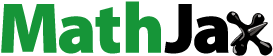
Abstract
The present study was aimed to develop and evaluate a microemulsion-based dermal drug delivery of an antiviral agent, acyclovir. A water-in-oil microemulsion was prepared using isopropyl myristate, Tween 20, Span 20, water and dimethylsulphoxide. It was characterized for drug content, stability, globule size, pH, viscosity and ex vivo permeation through mice skin. In vivo antiviral efficacy of optimized formulation was assessed in female Balb/c mice against herpes simplex virus-I (HSV-I)-induced infection. It was observed that optimized formulation when applied 24-h post-infection could completely inhibit the development of cutaneous herpetic lesions vis-à-vis marketed cream.
Keywords:
Introduction
Herpes simplex virus 1 and 2 (HSV-1 and HSV-2) are two members of the herpes virus family, Herpesviridae, that infect humans [Citation1]. HSV infection primarily affects the dermal or basal epidermal layer of the skin, mucous membranes and occasionally oesophagus and brain. There are numerous antiviral agents available for the alleviation of HSV infection, but acyclovir (ACV) is the most commonly prescribed treatment [Citation2,Citation3]. ACV, a synthetic analogue of 2′-deoxiguanosine, active against HSV-1, HSV-2, varicella zoster virus and to a lesser extent against Epstein–Barr virus and cytomegalovirus. Although both oral and topical ACV formulations are used clinically to treat HSV infections, these available therapies have a number of limitations [Citation4]. The oral absorption is dose dependent and highly variable with a bioavailability ranging from 10% to 30%. The mean plasma half-life (t1/2) of ACV is 3.25 h. Hence, repeated administration of high dose (200 mg; five times daily for 10 days) is required for the effective management of HSV infections [Citation5]. Furthermore, oral ACV has been associated with nausea, diarrhoea, rash or headache. Moreover, commercially available topical products of ACV have inadequate penetration of ACV through the stratum corneum and this leads to lack of availability of drug at the basal epidermis target site, therefore, it needs high frequency of application [Citation6–8] and simultaneously also associated with stinging and burning sensation. To overcome these problems, an attempt has been made to develop a microemulsion-based dermal formulation for effective delivery of ACV. Microemulsion systems, amongst various novel systems, offer the advantage of enhanced drug solubility, good thermodynamic stability, ease of production, enhancing effects on skin penetration ability over conventional formulations [Citation9]. In order to overcome the low permeability of drugs through skin, another popular strategy is the use of penetration enhancers [Citation10]. These agents partition into, and interact with, the stratum corneum constituents to induce a temporary, reversible increase in skin permeability [Citation10]. Terpenes are a class of very safe and effective penetration enhancers [Citation11], obtained from natural sources, the FDA classifies them as generally regarded as safe (GRAS) [Citation12]. Menthol is a naturally occurring cyclic terpene alcohol of plant origin, widely used as penetration enhancer [Citation13] in topical and transdermal delivery of variety of drugs including imipramine hydrochloride [Citation14], hydrocortisone, triamcinolone [Citation15], etodolac [Citation16], meloxicam [Citation17], valsartan [Citation18] and zidovudine [Citation19].
The aim of present study was to develop and evaluate water-in-oil (w/o) microemulsion-based topical formulations of ACV containing menthol and combination of menthol and propylene glycol as skin penetration enhancers. Improved penetration may help in attaining the sufficient therapeutic levels of drug in the skin to cure cutaneous herpetic infections. The response to topical acyclovir therapy was evaluated in a mouse model of zosteriform cutaneous HSV infection using HSV-1.
Materials and methods
Materials
HSV-1 virus was kindly supplied by National Institute of Virology, Pune. ACV was obtained as gift sample from Arochem Industries, Mumbai, India. Isopropyl myristate (IPM) was purchased from Loba Chemie Pvt. Ltd., Mumbai, India. Menthol was gifted by Seema International, Delhi, India. Propylene Glycol (PG) was purchased from Sisco Research Laboratories, Mumbai, India. Acyclovir Cream (Acivir®) manufactured by Cipla, Goa, India was used as control in the studies. All other reagents/chemicals used were of A.R. grade.
Animals
Female Balb/C mice (5–7 weeks old) weighing 15–20 g were used in this study. Animals were obtained from Central Animal House, Panjab University, Chandigarh, India. These were housed in polypropylene cages and employed for performing ex vivo permeation, histopathology and in vivo antiviral studies. All the animals were kept at ambient temperature with a 12–h night/day cycle and supplied with a standard pellet diet and water ad libitum. The protocols for animal use and care were approved by the Institutional Animal Ethics Committee (IAEC), Panjab University, Chandigarh, India.
Methods
Solubility studies
In order to select appropriate solvents in which ACV has sufficient solubility, the solubility studies were carried out in water, water: DMSO (1:3), phosphate buffer pH 6.4, water: PG (1:1), water: Polyethylene Glycol 400 (PEG 400) (1:1), water: PEG 600 (1:1), PG using the shake flask method [Citation20,Citation21]. An excess of drug was added to a fixed volume of each solvent (10 mL) in different flasks kept in a thermostatic water bath shaker for 24 h [Citation21]. The flasks were removed after 24 h, contents were filtered through 0.45-μ filter and filtrates were analysed spectrophotometrically at λmax 254 nm after appropriate dilution.
Drug partitioning study
Partitioning of ACV was determined in 1-octanol/water and IPM/water system. The two phases were mutually saturated in 1:1 ratio by shaking overnight on water bath shaker at 37 ± 0.5 °C for 24 h. The phases were then separated by centrifugation at 2000 rpm for 10 min. An aliquot (10 mL) of 1-octanol/IPM saturated water containing 0.5 mg/mL of ACV was mixed with an equal volume of water-saturated 1-octanol/IPM. Two phases were then allowed to equilibrate at 37 ± 0.5 °C for 24 h in a water bath shaker and the two phases were then separated by centrifugation at 2000 rpm for 15 min. The concentration of ACV in the aqueous layer was determined spectrophotometrically after suitable dilution. The apparent partition coefficient “Ko/w” was calculated as the ratio of the concentration of ACV in each phase by using the following equation [Citation22] as depicted in EquationEquation (1)(1)
(1) .
(1)
(1) where Caq is the initial concentration of ACV in the aqueous phase and Ceq is the concentration of ACV at equilibrium in aqueous phase.
Construction of pseudoternary-phase diagrams
To find the microemulsion region, pseudoternary-phase diagrams were constructed by the titration of varied concentrations of oil (IPM), surfactant (Tween 20) and cosurfactant (Span 20), with aqueous phase (water: DMSO (1:3)) at ambient temperature [Citation23–25]. The ratio of oil to the mixture of surfactant and cosurfactant was varied from 9:1 to 1:9. Aqueous phase was added drop by drop and vortexed for sufficient period of time till equilibrium was attained. After this, the mixtures were observed visually for transparency, phase separation and gel formation. Clear region obtained was oil-in-water microemulsion region. Separately the mixture of surfactant, cosurfactant and aqueous phase were titrated in a similar manner with oil to obtain clear w/o microemulsion region and checked for same parameters.
Preparation of microemulsion formulations
The w/o microemulsion region in the phase diagram was identified and a microemulsion formulations containing acyclovir was prepared [Citation25]. Acyclovir being a hydrophilic drug was dissolved in water: DMSO mixture (aqueous phase). Tween 20, Span 20 and water-soluble penetration enhancer (PG) were added and stirred for 10 min. Accurately weighed amount of oil along with oil-soluble penetration enhancer (menthol) was added dropwise under constant stirring to the aqueous phase. A clear microemulsion was obtained by stirring the mixtures for 10 min. The composition of different microemulsion formulations are listed in . For comparative studies, both conventional PEG ointment containing 5% w/w ACV and marketed cream Acivir® (5%) were used.
Table 1. Composition of various microemulsion formulations.
Characterization of microemulsion
Morphology, micromeritics and zeta potential
Morphology and structure of drug-loaded microemulsions was determined with the aid of transmission electron microscopy (TEM). Photomicrographs at suitable magnifications were obtained using negative staining with 1% phosphotungstic acid on carbon-coated copper grids [Citation25]. Analysis of microemulsion’s globule size was carried out by dynamic light scattering (DLS) technique, using a computerized Malvern’s Zetasizer®.
pH determination
The pH of prepared formulations was determined at room temperature with a glass electrode pH meter (Labindia Pico+, Mumbai, India).
Rheological behaviour studies
The developed formulations were studied for their rheological behaviour using Brookfield digital viscometer equipped with small adaptor and spindle number 21. The microemulsions were submitted to up and down cycles (0–100 rpm spindle rotation speed) at ambient temperature and the rheological behaviour of each dispersed system was evaluated by plotting the shear stress (F) versus the shear rate (G) [Citation21,Citation23].
Drug content
For the determination of drug content, 50 mg of the prepared microemulsion was dissolved in 1 mL of DMSO. 100 μL of the mixture was taken and diluted to 10 mL with distilled water and the absorbance of the resulting solution was measured at λmax 254 nm against a blank microemulsion treated in the same manner.
Thermodynamic stability studies of microemulsion systems
Stability under centrifugation
Resistance of a microemulsion to centrifugation depends on the difference in density between the oily and aqueous phases and also on the resistance of the interfacial film. Therefore, with quite similar formulations which exhibit small density differences, the stability under centrifugation reflects the strength of the interfacial film. This study was carried out by taking 10 mL of microemulsion formulations in centrifuge tubes and centrifuged at 3500 rpm for 30 min [Citation26].
Hermetically sealed test tubes filled with the microemulsion were subjected to freeze/thaw cycle. The test tubes were vertically stored for 16 h in a freezer at −21 °C and then for 8 h at room temperature. The microemulsion was observed and any change was recorded. This cycle was repeated four times.
Temperature stress studies
Stability studies were conducted by storing the formulation at different temperature conditions. Each formulation was stored in sealed glass containers in refrigerator (4 ± 3 °C), at room temperature (25 ± 2 °C) and at higher temperature (40 ± 2 °C) for 45 days. After 1, 7, 14, 21, 30 and 45 days, the formulations were evaluated for any physical change like clarity, phase separation, precipitation of drug, colour change, drug content and pH [Citation21].
Evaluation of microemulsion
Ex vivo skin permeation study
Ex vivo permeation studies of microemulsion formulations of ACV were performed through mice skin using Franz diffusion cell assembly. The animals were sacrificed by cervical dislocation method. The hair on the dorsal side of mice was removed with an electric shaver. The shaven part of the skin was separated and excess fat and connective tissue were removed surgically using scalpel. The excised skin was washed with normal saline, examined for integrity and subsequently used [Citation27]. The skin was cut into circular pieces according to the size of external circumference of donor compartment. These pieces were mounted on the diffusion cell assembly with an effective diffusion area of 2.84 cm2, while keeping the stratum corneum towards the donor compartment and the permeation studies were conducted. The receptor compartment consisted of 30 mL phosphate buffer pH 6.4 maintained at 32 ± 0.5 °C [Citation28]. The prepared formulations (microemulsions (I-VI)/PEG ointment/marketed cream; Acivir® containing an amount equivalent to 3 mg of ACV were applied on to the membrane in the donor compartment. An aliquot of 2 mL sample was withdrawn at suitable time intervals and replaced immediately with an equal volume of fresh diffusion medium. The samples were analysed spectrophotometrically at 254 nm after suitable dilutions. The blank microemulsion formulations and controls were also subjected to similar treatment. At the end of the permeation experiments, the remaining formulation in the donor phase was scrapped off the skin, and the exposed skin surface was rinsed with water/DMSO (1:3) to remove excess drug from the surface. The receptor medium was then replaced with fresh water/DMSO (1:3). Receptor contents were allowed to stir for the next 24 h. On completion, the media was analysed for the amount of drug retained in skin [Citation29]. The permeation rate/flux values of ACV through mice skin was calculated from the slope of linear portion of the graph of cumulative amount of ACV permeated through skin plotted as a function of time. The permeability coefficient Kp (cm2/h) was calculated using EquationEquation (2)(2)
(2) :
(2)
(2) where Kp is the permeability coefficient, J the flux calculated at steady state and Co represents the drug concentration that remained constant in the vehicle [Citation30].
Statistical analysis
All the data were statistically analysed by one-way analysis of variance followed by multiple comparison Tukey’s test. p values of .05 was considered to be significant.
Histopathological examination
Formulation VI was applied uniformly on the dorsal pre-shaven region of mice and kept in contact with the skin for 4 h. After that the animals were sacrificed by spinal dislocation method and exposed dorsal surface was cut. Then, each specimen was fixed in 10% buffered formalin; embedded in paraffin and microtomed. The sections were stained with haematoxylin and eosin. Finally the specimens were observed under a high-power light microscope [Citation21].
In vivo antiviral studies
The therapeutic efficacy of different formulations of ACV was evaluated using murine model of cutaneous herpes simplex virus (HSV-I) infection. HSV-I was propagated in vero cells (African green monkey kidney cells) in minimum essential medium (MEM) supplemented with 0.22% sodium bicarbonate, 100 U of penicillin-streptomycin per mL, 2 mM L-glutamine and 2% foetal bovine serum (FBS) [Citation31]. Vero cells (African green monkey kidney cells) were cultivated in growth media. Cells were adsorbed as monolayer on glass surface. Then, the media were decanted and washed with phosphate buffer saline (PBS) pH 7.7. Then, trypsin 0.25% solution (composed of equal portions of trypsin, versene and glucose) in phosphate buffer saline was added to the monolayer and left in contact with the cells for 15–30 s, after which the solution was removed. The cells were then suspended in MEM to get the vero cell suspension. All the procedure was carried out in an aseptic area. Vero cell suspension (1 mL) was added aseptically to each well in 24-well plate and then incubated at 37 °C with 90% relative humidity and 5% CO2 overnight for complete monolayer formation.
Cytotoxicity assay
Cytotoxicity assay was used to calculate tissue culture infectious dose (TCID50). For cytotoxicity assay, 10-fold serial dilutions of a virus stock were prepared, and 0.2 mL aliquot was inoculated onto susceptible cell monolayers in each well and was incubated for 1–2 h at 36 ± 1 °C with 90% RH and 5% CO2. After incubation time, 0.8 mL of growth media containing 1% FBS was added and again incubated for 48 h at 35 ± 1 °C with 90% RH and 5% CO2. After 48 h of incubation, any change in cell (like ballooning, shrinkage, elongation, etc.) shape was confirmed and was scored as CPE [Citation32]. Finally, TCID50 was calculated using the formula given in EquationEquation (3)(3)
(3) :
(3)
(3)
Viral plaque assay
Plaque assay is one of the most important procedures in virology in measuring the virus titre (concentration of viruses) in a sample. To perform a plaque assay, 10-fold serial dilutions of a virus stock were prepared, and 0.2 mL aliquot was inoculated onto susceptible cell monolayers in each well and was incubated for 1–2 h at 36 ± 1 °C with 90% RH and 5% CO2 [Citation33]. After the incubation period, the monolayers were overlaid with 0.6% agarose (causes the formation of a gel) in MEM containing 2% FBS and incubated for 2–3 days [Citation31] to allow virus to attach to cells. When the plates were incubated, the original infected cells release viral progeny. Spreading of the new viruses is restricted to neighbouring cells by the gel. Consequently, each infectious particle produces a circular zone of infected cells called a plaque. Eventually, the plaque becomes large enough to be visible to the naked eye. Crystal violet was used as a dye to enhance the contrast between the living cells and the plaques. The titre of a viral stock was calculated in plaque-forming units (PFU) per millilitre. To determine the viral titre, the plaques were counted. To minimize error, only plates containing 10–100 plaques were counted. Each dilution was plated in triplicate to enhance accuracy. PFU/mL was calculated using formula given in EquationEquation (4)(4)
(4) :
(4)
(4)
Mouse HSV-1 infection
Female Balb/C mice were anesthetized by intraperitoneal injection of a mixture containing 70 mg of ketamine hydrochloride and 11.5 mg of xylazine per kg of body weight [Citation31]. Dorsal hair was removed using an electric clipper. Cutaneous infection with HSV-1 strain was initiated by first puncturing the skin of anaesthetized mice with a 27 gauge needle in cross hatch manner and subsequently spreading 100 μL (1000 TCID50/1.34 × 103 plaque-forming units) of viral stock over the puncture site. Mice were inoculated bilaterally on either side of the thoraco-lumbar region.
Measurement of viral disease and drug treatment
Formulations were applied over the site of viral inoculation once daily 24-h post-inoculation (i.e. prior to the appearance of zostiform rash). Approximately, 250 μL of formulation VI containing 0.75 mg ACV, 150 mg of marketed cream containing 7.5 mg ACV were applied to each mouse. For all treatment regimens with ACV, the total treatment time was 5 days. The efficacy of different formulations was evaluated in terms of evolution of lesions and reduction in number of lesions. Signs of disease were monitored using a subjective scoring system shown in [Citation4].
Table 2. The mean scores recorded (ranging from 0 to 4) depending on the degree of infection.
Results and discussion
Solubility studies
Solubility of ACV was determined in different media to select the appropriate medium for in vitro permeation studies and optimal aqueous medium for developing w/o microemulsion formulations of hydrophilic ACV. In water:DMSO (1:3), solubility of ACV was found to be maximum 13.7 ± 0.3 mg/mL; therefore, it was chosen as the most appropriate aqueous phase for the development of microemulsion. In phosphate buffer pH 6.4, the drug was found to be sufficiently soluble (1.46 ± 0.2 mg/mL) for being used as a receptor medium and for maintaining sink conditions during ex vivo permeation studies.
Partitioning studies
As the partitioning behaviour of a drug molecule plays an important role in skin lipid barrier transfer as well as drug loading into a carrier system containing both oil and aqueous phase, the partition coefficient of the drug was determined in octanol-water and IPM-water system. ACV showed a partition coefficient of −1.29 in octanol-water system and −1.15 in IPM-water system. The negative log p values indicates the hydrophilic nature of the drug. Since such types of hydrophilic drugs do not easily penetrate the lipoidal barriers of the skin, it was, therefore, a challenge to formulate a good carrier system for this drug in order to deliver it effectively through the skin.
Pseudoternary-phase diagram
The phase diagram facilitated the determination of concentration range of the components for the existence of microemulsion and helped in investigating the effect of different surfactant/cosurfactant weight ratios on the extent of stable microemulsion region. The pseudoternary-phase diagram is depicted in . Based upon microemulsion sustainability Tween 20 and Span 20 (3:1); water:DMSO (1:3); IPM were selected as surfactant/cosurfactant mixture; aqueous phase; oil phase.
Characterization of microemulsion
The microemulsion formulations were clear and transparent. The transmission electron micrograph revealed spherical nature of the microemulsion droplets (). The mean diameter of the optimized formulation was found to be 198.2 nm with polydispersity index of 0.180 () [Citation34,Citation35]. The zeta potential of the prepared microemulsion was found to be 0.279 mV. The pH was found to vary between 6.8 and 7.0, a near neutral range ideal for topical applications. Rheological studies showed Newtonian nature of the microemulsions. The viscosity of optimized formulation was 121.7 mPas at 29 °C, suggesting suitability for topical application. The drug content of different formulations was found to be in the range of 98.5 ± 0.24 to 99.2 ± 0.18%.
Thermodynamic stability studies
The selected microemulsion formulations when centrifuged at 3500 rpm for 30 min and subjected to freeze thaw cycles, showed no phase separation or drug precipitation, indicating that the microemulsion formulations were physically stable.
The selected microemulsion formulations remained clear even after a period of 3 months at 4 ± 3 °C, 25 ± 2 °C and 40 ± 2 °C and were found to be consistent with respect to their drug content, viscosity and transparency during the stability study.
Ex vivo skin permeation studies
The permeation profiles of ACV through mice skin from different formulations is shown in . The mean cumulative amount permeated per unit area in 24 h was found to be least from the conventional PEG ointment (240.047 ± 12.259 μg/cm2). This may be attributed to the drug–vehicle interactions that result in a lower thermodynamic activity of the drug [Citation36]. The retardant effect of PEG may be due to its inability to hydrate the stratum corneum or to a relative osmotic effect which tends to dehydrate the stratum corneum [Citation37]. As compared to PEG ointment, better drug permeation was observed from the marketed cream (372.646 ± 9.229 μg/cm2) because of the penetration enhancing properties of its components. It was observed that the permeation was significantly increased in all microemulsion based formulations. This is because in microemulsion formulation the drug was enclosed within the internal aqueous core, hence, its permeation increased because the droplets of internal phase could penetrate directly into the skin. Since the drug in marketed cream and PEG ointment was present in free form, it was not able to permeate the skin due to hydrophilic nature of the drug.
Figure 3. Comparison of cumulative amount permeated per unit area of acyclovir from various formulations in 24 h (The readers are referred to the web version of the article).
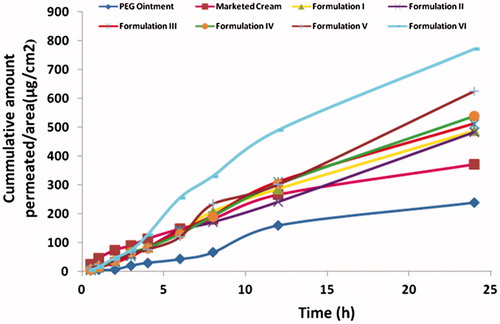
The flux of the Formulation I was found to be 23.46 ± 1.13 μg/cm2/h which was comparable with marketed cream, 21.77 ± 0.17 μg/cm2/h and was significantly higher than PEG ointment 7.64 ± 0.16 μg/cm2/h (. Formulation I showed an enhancement ratio of 3.07, when compared with PEG ointment. This could be explained on the basis of the drug–vehicle interaction that resulted in a lower thermodynamic activity of the drug [Citation36]. According to some researchers, the retardant effect of PEG is due to its inability to hydrate the stratum corneum or to a relative osmotic effect which tends to dehydrate the stratum corneum [Citation37].
Figure 4. Comparison of flux, skin retention and permeability coefficient of various formulations through mice. (The readers are referred to the web version of the article).
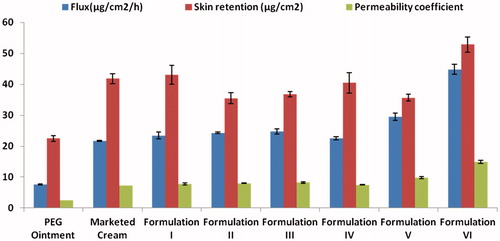
Also, the skin retention studies revealed that the retention from Formulation I (43.06 ± 3.1 μg/cm2) is comparable to marketed cream; 41.81 ± 1.58 μg/cm2 and 1.91 times higher than PEG ointment; 22.49 ± 0.99 μg/cm2 indicating the prolonged retention of drug in the layers of stratum corneum (. This was because the oil component of microemulsion formulation formed a depot in the skin, which helped in more retention of drug in skin.
The ex vivo permeation of ACV from various formulations through mice skin was significantly enhanced with the use of permeation enhancers like menthol, PEG, etc. Upon increasing the concentration of permeation enhancer, menthol from 1.0% (Formulation II) to 5.0% (Formulation IV), the drug permeation was enhanced. Formulation II (1% menthol) showed no enhancement in drug permeation. Formulation III (2.5% menthol) and IV (5% menthol) showed increased permeation by 1.05 and 1.11 times, respectively, with respect to Formulation I. The enhancement achieved with menthol may be due to loosening of the stratum corneum lipid bilayer due to breaking of H − bond between ceramides. Ceramides (abundantly present in stratum corneum) are tightly packed in the bilayer due to the high degree of hydrogen bonding. This hydrogen bonding provides strength and stability to lipid bilayer and imparts the barrier property to stratum corneum. When skin was treated with menthol, the existing network of hydrogen bonds between ceramides might get loosened because of “competitive hydrogen bonding”. This could result in penetration of water into the lipids of the stratum corneum lipid bilayer that leads to creation of new aqueous channels, which was responsible for increased hydrophilicity of the stratum corneum bilayer and resulted in favourable conditions for ACV, a hydrophilic drug, to penetrate into skin.
Formulation V containing 5% PG also showed significant increase in drug permeation (625.195 ± 8.629 μg/cm2) by 1.28 times, when compared with Formulation I. The enhancement achieved might be due to the fact that PG permeated well through human stratum corneum and altered the thermodynamic activity of the drug in the system which in turn modified the driving force for diffusion, and partitioned into the tissue facilitating uptake of the drug into skin. Also, there might be some minor disturbance to intercellular lipid packing within the stratum corneum bilayer, thus enhancing the drug permeation. Moreover, it is reported that when PG is used in combination with other penetration enhancer, it shows synergistic effect on drug permeation [Citation10]. So, a combination of 5% PG and 5% menthol (Formulation VI) was evaluated with respect to its permeation characteristics. The significant enhancement in the mean cumulative amount permeated per unit area in 24 h (771.76 ± 1.66) along with maximum flux (44.84 ± 1.58 μg/cm2/h) and skin retention (52.87 ± 2.44 μg/cm2) indicated that combination of menthol and PG also acts as the best penetration enhancer for ACV among the prepared microemulsion formulations.
Histopathological studies
Dermal tolerance of blank microemulsion and formulation VI containing penetration enhancers was evaluated. No change was observed in the anatomical structure and no pathological changes were found indicating the safety of developed microemulsion formulation on the skin (.
In vivo pharmacodynamic studies
Cytotoxicity assay
Cytotoxicity assay was carried out to determine TCID50 values of viral stock. Various dilutions ranging from 10−1 to 10−8 were made and TCID50 calculated using cytotoxicity assay was found to be 0.27 at 10−3 dilution.
Plaque assay
The titre of a viral stock was calculated in plaque-forming units (PFU) per millilitre. The plaque assay was used to prepare clonal viral stocks, to inoculate into mice. The infectivity of viral stock was calculated to be 1.34 × 104 PFU/mL
Efficacy of different formulations
Exposure to HSV-1 at mucosal surfaces or abraded skin sites permits the entry of virus and initiation of its replication in cells of epidermis and dermis [Citation38]. The pharmacodynamic evidence of the ACV formulations was carried out by employing the murine model of cutaneous HSV type-I infection. The efficacy of the optimized formulation VI containing 5% menthol and 5% PG as enhancers was tested after once daily application (post-infection) in mice. In the untreated infected animals (negative control), pathological signs of primary infection were visible 24-h post-infection. When the virus is inoculated in the skin, the primary infection occurs, it starts spreading probably by retrograde axonal flow, to sensory ganglia and the central nervous system. Thereafter, the virus reaches axons that innervate skin within the same dermatome as the inoculation site, spreads via orthograde flow into the skin and produces herpetic lesions within the affected dermatome [Citation31]. With the infected mice treated with the optimized formulation VI, it was seen that the infected area got cleared on second day after once daily application in comparison to five times daily application with marketed cream. depicts the visual evaluation of eradication of infection with application of formulation VI in comparison to marketed cream. The reduction in mean infection score is depicted in . Higher therapeutic efficacy was observed due to better penetration profile of microemulsion system compared to the marketed cream. The prepared microemulsion formulation was highly effective with single daily application of 1/10 dose as compared to marketed cream of ACV. The side effects like burning and stinging associated with marketed cream were not observed due to significant reduction in dose.
Figure 6. Visual evaluation of eradication of infection (a) Mice showing ulcerative lesions due to HSV infection (b) mice treated with once daily application of Formulation VI (c) mice treated with five times application of marketed cream. (The readers are referred to the web version of the article).
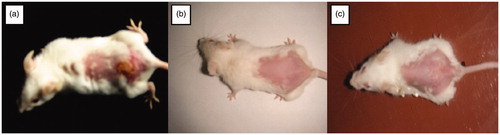
Conclusions
It can be concluded that microemulsion formulations containing penetration enhancers can efficiently increase the penetration and skin retention of ACV. Both ex vivo skin permeation studies and in vivo investigations using murine model of zosteriform cutaneous HSV-I infection confirmed the superior efficacy of the developed microemulsion formulations in comparison to conventional ointment and cream. Therefore, the developed system possessing optimum formulation characteristics could be utilized to reduce encumbrance of conventional oral and topical therapy. However, further human clinical investigations are essential to fully establish the antiviral potential of microemulsion-based ACV formulations for the topical treatment of cutaneous infections of HSV.
Acknowledgements
The authors gratefully acknowledge the gift sample of ACV supplied by Arochem Industries (Mumbai, India).
Disclosure statement
The authors report no conflicts of interest. The authors alone are responsible for the content and writing of this article.
References
- Ryan KJ, Ray CGSMM. Sherris medical microbiology. 4th ed. New York (NY): McGraw Hill; 2004.
- Kleymann G. Helicase primase: targeting the Achilles heel of herpes simplex viruses. Antivir Chem Chemother. 2004;15:135–140.
- Jain S, Mistry MA, Swarnakar NK. Enhanced dermal delivery of acyclovir using solid lipid nanoparticles. Drug Deliv Transl Res. 2011;1:395–406.
- Bolger GT, Allen T, Garneau M, et al. Cutaneously applied acyclovir acts systemically in the treatment of herpetic infection in the hairless mouse. Antiviral Res. 1997;35:157–165.
- Bryson YJ, Dillon M, Lovett M, et al. Treatment of first episodes of genital herpes simplex virus infection with oral acyclovir. A randomized double-blind controlled trial in normal subjects. N Engl J Med. 1983;308:916–921.
- Fiddian AP, Yeo JM, Clark AE. Treatment of herpes labialis. J Infect. 1983;6:41–47.
- Freeman DJ, Sheth NV, Spruance SL. Failure of topical acyclovir in ointment to penetrate human skin. Antimicrob Agents Chemother. 1986;29:730–732.
- Parry GE, Dunn P, Shah VP, et al. Acyclovir bioavailability in human skin. J Invest Dermatol. 1992;98:856–863.
- Lawrence MJ, Rees GD. Microemulsion-based media as novel drug delivery systems. Adv Drug Deliv Rev. 2000;45:89–121.
- Williams AC, Barry BW. Penetration enhancers. Adv Drug Deliv Rev. 2004;56:603–618.
- Hua N, Liu W, Guo RC. Development and evaluation of terpene penetration enhancers. Zhongguo Zhong Yao Za Zhi. 2008;33:2875–2882.
- Vaddi HK, Ho PC, Chan SY. Terpenes in propylene glycol as skin-penetration enhancers: permeation and partition of haloperidol, Fourier transform infrared spectroscopy, and differential scanning calorimetry. J Pharm Sci. 2002;91:1639–1651.
- Patel T, Ishiuji Y, Yosipovitch G. Menthol: a refreshing look at this ancient compound. J Am Acad Dermatol. 2007;57:873–878.
- Jain AK, Thomas NS, Panchagnula R. Transdermal drug delivery of imipramine hydrochloride. I. Effect of terpenes. J Control Release. 2002;79:93–101.
- Godwin DA, Michniak BB. Influence of drug lipophilicity on terpenes as transdermal penetration enhancers. Drug Dev Ind Pharm. 1999;25:905–915.
- Tas C, Ozkan Y, Okyar A, et al. In vitro and ex vivo permeation studies of etodolac from hydrophilic gels and effect of terpenes as enhancers. Drug Deliv. 2007;14:453–459.
- Chang JS, Tsai YH, Wu PC, et al. The effect of mixed-solvent and terpenes on percutaneous absorption of meloxicam gel. Drug Dev Ind Pharm. 2007;33:984–989.
- Rizwan M, Aqil M, Ahad A, et al. Transdermal delivery of valsartan: I. Effect of various terpenes. Drug Dev Ind Pharm. 2008;34:618–626.
- Narishetty ST, Panchagnula R. Transdermal delivery of zidovudine: effect of terpenes and their mechanism of action. J Control Release. 2004;95:367–379.
- Avdeef A. Solubility of sparingly-soluble ionizable drugs. Adv Drug Deliv Rev. 2007;59:568–590.
- Sharma G, Dhankar G, Thakur K, et al. Benzyl benzoate-loaded microemulsion for topical applications: enhanced dermatokinetic profile and better delivery promises. AAPS PharmSciTech. 2016;17:1221–1231.
- Shojaei AH, Berner B, Xiaoling L. Transbuccal delivery of acyclovir: I. In vitro determination of routes of buccal transport. Pharm Res. 1998;15:1182–1188.
- Chhibber T, Wadhwa S, Chadha P, et al. Phospholipid structured microemulsion as effective carrier system with potential in methicillin sensitive Staphylococcus aureus (MSSA) involved burn wound infection. J Drug Target. 2015;23:943–952.
- Schmidts T, Nocker P, Lavi G, et al. Development of an alternative, time and cost saving method of creating pseudoternary diagrams using the example of a microemulsion. Colloid Surf A: Physicochem Eng Aspects. 2009;340:187–192.
- Negi P, Singh B, Sharma G, et al. Phospholipid microemulsion-based hydrogel for enhanced topical delivery of lidocaine and prilocaine: QbD-based development and evaluation. Drug Deliv. 2016;23:951–967.
- Roland I, Piel G, Delattre L, et al. Systematic characterization of oil-in-water emulsions for formulation design. Int J Pharm. 2003;263:85–94.
- Zhu W, Guo C, Yu A, et al. Microemulsion-based hydrogel formulation of penciclovir for topical delivery. Int J Pharm. 2009;378:152–158.
- Bolzinger MA, Briancon S, Pelletier J, et al. Percutaneous release of caffeine from microemulsion, emulsion and gel dosage forms. Eur J Pharm Biopharm. 2008;68:446–451.
- Jain S, Jain P, Umamaheshwari RB, et al. Transfersomes-a novel vesicular carrier for enhanced transdermal delivery: development, characterization, and performance evaluation. Drug Dev Ind Pharm. 2003;29:1013–1026.
- Zhao X, Liu JP, Zhang X, et al. Enhancement of transdermal delivery of theophylline using microemulsion vehicle. Int J Pharm. 2006;327:58–64. Epub 2006 Jul 21.
- Piret J, Desormeaux A, Gourde P, et al. Efficacies of topical formulations of foscarnet and acyclovir and of 5-percent acyclovir ointment (Zovirax) in a murine model of cutaneous herpes simplex virus type 1 infection. Antimicrob Agents Chemother. 2000;44:30–38.
- Koch C, Reichling J, Schneele J, et al. Inhibitory effect of essential oils against herpes simplex virus type 2. Phytomedicine. 2008;15:71–78.
- Sakuma S, Yamamoto M, Kumano Y, et al. An acyclovir-resistant strain of herpes simplex virus type 2 which is highly virulent for mice. Arch Virol. 1988;101:169–182.
- Alam P, Ansari MJ, Anwer MK, et al. Wound healing effects of nanoemulsion containing clove essential oil. Artif Cells Nanomed Biotechnol. 2017;45:591–597.
- Laxmi M, Bhardwaj A, Mehta S, et al. Development and characterization of nanoemulsion as carrier for the enhancement of bioavailability of artemether. Artif Cells Nanomed Biotechnol. 2015;43:334–344.
- Davis SS, Hadgraft J, Al-Khamis K. Percutaneous absorption of methyl salicylate from propylene glycol vehicles. J Pharm Pharmacol. 1981;33:97–102.
- Barrett CW, Hadgraft JW, Sarkany I. The influence of vehicles on skin penetration. J Pharm Pharmacol. 1964;16:SUPPL:104–SUP7T.
- Wang F, Kief E. Viral diseases. In: Fauci AS, Braunwald E, Isselbacher KJ, Wilson JD, Martin JB, Kasper DL, Hauser SL, Longo DL, editors. Harrison’s principles of internal medicine. Singapore: McGraw-Hill; 1998. p. 1076–1085.