Abstract
Hyaluronic acid (HA) has inherent ability to target the CD44 receptors and internalize into tumour cells via receptor-mediated endocytosis. Therefore, conjugation of this natural linear polysaccharide to polymeric NPs or micelles, as one of the most promising approaches, could be useful for future clinical applications such as drug delivery. Accordingly, we report on the synthesis of mitoxantrone (MTX)-conjugated polymeric nanoparticles (NPs) composed of polyethylene glycol-HA (PEG-HA) for MTX delivery toward special tumour cells. To determine the size of the polymeric NPs, field emission scanning electron microscopy (FESEM) and particle size analyzer system Zetasizer_nanoZS were employed. The in vitro cytotoxicity analysis of MTX-loaded HA-PEG NPs and free MTX against two cell lines with different levels of CD44 expression (MDA-MB-231 (very high) and MCF-7 (low) was conducted by MTT assay. Also, computational molecular docking was employed to study in detail the active site residues and the critical interactions between HA-EDA-PEG-EDA-MTX NPs and CD44 receptor. The particle size analysis and electron microscopy showed the average size of polymeric NPs less than 350 nm. FT-IR spectrophotometry analysis and also NMR confirmed the conjugation of HA and MTX onto the PEG. Cytotoxicity assay revealed that the engineered polymeric NPs were able to specifically bind to and significantly inhibit the CD44 receptor-positive MDA-MB-231 cells, but not the CD44-negative MCF-7 cells. Furthermore, analysis of the binding modes revealed that for the best-docked pose nearly 10 conventional hydrogen bond can occur between the MTX-EDA-PEG-EDA-HA NPs and amino acids of CD44 receptor. Based on these findings, we suggest the HA-PEG-MTX NPs as an effective functional-targeted nanomedicine toward therapy of CD44-positive cancers.
Introduction
Mitoxantrone (MTX), one of the anthraquinone derivatives, is commonly used in the treatment of broad spectrum of tumours such as metastatic breast cancer and prostate cancer as well as acute leukemia, and lymphomas [Citation1]. It also acts as an immunomodulatory agent for reducing the neurological disorder of relapsing-remitting multiple sclerosis (MS) [Citation2]. The mechanism of action of the MTX as an apoptosis agent is inhibition of the mitochondrial pathway plus inhibition of topoisomerase II and single- and double-strand breaks in DNA [Citation3]. Nevertheless, inadvertent side effects such as cardiotoxicity pose major obstacles for use MTX in cancers treatment [Citation4]. So, a major approach which could be useful for decreasing the side effects is specific drug delivery via polymeric nanoparticles (NPs). These nano-sized vehicles will demonstrate the combined advantages of high drug loading, specific and efficient cellular uptake, long circulation time and good sustained release [Citation5].
On one hand, one of the shielding polymers with high water solubility which could passively accumulate in tumour cells is polyethylene glycol (PEG). As a consequence, PEG can be stable in biological fluids to avoid reticuloendothelial system (RES) clearance followed by elimination from the body. This biocompatible polymer could localize in the tumours due to enhanced permeability and retention (EPR) effect, therefore, achieving slow clearance, extended-interval dosing and less toxicity of the carried drugs [Citation6]. On the other hand, polymeric NPs can be activated for further functionalization with targeting and/or therapy agents [Citation4,Citation7,Citation8].
The active targeting of NPs to cancer cells via receptor-mediated endocytosis mechanism can be achieved by conjugation of targeting moiety. These targeting ligands not only recognize the specific high-expressed receptors but also decrease inadvertent side effects [Citation9]. In our previous works, we had evaluated the role of folate receptors (FRs) in tumour-targeted drug delivery. All the studies have confirmed that folic acid and also methotrexate significantly promoted specific targeting of folate high expressed tumour cells [Citation4,Citation10,Citation11].
But in this study, we used hyaluronic acid (HA), which is of best ligands in targeted drug delivery, for specific targeting of cancer cells by NPs. In recent years, this endogenous anionic polysaccharide has received growing scientific attention because of possessing CD44 receptor targeting potential. It is composed of alternating residues of glucuronic acid and N-acetyl-D-glucosamine which can bind to glycoprotein CD44 receptors through the hydrogen binding between carboxyl groups of HA and the amino group of CD44 receptors [Citation9,Citation12]. The CD44 receptors which are abundantly overexpressed on different types of cancer cells such as fibrosarcomas, breast cancer, mesotheliomas and prostate cancer, have molecular weight nearly 85–250 kDa. The CD44 activation by HA is associated with some signaling pathways, e.g. tumour growth, angiogenesis, progression and metastasis [Citation13]. But, the biocompatibility and biodegradability of HA as well as abundance of functional groups for more modification such as COOH and OH could be useful for NPs to improve the efficiency of anti-cancer drugs [Citation14]. An approach that could be useful for predicting the orientation of the CD44–ligand interactions is molecular docking method. By using the knowledge gained from the docking study, the interaction between CD44 and its native carbohydrate ligand, HA, could be assessed. However, our knowledge of interactions and structure of human CD44 is limited which might be due to the difficulty of purifying the samples, the flexibility of the structure and the membrane environment [Citation15]. But, it is conceivable that by molecular docking study on the MTX-EDA-PEG-EDA-HA NPs and HA, the changes caused by conjugation to HA be anticipated and the interaction between synthetic compound and CD44’s active site be simulated. Accordingly, we report the synthesis of polymeric NPs which could demonstrate the combined advantages of polyethylene glycol and HA to elevate the MTX efficacy in cancer chemotherapy.
Material and methods
HA and protease were purchased from Sigma Aldrich (Schnelldof, Germany) and dialysis bag (MWCO = 10 kD) was purchased from Sigma-Aldrich (St. Louis, MO). Polyethylene glycol (PEG, Mw 4000), 4-toluenesulphonyl chloride (tosyl chloride or TsCl), N-hydroxysuccinimide (NHS), dimethylformamide (DMF), 3-(4,5-dimethyl-2-thiazolyl)-2,5-diphenyl-2H-tetrazolium bromide (MTT) and formamide were purchased from Merck (Darmstadt, Germany). Ethylenediamine (EDA), triethylamine (TEA) and 1,1′-carbonyldiimidazole (CDI) were obtained from Merck (Schuchardt, Germany). MTX was obtained from EBEWE Pharma (Holzkirchen, Germany). N,N'-Dicyclohexylcarbodiimide (DCC) was purchased from Alfa Aesar (Karlsruhe, Germany). MDA-MB-231 and MCF-7 cell lines were obtained from the Pasteur Institute Cell Bank (Tehran, Iran). RPMI 1640, penicillin G (10,000 units/ml)–streptomycin (10,000 μg/mL), trypsin 0.25% and phosphate-buffered saline (PBS) were purchased from Caisson labs (North Logan, UT). Foetal bovin serum (FBS) was purchase from Gibco (Carlsbad, CA).
Synthesis of polyethylene glycol ditosylate (PEG-diTS)
PEG (10 g, 2.5 mmol) was dissolved in 10 ml anhydrous dichloromethane which previously was dried with magnesium sulphate. Then, tosyl chloride (1.43 g, 7.5 mmol) and TEA (0.7 ml, 5 mmol) were added to the reaction mixture and stirring was continued for 24 h at room temperature. Thereupon, the white PEG-diTS was precipitated in diethyl ether. For washing, product was dissolved in saturated NaCl solution (35% w/v) and was extracted with dichloromethane and reprecipitated in diethyl ether. The yield was 88%.
Synthesis of bis(ethylenediamine) polyethylene glycol
PEG-ditosylate (4.3 g, 1 mmol) was dissolved in 5 ml of anhydrous dichloromethane and was reacted with five equivalents of EDA (0.33 ml). The mixture was stirred overnight and then product was precipitated in diethyl ether and was vacuum-rotary evaporated to dryness. The yield was 54%. (product 3) schematically depicts the synthesis of Bis(EDA)-PEG.
Conjugation of MTX to bis(EDA)-PEG
In the first step, bis(EDA)-PEG (184 mg, 0.045 mmol) was dissolved in DMF and then CDI (8 mg, 0.05 mmol) and TEA (6 μL, 0.045 mmol) was added to the reaction mixture and stirred for 1 h under argon blanket at RT. Thereupon, the product was precipitated by cool diethyl ether and was vacuum-rotary evaporated to dryness. Finally, carbonyl imidazole-mediated was redissolved in 3 ml of DMSO for storage at 4 °C.
In the second step of this synthesis approach, MTX (20 mg, 0.045 mmol) separately was dissolved in 3 ml of DMSO and was added dropwise to the solution of previous step over 2 h. Reaction was continued overnight by stirring at RT under argon blanket. After proper time, the dark blue products (MTX-EDA-PEG-EDA) were precipitated by diethyl ether and were vacuum-rotary evaporated to dryness. (product 4) schematically represents the fabrication of the MTX-EDA-PEG-EDA.
Conjugation of HA to MTX-bis(EDA)-PEG
HA (18 mg, 0.045 mmol, equal to the number of moles of carboxyl groups) was dissolved in the mixture of 5 ml of formamide and 1 ml DMF, then temperature was raised gently to 70 °C. DCC (28 mg, 0.135 mmol) and NHS (15.5 mg, 0.135 mmol) were added to the reaction mixture and stirring was continued at this temperature for 5 h to activate –COOH of HA. Thereupon, MTX-conjugated PEG which produced in previous step and 12.5 μL of TEA were dissolved in 2 ml of DMF and were added to the reaction mixture and stirred overnight at 70 °C. Finally, the resulting solution was dialyzed against deionized water for 3 d followed by lyophilization (, product 5).
Characterization of synthesized products
To determine the size of the MTX-EDA-PEG-EDA-HA NPs, field emission scanning electron microscopy (FESEM) (Mira 3-XMU, Brno, Czech Republic) and particle size analyzer system Zetasizer_nanoZS (Malvern, England) were employed. All the steps of synthesis were validated by FTIR spectroscopy using Shimadzu IR PRESTIGE 21 spectrophotometer (Shimadzu Scientific Instruments, Tokyo, Japan). NMR analysis of final product (MTX-EDA-PEG-EDA-HA) was taken up in D2O by a BRUKER 300 MHz AVANCE III (Bruker, Rheinstetten, Germany) instrument.
Drug-loading and drug-release assays
The loading efficiency of MTX on polymeric NPs was determined by UV spectrophotometry (CECIL, CE1021) at 608 nm where the line equation of calibration curve for six standard concentrations of MTX (0.022, 0.011, 0.0055, 0.0028, 0.0014, and 0.0007 mM) was y = 15.779x + 0.0357 (R2 = 0.9996).
Release profiles of MTX from MTX-EDA-PEG-EDA-HA NPs were determined in pH 7.4 and also pH 5 containing 1.5 mg/mL of pure protease. For this purpose, 1.0 mg/mL of prepared NPs were sealed at 37 °C into a dialysis bag (MWCO = 10 KD) and placed in 40 ml of mentioned buffers for 72 h. Also, for the control, release of free MTX was done in the same conditions.
Cytotoxicity test
The in vitro cytotoxicity analysis of MTX-EDA-PEG-EDA-HA NPs and free MTX against two cell lines with different levels of CD44 expression (MDA-MB-231 (very high) and MCF-7 (low)) [Citation16,Citation17] was conducted by MTT assay. The cells were cultured in RPMI 1640 media supplemented with 10% FBS and 100 μL of penicillin G/streptomycin mixture at 37 °C in a humidified CO2 incubator [Citation18,Citation19]. Cell passaging was done by trypsinization. Then, 200 μL of the suspension of cultured cells at a density of 1 × 104 cells/well were transferred onto each well of 96-well culture plates and incubated for 24 h. Thereupon, the cells were treated and incubated for 48 and 72 h with different concentrations (i.e. ranging from 25 to 400 μM) of MTX-EDA-PEG-EDA-HA NPs and free MTX, when the concentration of MTX-EDA-PEG-EDA-HA NPs was set to be equivalent to the free MTX. At appropriate times, 200 μL of MTT solutions composed of 150 μL of fresh media and 50 μL MTT were added to each well and incubated for 4 h. MTT was aspirated off and the formazan crystals of each well were solubilized in 200 μL of DMSO and 25 μL Sorenson’s buffer followed by reading the absorbance at 570 nm using a spectrophotometer (BioTek Instruments, Inc., Bad Friedrichshall, Germany).
Molecular docking study
The crystal structure of CD44, as the receptor, was taken from the protein data bank with PDB ID 1POZ [Citation20]. The schematic structures of HA and MTX-EDA-PEG-EDA-HA NPs, as the ligands, were drawn in accordance with the references by ChemDraw Pro 15.0 software (Cambridge Soft Corporation, Cambridge, MA). After minimization of HA and MTX-EDA-PEG-EDA-HA NPs structures, molecular docking was performed with Smina software (ESI Software, München, Germany) at the default AutoDock Vina scoring function consists of three steric terms, a hydrogen bond term, a hydrophobic term and a torsion count factor [Citation19,Citation21]. Besides the default scoring function, it possesses an assortment of simple property counts includes electrostatic term, AutoDock 4 desolvation term, non-hydrophobic contact term and Lennard–Jones 4 − 8 van der Waals term [Citation22]. In the ligand–protein docking by Smina and for scoring purposes, the heavy atom interactions between the ligand and receptor along with intramolecular heavy atom interactions are considered. For the recognition of the binding sites in CD44 receptor, blind docking was carried out with setting of grid size to 36, 42 and 62 along x, y and z axes with a grid spacing of 1 Å after assigning the protein and probe with Kollman charges. The grid center was set at 2, 1 and −10 Å and the other parameters were set to default settings. Then, Smina was run with default settings, which samples nine HA and MTX-EDA-PEG-EDA-HA NPs conformations using the Vina docking routine of Monte Carlo stochastic sampling. For each of the docking cases, the lowest energy docked conformation, according to the Smina scoring function, was selected as the binding mode. The output from Smina was presented with BIOVIA Discovery Studio client 2016 [Citation19].
Results
Characterization of MTX-EDA-PEG-EDA-HA NPs
In the first step of synthesis, hydroxyl groups of PEG were activated by tosyl chloride when three molar excess of TsCl were added to the reaction mixture. As seen in the FTIR spectra of PEG-diTS (), OH signals of PEG at 3500 cm−1 are disappeared and the aromatic peaks at 1350 and 2580 cm−1 are appeared in comparison with FTIR spectra of PEG (). These activated intermediates were then used to prepare bis(ethylenediamine)-polyethylene glycol which have two amino groups on either side of it. For this reason, in , the related peaks of these amino groups are appeared at1640 and 3420 cm−1, and the related signals of tosyl groups are disappeared.
With one equivalent of CDI at slightly basic conditions (pH 9), an amino group on one side of the polymer was activated for more decoration with MTX. The main absorption peak in the FTIR spectrum of this dark blue product is νmax = 1720 cm−1 which confirm created amide bond between MTX and PEG (). And finally, for conjugation of HA to the polymeric NPs, carboxyl groups of HA were activated using DCC and NHS, and were then chemically coupled to the amino group of MTX-EDA-PEG-EDA at the other end. schematically depicts whole steps of the synthesis. The FTIR spectroscopy confirmed the modification of polymeric NPs with HA (); for instance, the broad and strong peaks at 1700 and 3300 cm−1, respectively, show the existence of the numerous carboxyl and hydroxyl groups in HA.
From the 1H NMR results, it is conceivable that MTX-EDA-PEG-EDA-HA NPs are formed. The specific peaks in showed clearly signals attributable to PEG moieties (δ 3.55–3.70 related to –O–CH2–CH2–O–), HA backbone (δ 2.45 related to N-acetyl group, δ 3.0–4.0 related to glucosidic H and δ 4.35–4.71 related to anormeric H) and MTX drug (δ 7.27 and 7.57 related to aromatic rings). The characteristic peaks of MTX in comparison with characteristic peaks of PEG and HA are minimal. Also, the degree of substitution (DS) of PEG to HA was calculated to be 13 by comparing the integration ratio between the peaks for HA (2.45) and PEG (3.55–3.70 ppm) in 1H-NMR spectra.
Particle size analyzer measures the size and the distribution of MTX-EDA-PEG-EDA-HA NPs in a sample solution. As shown in , an average size of about 338 nm was determined for these polymeric NPs. Also, the size of prepared NPs was characterized using the FESEM images in low and high magnifications. It can be concluded from that the average size of polymeric NPs is less than 350 nm.
Drug loading and reduction-triggered release of MTX from NPs
Chemical conjugation of MTX into polymeric NPs was done by creation of amide bond utilizing carbonyldiimidazole and was determined by UV spectrophotometry. Loading content of MTX (52.67%) displayed good capacity for drug encapsulation into polymeric NPs. Also, the in vitro drug release studies were carried out using MTX-EDA-PEG-EDA-HA NPs and free MTX at physiological condition (pH 7.4, 37 °C) and also at lysosome-like condition (pH 5, 37 °C and pure protease). The results of this study were showed that the release of MTX from MTX-EDA-PEG-EDA-HA NPs at pH 7.4 was largely inhibited by amide bond compared with free MTX. As shows, 44.12% of drug was released in 72 h from MTX-EDA-PEG-EDA-HA NPs while nearly 96.70% of free MTX crossed across the pores of the dialysis membrane after 5 h. Due to the presence of protease enzyme in dialysis bag and pH 5, it is conceivable that the release rate of MTX from MTX-EDA-PEG-EDA-HA NPs be greater than the release rate under physiological condition. It can be concluded from that 87.70% of MTX has been released in 72 h under simulated intracellular condition.
Cytotoxicity of MTX-loaded HA-PEG NPs
illustrates the cytotoxicity results for two cancer cell lines (e.g. MDA-MB-231 and MCF-7) incubated with five different concentrations of MTX-EDA-PEG-EDA-HA NPs and free MTX for 48 and 72 h. The MTX-EDA-PEG-EDA-HA NPs showed significant cytotoxic effects on cell viability in the CD44-positive MDA-MB-231 breast cancer cell line. On the contrary, MTX-EDA-PEG-EDA-HA NPs did not show any appreciable cytotoxicity in the CD44-negative MCF-7 epithelial cell line throughout the entire times of treatment. In MDA-MB-231 cell line, the IC50 value of MTX conjugated-NPs was evaluated 103 μM.
Figure 5. The cytotoxicity results of MTX-EDA-PEG-EDA-HA NPs and pure mitoxantrone in the (A) MDA-MB-231 and (B) MCF-7 cancer cell lines for 48 and 72 h. Data represents mean (n = 5) +SD.
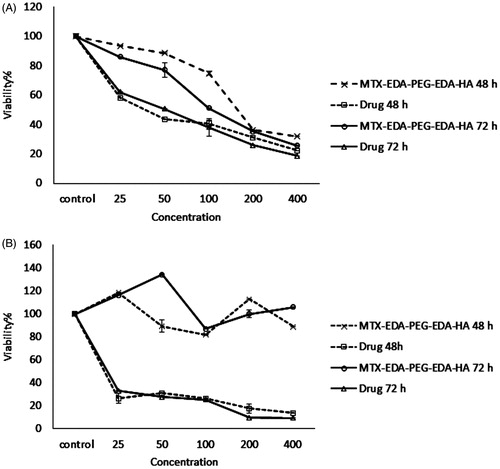
Conjugation of MTX on HA-polymeric NPs poses a major obstacle for localization of MTX in the CD44-negative tumour cells. As a consequence, free MTX induced cytotoxicity in two breast cancer cell lines, MCF-7 and MDA-MB-231 throughout the entire range of concentrations after incubation for 72 h. The IC50 values of 9 μM and 52 μM were achieved for free MTX in MCF-7 and MDA-MB-231 cell lines, respectively.
Molecular docking analysis
The results from the Smina output showed that HA and MTX-EDA-PEG-EDA-HA NPs can create nine different conformations which are ranked according to affinity score in . The best binding conformation of HA and MTX-EDA-PEG-EDA-HA NPs simultaneously within CD44 receptor is presented in . Also, the best-docked conformation of the HA within CD44 receptor along with the labelled key residues associated to affinity −6.7 kcal mol−1 is shown in . It can be concluded that 4 conventional hydrogen bond can occur between the O atoms of HA and SER109, TYR79, THR108 and TYR 105 of the CD44 receptor. Moreover, the SER109 and TYR105 of CD44 receptor form the unique carbon-hydrogen bond (C–H bond) with the HA ligand. Additionally, a pi–alkyl interaction between HA and the TYR42 of receptor is observed.
Figure 6. The docking results of HA and MTX-EDA-PEG-EDA-HA NPs with 3D structure of CD44 (A) the binding site of HA and MTX-EDA-PEG-EDA-HA NPs in CD44. (B) The conformation of HA in the binding site of CD44 and (C) the conformation of MTX-EDA-PEG-EDA-HA NPs in the binding site of CD44.
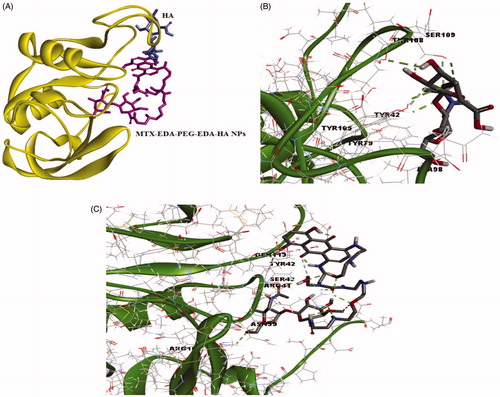
Table 1. Smina score of CD44 with different conformers of HA and MTX-EDA-PEG-EDA-HA NPs.
In , the best-docked conformation of the MTX-EDA-PEG-EDA-HA NPs within CD44 receptor which is related to affinity −5.7 kcal mol−1 along with the labelled key residues is demonstrated. Detailed analysis of the best-docked conformation for MTX-EDA-PEG-EDA-HA NPs shows that 10 conventional hydrogen bond can occur between this ligand and ARG162, ASN39, ARG41, GLN113, TYR42 and SER43 of the CD44 receptor. The SER43, one of the last amino acids of CD44 receptor, also has the C–H bond with the MTX-EDA-PEG-EDA-HA NPs. Moreover, there are two pi–pi stacking interactions between TYR42 of receptor and MTX-EDA-PEG-EDA-HA NPs. Also 3D protein docking was done for mentioned ligands; and accordingly in , the 2D schematic interaction model is provided to investigate and explain the interaction models between ligands and CD44 receptor. In general, molecular docking study confirmed that MTX-EDA-PEG-EDA-HA NPs can bind to the receptor-binding site more effective in comparison with HA, and, therefore, it can suggest as a novel and potent carrier for MTX delivery toward CD44-positive cancer cells.
Discussion
HA has inherent ability to target the CD44 receptors and internalize into tumour cells via receptor-mediated endocytosis [Citation23]. Therefore, conjugation of this natural linear polysaccharide to polymeric NPs or micelles, as one of the most promising approaches, could be useful for future clinical applications such as tissue engineering, drug delivery and molecular imaging [Citation9,Citation24]. Accordingly, the biodegradable and biocompatible HA-polymeric NPs possessing CD44 targeting potential were synthesized for MTX delivery toward special tumour cells.
MTX has been used as a clinical anticancer against some types of cancers such as breast cancer, ovarian cancer, lymphoma, acute non-lymphocytic leukaemia and non-Hodgkin’s lymphoma [Citation25]. The pharmacological activity of MTX was showed that it can significantly promote the single- and double-strand disruptions in DNA by stabilizing the cleavable complex formed between DNA and the nuclear enzyme topoisomerase II [Citation3]. Despite the potential advantages of the MTX, it affects both cancerous and normal cells and demonstrates unwanted side effects, particularly dose-related cardiomyopathy [Citation26]. In our previous works, it was found that the folate receptor-targeting NPs can be internalized into the FR-positive MCF-7 cells through receptor-mediated endocytosis [Citation4,Citation10,Citation27]. But in this work, we focus on CD44 receptor for specific cellular uptake. The physicochemical and morphological characterization analysis (NMR, FTIR and SEM) showed that conjugation of PEG with HA formed robust nano-sized NPs with an average size of ∼350 nm depending on DS, which is defined as the number of PEG units per 100 sugar residues of HA polymer. Moreover, monodispersity index (MDI) and polydispersity index (PDI) are main factors that are provided by Zetasizer, where MDI is related to the amount of uniformity in the size and shape of a set of particles and PDI measures the second moment of the size distribution of the NP population [Citation28]. The results of particle size analyzer () confirmed that MTX-EDA-PEG-EDA-HA NPs are monodisperse NPs with mean diameter of 338 nm and with low PDI of 0.622 [Citation29].
Table 2. Size information of MTX-EDA-PEG-EDA-HA NPs gained from zetasizer.
Lysosomal enzymes such as glycosidases, proteases and sulphatases which are active at the acidic pH (about 5) can hydrolyze the extracellular molecules [Citation30,Citation31]. Therefore, we hypothesize that the release of the chemically conjugated MTX from polymeric NPs to be an enzyme-dependent process. The results from the release at pH 7 showed that liberation of MTX from HA-polymeric NPs is largely inhibited by amide bond, while release of the MTX at pH 5 in the presence of protease as a selected hydrolases is enzyme-dependent release. In general, it can be concluded that the rate of liberation of MTX astonishingly can be depend on the presence of enzyme; because at the same times, the enzymes could release two equal of the drugs ().
There is no doubt that the highly permeable vascular structure of the neoplasm due to rapid and abnormal angiogenesis can lead to higher accumulation of NPs in intracellular compartments of tumours [Citation32]. But, in these passive targeting methods, specific cellular uptake is lacking, and that is why conjugation with cell surface targeting agents is the best strategy for improved cellular uptake of NPs by vesicular machineries of the target cells. In recent years, HA nanoshells have received growing scientific attention because of specific binding to CD44 receptors. This non-toxic glycosaminoglycan polymer has got many functional groups required for chemical modification or conjugation with other biocompatible polymers such as PEG; so, because of their lower immunogenicity, they can create tolerable polymeric NPs for the human body [Citation33].
Molecular docking method is a computational chemistry tool which is essential to gain further insights into the ligand–receptor interactions and the rational drug design process [Citation34,Citation35]. This method is used in two situation; prior to experimental screening where it can be considered as powerful computational filters to reduce experiments and costs needed for the effective development of medicinal compounds, and after experimental screening where it can help to better understanding of bioactivity mechanisms [Citation19]. In order to investigate the interaction between a rigid binding site and flexible ligands, authors performed docking of both HA and MTX-EDA-PEG-EDA-HA NPs into the 3D X-ray structure of CD44 receptor due to search the best binding site among all the probable cavities in the protein structure. Molecular docking of MTX-EDA-PEG-EDA-HA NPs confirms the ability of ligand to create 10 conventional hydrogen bonds with amino acids of CD44 receptor. Also, the results from the MTT assay showed that MTX-EDA-PEG-EDA-HA NPs can induce cytotoxicity in MDA-MB 231 (∼74% toxicity after 72 h) in a time- and concentration-dependent manner. On the contrary, MTX-EDA-PEG-EDA-HA NPs did not show any appreciable cytotoxicity throughout the entire range of times and concentrations in MCF-7 cell lines and the cell viability remained at more than 98% after 72 h treatment. Therefore, we were witnesses of the targeting of CD44-positive MDA-MB-231 breast cancer cell line by naturally occurring high-molecular weight HA.
Conclusions
Due to naturality, biodegradability and solubility of both HA and polyethylene glycol, they are used in structure of nanodrugs for prolonging the circulation time and reducing the blood clearance. Accordingly, the biodegradable and biocompatible HA-polymeric NPs possessing CD44 targeting potential were synthesized and their properties were characterized by NMR, FTIR, SEM, particle size analyzer, molecular docking, MTT assay and enzyme-dependent release. The results from all trials showed that MTX-EDA-PEG-EDA-HA NPs can bind to the receptor-binding site more effective and show significant cytotoxic effects on cell viability in the CD44-positive cell lines. Therefore, we hypothesize that MTX-conjugated polymeric NPs could internalize into tumour cells through receptor-mediated endocytosis, could release via lysosomal enzymatic system and could be useful for future clinical applications.
Acknowledgements
The authors thank the Deputy of Research and Technology, and Student Research Committee of Zabol University of Medical Sciences, for all support provided.
Disclosure statement
No potential conflict of interest was reported by the authors.
References
- Barar J, Kafil V, Majd MH, et al. Multifunctional mitoxantrone-conjugated magnetic nanosystem for targeted therapy of folate receptor-overexpressing malignant cells. J Nanobiotechnol. 2015;13:26.
- Dores-Sousa JL, Duarte JA, Seabra V, et al. The age factor for mitoxantrone’s cardiotoxicity: multiple doses render the adult mouse heart more susceptible to injury. Toxicology. 2015;329:106–119.
- Rossato LG, Costa VM, de Pinho PG, et al. The metabolic profile of mitoxantrone and its relation with mitoxantrone-induced cardiotoxicity. Arch Toxicol. 2013;87:1809–1820.
- Heidari Majd M, Asgari D, Barar J, et al. Specific targeting of cancer cells by multifunctional mitoxantrone-conjugated magnetic nanoparticles. J Drug Target. 2013;21:328–340.
- Liu Y, Sun J, Cao W, et al. Dual targeting folate-conjugated hyaluronic acid polymeric micelles for paclitaxel delivery. Int J Pharm. 2011;421:160–169.
- Heidari Majd M, Akbarzadeh A, Sargazi A. Evaluation of host-guest system to enhance the tamoxifen efficiency. Artif Cells Nanomed Biotechnol. 2017;45:441–447.
- Sargazi A, Majd MH. Different applications of magnetic nanoparticles in the rapid monitoring of ochratoxin A. Orient J Chem. 2017;33:346–354.
- Sargazi A, Aliabadi A, Rahdari A, et al. A simple and fast method for magnetic solid phase extraction of ochratoxin A. J Braz Chem Soc. 2017;28:950–959.
- Lin WJ, Lee W-C, Shieh M-J. Hyaluronic acid conjugated micelles possessing CD44 targeting potential for gene delivery. Carbohydr Polym. 2017;155:101–108.
- Sargazi A, Kuhestani K, Nosrat Nahoki T, et al. Specific targeting of folate receptor by methotrexate conjugated modified magnetic nanoparticles: enzymatic release and cytotoxic study. Int J Pharm Sci Res. 2015;6:5047–5055.
- Saei AA, Barzegari A, Majd MH, et al. Fe3O4 nanoparticles engineered for plasmid DNA delivery to Escherichia coli. J Nanopart Res. 2014;16:2521.
- Banerji S, Wright AJ, Noble M, et al. Structures of the Cd44–hyaluronan complex provide insight into a fundamental carbohydrate-protein interaction. Nat Struct Mol Biol. 2007;14:234–239.
- Yin H, Zhao F, Zhang D, et al. Hyaluronic acid conjugated β-cyclodextrin-oligoethylenimine star polymer for CD44-targeted gene delivery. Int J Pharm. 2015;483:169–179.
- Choi KY, Chung H, Min KH, et al. Self-assembled hyaluronic acid nanoparticles for active tumor targeting. Biomaterials. 2010;31:106–114.
- Nguyen TT, Tran DP, Huy PDQ, et al. Ligand binding to anti-cancer target CD44 investigated by molecular simulations. J Mol Model. 2016;22:165.
- Hiraga T, Nakamura H. Comparable roles of CD44v8-10 and CD44s in the development of bone metastases in a mouse model. Oncol Lett. 2016;12:2962–2969.
- Qhattal HSS, Liu X. Characterization of CD44-mediated cancer cell uptake and intracellular distribution of hyaluronan-grafted liposomes. Mol Pharm. 2011;8:1233–1246.
- Mansouri-Torshizi H, Rezaei E, Kamranfar F, et al. Investigating the apoptosis ability of ethylenediamine 8-hydroxyquinolinato palladium (II) complex. Adv Pharm Bull. 2016;6:449–453.
- Shiri F, Shahraki S, Baneshi S, et al. Synthesis, characterization, in vitro cytotoxicity, in silico ADMET analysis and interaction studies of 5-dithiocarbamato-1,3,4-thiadiazole-2-thiol and its zinc(ii) complex with human serum albumin: combined spectroscopy and molecular docking investigations. RSC Adv. 2016;6:106516–106526.
- Teriete P, Banerji S, Noble M, et al. Structure of the regulatory hyaluronan binding domain in the inflammatory leukocyte homing receptor CD44. Mol Cell. 2004;13:483–496.
- Koes DR, Baumgartner MP, Camacho CJ. Lessons learned in empirical scoring with Smina from the CSAR 2011 benchmarking exercise. J Chem Inf Model. 2013;53:1893–1904.
- Huey R, Morris GM, Olson AJ, et al. A semiempirical free energy force field with charge-based desolvation. J Comput Chem. 2007;28:1145–1152.
- Jordan AR, Racine RR, Hennig MJP, et al. The role of CD44 in disease pathophysiology and targeted treatment. Front Immunol. 2015;6:182.
- Lee H, Lee K, Park TG. Hyaluronic acid − paclitaxel conjugate micelles: synthesis, characterization, and antitumor activity. Bioconjug Chem. 2008;19:1319–1325.
- An G, Morris ME. HPLC analysis of mitoxantrone in mouse plasma and tissues: application in a pharmacokinetic study. J Pharm Biomed Anal. 2010;51:750–753.
- Johnson JL, Ahmad A, Khan S, et al. Improved liquid chromatographic method for mitoxantrone quantification in mouse plasma and tissues to study the pharmacokinetics of a liposome entrapped mitoxantrone formulation. J Chromatogr B: Analyt Technol Biomed Life Sci. 2004;799:149–155.
- Heidari Majd M, Asgari D, Barar J, et al. Tamoxifen loaded folic acid armed PEGylated magnetic nanoparticles for targeted imaging and therapy of cancer. Colloids Surf B Biointerfaces. 2013;106:117–125.
- Dhiman S, Mishra N, Sharma S. Development of PEGylated solid lipid nanoparticles of pentoxifylline for their beneficial pharmacological potential in pathological cardiac hypertrophy. Artif Cells Nanomed Biotechnol. 2016;44:1901–1908.
- Zhong Y, Zhang J, Cheng R, et al. Reversibly crosslinked hyaluronic acid nanoparticles for active targeting and intelligent delivery of doxorubicin to drug resistant CD44+ human breast tumor xenografts. J Control Release. 2015;205:144–154.
- Mindell JA. Lysosomal acidification mechanisms. Annu Rev Physiol. 2012;74:69–86.
- Huang Y, Chu T, Liao T, et al. Downregulation of lysosomal and further gene expression characterization in lung cancer patients with bone metastasis. Artif Cells Nanomed Biotechnol. 2017;45:758–764.
- Carmeliet P, Jain RK. Angiogenesis in cancer and other diseases. Nature. 2000;407:249–257.
- Thomas RG, Moon MJ, Lee H, et al. Hyaluronic acid conjugated superparamagnetic iron oxide nanoparticle for cancer diagnosis and hyperthermia therapy. Carbohydr Polym. 2015;131:439–446.
- Ramírez D, Caballero J. Is it reliable to use common molecular docking methods for comparing the binding affinities of enantiomer pairs for their protein target? IJMS. 2016;17:525.
- Oksuzoglu E, Ertan-Bolelli T, Can H, et al. Antitumor activities on HL-60 human leukemia cell line, molecular docking, and quantum-chemical calculations of some sulfonamide-benzoxazoles. Artif Cells Nanomed Biotechnol. 2016;1–9.