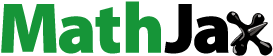
Abstract
Biosensors are the switching channels that make sense. The biosensors have found an empirical role in health applications (e.g. clinical diagnostics) as they represent the technological counterpart of living senses. On a global scale, alcohol analysis is indispensable for criminal justice systems, monitoring medical conditions of HIV patients & pregnant women as well as public safety issues regarding pilots, metro drivers, doctors etc. For addressing the clinical and toxicological problems, much advancement in the improvement of biosensors have been witnessed in recent decades. Currently, electrochemical biosensors dominate the field which harnesses the synergistic action of enzymes and nanomaterials for the analysis of ethanol. The enzymatic biosensors are the most explored biosensing devices among all the types of biosensors, and employment of nanomaterials has paved a way to the further improvements in this gem of a discovery. The relative comparison to precise the alcohol biosensors has been aptly discussed in the review on the basis of several analytical properties including fabrication, linearity, sensitivity, response time, detection limit as well as storage stability. Finally, the recent trends and emerging future prospects of alcohol biosensors have been reviewed.
Introduction
Over the years, there is a great necessity of fast and reliable affirmation of ethanol in various areas like forensic science, industries and clinical analysis. It is one of the most common poisonous compounds consumed by human beings. Ethanol is a causative factor in a variety of accidents, and it is often involved in clinical diagnostics analyses like urine, serum and blood analysis [Citation1]. When the concentration of ethanol reaches toxic levels during fermentation and distillation, it may lead to swelling and conjunctiva of the nasal mucous layer and exasperation of the skin. When the concentration of ethanol reaches to a higher level in serum, it can lead to alcohol poisoning. Therefore, the quantitative measurement of ethanol is very essential to control the fermentation processes, the quality of product in industrial fields like food, beverages and pulp industries [Citation2] and additionally in clinical science in view of its toxicological and mental effects on wellbeing of humans [Citation3]. In addition of these applications, it is also important to determine the ethanol content in environment and agricultural analyses [Citation4]. A variety of analytical methods have been accounted till now for the sensing of this analyte. Some of these conventional analytical methods have been described in [Citation5–11].
Table 1. Different methods other than enzymatic approaches used for the measurement of ethanol in various types of samples.
As enzymes have efficient catalytic properties and specificity, enzyme biosensors have emerged as a precious technique for quantitative analysis of alcohol by offering significant benefits such as portability, mass production, high sensitivity, potential for miniaturization, fast analysis, bedside clinical diagnosis as well as relatively low cost of construction over the conventional analytical methods [Citation1,Citation4]. In addition, there is no need of laborious sample preparation, good skilled operator and expensive instrumentation in these biosensors. Even if biosensors based on other bioactive elements are making progress at a fast pace, enzyme biosensors are still one of the most recurrently used in the analysis ranging from clinical diagnostics (e.g. blood, sweat, serum, breath, saliva and urine) to the food and alcoholic beverages (wine, brew and spirits). For various applications, the enzymes belonging to the category of oxidoreductases have been employed and integrated with a variety of transducers for the construction and commercialization of majority of biosensors. In amperometric enzyme biosensors, the biocatalytic oxidation or reduction of the analyte of interest results in a redox, electron transfer process at the surface of the electrode having immobilized enzyme. The current generated through this electron transfer process is directly proportional to the concentration of the analyte and quantified by using an electrochemical set-up. Developments regarding the use of novel electrode materials and different strategies for immobilization of enzymes have been reviewed. During a chemical reaction, immobilized enzymes do not lose their efficacy alongwith the potential of reusability which makes them more attractive and valuable. The formation of product in a controlled way can be achieved through the use of enzyme biosensors. The two major enzymes involved in catalytic reaction of ethanol sensing amperometric biosensor are alcohol oxidase (AOX) and alcohol dehydrogenase (ADH). AOX (alcohol:oxygen oxidoreductase, EC 1.1.3.13, AOX) is an enzyme made up of eight indistinguishable monomeric subunits and there is non-covalent attachment of a cofactor named flavine adenine dinucleotide molecule (FAD) to every subunit [Citation12]. The chemical reaction catalyzed by the enzyme AOX involves the oxidation of primary low molecular weight alcohols into their corresponding aldehydes. Mostly all the ethanol biosensors which are based on the AOX rely upon the monitoring of oxygen utilization or hydrogen peroxide formation. In the case of ethanol biosensors which are based on ADH, oxidation of ethanol to acetaldehyde is catalyzed by the enzyme in the presence of NAD+ and further NAD+ is reduced to NADH. This NADH can be oxidized by releasing electrons and protons which is monitored by amperometric detection [Citation2]. The ADH has the preferred standpoint to be steadier and more selective to ethanol and not being dependent on oxygen but additionally it face drawback of being unstable and the inconvenience to be reliant on the coenzyme NAD+ which needs continuous recovery in the assay [Citation1]. Moreover, overpotentials higher than 0.4 V vs. reference electrode are obligatory for the oxidation of NADH on a bare electrode and its electrochemical detection. But unfortunately at this particular potential various different substances present in samples, is likewise oxidized and can meddle in the estimation. To overcome this disadvantage, it has been reported that a mediator can be utilized as an electrocatalyst for decreasing the overpotential and preventing electrode fouling. When a mediator is added in the construction of biosensors, it enhances the rate of electron exchange between the cofactor of redox enzymes and electrodes [Citation13]. Consequently, there is decline in working potential and reduction in the number of interferences permitting a higher sensitivity to the biosensor. As an alternative, AOX does not require cofactor regeneration. A standout amongst the most distinctive features of AOX is the self-bound cofactor associated with the redox center of this enzyme that opposes the need to supplement cofactor amid the catalytic reaction. This flavin-based redox chemical has increased enormous significance in the improvement of biosensors. The immobilized AOX has more advantages over the native enzyme because of its tolerance towards the denaturing reagents and high thermal resistance, and their multiple and repetitive use make it more preferable over the native ones [Citation14]. Integration of nanomaterials decorated with biomolecules and polymers have been explored to achieve better biocompatibility and specific targeting.
Basic principle of enzyme-based biosensors
An enzyme biosensor is an analytical device, which is based on a principle that employs the interaction of an enzyme immobilized on electrode surface with a specific analyte (the compound whose concentration is to be determined in the sample) and production of a physical or chemical change as shown in . The role of transducer or the detecting element is to transforms the physical or chemical signal resulting from the interaction of the enzyme and a specific analyte into an electrical signal that can be easily measured. Finally, the amplification and interpretation of the electrical signal is done and displayed as analyte concentration in the solution/preparation. For the accurate detection of the analyte in different samples, the enzyme must be extremely specific to the analyte [Citation12]. Potentiometric biosensors detect changes in the electrical potential produced as a result of changed distribution of electron after the biological reaction between the enzyme and the analyte. These biosensors utilize ionselective electrodes for converting the biological reaction to the electronic signal. Mostly pH meter glass electrodes are used. The concentration of the analyte is directly proportional to the potential difference of potentiometric electrode and the reference electrode. Calorimetric or thermometric biosensors are employed in the enzyme catalyzed reactions which are endothermic or exothermic in nature. These biosensors measure the heat absorbed or released by the reaction. Optical biosensors utilize the principle of optical measurements like fluorescence and absorbance by detecting the light produced or absorbed as a result of an enzyme-catalyzed reaction. The piezoelectric biosensor or acoustic biosensor utilizes the piezoelectric effect, to evaluate changes in pressure, temperature, force and acceleration by converting them to an electrical charge. In piezoelectric effect, when certain crystals which possess positive or negative charges are subjected to stress, they separate and generate an electric field. In amperometric enzyme biosensors, when an oxidation or reduction reaction takes place at the surface of an electrode, the current is produced which is directly proportional to the concentration of that analyte present in the particular sample. So the main principle involved in the working of amperometric enzyme biosensors function by the measurement of the current response generated when potential is applied between working and reference electrodes. This current is produced as a result of the electrocatalytic oxidation or reduction reaction of the electroactive species involved in the chemical reaction and its magnitude is directly correlated to the analyte concentration. In general, amperometric enzyme sensors are center of attention for the detection of ethanol in blood or saliva due to their high performance and simple designing. In most of the enzymatic amperometric biosensors, either there is consumption of O2 e.g. all the oxidases, or production of H2O2 (except oxidases which generate water), or the reduced form of β-nicotinamide adenine dinucleotide is produced indirectly by the catalytic breakdown of substrate (such as by dehydrogenases).
Enzymes involved in alcohol biosensing
The enzyme-based alcohol biosensors involve coupling of an enzyme with the biosensing electrode by placing it in close proximity to the surface of an electrode. The enzyme employed in the chemical reaction consumes electroactive reactant or generate electroactive species during catalysis. As a result of the production or depletion process, the direct measurement of the concentration of analyte can be done. The two key enzymes which have attracted the attention in alcohol detection are AOX and ADH as the test case for many biological applications. These are the most frequently used biological recognition elements due to the occurrence of their simple enzymatic reaction and effortless fabrication of the sensor.
Properties of alcohol oxidase
Alcohol oxidase (AOX, alcohol oxidoreductase, EC 1.1.3.13) is the chief enzyme for alcohol metabolism in Hansenula polymorpha, Pichia pastoris, Candida boidinni and some other yeast species. The initial step of alcohol catabolism in the cells of yeast, to be specific its oxidation to acetaldehyde, with corresponding generation of hydrogen peroxide is done by the enzyme AOX as per the reaction represented in equation.
The oxidation reaction of alcohols by AOX is irreversible in nature, owing to the strong oxidising character of O2. The enzymatically active form of AOX is a homo-octameric flavoenzyme having size approximately 600 kDa and bound with a flavin adenine dinucleotide which serves as a cofactor to the enzyme [Citation15]. This flavoenzyme consists of eight identical subunits which are sequentially arranged in a quasi-cubic arrangement. In the catalytic process, first there is reduction of the cofactor i.e. FAD into the hydrogenated form (FADH2) and then again its reoxidation to the native form. The standard pathways to monitor a reaction catalysed by an oxidase enzyme have been performed by evaluating either the consumption of O2 or the production of H2O2.
AOX biosensors based on O2 detection
Electrochemical methods are commonly used in the AOX biosensors that screen the utilization of O2, albeit optical strategies can likewise be utilized. The most well-known technique for observing O2 depends on a Clark-type O2 electrode. The vast majority of ethanol sensors taking into account O2 probes comprise of an electrode enclosed by a layer containing immobilized AOX. The primary AOX electrode comprised of the AOX immobilized and mounted on the surface of a platinum electrode and a nylon fabric and an O-ring were used to secure it. The sign yield of the electrode is the distinction between the base oxygen level (100% immersion) and the level accomplished as an aftereffect of oxygen consumption by the enzymatic response. The advantage of oxygen-based sensor lies in the fact that there is no electrochemical obstruction from other constituents present in the sample. However, the oxygen-dependent nature of these sensors led to some practical limitations like slow response, and low accuracy and reproducibility [Citation16]. In optical method, some biosensors utilizing the phenomenon of fluorescence for the estimation of alcohol have been reported in literature. The other type of optical biosensors was also constructed using O2 sensitive dyes like ruthenium complex derivative which were immobilized on a PVC membrane together with AOX enzyme [Citation17]. Due to detection of O2 has limitation particularly in low accuracy and reproducibility. To overcome these drawbacks, the most commonly used alternative is the detection of H2O2.
AOX biosensors based on H2O2 detection
AOX biosensors can employ optical and electrochemical methods [Citation18] that rely on the production of H2O2. In electrochemical method, amperometric electrodes can be used to detect the H2O2 produced by AOX, owing to the chemical reaction involving oxidation or reduction of H2O2 at the surface of the working electrode.
The most important advantage of the H2O2 sensor over different sorts of sensors is the comparative ease of fabrication and miniaturization. The broad linearity and more extensive direct range are the attributes of these sensors. In any case, the high potential indispensable to oxidize H2O2 represents an issue of electrochemical impedance, owing to the occurrence of some reducing compounds like uric acid and ascorbic acid in real sample matrices which are likewise oxidized at the same potential. Besides, time consuming reactions are scrutinized. Alternative routes have been proposed to follow the production of H2O2 keeping in mind the end goal to defeat the disadvantages experienced with the direct H2O2 detection. One of ways to diminish the essential applied potential for the chemical reaction either the reduction or oxidation of H2O2, is to amend the electrode with an electrocatalyst species (mediators). Prussian blue [Citation19], Meldola blue [Citation1,Citation13], ferrocyanide [Citation20], ferrocene [Citation21], cobalt ion and Co-phthalocyanine [Citation22] have been proposed as redox mediators. In optical biosensor, recently a method has been accounted for to take after the oxidation of alcohols by AOX. In this method, PANI has been used to detect the H2O2 generated in the reaction, where H2O2 oxidize the PANI, by changing the color from green to blue. The biosensor was constructed on the basis of dip stick format for simple use and clear visual detection [Citation23].
HRP-based H2O2 biosensors
To combat with various problems due to the presence of interfering compounds in the samples, horseradish peroxide (HRP) is used in the amperometric biosensor which provides an attractive alternate for the measurement of hydrogen peroxide. HRP is the most extensively studied and commonly used enzymes for the construction of H2O2 biosensors. The AOX and peroxidase-based reaction involve the oxidation of alcohols by AOX that produce H2O2, which further reduces to H2O by HRP, where the redox reaction can be monitored electrochemically as shown in . Moreover, the reduced form of HRP can be chemically re-oxidized by H2O2. The HRP has its active sites deeply buried in a protein shell, so the direct electron transfer between HRP and an electrode is very difficult and the electron transfer will slow down due to the large distance between the active sites and the electrode surface. The use of a mediator for the electron transfer, however, is more effective. Hence electrodes along with a mediator have been proved to fasten the transfer of electrons between the electrode and HRP, thereby lowering the detection limit and increasing the sensitivity. The majority of the optical systems reported in the text for the estimation of ethanol till now are mostly relied on the bi-enzymatic system which uses both the enzymes AOX and HRP together to reduce H2O2 to H2O at the expenditure of hydrogen donor molecules. An ample range of fluorigenic, luminogenic and chromogenic substrates has been used. 2,2-Azino-di (3-ethylbenzthiazoline-6-sulfonate), ABTS is the most preferred substrate in the midst of all. ABTS in its oxidized state shows blue color and a wavelength of around 415 nm is used to detect it. In this method, AOX and HRP were immobilized on the inner surface of a 96-well microtiter plate. Samples containing ethanol were added to the AOX microtiter plate and after the reaction (30 min), a sample was transferred to the HRP plate containing the chromogen, ABTS. A linear response in the range 0.1–1 mM was obtained for ethanol concentrations. Various alcohol biosensors utilizing AOX have been shown in [Citation24–32].
Table 2. AOX-based biosensors.
Properties of ADH
Alcohol dehydrogenase (ADH; EC 1.1.1.1) is also known as Alcohol:NAD+ oxidoreductase as it belongs to the oxidoreductase family. The main function of the enzyme is to catalyze the oxidation of alcohols, utilizing NAD+ or NADP+ as the electron acceptor in the chemical reaction. The nature of the reaction is reversible and there can be a variety of substrates like primary aliphatic and aromatic alcohols other than methanol. ADH catalyzed reaction for the detection of ethanol has been shown in . Alcohol biosensors utilizing ADH enzyme have been accounted to be more stable and explicit over the AOX-based biosensors. The prerequisite of external addition of the coenzyme NAD+ during the chemical reaction involved in ADH biosensors poses a limitation to them. Moreover, it is necessary that the cofactor should not become irreversibly entrapped or linked to the enzyme rather it should be close enough to activate the enzyme only. Mostly, ADHs are found in yeasts. ADH present in liver has a more broad specificity than yeast ADH enzyme. Yeast ADH catabolize ethanol and is somewhat active only on the straight chain primary alcohols. It shows little activity towards certain secondary and branched chained alcohols. ADH is a tetramer, each subunit surrounded by one zinc atom. The two distinct active sites are present in each subunit which can be characterized by the presence of sulfhydryl groups. Furthermore, these active sites can be distinguished on the basis of differential reactivity with iodoacetate and butyl isocyanaten. A histidine residue has a crucial role in the activity of the enzyme. The common ways to monitor an ADH catalyzed reaction have been performed by detection of the products, i.e. the increase in NADH concentration. In living cells, NAD+ and NADH (products of the chemical reaction between ADH and primary alcohols) are considered to be the fundamental charge carriers. NAD+ participates in enzymatic catalysis of about more than 300 dehydrogenase enzymes which makes it a very significant cofactor. In electrochemical biosensor, the NAD+/NADH redox couple have been used as sensing scheme in various electrodes. However, there are only a few number of electrochemical biosensors based on ADHs for the reason that there is frequent constraint for cofactors regeneration [Citation33]. The high over-potentials are required for the electrochemical oxidation of NADH to the corresponding oxidized form NAD+ at a bare electrode surface because the reaction is highly irreversible; many works have been focused on the development of new efficient electrode materials [Citation34]. In order to reduce the high over-potentials and to minimize the side reactions, various redox mediators immobilized on the electrode surface have been reported in the literature. In general, the chemical reaction between oxidized mediator (MEDox) and NADH yields NAD+ as a product. Furthermore, as a result of the reaction between NAD+ and ADH, ethanol was converted to acetaldehyde. The redox reaction occurred on the electrode surface according to the following mechanism:
Ferrocene [Citation35], Catechols [Citation36], phenoxazines [Citation37], phenothiazines [Citation38], and conducting polymers [Citation39] are examples of immobilized redox mediators that have been studied comprehensively. The use of such mediators immobilized on an electrode coupled with ADHs and their applications to alcohol biosensor development have been discussed. Some of the ADH-based biosensors have been described below in [Citation40–49].
Table 3. ADH-based biosensors.
Material of the electrode
In designing of biosensors, the consideration of both the target analyte and the complexity of the matrix in which the analyte will be quantified are required. The measurements of an amperometric biosensor depend robustly on the material of the working electrode. Carbon paste electrodes have been the focus of research for the development of amperometric biosensors since late 1980s [Citation50]. Owing to the chemical–physical properties of carbon nanotubes or graphene and catalytic properties like chemical stability, high surface area, signal reproducibility and good biocompatibility, carbon is one of the most widely used material in electrochemical biosensors. Furthermore, the use of metals like gold, platinum and palladium has been exploited as transducers because the electron transfer is fast and H2O2 produced by the first generation oxidase enzyme-based biosensors is proficiently electro-oxidized to generate a signal [Citation51]. An assembly of three electrode systems i.e. a working electrode, a counter current electrode and a reference electrode contained in an electrochemical cell is required for the amperometric measurements of a biosensor. The working electrode having immobilized enzyme or different electroactive materials is the most important of all as the transfer of electrons occurs at its surface. Depending on the redox behavior of the analyte involved and the background current required over the potential region, the working electrode is selected for the measurements. At the surface of working electrode, a constant potential is maintained. In an electrochemical cell, the measurement of current flowing through the solution between the counter and working electrodes is done. The reference electrode (Ag/AgCl or saturated calomel electrode) is used to control the applied potential in the system. Hence it provides stability and reproducibility. Gold and platinum are the most commonly used electrode materials in the construction of alcohol biosensors.
Methods of AOX/ADH immobilization
The compact attachment of the enzyme onto the working electrode surface describes one of the most significant steps in the construction of an enzymatic biosensor. There are various kinds of interactions between the enzyme and the electrode material which govern this attachment process and responsible for the performance of the biosensor. There are a variety of physical and chemical methods for the immobilization of enzymes ranging from physisorption to the covalent cross-linkage. Several techniques which have been used for the immobilization of AOX/ADH during the construction of alcohol biosensor are depicted in .
Physical adsorption
In physical adsorption, there is a simple attachment of AOX/ADH enzyme onto the surface of the working electrode through weak electrostatic interactions and Van der Waals forces between the enzyme and the transducer. The electrodes are dipped in a solution of enzyme for a set period of time [Citation52]. Sometimes adsorption and dip-evaporation can be done followed by the exposer of glutaraldehyde or hexamethylenediamine for crosslinking the enzyme on the electrode surface. It is simple and economical method of immobilization without any kind of damage to the enzyme and support. Non-specific binding, leakage and overloading of the enzyme onto the support has been reported. In addition, there is short response time and poor operational stability.
Physical entrapment
The network of a polymer matrix or membrane and sol–gel matrix is utilized for the entrapment of the AOX/ADH enzyme in the process of physical entrapment. The basic principle behind the process is that the penetration of the substrate is allowed while retaining the protein. It is a convenient, cheap and simple one-step method of immobilization with no damage to the enzyme and support. It is also suitable for a vast range of bioreceptors even at low temperature. There is problem of enzyme leakage and unstable nonspecific immobilization along with the problems of pore size control and reproducibility.
Covalent coupling
It is the most widely used procedure for the immobilization of AOX/ADH enzyme. A stable covalent bond is formed between the nucleophilic functional groups of the enzyme (like carboxyl groups, hydroxyl groups, amino groups, sulfhydryl groups, thiols) and the transducer, and hence the covalent linkage is established between the enzyme and the surface of a transducer. It is a flexible method with specific physical and chemical properties for an extensive range of preferences for opting a carrier material. There is absence of diffusion barriers and enzyme leakage. This is a significant technique for improving the lifetime of the electrochemical biosensors. It is an expensive and complicated procedure which requires a large amount of enzyme. The functional enzymes are easily accessible to denaturation as well.
Self-assembled monolayer
When some amphiphilic molecules are organized in a layer, they constitute a self-assembled monolayer (SAM). These amphiphilic molecules have a head group at one end which illustrates a reversible affinity for a particular substrate. The presence of a functional group at the terminal end of the tail of these molecules has also been accounted. SAMs are nano-scale structures with the numerous useful properties. The thickness range of SAM is about 1–3 nm which makes them the most elementary form of an organic thin-film material at nanometer-scale. Narváez et al. reported an immobilization method of AOX on 3-mercapto-1-propane sulfonic acid (MPS) SAM-modified Au electrodes for alcohol detection. This reagentless alcohol biosensor was schematically corresponding to Au/MPS/RP/HRPmod/Binder/AOX [Citation53]. It offers a simple route to functionalize the surfaces of electrode with organic molecules having groups like thiols, amines, acids and disulfides. Its nanometer size and high molecular recognition properties add to the values. It is very complex to reproduce and easily prone to electrode fouling.
Electropolymerization
When the polymerization is carried out in the influence of an electric current, it is known as electropolymerization. It can be performed by two methods – one is to hold the film at a suitable oxidation potential of the monomer, and another is to use consecutive cyclic voltammetry under a suitable positive scan potential region. It is a flexible method as polymer thickness can be controlled and electrode fouling can be prevented. It can be utilized for the immobilization of a mediator as many polymers behave as mediators. It is limited only to the conducting substrates.
Different strategies to recuperate AOX/ADH-based biosensors
Different approaches have been developed regarding the construction of amperometric enzyme electrodes to facilitate the direct electron transfer process in alcohol detection and improving the performance and operational stability of the biosensors. The execution of amperometric biosensor is based on the measurement of current which is related to the transfer of electrons from the active site of the immobilized enzyme to the surface of the working electrode. Generally this electron transfer reaction at the time of catalysis of biological molecules is very slow at ordinary electrodes surface. The challenging aspect of these electrochemical biosensors is to alleviate the rate of electron transfer between the enzyme and the electrode surface. Great attention has been focused for solving this problem by some methods which include the use of mediator-modified enzymes, electrodes modified by membrane for electron transfer, by conducting or non-conducting-polymer matrices, by sol–gel-based supports, by hydrogel supports, by screen printed support or use of nanoparticles as electrode materials. Some of these strategies are reviewed and their applications to alcohol biosensors are described.
Membrane-based AOX/ADH biosensors
The immobilization of the enzyme onto a membrane is the most frequent mode of techniques used in the biosensors. Primarily, the use of enzyme electrode in biosensors was depicted by Clark [Citation54]. The performance of these biosensors stays unbeatable as a result of their simplicity and effortlessness of assembly. An enzyme can be inserted between the electrode and a membrane for immobilizing it in the easiest way. It has been observed that when a large amount of enzyme was loaded, enhanced results of a biosensor are usually attained. When an enzyme is cross-linked with a bifunctional reagent, higher enzyme loadings can be achieved. The mutual cross-linking of enzyme AOX with another enzyme like BSA or catalase results in the achievement of higher enzyme activity, excellent stability and reproducibility. The membranes can be used either as a supporting material for the immobilization itself or serve as a retention layer. Later, the enzyme is physically confined between the surface of electrode and the membrane. The encapsulation of enzyme AOX within a sol–gel utilizing tetraethoxysilane as gel precursor has also been accounted. Using cold plasma polymerisation method, ethylenediamine was used to modify polycarbonate membranes so that amino groups can be incorporated on their surface that will help in the covalent binding of enzyme with glutaraldehyde. Membranes behave as a protective layer because they prevent the electrochemically interfering compounds from accomplishing the surface of the electrode by the strategy of capturing them inside the polymeric matrices for example, polyacrylamide and gelatin [Citation19]. Nitrocellulose acetate and polycarbonate membranes have been exploited to serve as an efficient barrier to combat with the problem of interfering species contained in the beer samples. Immobilization of AOX inside a polyelectrolyte layer of Nafion (a perfluorinated ionomer), has likewise been accounted for which work as a barrier for negatively charged compounds, for example, ascorbate. The electropolymerised films which are non-conducting in nature can be effectively utilized for the designing of biosensors because of their particular selectivity for H2O2 over different compounds and they also act as a selective barrier for electroactive obstructions. At the surface of the electrode, small monomers can likewise be electropolymerised for their utilization in preparation of a membrane. Moreover, it can be used as an optional strategy for trapping the enzymes in a polymer matrix. AOX has been immobilized inside a film of 2-(4-aminophenyl)-ethylamine (AP-EA) and 2, 6-dihydroxynaphthalene (2, 6-DHN) [Citation55]. The electropolymerisation was performed through cyclic voltammetry within the sight of AOX and glutaraldehyde. This allows linking of the enzyme to the amino groups of the monomer AP-EA through covalent linkage. Later, o-phenylenediamine has likewise been electropolymerised to be used as a protective membrane. Instead of incorporating the enzyme within the membrane, the other method like directly immobilizing it onto the electrode surface using covalent coupling or adsorption has been reported.
Conducting polymer and non-conducting polymer-based AOX/ADH biosensors
The use of conducting polymers has widely drawn attention for the construction of biosensors. These electrically conducting polymers serve dual purpose of immobilization matrices as well as redox systems for transferring the electrical charge. So the attractive properties of conducting polymers result in superior electron transport capacity and sensitivity of biosensor. In biosensor applications, polypyrrole represents the most commonly used conducting polymer as it possess good chemical stability and biocompatibility alongwith the advantage of easy polymerization. It is environmental friendly. Polyvinylsulfonate (a porous polymer) is used as a support material for composite membranes and can enhance the mechanical strength and conductivity of PPy matrix [Citation56]. Recently in 2011, Serafin et al. fabricated a novel disposable enzyme biosensor for ethanol detection by electrodepositing a conducting polymer poly (3, 4-ethylenedioxythiophene) (PEDOT) and ADH in an ionic liquid medium onto gold nanoparticles-modified screen printed electrodes [Citation57]. A linear range between 5 and 100 μM was obtained for ethanol with a detection limit of 2 μM. In 2012, a novel conducting polymer of TBTD (4, 7-dithien-2-yl-2, 1, 3-benzothiadiazole) was used to construct an amperometric AOX biosensor. AOX was immobilized on the conducting polymers of PEDOT (3, 4-ethylenedioxythiophene) and TBTD. Using ethanol, the proposed biosensor was characterized, and sensitivity was found to be 203.70 nA/mM [Citation32]. In 2014, Çolak et al. constructed an amperometric ethanol biosensor with nicotinamide adenine dinucleotide (NAD+) and ADH on surface of a Pt/Ppy-PVS/MB electrode. The biosensor showed good reproducibility and storage stability [Citation58]. A new combination of the electroactive polymer poly(brilliant green) (PBG) or conducting polymer poly(3,4-ethylenedioxythiophene) (PEDOT) along with carbon nanotubes has been investigated by Barsan et al. for the detection of ethanol to obtain CNT/PBG and CNT/PEDOT-modified carbon film electrodes (CFE) incorporating the enzymes, AOX or ADH as test enzyme. While comparing these biosensors using AOX and ADH enzymes, AOX/CNT/PBG/CFE was found to give a higher sensitivity and a lower detection limit than ADH-based biosensor [Citation25]. Recently this year, a new robust platform GCE/SWCNT-polytyr(oxidized) has been reported for the construction of ADH-based ethanol biosensor. GCE/SWCNT-Polytyr(oxidized)/ADH/Naf offers excellent analytical characteristics and a successful application in quantification of ethanol in alcoholic beverages [Citation47]. An amperometric ethanol biosensor based on a non-conducting polymer poly (vinyl alcohol) was fabricated by Tsai et al. The biosensor was based on poly (vinyl alcohol)-multiwalled carbon nanotube-ADH biocomposite. It showed sensitivity of 196 nA mM−1 with a response time of 8 s. The linear range was found out to be 1.5 mM [Citation41]. Recently, a stable and sensitive amperometric biosensor was fabricated by using Polyamidoamine (PAMAM)/Cysteamine/AOX-Modified Gold Electrode for the detection of ethanol by Akin et al. The optimized biosensor showed a wide linearity from 0.025 to 1.0 mM and a detection limit of (LOD) 0.016 mM with 100 s response time [Citation59].
Sol–gel-based AOX/ADH biosensors
For the construction of ethanol biosensors, sol–gel matrices have also been utilized. These are the biocompatible porous structures formed by hydrolysis and co-condensation of sol–gel precursor like alkoxysilanes along with acid or base catalysis. The product form is a network of Si–O bond which provide space for immobilizing bioactive molecules. The sol–gel chemistry retains the natural conformation as well as reactivity of the enzyme intact. Sol–gel derived materials play a significant role in alleviating electron transfer process in biosensors. In the past few years, many sol–gel matrices-based ethanol enzyme biosensors have been extensively reported. Xu et al. reported a electrogenerated chemiluminescence biosensor which utilized ADH and tris(2,2′-bipyridyl)ruthenium (II) immobilized in sol–gel hybrid film for the detection of ethanol. The prepared biosensor showed a detection limit of 1.0 × 10−5 M and a wide linear range from 2.5 × 10−5 to 5.0 × 10−2 M [Citation45]. Recently, a novel amperometric alcohol biosensor was developed by Chinnadayyala et al. This third generation sensor was fabricated by immobilizing ferrocene entrapped alcohol oxidase (FcAOX) and horseradish peroxidase (HRP) coated with sol–gel chitosan film on a multi-walled carbon nanotube (MWCNT) modified glassy carbon electrode by layer-by-layer technique. The proposed biosensor showed a good linear range of 5.0 × 10−6 to 30 × 10−4 mol L−1 with sensitivity of 150 μA mM−1 cm−2 [Citation29].
Hydrogel matrices-based AOX/ADH biosensor
Hydrogels have become one of the idyllic enzyme-immobilization materials as they have a cross-linked network of polymers swollen with water. The biorecognition elements are embedded in these polymer networks. The enzymatic biosensors that use hydrogel as an encapsulant have shown high sensitivities over the other techniques of immobilization because a large number of hydroxyl groups present in the hydrogel offer a biocompatible microenvironment for retaining the natural configuration of the enzyme. Recently, SL Burrs reported a comparison of four different hydrogels which are utilized as protein encapsulants in the alcohol biosensor based on graphene-nanometal-enzyme composites. The enzyme AOX was encapsulated in four different hydrogels – chitosan, cellulose nanocrystals (CNC), poly-N-isopropylacrylamide (PNIPAAM) or silk fibroin hydrogels, and then spin coated onto a nanoplatinum-graphene-modified electrode [Citation60]. Chitosan and PNIPAAM showed the higher sensitivity (0.46 ± 0.2 and 0.3 ± 0.1 μA mM−1, respectively) as well as the faster response time (4.3 ± 0.8 and 4.8 ± 1.1 s, respectively) over silk and CNC hydrogels (0.09 ± 0.02 and 0.15 ± 0.03 μA mM−1, respectively. CNC and silk hydrogels showed longer response time (8.9 ± 2.1 and 6.3 ± 0.8 s, respectively). The transport of silk, PNIPAAM and chitosan hydrogels was excellent due to the high porosity of these gels which makes them significantly better than CNC bionanocomposites. The use of hydrogel matrices is beneficial as it requires only one enzyme, all the materials are non-toxic, mediators/cofactors are not required and there is improved shelf life of the biosensor.
Screen printed electrode (SPE)-based ethanol biosensors
The growing need of developing analytical methods to perform rapid “in situ” analyses of ethanol led to the emergence of miniaturized disposable screen printed electrodes (SPE). The manufacturing of SPEs involves the deposition of multiple successive layers of different ink onto the planar ceramic or plastic supports. The composition of the inks which are used in printing process can be modified by adding different substances like enzymes, complexing agents and metals etc. SPEs present great flexibility for their use in biosensors as transducers because they can be easily modified by using nanostructures, mediators or conducting polymers to improve electron transfer rate. They are easy to prepare as well as suitable for working with micro-volumes. In addition, SPEs show excellent selectivity, reusability and sensitivity. Ramaa et al. studied different alcohol biosensors based on screen-printed carbon electrodes and compared them. They developed single-use alcohol enzyme sensors by utilizing AOX from 3 different yeasts namely Candida boidinii, Hansenula sp. and Pichia pastoris and using 3 different mediators based Screen-printed carbon Electrodes as transducers. The mediators such as Ferrocyanide, Prussian blue and Co-phthalocyanine were incorporated into the ink of the working electrode. The best results were shown by biosensor using Screen-Printed Co-phthalocyanine/Carbon Electrode and enzyme AOX purified from Hansenula sp. It showed a wide linear response of 0.05–1.00 mM and sensitivity of around 1211 nA mM−1 [Citation20]. Boujtita et al. developed a disposable ethanol sensor based on a chemically altered screen-printed carbon electrode coated with AOX for the measurement of ethanol in beer [Citation61]. Then Luo et al. reported a biosensor for detecting serum alcohol concentration. They fabricated the biosensor by cross-linking the enzyme AOX and NAD+ on the screen printed electrode modified with Meldola's Blue (MB) mediator absorbed on Nafion. The biosensor showed satisfactory results for the measurement of serum alcohol [Citation62]. Later, Erhui Hua reported an integrated disposable ethanol biosensor based on the ADH/NAD+/Meldola’s blue (MDB)/graphitized mesoporous carbons (GMCs)/chitosan nanobiocomposite fabricated on a screen printed electrode (SPE). It presented excellent sensitivity of 10.36 nA μM−1 with fast response time of 5 s [Citation33]. Recently in 2016, an ethanol biosensor was developed by Melike et al. by modifying screen printed carbon electrode with gold nanoparticles (AuNPs), polyneutral red (PNR), multiwalled carbon nanotubes (MWCNTs) and ADH enzyme. This biosensor was tested on real alcoholic beverage samples. The sensitivity and limit of detection of the proposed sensor was found to be 0.49 μA mM−1 and 96.1 μM, respectively [Citation63].
Nanoparticles (NPs)-based ethanol biosensors
Nanoparticles-based ethanol biosensors have gained considerable recognition in a short period of time as they have many advantages in terms of promoting the catalytic reduction of redox species and inhibiting the oxidation of interfering species. NPs-based biosensors have attracted great attention due to their unique characteristics like high catalytic efficiency, large surface-to-volume ratio, strong adsorption ability and quick response time. In addition, nanoparticles have electroactive surface of electrode which results in enhancing the rate of electron transport between the electrolyte medium and the electrode. The use of carbon nanotubes (CNT) – single-walled (SWCNTs) and multi-walled (MWCNTs), zinc oxide nanostructures (ZnO), Pt nanoparticles and gold nanoparticles (AuNPs) has been extensively used in fabricating biosensors for ethanol detection. Application of superparamagnetic nanoparticles and mesoporous materials as matrices for the firm immobilization of enzyme has proved to a distinction due to their high surface to volume ratio [Citation64]. Liao et al. reported a disposable amperometric ethanol biosensor based on the screen-printed carbon electrodes mediated with ferricyanide-magnetic nanoparticle mixture. It showed wide linear range of 1.0 to 9.0 mM with fast response time [Citation65]. Later, Jia et al. fabricated an electrogenerated chemiluminescence ethanol biosensor which was based on the ADH functionalized Ru(bpy)32+ doped silica nanoparticles. The biosensor exhibited satisfactory performance with a low detection limit of 5.0 × 10−8 M and a wide linear range of 10−7 to 10−2 M [Citation66]. Gómez-Anquela et al. developed a high performance biosensor for the rapid detection of ethanol in wine by using thiotic acid-capped gold nanoparticles coordinated with ADH and Azure A film electrografted on screen-printed carbon electrodes. The sensor showed excellent analytical performance with a detection limit of 0.14 ± 0.01 μM and storage stability of about one month [Citation67]. In 2016, recently a novel ethanol biosensor is proposed by Ozdokur et al. based on the catalytic effect of MnOx–MoOx mixed oxide electrode decorated with Pt nanoparticles. The proposed whole cell biosensor was constructed using this film of electroactive metal for the determination of ethanol. The sensor exhibited a wide linear range of 0.075–5.0 mM with a fast response time of 63 s. Excellent results were obtained in comparison to the conventional methods [Citation68].
Role of mediators
In biosensors, the electrochemical detection of NADH requires high overpotentials but at this potential a large number of other species present in the sample also get oxidized and interfere with the measurements. Many efforts have been devoted to overcome this drawback and develop new efficient electrode materials. In addition, the fundamental requirement of high efficiency in the direct electrode transfer between enzyme and the electrode and led to the improvement of enzyme mediators. Mediator is basically a low molecular weight artificial electroactive species that works as an electron transferring agent by acting as a shuttle or a bridge between the redox center of the enzyme and the surface of the indicator electrode. During the catalytic process, the mediator is first reduced to Mred by the cofactor and then oxidized back into Mox when it comes into contact with the anode, which is polarized at the appropriate potential. The amperometric measurement of the rate of production of reduced mediator (Mred) is done by oxidation at the electrode. There is a large number of advantages of using mediators such as low dependence of measurements on oxygen concentration, less interference of unwanted species, prevention of electrode fouling, determination of working potential of the enzyme electrode by the oxidation potential of mediator as well as rapid rates of electron transfer. The disadvantage of most mediators includes their toxicity and solubility in an aqueous solution. Some of the characteristics of an ideal mediator are (i) During the electron transfer, it should not participate in side reactions. (ii) It should react rapidly with the reduced enzyme. (iii) It should be stable under required working conditions. (iv) It should show reversible heterogeneous kinetics. (v) It should possess stable reduced and oxidized forms. (vi) The reduced form of mediator should not react with oxygen. (vii) It should have a lower redox potential than other interfering electroactive species present in the sample. To study the properties of a mediator, a technique called direct current voltammetry is used. This technique is very useful in selecting an appropriate mediator for an amperometric biosensor. The role of a mediator has been shown schematically in .
There are conducting polymers, transition metal compounds and organic dyes-based mediators which are used in alcohol biosensors. Some conducting polymer-based mediators used in alcohol biosensing include polyaniline, poly(thionine) [Citation31], poly(o-aminophenol)- and poly(o-phenylenediamine), polyethylene glycol, poly(vinyl alcohol), polytyrosine and poly(3,4-ethylenedioxythiophene) (PEDOT). The immobilization of mediators like quinines [Citation33], phenoxazines, phenylendiamines, oil-soluble mediators based on phenothiazinium chloride, ferrocene, conducting charge transfer complexes and organic dyes like Meldola blue [Citation2], Prussian blue [Citation19] & poly (brilliant green) [Citation25] has been extensively studied.
Future prospectives
Application of enzyme biosensors has acquired paramount importance in many areas of food safety standards, environmental monitoring, biomedicine and security assessment. Imperative utilization of enzyme biosensors will lead to the designing of more competent regenerative biosensors to widen the spectra of analysis of complex real samples in future. It is a challenge to simultaneously providing researchers more tools for understanding the effects of alcohol as well as to put such valuable data in the hands of the consumer. The development of sleek, unobtrusive, non-invasive and economic wearable biosensors that more accurately detect alcohol concentration in the blood or other bodily fluids such as saliva or sweat in real-time will be of value in research medical applications. Next level of development should aim for discovering the device that would be able to quantitate blood alcohol level, interpret the data, and transmit or store data to a smartphone or other visual portable device through the wireless transmission. Although these necessities have yet to be fully realized, latest researches in microfabrication may allow us to achieve success in field of sensors with the high spatial sensitivity. The use of non-invasive technologies to devices that measure alcohol concentration in the blood, other bodily fluids will be given highest priority as opposed to detection of alcohol exuded through the skin in sweat. This challenge seeks innovative solutions in non-invasive and disposable wearable forms that quantify in vivo alcohol through the invention, or the adaptation and miniaturization, of technologies such as (but not limited to) spectroscopy or wave technology. Innovation should be encouraged!
Disclosure statement
The authors report no conflicts of interest. The authors alone are responsible for the content and writing of this article.
References
- Santos AS, Pereira AC, Durán N, et al. Amperometric biosensor for ethanol based on co-immobilization of alcohol dehydrogenase and Meldola’s Blue on multi-wall carbon nanotube. Electrochim Acta. 2006;52:215–220.
- Santos AS, Freire RS, Kubota LT. Highly stable amperometric biosensor for ethanol based on Meldola’s blue adsorbed on silica gel modified with niobium oxide. J Electroanal Chem. 2003;547:135–142.
- Niculescu M, Erichsen T, Sukharev V, et al. Quinohemoprotein alcohol dehydrogenase-based reagentless amperometric biosensor for ethanol monitoring during wine fermentation. Anal Chim Acta. 2010;463:39–51.
- Kochana J, Adamski J. Detection of NADH and ethanol at a graphite electrode modified with titania sol-gel/Meldola’s Blue/MWCNT/Nafion nanocomposite film. Cent Eur J Chem. 2012;10:224–231.
- Tangerman A. Highly sensitive gas chromatographic analysis of ethanol in whole blood, serum, urine, and fecal supernatants by the direct injection method. Clin Chem. 1997;43:1003–1009.
- McCarver-May DG, Durisin L. An accurate, automated, simultaneous gas chromatographic headspace measurement of whole blood ethanol and acetaldehyde for human in vivo studies. J Anal Toxicol. 1997;21:134–141.
- Mendes LS, Oliveira FCC, Suarez PAZ, et al. Determination of ethanol in fuel ethanol and beverages by Fourier transform (FT)-near infrared and FT-Raman spectrometry. Anal Chim Acta. 2003;493:219–231.
- Tarnowski DJ, Korzeniewski C. Amperometric detection with membrane-based sampling for percent-level determinations of ethanol. Anal Chim Acta. 1996;332:111–121.
- Tipparat P, Lapanantnoppakhun S, Jakmunee J, et al. Determination of ethanol in liquor by near-infrared spectrophotometry with flow injection. Talanta. 2001;53:1199–1204.
- Vonach R, Lendl B, Kellner R. High-performance liquid chromatography with real-time Fourier-transform infrared detection for the determination of carbohydrates, alcohols and organic acids in wines. J Chromatogr A. 1998;824:159–167.
- Mattos IL, Sartini RP, Zagatto EAG, et al. Spectrophotometric flow injection determination of ethanol in distilled spirits and wines involving permeation through a silicon tubular membrane. Anal Sci. 1998;14:1005–1008.
- Svensson K, Bülow L, Kriz D, et al. Investigation and evaluation of a method for determination of ethanol with the SIRE Biosensor P100, using alcohol dehydrogenase as recognition element. Biosens Bioelectron. 2005;21:705–711.
- Pereira AC, Aguiar MR, Kisner A, et al. Amperometric biosensor for lactate based on lactate dehydrogenase and Meldola Blue coimmobilized on multi-wall carbon nanotube. Sens Actuat B. 2007;124:269–276.
- Guzman-Vazquez de Prada A, Pena N, Parrado C, et al. Amperometric multidetection with composite enzyme electrodes. Talanta. 2004;62:896.
- Vonck J, Van Bruggen EF. Electron microscopy and image analysis of two-dimensional crystals and single molecules of alcohol oxidase from Hansenula polymorpha. Biochim Biophys Acta. 1990;1038:74–79.
- Koyuncu D, Erden PE, Pekyardımcı S, ¸, et al. A new amperometric carbon paste enzyme electrode for ethanol determination. Anal Lett. 2007;40:1904–1922.
- Lau RCW, Choi MHF, Lu JZ. Alcohol sensing membrane based on immobilized ruthenium(II) complex in carboxylated PVC and surface covalently bonded alcohol oxidase. Talanta. 1999;48:321–331.
- Gahlaut A, Gothwal A, Chhillar AK, et al. Electrochemical based diagnosis of ascorbic acid. Sens Transducers. 2012;143:143–151.
- Karyakin AA, Karyakina EE, Gordon L. Prussian-Blue-based amperometric biosensors in flow-injection analysis. Talanta. 1996;43:1597–1606.
- Ramaa EC, Biscaya J, Garcíaa MBG, et al. Comparative study of different alcohol sensors based on Screen-Printed Carbon Electrodes. Anal Chim Acta. 2012;728:69–76.
- Ghica ME, Brett CMA. Development of a carbon film electrode ferocene-mediated glucose biosensor. Anal Lett. 2005;38:907–920.
- Sehlotho N, Griveau S, Ruillé N, et al. Electro-catalyzed oxidation of reduced glutathione and 2-mercaptoethanol by cobalt phthalocyanine-containing screen printed graphite electrodes. Mater Sci Eng C. 2008;28:606–612.
- Kuswandi B, Irmawati T, Hidayat MA, et al. A simple visual ethanol biosensor based on alcohol oxidase immobilised onto polyaniline film for halal verification of fermented beverage samples. Sensors. 2014;14:2135–2149.
- Gahlaut A, Dahiya M, Dhull R, et al. Fabrication of Nafion/HRP-SWCNT/MWCNT-ZnO based alcohol biosensor: diagnostics for forensic analysis. Int J Chem Anal Sci. 2014;5:15–20.
- Barsan MM, Brett CMA. An alcohol oxidase biosensor using PNR redox mediator at carbon film electrode. Departamento de Química, Universidade de Coimbra, 3004-535 Coimbra, Portugal. Talanta. 2008;74:1505–1510.
- Gorton L, Csöregi E, Domínguez, et al. Selective detection in flow analysis based on the combination of immobilsed enzymes and chemically modified electrodes. Anal Chim Acta. 1991;250:203–248.
- Hämmerle M, Hilgert K, Horn MA, et al. Analysis of volatile alcohols in apple juices by an electrochemical biosensor measuring in the headspace above the liquid. Sens Actuat B Chem. 2011;158:313–318.
- Wen G, Zhang Y, Shuang S, et al. Application of a biosensor for monitoring of ethanol. Biosens Bioelectron. 2007;23:121–129.
- Chinnadayyala SR, Kakoti A, Santhosh M, et al. A novel amperometric alcohol biosensor developed in a 3rd generation bioelectrode platform using peroxidase coupled ferrocene activated alcohol oxidase as biorecognition system. Biosens Bioelectron. 2014;55:120–126.
- Das M, Barbora L, Das P, et al. Biofuel cell for generating power from methanol substrate using alcohol oxidase bioanode and air-breathed laccase biocathode. Biosens Bioelectron. 2014;59:184–191.
- Morales A, Céspedes F, Martínez-Fàbregas E, et al. Ethanol amperometric biosensor based on an alcohol oxidase–graphite–polymer biocomposite. Electrochim Acta. 1998;43:3575–3579.
- Tanriverdi S, Tuncagil S, Toppare L. A new amperometric alcohol oxidase biosensor based on conducting polymer of (4, 7-dithien-2-yl-2, 1, 3-benzothiadiazole). J Macromol Sci. 2012;49:185–190.
- Hua E, Wang L, Jing X, et al. One-step fabrication of integrated disposable biosensor based on ADH/NAD+/meldola’s blue/graphitized mesoporous carbons/chitosan nanobiocomposite for ethanol detection. Talanta. 2013;111:163–169.
- Salimi F, Negahdary M, Mazaheri G, et al. A novel alcohol biosensor based on alcohol dehydrogenase and modified electrode with ZrO2 nanoparticles. Int J Electrochem Sci. 2012;7:7225–7234.
- Chi QJ, Dong SJ. A comparison of electrocatalytic ability of various mediators adsorbed onto paraffin impregnated graphite electrodes for oxidation of reduced nicotinamide coenzymes. J Mol Catal A. 1996;105:193–201.
- Jaegfeldt H, Torstensson ABC, Gorton L, et al. Catalytic oxidation of reduced nicotinamide adenine dinucleotide by graphite electrodes modified with adsorbed aromatics containing catechol functionalities. Anal Chem. 1981;53:1979–1982.
- Hajizadeh K, Tang HT, Halsall HB, et al. Chemical cross-linking of a redox mediator thionin for electrocatalytic oxidation of reduced β-nicotinamide adenine dinucluotide. Anal Lett. 1991;24:1453–1469.
- Lobo MJ, Mirande AJ, Fonseca JML, et al. Electrocatalytic detection of nicotinamide coenzymes by poly(o-aminophenol)- and poly(o-phenylenediamine)-modified carbon paste electrodes. Anal Chim Acta. 1996;325:33–42.
- Yao Q, Yabuki A, Mizutani F. Preparation of a carbon paste/alcohol dehydrogenase electrode using polyethylene glycol-modified enzyme and oil-soluble mediator. Sens Actuat B. 2000;65:147–149.
- Zhen S, Wang Y, Liu C, et al. A novel microassay for measuring blood alcohol concentration using a disposable biosensor strip. Forensic Sci Int. 2011;207:177–182.
- Tsai YC, Huang JD, Chiu CC. Amperometric ethanol biosensor based on poly (vinyl alcohol)–multiwalled carbon nanotube–alcohol dehydrogenase biocomposite. Biosens Bioelectron. 2007;22:3051–3056.
- Svensson K, Bülow L, Kriz D, et al. Investigation and evaluation of a method for determination of ethanol with the SIRE Biosensor P100, using alcohol dehydrogenase as recognition element. Biosens Bioelectron. 2005;21:705–711.
- Alpat S, Telefoncu A. Development of an alcohol dehydrogenase biosensor for ethanol determination with toluidine blue O covalently attached to a cellulose acetate modified electrode. Sensors (Basel). 2010;10:748–764.
- Tian F, Zhu G. Toluidine blue modified self-assembled silica gel coated gold electrode as biosensor for NADH. Sens. Actuat B. 2004;97:103–108.
- Xu Z, Guo Z, Dong S. Electrogenerated chemiluminescence biosensor with alcohol dehydrogenase and tris(2,2'-bipyridyl)ruthenium (II) immobilized in sol-gel hybrid material. Biosens Bioelectron. 2005;21:455–461.
- Eguilaz M, Gutierrez F, González-Domínguez JM, et al. Single-walled carbon nanotubes covalently functionalized with polytyrosine: A new material for the development of NADH-based biosensors. Biosens Bioelectron. 2016;86:308–314.
- Zhu Z, Garcia-Gancedo L, Flewitt AJ, et al. A critical review of glucose biosensors based on carbon nanomaterials: carbon nanotubes and graphene. Sensors. 2012;12:5996–6022.
- Antiochia R, Lavagnini I. Alcohol biosensor based on the immobilization of meldola blue and alcohol dehydrogenase into a carbon nanotube paste electrode. Anal Lett. 2006;39:1643–1655.
- Wang J, Musameh M. A reagentless amperometric alcohol biosensor based on carbon-nanotube/teflon composite electrodes. Anal Lett. 2003;36:2041–2048.
- Pingarròn JM, Yáñez-Sedeño P, González-Cortés A. Gold nanoparticle-based electrochemical biosensors. Electrochim Acta. 2008;53:5848–5866.
- Rothwell SA, Killoran SJ, O'Neill RD. Enzyme immobilization strategies and electropolymerization conditions to control sensitivity and selectivity parameters of a polymer-enzyme composite glucose biosensor. Sensors. 2010;10:6439–6462.
- Sassolas A, Blum LJ, Leca-Bouvier BD. Immobilization strategies to develop enzymatic biosensors. Biotechnol Adv. 2012;30:489–511.
- Narváez A, Suárez G, et al. Reagentless biosensors based on self-deposited redox polyelectrolyteoxidoreductases architectures. Biosens Bioelectron. 2000;15:43–52.
- Clark LC, Lyons C. Electrode systems for continuous monitoring in cardiovascular surgery. Ann N Y Acad Sci. 1962;102:29
- Lubrano GJ, Faridnia MH, Palleschi G, et al. Amperometric alcohol electrode with extended linearity and reduced interferences. Anal Biochem. 1991;198:97–103.
- Çolak O, Yaşar A, Cete S, et al. Glucose biosensor based on the immobilization of glucose oxidase on electrochemically synthesized polypyrrole-poly(vinyl sulphonate) composite film by cross-linking with glutaraldehyde. Artif Cells Nanomed Biotechnol. 2012;40:354–361.
- Serafin V, Agüí L, Yáñez-Sedeño p, et al. A novel hybrid platform for the preparation of disposable enzyme biosensors based on poly(3,4-ethylenedioxythiophene) electrodeposition in an ionic liquid medium onto gold nanoparticles-modified screen-printed electrodes. J Electroanal Chem. 2011;656:152–158.
- Çolak O, Arslan F. Amperometric biosensing of ethanol based on integration of alcohol dehydrogenase with a Pt/PPy–PVS/MB electrode. Turk J Chem. 2015;39:84–95.
- Akin M, Yuksel M, Geyik C, et al. Alcohol biosensing by polyamidoamine (PAMAM)/cysteamine/alcohol oxidase-modified gold electrode. Biotechnol Progress. 2010;26:896–906.
- Burrs SL, Vanegas DC, Rong Y, et al. A comparative study of graphene-hydrogel hybrid bionanocomposites for biosensing. Analyst. 2015;140:1466–1476.
- Boujtita M, Hart JP, Pittson R. Development of a disposable ethanol biosensor based on a chemically modified screen-printed electrode coated with alcohol oxidase for the analysis of beer. Biosensor Bioelectron. 2000;15:257–263.
- Luo P, Xie G, Liu Y, et al. Electrochemical detection of blood alcohol concentration using a disposable biosensor based on screen-printed electrode modified with Nafion and gold nanoparticles. Clin Chem Lab Med. 2008;46:1641–1647.
- Melike B, Erol A. Biosensor application of screen-printed carbon electrodes modified with nanomaterials and a conducting polymer: Ethanol biosensors based on alcohol dehydrogenase. Sens Actuat B Chem. 2016;6:164.
- Gupta MN, Kaloti M, Kapoor M, et al. Nanomaterials as matrices for enzyme immobilization. Artif Cells Blood Substit Immobil Biotechnol. 2011;39:98–109.
- Liao MH, Guo JC, Chen WC. A disposable amperometric ethanol biosensor based on screen-printed carbon electrodes mediated with ferricyanide-magnetic nanoparticle mixture. J Magn Magn Mater. 2006;304:421–423.
- Jia TT, Cai ZM, Chen XM. Electrogenerated chemiluminescence ethanol biosensor based on alcohol dehydrogenase functionalized Ru(bpy)3(2+) doped silica nanoparticles. Biosens Bioelectron. 2009;25:263–267.
- Gómez-Anquela C, García-Mendiola T, Abad JM, et al. Scaffold electrodes based on thioctic acid-capped gold nanoparticles coordinated alcohol dehydrogenase and Azure A films for high performance biosensor. Bioelectrochemistry. 2015;106:335–342.
- Ozdokur KV, Demir B, Atman E, et al. A novel ethanol biosensor on pulsed deposited MnOx-MoOx electrode decorated with Pt nanoparticles. Sens Actuators B Chem. 2016;237:291–297.