Abstract
At present, combined therapy has become an effective strategy for the treatment of cancer. Co-delivery of the chemotherapeutic drugs and siRNA can more effectively inhibit tumor growth by nano drug delivery systems (NDDSs). Here, we prepared and evaluated a multifunctional envelope-type nano device (MEND). This MEND was a kind of composite lipid-nanoparticles possessing both the properties of liposomes and nanoparticles. In this study, an acid-cleavable ketal containing poly (β-amino ester) (KPAE) was used to bind siBCL-2 and the KPAE/siBCL-2 complexes were further coated by epirubicin (EPI) containing lipid to form EPI/siBCL-2 dual loaded lipid-nanoparticles. The results showed that the average size of EPI/siBCL-2-MEND was about 120 nm, and the average zeta potential was about 41 mV. The encapsulation efficiency (EE) of EPI and siBCL-2 was 86.13% and 97.07%, respectively. EPI/siBCL-2 dual loaded lipid-nanoparticles showed enhanced inhibition efficiency than individual EPI-loaded liposomes on HepG2 cells by MTT assay. Moreover, western blot experiment indicated co-delivery of EPI/siBCL-2 can significantly down-regulate the expression of P-glycoprotein (P-gp), while free EPI and EPI-loaded liposomes up-regulated it. Therefore, the strategy of co-delivering EPI and siBCL-2 simultaneously by lipid-nanoparticles showed promising potential in reversing multidrug resistance of tumor cells.
Introduction
In the treatment of cancer, the efficacy of chemotherapy is often depressed due to the drug resistance of tumor cells, which is usually caused by abnormal gene expression. The combination of siRNA and chemotherapy drugs may be a newly promising strategy for cancer treatment, which is co-delivering siRNA and chemotherapy drugs to specific tissues or cells by carriers to exert effect in treatment [Citation1–3].
But naked siRNA is easily degraded by RNases in vivo, and difficult to pass through cell membranes due to its high molecular weight and electronegativity [Citation4–6], which cause that one of the key challenges for combined therapy is the development of suitable, efficient and low toxic vectors. Non-viral vectors show low toxicity, low carcinogenic rate, easy preparation convenient batch production and so on, but the transfection efficiency is significantly lower than that of viral ones [Citation7,Citation8]. Therefore, how to further improve the transfection efficiency of non-viral vector is a major concern. In 2004, Harashima developed a multifunctional envelope type nano carrier (MEND) [Citation9,Citation10], which has advantages of both nanoparticles and liposomes. MEND is similar to envelope type shell nanoparticles, which is based on compression DNA, ODN or siRNA protein as the core, wrapped with liposome targeting genes and functional ligands. MEND has low immunogenicity, good biocompatibility and high transfection efficiency.
In the acid and enzyme-containing environment of endosome, the siRNA was easily degraded. Due to the proton sponge effect, the cationic polymer can achieve the function of the endosomal escape [Citation11]. Through electrostatic interaction, cationic polymers are used to condense the negatively charged siRNA, which can enhance the proton sponge effect to protect siRNA [Citation12–15]. KPAE as the pH-sensitive material, is a cationic polymer which can efficiently condense siBCL-2, protect it effectively and ensure the release of intracellular siBCL-2 intelligently. In addition, due to the degradation products of KPAE in acidic conditions are neutral small molecules, such as acetone and H2O, which can avoid the occurrence of inflammation, so it can be indicated that KPAE shows good biocompatibility [Citation16]. SiBCL-2 is usually used to inhibit the expression of BCL-2 gene to promote the apoptosis of tumor cells [Citation17,Citation18]. Studies have shown that PEKR pathway can regulate the expression of BCL-2 and P-gp in insulin resistant HepG2 cells [Citation19], which can be inferred that there is a link between the expression of BCL-2 and P-gp. We assumed that the expression of P-gp can be decreased by down-regulating the expression of BCL-2.
The modification of MEND can improve the stability and drugs delivery efficiency. According to the method of Harashima, we prepared pH-sensitivity and long-circulation MEND PEG-DSPE. Polyethylene glycol (PEG) can form a hydrophilic layer on the surface of liposomes, then the size of MEND is reduced to less than 200 nm, which can extend the blood circulation time of MEND through improving the hydrophilicity of MEND particles [Citation20–23].
Experiments
Materials and instruments
DOTAP. Shanghai A.V.T. Co., Ltd; DSPE-PEG2000, Shanghai A.V.T. Co., Ltd; NC-siRNA, FAM-siRNA and BCL-2 siRNA, Shanghai GenePharma Co., Ltd; Nuclear Magnetic Resonance Spectrometer (JNMECP600), JEOL; Zetasizer Nano instrument (Zetasizer Nano-ZS90), Malvern Instruments Ltd.; Transmission electronic microscope (JEM2010), JEOL; Automatic diagital gel imaging analysis system (JS-380B), Peiqing Technology (Shanghai) Co., Ltd; Multifunctional enzyme standard instrument workstation (FlexStation-3), Molecular Devices (Beijing) Co., Ltd; Other chemicals were all of analytical or chromatographic grade.
Synthesis and characterization of ketal containing poly (amino ester)
We synthesized KDA and KPAE according to the methods of Kyeonghye Guk [Citation24]. In details, KDA was synthesized through transketalization of 2,2-dimethoxypropane with 2-hydroxyethylacrylate in the presence of p-toluenesulfonic acid (p-TSA) catalyst in dry benzene. 2-Hydroxyethylacrylate (69.6 mmol), 2,2-dimethoxypropane (34.8 mmol), p-toluenesulfonic acid (0.01 g) and 1.01 g of hydroquinone were stirred in dry benzene (9 ml) and distilled to remove the methanol–benzene azeotrope (90 °C), after 6 h, the remaining benzene was removed in vacuum. Then, KDA (3.7 mmol) and diethylenetriamine (3.78 mmol) were separately dissolved in 5 mL of dry dimethyl sulfoxide (DMSO). The reaction system was stirred at 40 °C for 24 h. The product solution was placed into a dialysis bag with a MWCO of 1000, dialyzed against ultrapure water to remove the unreacted monomers. The water was refreshed at 1, 3, 12 and 24 h after the start of dialysis, then aqueous solution in the dialysis bag was lyophilized to obtain the KPAE. KPAE could bind siRNA in water by electrostatic interaction. The chemical structure of KPAE synthesized was determined by 1H NMR spectrometer.
Preparation of MEND
We used the rotary-film evaporation method to prepare liposome membrane. Appropriate weights of PEG-DSPE and DOTAP were respectively dissolved in chloroform to obtain solutions with the concentration of 2.0 mg/ml. EPI (0.7 mg), PEG-DSPE (1 mg) and DOTAP (6 mg) were added into a round-bottom flask and dissolved by 3.5 ml of chloroform. The solution was rotated and evaporated for 30 min to remove the chloroform completely. KPAE/siRNA complexes were obtained by adding KPAE into siRNA (40 μg) dissolved in 5 ml diethylpyrocarbonate (DEPC) with weight ratio of 10:1. The phospholipids film was hydrated by the aqueous solution. The resulting hydrate was treated with a probe-type ultrasonicator, obtaining EPI/siRNA-MEND.
Determination of the encapsulation efficiency (EE) of EPI
Chromatographic conditions
Chromatographic column, XSELECT CSH C18 (4.6 × 250 mm, 5 μm); Mobil phase, Methanol–acetonitrile–water (50:40:10), Flow rate, 1.0 ml/min; UV detection wavelength, 233 nm; Column temperature, 25 °C; and Injection volume, 20 μl.
Specificity
EPI (0.4 mg) was dissolved and diluted with methanol to 10 ml to obtain the EPI solution (40 μg/ml). According to Experiments 2.3, preparing MEND and EPI-MEND. MEND (1 ml) was dissolved and diluted with methanol to 10 ml to obtain the MEND solution; According to the preparation method of the MEND solution, prepared EPI-MEND solution.
Standard curve
EPI (0.005 g) was dissolved and diluted with methanol to 50 ml to obtain the EPI stock solution (100 μg/ml). Appropriate volumes of stock solution were respectively dissolved and diluted with methanol to obtain standard solutions with concentration of 1.0, 2.5, 5.0, 10.0, 25.0 and 50.0 μg/ml. According to Experiments 2.4.1, the standard solutions were injected to HPLC for analysis.
Precision test
The stock solutions were respectively transferred and diluted with methanol to obtain solutions with concentration of 1.0, 5.0 and 50.0 μg/ml. Five times were analyzed in 1 day to calculate intra-day precision.
Recovery test
50, 250 and 2500 μl of stock solutions were transferred into 0.5 ml of EPI-MEND solutions, respectively. The mixed solutions were demulsified and diluted with methanol to obtain solutions with concentration of 1.0, 5.0 and 50.0 μg/ml, determined by HPLC.
Separation efficiency of filter membrane
According to Experiments 2.3. preparing EPI-MEND and dividing into two equal parts. A formula dosage of EPI was added to one, and the other one was not. Both of two parts were filtrated by 0.22 μm filter membrane. 0.1 ml of the two filtrates were demulsified and diluted with methanol to 10 ml, determined by HPLC.
Solubility of EPI
Formula dosage of EPI, DOTAP, DSPE-PEG was added into PBS (pH 7.4). Treated with a probe-type ultrasonicator, 250 W for 5 min. The mixture was filtrated by 0.22 μm filter membrane. 1 ml of filtrate was diluted with methonal to 5 ml. Repeating determination for 3 times to obtain the solubility, marked as C1.
EE of EPI
EPI-MEND solution was filtrated by 0.22 μm filter membrane. 0.1 ml of filtrate was demulsified and diluted with methanol to 5 ml, determined by HPLC. The obtained concentration was marked as C.
In addition, 0.1 ml of EPI-MEND solution was demulsified and diluted with methanol to 10 ml. Analysis obtained concentration was marked as C0. The calculation formula of EE is as follows. EE (%) = (C − C1)/C0 × 100%.
Determination of the EE of siRNA
Precision test
FAM-siRNA (20 μg) was dissolved in DEPC to 400 μl to obtained FAM-siRNA stock solution (50 ng/ml). Appropriate volumes of stock solution were respectively dissolved and diluted with DEPC obtain standard solutions with concentration of 0.05, 0.1, 0.25, 0.5, 1.0, 2.5 and 5.0 ng/ml. The standard solutions were determined with multifunctional enzyme standard instrument workstation for analysis.
EE of siRNA
FAM-siRNA-MEND was prepared, and 400 μl sample was treated with ultrafiltration centrifugation for 10 min in centrifuge tubes with a MWCO of 30,000. The concentration of siRNA in the supernatant was determined with multifunctional enzyme standard instrument workstation, marked as C. The EE of FAM-siRNA was calculated using the following formula: EE (%) = (M − CV)/M × 100%. V is the volume of the supernatant containing after centrifuge, M is the content of FAM-siRNA packaged within MEND.
Cytotoxicity assay
The HepG2 cells were cultured in normal DMEM media with 10% FBS and 100 units/ml penicillin, and 100 mg/ml streptomycin in 5% CO2 and 95% humidity atmosphere in humidifier. The cell viability/cytotoxic potential of individual formulation was performed by MTT assay. Briefly, cells were seeded into 96-well plate at a seeding density of 4 × 104 cells per well and incubated for 24 h. Following day, medium was removed, and cells were incubated with various concentrations of free EPI, EPI-MEND and EPI/siBCL-2-MEND, respectively. The cells were incubated for another 24 h, MTT solution (5 mg/ml in serum free media) was added into each well and incubated for 4 h. The purple blue formazan crystals were extracted by the addition of DMSO, and absorbance was measured at 490 nm using multifunctional enzyme standard instrument workstation.
Cellular uptake by flow cytometer analysis
Flow cytometry was used to detect the cell uptake rate of each experimental group of drugs in the 4 h of transfection in HepG2 cells. The establishment of free EPI group, naked FAM-siRNA group and EPI/FAM-siRNA-MEND group was separately transfected. FAM-siRNA and EPI were detected with FL1 channel (excitation wavelength is 488 nm, and emission wavelength is 530 ± 15 nm) and FL2 channel (excitation wavelength is 488 nm, and emission wavelength is 585 ± 20 nm), respectively.
Endocytosis of MEND
To confirm the cellular endocytosis of MEND, double staining was performed. The cells were separately treated with FAM-siRNA-MEND (green fluorescence) and EPI-MEND (red fluorescence) for 4 h, then the cell nucleus was stained with Hoechst 33342 which emits blue fluorescence, and the lysosome was stained with LysoTracker-Blue DND-22 which emits blue fluorescence.
Western blot analysis
Transfected HepG2 cells were washed in ice-cold phosphate buffer solution for three times and lysed in RIPA buffer with 1 × halt protease and phosphatase inhibitor cocktails. Samples were quantified using a BCA protein assay kit. Protein samples were separated using PAGE (10%) and transferred to nitrocellulose membranes. After blocked with 1 × TBST containing 5% milk, the blotting membrane was incubated overnight at 4 °C with rabbit anti-P-gp (1:1000) and rabbit anti-β-actin (1:2000). The membrane was incubated with an anti-rabbit and anti-mouse lgG-HRP secondary antibody (1:2000) for an hour at room temperature and visualized using the Automatic digital gel imaging analysis system.
In vivo distribution test
The BALB/c nude mice were randomly divided into six groups: control group (saline injection group), MEND group, EPI-MEND group, Cy5-siRNA-MEND group, free EPI group and EPI/Cy5-siRNA-MEND group, then injected through the tail vein. Two hours later, the mice's heart, liver, spleen and kidney were removed and placed in the living animal imaging system to observe the in vivo distribution.
Results and discussions
Synthesis and characterization of KPAE
KPAE was synthesized with KDA and diethylenetriamine via Michael addition polymerization in dry dimethyl sulfoxide. After 24 h of polymerization at 40 °C, KPAE was obtained as reddish-brown viscous liquid. The chemical structure of KPAE was identified by 1H NMR. As shown in , 1H NMR in CDCl3 on a 400 MHz spectrometer: δ = 1.4 (m, OCCH3), 2.3–2.9 (m, NHCH2CH2), 3.1–3.5 (m, CH2CH2CO), 3.5–3.8 (m, OCH2CH2). The weight of KPAE copolymer was measured to be 7000–10000 Da by using gel permeation chromatography (GPC). And in acid environment, ketal groups in KPAE degraded into small molecules, and weight average molecular weight was decreased (). While in acid environment like endosomes or lysosomes (pH 5.5), ketal groups in KPAE degraded and enhanced the endosomal/lysosomal escape and release of siRNA but stable in the neutral pH of cytosol [Citation25].
Preparation of MEND
MEND solution was dropped on a 300 mesh copper grid and kept for 3–5 min, and the excess fluid was removed by absorbent paper. Negative staining was performed using 5% aqueous phosphotungstic acid for 30 min before observation with transmission electron microscope (TEM), as shown in . The liposome present spherical structure and fingerprint structure which can effectively encapsulate lipophilic drugs [Citation26,Citation27]. The particle sizes and zeta potentials of EPI/siRNA-MEND were determined with a zetasizer nano instrument. The particle average size is 120 nm (. The appropriate size (<200 nm) can preferentially accumulated in the tumor region via EPR effect [Citation28–30]. The zeta potential is 41 mV (), which can easily uptake by cells with high positive charge. Agarose gel electrophoresis was employed to evaluated siRNA enwrapping capacity. As shown in , channel 1 (naked siRNA) had an obvious strip, while siRNA migration was retarded in channel 2 (EPI/siRNA-MEND), which indicated that the MEND can effectively enwrap siRNA.
Determination of the EE of EPI
The result of specificity was shown in , and the drug peak and impurity peaks are well detached. Excipients and reagents are no interference for drug determination. The standard curve was built by plotting the peak area (A) versus the concentration of EPI (X). A = 39.231X − 10.695 (R2 = 0.9998), which indicated that there was a good linear relation between the peak area and the concentration of EPI within the range of 1.0–50.0 μg/ml. RSD of intra-day precision was <1%, which showed that the developed method is precise enough for the contend determination of EPI. The results of recovery experiment (RSD <1%) indicated that the method is exact and feasible for the measurement of EPI. The average recovery of free EPI is just 4.24%, which demonstrated that the filter membrane can separate free EPI from MEND effectively. Through determination, the result showed that the solubility of EPI (C1) in PBS (pH 7.4) was 8.66 μg ml−1 (n = 3), which accounts for about 6.19% of EPI concentration in MEND. According to the formula, we calculated that the EE of EPI was 86.13%.
Determination of the EE of siRNA
The standard curve was built by plotting the peak area (Y) versus the concentrations of FAM-siRNA (C). Y = 976.01 C + 121.68 (R2=0.9987). The result indicated that there was a good linear relation between the peak area and the concentration of FAM-siRNA within the range of 0.05–5.0 ng/ml. Finally, according to the formula, we determined that the EE of siRNA was 97.07%. It was proved that the liposome had a higher EE to siRNA.
Cytotoxicity assay
One of the key points of the polymer as gene carrier is cytotoxicity [Citation15,Citation31]. By measuring the survival rate of cells in each experimental group, it showed that blank MEND vector system in certain dose range of HepG2 cells without significant toxicity, and the survival rate proved that EPI/siBCL-2-MEND combined preparations compared to separate after loading EPI or siBCL-2 of HepG2 cells have significantly stronger inhibitory effect and statistically significant. As shown in , free EPI, EPI-MEND, EPI/siBCL-2-MEND exhibited a dose-dependent cytotoxicity on the cell line tested. The results in the substantial reduction in the IC50 value of EPI when used on synergistic combinations. The IC50 value of EPI-MEND was 4.322 μg/ml, whereas EPI/siBCL-2-MEND exhibited 2.541 μg/ml. The results showed that siBCL-2 played a role in promoting apoptosis. Therefore, it can be concluded that the combination treatment had higher curative effect than that of the drug alone.
Cellular uptake by flow cytometry
Flow cytometer analysis was used to further prove the cellular uptake of EPI/FAM-siRNA-MEND in HepG2 cells. As we can see, after 4 h of incubation, the fluorescence intensity can be observed (), the uptake rate of EPI-MEND group was 98%. The transfection efficiency of FAM-siRNA-MEND vector system was forty times higher than that of naked siRNA group. It is showed that the carrier system can better carry siRNA into cells with the higher transfection efficiency. At the same time, the system has little effect on the uptake rate of EPI that will improve the curative effect of the treatment of cancer. Intravenous administration of free EPI or PEGylated EPI-MEND, free of EPI in vivo is rapidly cleared [Citation32], which results EPI concentration in blood decreased rapidly and EPI concentrations in tumor tissue far lower than PEGylated EPI-MEND. Studies have indicated that the free EPI clearance rate in the body is 450 times as that of PEGylated MEND [Citation33].
Endocytosis of MEND
Fluorescence microscope was used to observe the endocytosis of MEND by cells. In , the green component in the cytoplasm demonstrated that that polyplexes could easily escape from endosome/lysosome to cytoplasm, which confirmed the acid sensitivity of KPAE. In , images showed that EPI could be distributed in the nucleus. Therefore, we concluded that MEND efficiently co-delivered EPI and siRNA into cells, rapidly passed the membrane of endosome/lysosome and released the EPI and siRNA into nucleus and cytoplasm, respectively. These results provided evidence that EPI and siRNA could be co-delivered by MEND as therapeutic drug.
Western blot analysis
We evaluate the biological role of MEND in HepG2 cells. As shown in . (Top panel) compared with untreated cells, free EPI stimulated the expression of P-gp, which makes intracellular drug pump out of the cells, making the cells produce drug resistance. Then, we conducted the next verification test. As shown in . (Bottom panel), EPI-MEND could not effectively avoid the over expression of P-gp, while siBCL-MEND and EPI/siBCL-2-MEND can significantly down-regulate the expression of P-gp. It may be that there is a certain link between BCL-2 and P-gp [Citation19], making the expression of P-gp decreased with the down-regulation of BCL-2. This result suggested that EPI/siBCL-2-MEND can be used to inhibit the multi-drug resistance of tumor cells.
In vivo distribution test
Living animal imaging system to observe the in vivo distribution of MEND. According to ), Cy5-siRNA-MEND was distributed in the liver, while EPI/Cy5-siRNA-MEND was mainly distributed in the liver but also a small part in the kidney. And the indensity of EPI/Cy5-siRNA-MEND was stronger than that of Cy5-siRNA-MEND. We concluded that it may be due to siRNA neutralized some positive charges, leading to a decrease in the zeta potential and a longer circulation in vivo. At the same time, as shown in , the fluorescence intensity of EPI/Cy5-siRNA-MEND was the strongest, while the fluorescence intensity of free EPI was weaker than that of EPI-MEND. The results may be due to the clearance rate of free EPI in vivo was faster than that of liposome encapsulated drug. In , the specific values of fluorescence intensity could also prove the above views.
Figure 8. Picture of in vivo distribution of MEND. (A) Distribution of Cy5-siRNA-MEND and EPI/Cy5-siRNA-MEND (excitation wavelength was 650 nm). (B) Distribution of EPI/Cy5-siRNA-MEND, free EPI and EPI-MEND (excitation wavelength was 480 nm). (C) Comparison of Cy5-siRNA fluorescence in the liver. (D) Comparison of EPI fluorescence in the liver.
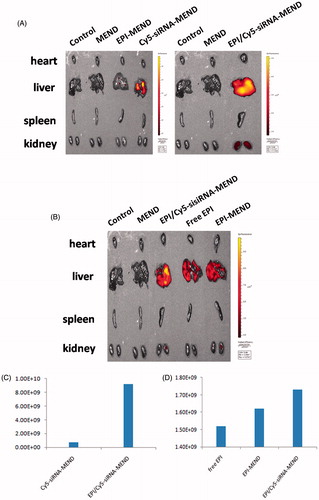
Conclusions
This study investigated the potential of co-delivery of EPI and siBCL-2 in a MEND constructed by pH-sensitive polymer and PEGylated liposome. Briefly, we synthesized KPAE, which could bind siRNA in water by electrostatic interaction, while in acid environment like endosomes or lysosomes, ketal groups in KPAE degraded and enhanced the endosomal/lysosomal escape and release of siRNA, which can achieve efficient transfection of siRNA to tumor cells by avoiding the rapid enzymatic degradation and poor cell membrane penetration. The HPLC method was used to determinate the EE of EPI, and the ultrafiltration centrifugation method was used to determinate that of FAM-siRNA. Results indicated the methods are accurate and feasible in determining content of EPI and FAM-siRNA, respectively. By flow cytometry to detect the cell transfection rate, results show that the endocytosis rate of EPI/FAM-siRNA-MEND vector is about forty times more than the single FAM-siRNA, proved that co-loading MEND can be more effectively to carry the siRNA into cells. Although free EPI with easy access to tumor cells, it is quickly removed in vivo.
According to the use of siBCL-2, it can be seen that EPI/siBCL-2-MEND can down-regulate the expression of P-gp, which can inhibit the multi-drug resistance of tumor cells. The EPI/siBCL-2-MEND exhibited sustained release kinetics and effectively inhibited the cell proliferation, and the further research is ongoing. From the above, we considered this pH-responsive lipid-nanoparticles for co-delivery of siBCL-2, and EPI was a promising potential in reversing multidrug resistance of tumor cells.
Acknowledgements
This work was supported by Shandong science and technology development projects in 2014 (2014GSF118168) and National Natural Science Fund Project in 2016 (81673360).
Disclosure statement
The authors report no conflicts of interest. The authors alone are responsible for the content and writing of this article.
Additional information
Funding
References
- Qu M, Zeng R, Long J, et al. Liposome-based co-delivery of siRNA and docetaxel for the synergistic treatment of lung cancer. Int J Pharm. 2014;474:112–122.
- Sun TM, Du JZ, Yao YD, et al. Simultaneous delivery of siRNA and paclitaxel via a two- in-one micelleplex promotes synergistic tumor suppression. ACS Nano. 2011;5:1483–1494.
- Lee SJ, Yook S, Yhee JY, et al. Co-delivery of VEGF and Bcl-2 dual-targeted siRNA polymer using a single nanoparticle for synergistic anti-cancer effects in vivo. J Control Release. 2015;220:631–641.
- Kaps L, Nuhn L, Aslam M, et al. In vivo gene-silencing in fibrotic liver by siRNA-loaded cationic nanohydrogel particles. Adv Healthc Mater. 2015;4:2809–2815.
- Devi GR. SiRNA-based approaches in cancer therapy. Cancer Gene Ther. 2006;13:819–829.
- Lee JB, Hong J, Bonner DK, et al. Self-assembled RNA interference microsponges for efficient siRNA delivery. Nat Mater. 2012;11:316–322.
- Hart SL. Multifunctional nanocomplexes for gene transfer and gene therapy. Cell Biol Toxicol. 2010;26:69–81.
- Mastrobattista E, van der Aa MA, Hennink WE, et al. Artifical viruses:a nanotechnological approach to gene delivery. Nat Rev. 2006;5:115–121.
- Kogure K, Akita H, Harashima H. Multifunctional envelope-type nano device for non-viral gene delivery: concept and application of programmed packaging. J Control Release. 2007;122:246–251.
- Kogure K, Akita H, Harashima H. Multifunctional envelope-type nano device (MEND) as a non-viral gene delivery system. Adv Drug Deliv Rev. 2008;60:559–571.
- Hu YH, Atukorale PU, Lu JJ, et al. Cytosolic delivery mediated via electrostatic surface binding of protein, virus, or sirna cargos to pH-responsive core-shell gel particles. Biomacromolecules. 2009;10:756–765.
- Zhou JH, Wu YD, Wang CR, et al. pH-sensitive nanomicelles for high-efficiency siRNA delivery in vitro and in vivo: an insight into the design of polycations with robust cytosolic release. Nano Lett. 2016;16:6916–6923.
- Cheng Q, Du LL, Meng LW, et al. The promising nanocarrier for doxorubicin and siRNA co-delivery by PDMAEMA-based amphiphilic nanomicelles. ACS Appl Mater Interfaces. 2016;8:4347–4356.
- Boyer C, Priyanto P, Davis TP, et al. Anti-fouling magnetic nanoparticles for siRNA delivery. J Mater Chem. 2010;20:255–265.
- Xu FJ, Chai MY, Li WB, et al. Well-defined poly(2-hydroxyl-3-(2-hydroxyethylamino)propyl methacrylate) vectors with low toxicity and high gene transfection efficiency. Biomacromolecules. 2010;11:1437–1442.
- Jere D, Xu CX, Arote R, et al. Poly(beta-amino ester) as a carrier for si/shRNA delivery in lung cancer cells. Biomaterials. 2008;29:2535–2547.
- Reddy TL, Garikapati KR, Reddy SG, et al. Simultaneous delivery of paclitaxel and Bcl-2 siRNA via pH-sensitive liposomal nanocarrier for the synergistic treatment of melanoma. Sci Rep. 2016;6:35223.
- Wu D, Yang JB, Xing Z, et al. Phenylboronic acid-functionalized polyamidoamine-mediated Bcl-2 siRNA delivery for inhibiting the cell proliferation. Colloids Surf B Biointerfaces. 2016;146:318–325.
- Liu XY, Li LJ, Li J, et al. Insulin resistance contributes to multidrug resistance in HepG2 cells via activation of the PERK signaling pathway and upregulation of Bcl-2 and P-gp. Oncol Rep. 2016;35:3018–3024.
- Kumar V, Ahmed D, Anwar F, et al. Enhanced glycemic control, pancreas protective, antioxidant and hepatoprotective effects by umbelliferon-α-D-glucopyranosyl-(2(I) → 1(II))-α-D-glucopyranoside in streptozotocin induced diabetic rats. Springerplus. 2013;2:639.
- Hatakeyama H, Akita H, Harashima H. Development of a novel systemic gene delivery system for cancer therapy with a tumor-specific cleavable PEG-lipid. Gene Ther. 2007;14:68–77.
- He ZL, Huang JW, Xu YY, et al. Co-delivery of cisplatin and paclitaxel by folic acid conjugated amphiphilic PEG-PLGA copolymer nanoparticles for the treatment of non-small lung cancer. Oncotarget. 2015;6:42150–42168.
- Jin GM, Jin MJ, Yin XZ, et al. A comparative study on the effect of docetaxel-albumin nanoparticles and docetaxel-loaded PEG-albumin nanoparticles against non-small cell lung cancer. Int J Oncol. 2015;47:1945–1953.
- Guk K, Lim H, Kim B, et al. Acid-cleavable ketal containing poly(β-amino ester) for enhanced siRNA delivery. Int J Pharm. 2013;453:541–550.
- Sankaranarayanan J, Mahmoud EA, Kim G, et al. Multiresponse strategies to modulate burst degradation and release from nanoparticles. ACS Nano. 2010;4:5930–5936.
- Shah SM, Goel PN, Jain AS, et al. Liposomes for targeting hepatocellular carcinoma: Use of conjugated arabinogalactan as targeting ligand. Int J Pharm. 2014;477:128–139.
- Ashley JD, Stefanick JF, Schroeder VA, et al. Liposomal carfilzomib nanoparticles effectively target multiple myeloma cells and demonstrate enhanced efficacy in vivo. J Control Release. 2014;196:113–121.
- Park J, Reddy Kadasala N, Abouelmagd SA, et al. Polymer-iron oxide composite nanoparticles for EPR-independent drug delivery. Biomaterials. 2016;101:285–295.
- Theek B, Baues M, Ojha T, et al. Sonoporation enhances liposome accumulation and penetration in tumors with low EPR. J Control Release. 2016;231:77–85.
- Attia ABE, Oh P, Yang C, et al. Insights into EPR effect versus lectin-mediated targeted delivery: biodegradable polycarbonate micellar nanoparticles with and without galactose surface decoration. Small. 2014;10:4281–4286.
- Lee BS, Yip AT, Thach AV, et al. The targeted delivery of doxorubicin with transferrin-conjugated block copolypeptide vesicles. Int J Pharm. 2015;496:903–911.
- Gabizon A, Catane R, Uziely B, et al. Prolonged circulation time and enhanced accumulation in malignant exudates of doxorubicin encapsulated in polyethyleneglycol coated liposomes. Cancer Res. 1994;54:987–992.
- Arranja A, Schroder AP, Schmutz M, et al. Cytotoxicity and internalization of pluronic micelles stabilized by core cross-linking. J Control Release. 2014;196:87–95.