentration: 10–100 mg/mL (variable). Adsorption conditions: initial DNA concentration: 400 ng/μL. Medium pH: 6.0, sorbent concentration: 10 mg/mL. Adsorption time: 2 h, room temperature, stirring rate: 250 rpm. Sorbent type: (1) monodisperse silica, (2) polydisperse silica, (3) poly(TMSPM-co-EDMA), (4) poly(METMA-Cl-co-GDMA), (5) poly(GDGDA-co-GDMA) and (6) poly(SVP-co-GDMA).
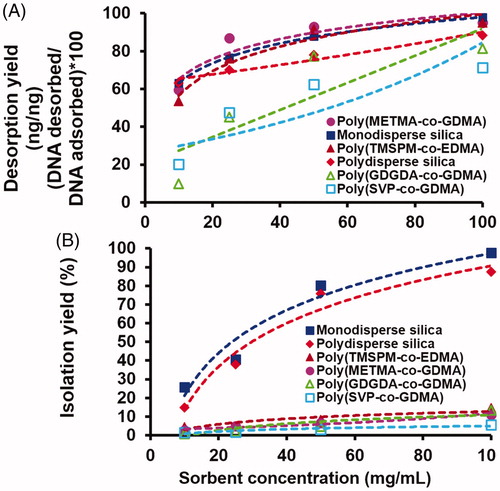
Conclusions
Monodisperse-porous silica microspheres with bimodal pore-size distribution were proposed as a new high performance sorbent for batch DNA isolation under equilibrium conditions. The high specific surface area originated from their mesoporous compartment and low intraparticular diffusion resistance depending upon the presence of macroporous compartment led to the higher equilibrium adsorption and easier desorption of DNA with the proposed sorbent. The low specific surface area and strong non-specific interactions between DNA and surface functional groups should be probably the main reasons of the extremely lower DNA isolation yields observed with the polymer based microspheres.
Disclosure statement
The authors report no declarations of interest.
Additional information
Funding
References
- Breadmore MC, Wolfe KA, Arcibal IG, et al. Microchip-based purification of DNA from biological samples. Anal Biochem. 2003;75:1880–1886.
- Ferreira GNM, Cabral JMS, Prazeres DMF. Studies on the batch adsorption of plasmid DNA onto anion-exchange chromatographic supports. Biotechnol Prog. 2000;16:416–424.
- Duarte GR, Price CW, Littlewood JL, et al. Characterization of dynamic solid phase DNA extraction from blood with magnetically controlled silica beads. Analyst. 2010;135:531–537.
- Gabor B, Cernigoj U, Barut M, et al. Reversible entrapment of plasmid deoxyribonucleic acid on different chromatographic supports. J Chromatogr A. 2013;1311:106–114.
- Nakagawa T, Tanaka T, Niwa D, et al. Fabrication of amino silane-coated microchip for DNA extraction from whole blood. J Biotechnol. 2005;116:105–111.
- Potrich C, Lunelli L, Pasquardini L, et al. One-shot genetic analysis in monolithic silicon/pyrex microdevices. Biomed Microdev. 2012;14:1103–1113.
- Price CW, Leslie DC, Landers JP. Nucleic acid extraction techniques and application to the microchip. Lab Chip. 2009;9:2484–2494.
- Sahoo SL, Liu CH. Adsorption behaviors of DNA by modified magnetic nanoparticles: effect of spacer and salt. Colloids Surf A Physicochem Eng Asp. 2015;482:184–194.
- Wu Q, Bienvenue JM, Hassan BJ, et al. Microchip-based macroporous silica sol–gel monolith for efficient isolation of DNA from clinical samples. Anal Chem. 2006;78:5704–5710.
- Shan Z, Jiang Y, Guo M, et al. Promoting DNA loading on magnetic nanoparticles using a DNA condensation strategy. Colloids Surf B Biointerfaces. 2015;125:247–254.
- Hu LL, Hu B, Shen LM, et al. Polyethyleneimine–iron phosphate nanocomposite as a promising adsorbent for the isolation of DNA. Talanta. 2015;132:857–863.
- Ghaemi M, Absalan G. Study on the adsorption of DNA on Fe3O4 nanoparticles and on ionic liquid-modified Fe3O4 nanoparticles. Microchim Acta. 2014;181:45–53.
- Chen XW, Mao QX, Liu JW, et al. Isolation/separation of plasmid DNA using hemoglobin modified magnetic nanocomposites as solid-phase adsorbent. Talanta. 2012;100:107–112.
- Cernigoj U, Vidic U, Barut M, et al. A multimodal histamine ligand for chromatographic purification of plasmid DNA. J Chromatogr A. 2013;1281:87–93.
- Mahut M, Gargano A, Schuchnigg H, et al. Chemoaffinity material for plasmid DNA analysis by high-performance liquid chromatography with condition-dependent switching between isoform and topoisomer selectivity. Anal Chem. 2013;85:2913–2920.
- Mota E, Sousa A, Cernigoj U. Rapid quantification of supercoiled plasmid deoxyribonucleic acid using a monolithic ion exchanger. J Chromatogr A. 2013;1291:114–121.
- Sabarudin A, Huang JC, Shu S, et al. Preparation of methacrylate-based anion-exchange monolithic microbore column for chromatographic separation of DNA fragments and oligonucleotides. Anal Chim Acta. 2012;736:108–114.
- Sousa A, Sousa F, Queiroz JA. Advances in chromatographic supports for pharmaceutical-grade plasmid DNA purification. J Sep Sci. 2012;35:3046–3058.
- Zhang HP, Bai S, Xu L. Fabrication of mono-sized magnetic anion exchange beads for plasmid DNA purification. J Chromatogr B. 2009;877:127–133.
- Smrekar V, Smrekar F, Strancar A, et al. Single step plasmid DNA purification using methacrylate monolith bearing combination of ion-exchange and hydrophobic groups. J Chromatogr A. 2013;1276:58–64.
- Matos T, Queiroz JA, Bulow L. Plasmid DNA purification using a multimodal chromatography resin. J Mol Recognit. 2014;27:184–189.
- Medina-Llamas JC, Chávez-Guajardo AE, Andrade CA, et al. Use of magnetic polyaniline/maghemite nanocomposite for DNA retrieval from aqueous solutions. J Colloid Interface Sci. 2014;434:167–174.
- Trachtová Š, Španová A, Tóth J, et al. Solid-phase DNA isolation from food matrices using hydrophilic magnetic microspheres. Food Bioprod Process. 2015;94:375–381.
- Carlson K, Flick L, Hall M. DNA adsorption onto calcium aluminate and silicate glass surfaces. Colloids Surf B Biointerfaces. 2014;117:538–544. 1.
- Krajnc NL, Smrekar F, Strancar A, et al. Adsorption behavior of large plasmids on the anion-exchange methacrylate monolithic columns. J Chromatogr A. 2011;1218:2413–2424.
- Reedy CR, Price CW, Sniegowski J, et al. Solid phase extraction of DNA from biological samples in a post-based, high surface area poly(methyl methacrylate) (PMMA) microdevice. Lab Chip. 2011;11:1603–1611.
- Günal G, Kip C, Öğüt SE, et al. Human genomic DNA isolation from whole blood in a simple microfluidic system with silica and polymer based stationary phases. Mater Sci Eng C Mater. 2017;74:10–20.
- Ma Y, Qi L, Ma J, et al. Large-pore mesoporous silica spheres: synthesis and application in HPLC. Colloids Surf A. 2003;229:1–8.
- Igwe JC, Abia AA. A bioseparation process for removing heavy metals from waste water using biosorbents. Afr J Biotechnol. 2012;5:1167–1179.
- Tempkin MI, Pyzhev V. Kinetics of ammonia synthesis on promoted iron catalyst. Acta Phys Chim. 1940;12:327–356.