Abstract
Exosomes is a key component of cell paracrine secretion and can exert important effects in various disease models. However, the role of exosomes in neuron repair of spinal cord injury (SCI) has rarely been reported. In this study, Exosomes were isolated from cerebrospinal fluid of SCI and normal, and incubated neuron in vitro respectively to research its biological function in cell proliferation. The results demonstrated these exosomes all expressed CD9, CD63, CD81, Alix and Tsg101; however, only exosomes derived from cerebrospinal fluid of SCI could promote proliferation of neuron via ERK signaling pathway, and decrease cell apoptosis. Exosomes contain cytosolic content, including proteins, mRNAs and non-cording RNAs, and play a role in important biological function. Our research showed exosomes derived from cerebrospinal fluid of SCI, they can influence neuron cell proliferation in vitro, we did not observe these characters in exosome derived from normal cerebrospinal fluid.
Introduction
Spinal cord injury (SCI) is a very severe disorder that can be caused by trauma ranging from traffic accidents to war wounds. SCI results in neuronal degeneration and demyelination due to oligodendrocyte apoptosis at the region of trauma, which causes severe functional impairment of motor and sensory pathways [Citation1]. Cerebrospinal fluid (CSF) is a clear, colorless body fluid found in the brain and spine. It is produced in the choroid plexuses of the ventricles of the brain. It acts as a cushion or buffer for the brain's cortex, providing basic mechanical and immunological protection to the brain inside the skull. The CSF occupies the subarachnoid space (between the arachnoid mater and the pia mater) and the ventricular system around and inside the brain and spinal cord. It constitutes the content of the ventricles, cisterns and sulci of the brain, as well as the central canal of the spinal cord. The physical condition and biological composition of CSF was changed when SCI had happened in body.
Exosomes are secreted nano-vesicles (30–100 nm diameter) formed through the inward budding of multi-vesicular bodies (MVBs). Exosomes are released from multiple cell types, contain protein and RNA species, and have been exploited as a novel reservoir for disease biomarker discovery. They can traffic and transfect proteins, mRNAs and miRNAs into target cells to information between cells and may cause pathology. In the nervous system, most research into exosomes has been in vitro, for example, primary cultures of rat cortical neurons [Citation2,Citation3], differentiated cortical neurons [Citation4] and glial cells [Citation5] have been demonstrated to release exosomes. Using transmission electron microscopy (TEM) and laser correlation spectroscopy, structures with a similar morphology to exosomes have been reported in human CSF [Citation6,Citation7]. In this research, exosomes were isolated from rat CSF between normal and SCI respectively, and then these exosomes were incubated with rat neurons to assess biological function in cell proliferation and apoptosis.
Materials and methods
SCI
Rats were anesthetized with an intraperitoneal injection of 0.15 mL/100 g of 2% pentobarbital (Sinopharm Chemical Reagent Co., Ltd, Shanghai, China). The dorsal area between the neck and hind limbs and extending 2 cm bilaterally from the spine was shaved and disinfected with 75% ethanol swabs. A midline incision exposed the spinal column at the level of T6–T10 and the paravertebral muscles were dissected bilaterally to visualize the transverse apophyses. Laminectomy was performed at T6–T10. Contusion injury was induced using a MASCIS Impactor (http://keck.rutgers.edu/MASCIS/mascis.html) by dropping a 10-g rod from a height of 25 mm. This procedure delivers a sudden impact of a desired force to the exposed spinal cord of a rat suspended by clamps on the vertebrae cranial and caudal to the laminectomy. Animals received manual bladder emptying twice daily and were inspected for weight loss, dehydration, and autophagia with appropriate veterinary care provided as needed. Cerebrospinal fluid was collected from 20 rats according to a previous report [Citation3].
Exosome collection
Exosomes isolated from CSF via ultracentrifugation were processed for visualization by electron microscope (EM), as described in Thery et al. [Citation8]. H-7100 EM (Hitachi, Tokyo, Japan) and Advantage-HSCCD camera (AMT, Woburn, MA) were used.
Western blotting
CD9, CD63, CD81, Alix and Tsg10, the markers of exosome, were detected by western blot analysis. Exosomes were lysed using M-PER Protein Extraction Reagent (Pierce, Washington, DC) supplemented with a protease inhibitor cocktail (DMSF). Protein concentrations of the extracts were measured using the BCA assay (Pierce, Washington, DC) and equalized with extraction reagent. Equal amounts of extracts were loaded and subjected to SDS-PAGE, followed by transfer onto nitrocellulose membranes. Antibodies and horseradish peroxidase-coupled secondary antibodies were purchased from Santa Cruz, USA. Membranes were probed using ultra-enhanced chemiluminescence western blotting detection reagents. GAPDH was used as an internal control.
Cell culture
Briefly, to isolate the cells, rat spinal cord tissue was placed in Hank’s-buffered saline solution at 4 °C without Ca2+ or Mg2+ and was washed extensively with equal volumes of Hank’s-buffered saline solution. Tissue pieces were minced into small pieces (1 mm3) and were enzyme digested at 37 °C for 20 min with 0.25% trypsin. Enzyme activity was neutralized with DMEM, containing 10% fetal bovine serum (FBS), and tissue was centrifuged at 1200 g for 10 min to obtain a high cell density in the pellet. Cultured cells were then incubated overnight at 37 °C in 5%CO2 in 10% FBS containing DMEM. The cell culture medium was replaced after 48 h and every fourth day thereafter [Citation9].
Immunofluorescent staining
Neurons were fixed with 4% PFA in PBS for 1 h, followed by three rinses with PBS. Neurons were then permeabilized with 0.01% Triton X-100 for 30 min. After rinsing three times with PBS, neurons were blocked with goat serum for 30 min, then incubated with primary antibodies in a humidified chamber at 4 °C overnight. After three five-min washes with PBS the following day, cells were incubated with FITC-labeled secondary antibodies at RT for 1 h. Neurons were given a final wash with PBS three times for 5-min each. Immunofluorescent data were recorded using a confocal optical system (Nikon TE2000, Tokyo, Japan).
Cell viability and bromodeoxyuridine (BrdU) analysis of neurons
To test viability, neurons were reseeded in six-well plates, treated with CSF exosomes from SCI model and normal, and analyzed with MTT (3-(4,5-dimethyl-2-thiazolyl)-2,5-diphenyl-2-H-tetrazolium bromide) [Citation10,Citation11]. Briefly, culture medium in each well was replaced with 500 μl of fresh medium containing 10 μl of 5 mg/ml MTT stock solution (Sigma-Aldrich, St. Louis, MO). Medium was removed after 5 h and replaced with 500 μl dimethyl sulfoxide (DMSO, Sigma-Aldrich, St. Louis, MO) per well and held for 10 min at 37 °C, after which samples were mixed and absorbance was read at 540 nm. BrdU analyses were used to measure cell proliferation (BrdU incorporation into DNA of proliferating cells). Neurons were treated with 10 μM BrdU (Sigma-Aldrich, St. Louis, MO) for 24 h and then fixed for immunofluorescent antibody assays.
Influence of ERK pathway in cell proliferation
Neurons were plated in six-well plates at a density of 1 × 104 cells per well, treated with CSF exosomes from SCI model and normal, and then ERK inhibitor were used to test role of ERK pathway in neurons proliferation. After 7 d, culture cell viability was assayed with MTT and BrdU. To confirm molecular mechanisms underlying cell proliferation, Western blot was used to measure phosphorylation and expression of ERK1/2.
Statistical analysis
Statistical analyses of data were performed with a one-way ANOVA followed by the Tukey–Kramer honestly significant difference (HSD) test for the three sets of results (p < .05 was considered statistically significant). Statistical analyses were assessed with JMP® Statistical Discovery Software (SAS Institute, Cary, NC).
Results
SCI in rats
The severity of the trauma at the level of the perilesional area, assessed as the presence of edema as well as alteration of the white matter, was evaluated at 24 h after injury. A significant damage to the spinal cord was observed in the spinal cord tissue of control rats subjected to SCI when compared with sham-operated rat (). The results of the “motor function score” evaluation are presented as mean value ± SD in . Trauma caused a significant change in motor function score as compared to the control group values (normal). Statistical analysis revealed difference rates between two groups (p > 0.05).
Figure 1. Spinal cord injury in rats. (A) Spinal cord contusion injury resulted in a loss of tissue structure within the central region of the spinal cord by 2 week post-injury, the pathological tissue was formed after injury and dropped out when tissue sliced. (B) Evaluation of motor function score. Trauma caused a significant change in motor function score as compared with the control group values (normal).
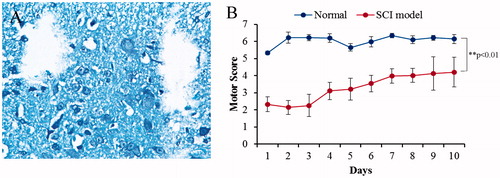
Characterization of CSF-derived exosomes
We characterized the CSF-derived exosomes by transmission electron microscopy (TEM) and Western blotting for proteins, CD9, CD63, CD81, Alix and Tsg10. The results showed that the majority of exosomes exhibited a cup- or round-shaped morphology under electron microscopy, with a size ranging from 50 to 80 nm (). Moreover, these exosomes expressed characteristic exosomal surface marker proteins: CD9, CD63, CD81, Alix and Tsg10 ().
Bio-characteristics of rat neurons
Rat neurons derived from the dissociation of spine were adherently cultured in medium. Following culture, the cells contracted and became round, triangular or cone shaped with multipolar processes. The processes continued to grow with many branches forming and cone-like terminal expansions. A number of cells demonstrated very long processes, which appeared similar to long neuronal axons. Immunofluorescence and flow cytometry assay detected β-III-tubulin and MBP expression ().
SCI-CSF derived exosome promotes proliferation of rat neurons
Neurons proliferation was assessed using MTT and Brdu assay after SCI or normal-CSF derived exosome treatment, and results demonstrated that neurons proliferation was increased. The absorbance and immunofluorescent data were measured using ultraviolet spectrometry and confocal microscopy from 1- to 8-day cultured cells in control, normal CSF exosome and SCI-CSF exosome treatment respectively. These results showed a time-dependent increase in neurons from SCI-CSF exosome treatment. However, the proliferation efficiency showed no significant difference in neurons following treatment in normal CSF exosome and control. Additionally, gene expression of the ERK1/2 showed a time-dependent increase following SCI-CSF exosome treatment ().
Figure 4. Exosome promotes proliferation of rat neurons. (A) Cell viability in 1- to 8-day-cultured rat neurons after exosome derived CSF treatment via MTT assay. (B) Cell proliferation in 1- to 8-day-cultured rat neurons after exosome derived CSF treatment via BrdU labeling indicating increased proliferation with SCI-CSF-exosome treatment. (C) Western blot of activation of ERK1/2. Increased expression level of ERK1/2 after SCI-CSF-exosome treatment, but no significant changes after normal CSF-exosome treatment compared with the control.
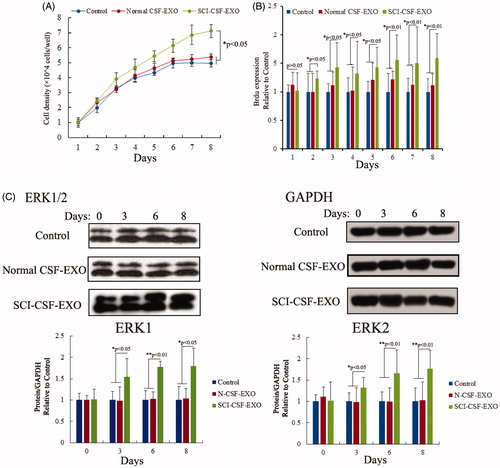
Role of ERK pathway in neurons proliferation
The ERK pathway (also known as the Ras-Raf-MEK-ERK pathway) is a chain of proteins in the cell that communicates a signal from a receptor on the surface of the cell to the DNA in the nucleus of the cell, including ERK1/2, MEK, JNK and P38. For testing function of ERK pathway in neurons proliferation, ERK pathway inhibitor (SCH772984) was selected to block ERK pathway number expression after SCI-CSF exosome treatment. The results demonstrated that neurons proliferation was stagnated after ERK pathway inhibitor and SCI-CSF exosome co-treatment using MTT and Brdu assay (), this result indicated ERK pathway played an important role in neurons proliferation.
Figure 5. Role of ERK pathway in neurons proliferation. (A) Cell viability in 1- to 8-day-cultured rat neurons after SCI-CSF-exosome, ERK pathway inhibitor treatment respectively via MTT assay. (B) Cell proliferation in 1- to 8-day-cultured rat neurons after SCI-CSF-exosome treatment, ERK pathway inhibitor treatment via BrdU labeling. The results indicated ERK pathway inhibitor was a blocker for rat neurons after SCI-CSF-exosome treatment. (C) Expression level of ERK pathway members after inhibitor treatment, the results indicating expression level of ERK pathway members, including ERK 1/2, JNK, MEK and P38, showed a time-lapse increase.
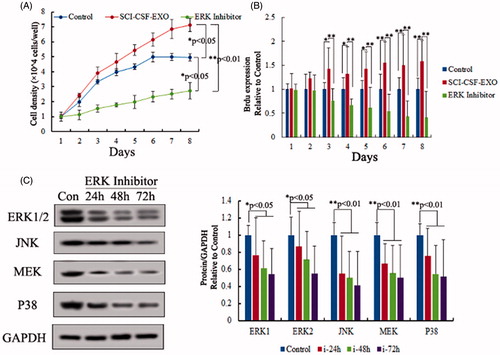
Discussion
SCI is a very severe disorder that can be caused by trauma ranging from traffic accidents to war wounds. SCI results in neuronal degeneration and demyelination due to oligodendrocyte apoptosis at the region of trauma, which causes severe functional impairment of motor and sensory pathways [Citation1]. Exosomes represent a novel reservoir for bio-information discovery because they contain protein, messenger RNA and non-cording RNA that has been demonstrated to change with the disease state of the affected organ [Citation12,Citation13]. To date, in the nervous system, most research into exosomes has been in vitro, for example, primary cultures of rat cortical neurons [Citation2], differentiated cortical neurons [Citation4] and glial cells [Citation5] have been demonstrated to release exosomes, but about function of exosome from injury nervous system in neurons underlying this process are unclear.
Release of exosomes from a variety of different neuronal cell lines has been described and there is evidence that exosomes may be involved in the pathogenesis of neurological disease [Citation14,Citation15]. Despite the potential importance of exosomes in the context of the central nervous system and neurological diseases the presence of exosomes in CSF has not been confirmed. In the present study we have demonstrated that SCI-CSF derived exosomes could promote neurons proliferation in vitro. Ependymal cells of the choroid plexus produce more than two-thirds of CSF. The choroid plexus is a venous plexus contained within the four ventricles of the brain, hollow structures inside the brain filled with CSF. The remainder of the CSF is produced by the surfaces of the ventricles and by the lining surrounding the subarachnoid space. Exosomal contents of CSF had changed when injury occurred in central nervous system, function of exosome contents after injury was unclear, all exosome contents were associated with cell type and tissue specificity.
Thakur et al. [Citation16] demonstrate that double-stranded DNA is present in exosomes from cancer cells and reflects the mutational status of the originated cells [Citation16]. Valadi et al. demonstrate that exosomes contain mRNA and miRNA [Citation17]. Exosome-carried RNcan shuttle between cells and thus is called “exosomal shuttle RNA” (esRNA). The protein composition of tumor cell-derived exosomes has been well characterized for a number of cancers by using different proteomic methods. The most common proteins, mRNA and miRNAs found in exosomes have been deposited in ExoCarta (www.exocarta.org). To date, 4563 proteins, 1639 mRNAs and 764 miRNAs have been identified in exosomes from different species and tissues by independent examinations. The exosomal contents vary between different physiological and pathological conditions and original cell types. Moreover, the composition of exosomes can be distinct from the originated cells due to the selective sorting of the cargo into exosomes. In this research, we indicated that exosomes derived from cerebrospinal fluid of SCI, they can promote neuron cell proliferation in vitro, we did not observe these characters in exosome derived from normal cerebrospinal fluid. But the molecular mechanisms of this finding were unclear. These molecular mechanisms still need to be further identified according to their functional characteristics, which will be accomplished in our future work.
In conclusion, it is indicated that SCI-CSF derived exosomes not only expressed CD9, CD63, CD81, Alix and Tsg101, but promoted proliferation of neuron via ERK signaling pathway, and decrease cell apoptosis. But the molecular mechanisms of this finding were unclear. These molecular mechanisms still need to be further identified according to their functional characteristics, which will be accomplished in our future work.
Disclosure statement
The authors declare that they have no competing interests.
References
- Angeli C, Ochsner J, Harkema S. Effects of chronic baclofen use on active movement in an individual with a spinal cord injury. Spinal Cord. 2012;50:925–927.
- Faure J, Lachenal G, Court M, et al. Exosomes are released by cultured cortical neurones. Mol Cell Neurosci. 2006;31:642–648.
- Gao Y, Bai C, Zheng D, et al. Combination of melatonin and Wnt-4 promotes neural cell differentiation in bovine amniotic epithelial cells and recovery from spinal cord injury. J Pineal Res. 2016;60:303–312.
- Lachenal G, Pernet-Gallay K, Chivet M, et al. Release of exosomes from differentiated neurons and its regulation by synaptic glutamatergic activity. Mol Cell Neurosci. 2011;46:409–418.
- Potolicchio I, Carven GJ, Xu X, et al. Proteomic analysis of microglia-derived exosomes: metabolic role of the aminopeptidase CD13 in neuropeptide catabolism. J Immunol. 2005;175:2237–2243.
- Harrington MG, Fonteh AN, Oborina E, et al. The morphology and biochemistry of nanostructures provide evidence for synthesis and signaling functions in human cerebrospinal fluid. Cerebrospinal Fluid Res. 2009;6:10.
- Filatov MV, Landa SB, Pantina RA, et al. Investigation of exosomes secreted by different normal and malignant cells in vitro and in vivo. Klin Lab Diagn. 2010;12:35–43.
- Thery C, Amigorena S, Raposo G, et al. Isolation and characterization of exosomes from cell culture supernatants and biological fluids. Curr Protoc Cell Biol. 2006;22. Chapter 3 (Unit 3.22).
- Xu D, Cao F, Sun S, et al. Inhibition of the Ras/Raf/ERK1/2 signaling pathway restores cultured spinal cord-injured neuronal migration, adhesion, and dendritic spine development. Neurochem Res. 2016;41:2086–2096.
- Kim JG, Yi JM, Park SJ, et al. Histone demethylase JMJD2B-mediated cell proliferation regulated by hypoxia and radiation in gastric cancer cell. Biochim Biophys Acta. 2012;1819:1200–1207.
- Bai C, Li X, Gao Y, et al. Melatonin improves reprogramming efficiency and proliferation of bovine-induced pluripotent stem cells. J Pineal Res. 2016;61:154–167.
- Zhou H, Pisitkun T, Aponte A, et al. Exosomal Fetuin-A identified by proteomics: a novel urinary biomarker for detecting acute kidney injury. Kidney Int. 2006;70:1847–1857.
- Zhou H, Cheruvanky A, Hu X, et al. Urinary exosomal transcription factors, a new class of biomarkers for renal disease. Kidney Int. 2008;74:613–621.
- Rajendran L, Honsho M, Zahn TR, et al. Alzheimer’s disease beta-amyloid peptides are released in association with exosomes. Proc Natl Acad Sci USA. 2006;103:11172–11177.
- Sharples RA, Vella LJ, Nisbet RM, et al. Inhibition of gamma-secretase causes increased secretion of amyloid precursor protein C-terminal fragments in association with exosomes. FASEB J. 2008;22:1469–1478.
- Thakur BK, Zhang H, Becker A, et al. Double-stranded DNA in exosomes: a novel biomarker in cancer detection. Cell Res. 2014;24:766–769.
- Zhang X, Yuan X, Shi H, et al. Exosomes in cancer: small particle, big player. J Hematol Oncol. 2015;8:83.