Abstract
A Tempol compound with an amine group (4-amino-2,2,6,6-tetramethylpiperidine-1-oxyl, NH2-Tempol) was cross-linked to hemoglobin in a one-step polymerization reaction to produce a novel hemoglobin-based oxygen carrier (HBOC) designated PolyHb-Tempol. The reaction parameters, including the reaction time, pH, temperature, and ratio of reactants, were optimized, and the physiochemical properties of the resulting product were characterized. PolyHb-Tempol didn’t show any toxicity towards endothelial cells. Furthermore, from observations of cell morphology and viability, PolyHb-Tempol showed a significant ability to inhibit or eliminate oxidative stress induced by superoxide free radicals. These results suggest that PolyHb-Tempol may potentially be suitable as an HBOC.
Introduction
Hemoglobin (Hb) is both an oxygen carrier and pro-oxidant that participates in complex redox processes during conditions of oxidative stress. As a blood substitute, Hb-based oxygen carriers (HBOCs) can exert both inflammatory and toxic effects from oxidative processes involving the reactive group [Citation1–4], such as the formation of ferric ions (Fe3+), ferryl ions (Fe4+), ferryl heme radicals (•H-Fe4+), ferryl protein radicals (•P-Fe4+), hemichromes, protein radical-induced globin chain cross-links, free heme/iron and non-heme radical species [Citation5–7]. Adverse microvascular effects of cell-free Hb have also hindered the development of safe and efficacious HBOCs. Although the pathophysiological relevance of Hb-induced oxidative stress is not well-understood, the interactions of Hb with reactive oxygen species/reactive nitrogen species (ROS/RNS) such as superoxide radical (OO2• -), hydrogen peroxide (H2O2), hydroxyl radical (OH•), and peroxynitrite (ONOO−), have been implicated. A means to remove all or most ROS/RNS would be advantageous for the development of a safe and efficacious HBOC.
Superoxide dismutase/catalase (SOD/CAT) or their mimics, polymerized with HBOCs, are currently being developed in order to avoid the potential for HBOC-mediated oxidative stress and ischemia/reperfusion (I/R) injury. Tempol (2,2,6,6-tetramethylpiperidine-1-oxyl) is a nitroxide compound that can shuttle between the nitroxide radical, the reduced hydroxylamine and the oxidized oxoammonium cation form [Citation8]. This compound can promote the metabolism of many ROS and improve nitric oxide bioavailability [Citation9–11]. Tempol has been studied extensively in animal models of oxidative stress and has been shown to preserve mitochondria against oxidative damage and improve tissue oxygenation. In a previous study, a variant of Tempol with a hydroxy group (4-hydroxy-2,2,6,6-tetramethylpiperidine-1-oxyl, OH-Tempol) was attached to Hb as a means of inhibiting ROS [Citation12,Citation13]. However, this attachment method required a “linker arm” modification of Hb. The multiple modification steps in this method are laborious and have the potential to cause Hb instability or precipitation [Citation14].
In the present study, we polymerized NH2-Tempol and Hb directly through glutaraldehyde (). The effects of polymerization parameters, including reaction time, pH of the solution, temperature and ratio of reactants, on the final product (PolyHb-Tempol) were investigated in detail. The optimized PolyHb-Tempol was characterized and its SOD activities evaluated.
Materials and methods
Materials
Ultra-pure porcine Hb, free of SOD and CAT, was obtained from Lifegen Co., Ltd. (Xi’an, China). ECV-304 cells were a gift from the Fourth Military Medical University (Xi’an, China). NH2-Tempol was synthesized according to published methods [Citation15,Citation16]. 5-Diphenyltetrazolium bromide (MTT) and xanthine/xanthine oxidase were purchased from J&K (Shanghai, China). Fetal calf serum (FCS) was purchased from Sijiqing Biological Engineering Materials Co. Ltd (Hangzhou, China), penicillin–streptomycin from HyClone Laboratories, Inc. (Logan, UT) and trypsin from Gibco (Waltham, MA). All other reagents were purchased from Sigma-Aldrich Co., Ltd. (St. Louis, CA).
Polymerization of NH2-Tempol and Hb
After optimization of reaction conditions (pH = 7.5, T = 10 °C, t = 2 h), 10 ml 50 mg/ml deoxy-Hb and 2.5 ml 10 mg/ml NH2-Tempol were added to a 100-ml three-neck flask. With a pressure-equalizing dropping funnel, 1.2 ml 1% aqueous glutaraldehyde was gradually added to the flask and stirred at 30 rpm for 2 h. The reaction was quenched by adding dimethylamineborane (4.8 ml, 94.6 mM). All of the solutions were deoxygenated with nitrogen before addition, and the whole reaction process was protected with nitrogen. The concentration of PolyHb-Tempol was expressed by the concentration of component Hb. After the reaction, the unpolymerized Hb and unreacted reagents were filtered out with a 100-kDa cut-off hollow fiber (UFP-100-E-3MA, GE Healthcare, Buckinghamshire, UK) then the remaining unreacted reagents were removed by dialysis against normal saline. The dialysate in the dialysis bag was analyzed with a UV2550 ultraviolet spectrophotometer (Shimadzu Corporation, Shimadzu, Japan) at 450 nm to determine the content of PolyHb-Tempol.
The Hb and methemoglobin (metHb) concentrations were determined via the HiCN method at 630 nm [Citation17]. Conditions selected were pH = 7.5, T = 10 °C, t = 2 h, and the concentration ratio Hb: glutaraldehyde (GA): NH2-Tempol = 1:20:24 to produce PolyHb-Tempol. The average particle size of PolyHb-Tempol was measured using a Zetasizer Ver. 6.11 (Malvern Instruments Ltd, Malvern, UK).
Characterization of PolyHb-Tempol polymer
The MALLS-SEC system used to determine the molecular weight of PolyHb-Tempol included a detector of Multi-Angle Static Light Scattering (DAWN II), an Optilab T-rEX refractometer (both from Wyatt Technology Corporation, Goleta, CA), and a pump (LC-10AT, Shimadzu Corporation). Prior to use, the mobile phase (0.2 mol/L phosphate buffer, PB) was filtered twice through Minisart® membranes (0.22 μm pore size, Sartorius Stedim, Gottingen, Germany). The system was then flushed with ultrapure water and balanced with the mobile phase. The semi-preparative column was Superdex™ 200, 10/300 GL (GE Healthcare, Buckinghamshire, UK). A hemox analyzer (TCS Scientific Corporation, New Hope, PA) was used to determine the partial pressure of oxygen required for 50% Hb saturation (P50) of PolyHb-Tempol.
Determination of ESR spectra of PolyHb-Tempol
The ESR (electron spin resonance) spectra of PolyHb-Tempol or Tempol were determined using an ESR instrument (JES-FA200, Jeol Ltd., Tokyo, Japan) [Citation18,Citation19], and were collected at room temperature on a Bruker EMX model X-band spectrometer operating at a frequency of 9.50 GHz. The static magnetic field was varied in the range 0–6000 G. The field derivative of microwave power absorption (dW/dH1) was registered as a function of the applied magnetic field H1. All samples were loaded into quartz ESR tubes to determine ESR spectra. The ESR densities of Tempol were used as the standard to calculate the molar number of Tempol modified in PolyHb-Tempol, namely Hb: nTempol.
Endothelial cell culture
ECV-304 cells were maintained in 1640 medium, supplemented with 10% FCS and penicillin–streptomycin (“complete medium”), in a humid atmosphere of 5% CO2 at 37 °C for 2–3 days. “Basic medium” consisted of 1640 medium. Following treatment, cells were harvested using 0.25% trypsin, 0.2% EDTA, centrifuged at 800g for 3 min, and washed twice with phosphate-buffered saline (1 × PBS).
Cytotoxicity assay
The cytotoxicity of PolyHb-Tempol was estimated using an MTT assay [Citation20,Citation21]. ECV-304 cells were seeded in 96-well plates at a concentration of 1 × 103–1 × 104 cells/well. After 2–3 days, the cells were treated with increasing concentrations of PolyHb-Tempol (0.064–1.6 mmol/L; 100 μL) or the same concentrations of Hb as a control. Then, 100 μL basic medium (BM) was added and the cells incubated in a humidified incubator at 37 °C for 24 h. Thereafter, 20 μL of MTT stock solution (0.5 mg/ml) was added and the cells incubated a further 4 h. Subsequently, the supernatants were aspirated and the formazan crystals in each well dissolved in 200 μL of DMSO. Absorbance was measured using a VersaMax microplate reader (Elx800UV, BioTek, Winooski, VT) at a wavelength of 490 nm.
MTT assay for measuring superoxide anion radical (O2• -) damage to cells
The xanthine/xanthine oxidase solution added to the cell medium to produce superoxide anion radicals (O2• -) was made by combining 2 ml 0.5 mol/L Tris–HCl, 1 ml 6 mg/ml xanthine, and 0.1 ml 0.36 U/ml xanthine oxidase. Superoxide anion radical (O2• -) damage was determined using an MTT cytotoxicity assay [Citation22].
Statistical analysis
Data are expressed as mean ± standard deviation (SD) of parallel independent experiments. All statistical and graphic analyses were performed using Origin 8 (Origin Lab Corporation, Northampton, MA), Adobe Illustrator CS6 (Adobe Systems Inc., San Jose, CA) and GraphPad Prism5 (GraphPad Software Inc., San Diego, CA).
Results
Selection of polymerization conditions
The preparation of HBOCs is typically through chemical modifications via various methods of intramolecular cross-linking, intermolecular polymerization, and surface conjugation. These methods all increase the molecular weight or the molecular radius of the HBOC and thereby avoid its rapid clearance and toxicity in the kidney [Citation23,Citation24]. In the polymerization process, oxyhemoglobin is apt to be oxidized to methemoglobin. An optimal preparation of PolyHb-Tempol should have a low metHb content, an appropriate particle size, and an ability to inhibit superoxide radicals efficiently. Accordingly, the parameters used to optimize the polymerization reaction conditions were metHb concentration, molecular radius and superoxide radical scavenging ability.
Reaction time
In order to optimize the reaction time, the initial concentrations of Hb:GA:NH2-Tempol = 1:10:12 (molar ratio) were chosen. The pH of the solution was adjusted to 8.5, the solution stirred at 25 °C, and glutaraldehyde gradually added to start the reaction. The reaction products at 0, 2, 4 and 6 h reaction time were evaluated. As shown in , the proportion of metHb in the reaction product increased rapidly during the first two hours of the polymerization process, and then leveled off at ∼46%. The superoxide radical scavenging activity of the product mirrored metHb in rising rapidly by 2 h and then leveling off at ∼72% with further reaction time (), which indicated Tempol can be easily modified to Hb through GA. The particle size of PolyHb-Tempol increased only slightly and remained close to the size of Hb (6–8 nm) by extending the reaction time beyond 2 h (). This suggests that the linking of Tempol to Hb is more efficient than the Hb polymerization reaction.
Figure 2. Effects of reaction time on the PolyHb-Tempol product. Time-dependent changes in the proportion of metHb (A), particle size (B) and superoxide radical scavenging activity (C) after glutaraldehyde addition to the Hb and NH2-Tempol. Data (C) is mean ± SD of three independent experiments. The letters above the bars (a, b) indicate significant differences (p < .05) between time points.
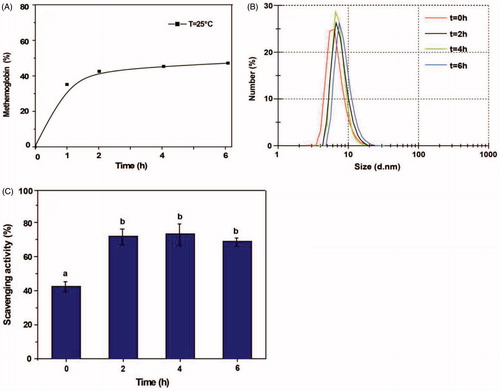
Temperature
As shown in , the proportion of metHb in the PolyHb-Tempol product was very much dependent on temperature, varying from 82% at 45 °C to 5% at 10 °C . The particle size of PolyHb-Tempol increased slightly with increasing temperature (). The superoxide radical scavenging activity first increased then subsequently decreased with increasing temperature, with a maximum value at 10 °C (). From these data, it can be seen that metHb can be inhibited and NH2-Tempol efficiently modified to Hb at 10 °C.
Figure 3. Effects of temperature on the PolyHb-Tempol product. Time-dependent changes in the proportion of metHb (A), particle size (B) and superoxide radical scavenging activity (C) after glutaraldehyde addition to the Hb and NH2-Tempol. Data (C) is mean ± SD of three independent experiments. The letters above the bars (a, b, c) indicate significant differences (p < 0.05) between temperature groups.
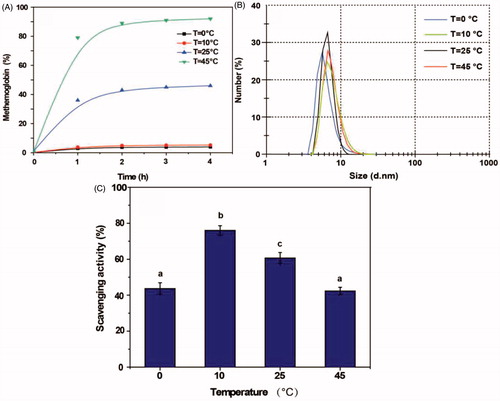
pH
As shown in , the proportion of metHb was highest (17.5%) under acidic (pH 6.5) conditions, but <5% at pH 8.5 and 9.5. However, the polymerization reaction almost failed to take place at pH 8.5 and 9.5 and the particle size remained almost unchanged. With a decrease in pH, the particle size increased (). Although PolyHb-Tempol had maximum superoxide radical activity at pH6.5 (), pH7.5 was determined to be the most suitable reaction condition in light of the increased generation of metHb under acidic conditions.
Figure 4. Effects of pH on the PolyHb-Tempol product. Time-dependent changes in the proportion of metHb (A), particle size (B) and superoxide radical scavenging activity (C) after glutaraldehyde addition to the Hb and NH2-Tempol. Data (C) is mean ± SD of three independent experiments. The letters above the bars (a, b, c) indicate significant differences (p < .05) between the pH groups.
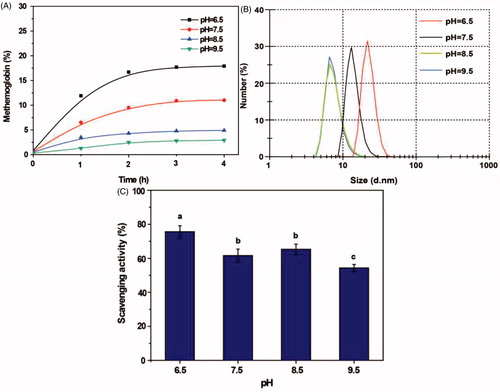
Ratio of reactants
As shown in , a higher ratio of glutaraldehyde and NH2-Tempol to Hb resulted in a higher proportion of metHb, with the highest level for Hb:GA:NTP = 1:30:36. The particle size of the product was almost unchanged from that of Hb at a reactant ratio of Hb:GA:NTP = 1:5:6, indicating that little polymerization took place under these conditions (). With an increasing ratio of GA and NTP to Hb, the particle size of PolyHb-Tempol gradually increased (diameters of 7, 30 and 82 nm, with ratios of Hb:GA:NTP of 1:10:12, 1:20:24 and 1:30:36, respectively). From , it can be seen that the superoxide radical scavenging activities is ∼70% with a ratio of Hb:GA:NTP of between 1:5:6 and 1:20:24. However, when the ratio of Hb:GA:NTP reached 1:30:36, the superoxide radical scavenged fell to the 32%. We used the reaction conditions (time 2 h, temperature 10 °C, pH 7.5, ratio of Hb:GA:NTP = 1:20:24) that produced PolyHb-Tempol of the optimal size, 30 nm, in our subsequent research.
Figure 5. Effects of ratio of reactants on the PolyHb-Tempol product. Time-dependent changes in the proportion of metHb (A), particle size (B) and superoxide radical scavenging activity (C) after glutaraldehyde addition to the Hb and NH2-Tempol. Data (C) is mean ± SD of three independent experiments. The letters above the bars (a, b) indicate significant difference (p < .05) between the concentration ratio groups.
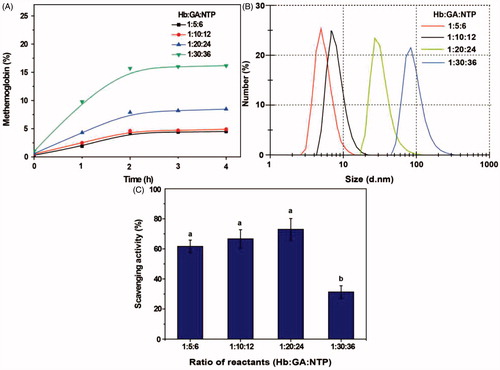
Characterization of PolyHb-Tempol
The physicochemical properties of the PolyHb-Tempol product used in subsequent experiments are presented in . This preparation of PolyHb-Tempol represents, on average, each 1 mM of tetrameric Hb covalently bound 7.3 mM Tempol. shows that the ESR spectrum of PolyHb-Tempol was similar to that of Tempol, which provides validation that Tempol was polymerized to Hb. Determination of the molecular weight (Mw) of the PolyHb-Tempol preparation by size-exclusion chromatography coupled to multi-angle laser light scattering indicated that its mean molecular weight was ∼783 kDa. The P50 of the PolyHb-Tempol preparation, as determined using a Hemox analyzer, was 30 mmHg, which is similar to the red blood cells (P50 = 26–28 mmHg).
Table 1. Physicochemical properties of PolyHb-tempol.
Toxicity of PolyHb-Tempol on cells
Although Tempol is as effective as the native SOD enzyme in preventing O2• - generation by angiotensin II in vascular smooth muscle cells, its ED50 was shown to be 10-fold higher [Citation25]. For use of PolyHb-Tempol as a red blood cell substitute, the transfusion volume would not be >70% of the total blood volume of a person, so we examined the toxicity PolyHb-Tempo towards ECV-304 cells at concentrations of up to 1.6 mmol/L. As shown in , neither PolyHb-Tempol nor Hb demonstrated significant toxicity, compared with the control group, over a concentration range from 0.064 to 1.6 mmol/L. The concentration of PolyHb-Tempol was expressed by the concentration of component Hb.
Protective effect of PolyHb-Tempol on cells
The protective effects on cells of PolyHb-Tempol and Hb, across varying concentrations of component Hb, were assessed and compared. The viabilities of endothelial cells, under conditions of superoxide anion radical (O2• -) damage induced by xanthine/xanthine oxidase, were determined using an MTT assay. shows that the cell viabilities gradually increased with an increase in Hb concentration with either added product. The concentration of PolyHb-Tempol was expressed by the concentration of component Hb. The cell viabilities in the PolyHb-Tempol groups were higher than for the Hb groups over the Hb concentration range of 2.5–62.5 μmol/L. However, the cell viabilities of the two groups were the same (∼90%) at concentrations of 312.5 μmol/L Hb. These data indicate that Hb alone has some ability to scavenge free radicals. The higher the Hb concentration is, the stronger the superoxide radical scavenging activity is. When the Hb concentration is high enough, the Hb protective effect is similar to that of PolyHb-Tempol.
Figure 8. Viability of following treatment of endothelial cells with xanthine/xanthine oxidase to induce O2• -. Endothelial cell viability was assessed using an MTT assay. Data is mean ± SD of five independent experiments, *p < .05 versus untreated control values, #p < .05 versus O2• - control values.
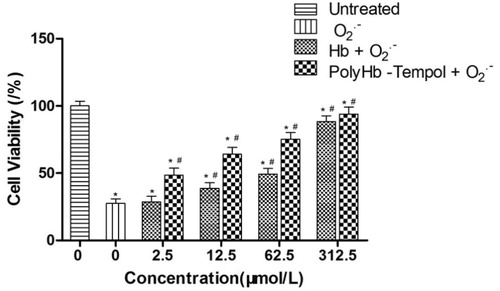
In order to further evaluate the protective effect of PolyHb-Tempol, endothelial cell morphology was assessed by phase-contrast microscopy after incubation of ECV-304 cells in 96-well plates with 200 μL complete medium for 2–3 days. shows the morphology of the cells incubated a further 24 h with basic medium (), or basic medium containing Hb (62.5 μmol/L) (), or containing 50 μL PolyHb-Tempol (62.5 μmol/L) (), or containing 50 μL xanthine/xanthine oxidase solution (), or 50 μL Hb and 50 μL xanthine/xanthine oxidase solution (), or 50 μL PolyHb-Tempol and 50 μL xanthine/xanthine oxidase solution (). Cells treated with xanthine/xanthine oxidase showed signs of cytoplasmic swelling, rounding, and detachment from the monolayer, consistent with superoxide anion radical (O2• -) damage [Citation26,Citation27]. Many of the detached rounded cells appeared smaller and were more irregularly shaped (). However, after Hb or PolyHb-Tempol was added to the cells, more cells retained a normal morphology [∼50% for Hb () and 75% for PolyHb-Tempol ()]. These results showed that PolyHb-Tempol is more efficient at scavenging free radicals than Hb alone and may potentially be suitable as an HBOC.
Figure 9. Morphologic changes of endothelial cells. Endothelial cells were incubated with 200 μL basic medium alone (A), 100 μL Hb (62.5 μmol/L) (B), 50 μL PolyHb-Tempol (62.5 μmol/L) (C), 50 μL xanthine/xanthine oxidase (D), 50 μL Hb and 50 μL xanthine/xanthine oxidase (E), or 50 μL PolyHb-Tempol (62.5 μmol/L) and 50 μL xanthine/xanthine oxidase (F). All volumes were made up to 200 μL with basic medium. Cells were viewed by phase-contrast microscopy and photographed (×40 objective).
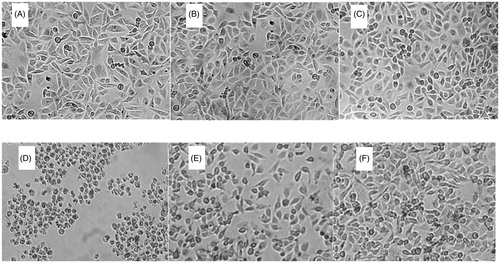
Discussion
Superoxide anions are continuously generated during normal cell metabolism, and can be generated at higher concentrations in certain pathological conditions. Tempol, a type of SOD mimetic, can play a protective role against oxidative damage. . From our cell-based assays, it is speculated that PolyHb-Tempol shares this property of Tempol and can inhibit oxidative stress in vivo.
An ideal blood substitute should be safe and effective. However, few HBOCs are currently in clinical use. Adverse pathophysiological outcomes from administering HBOCs include induced inflammatory response and vascular dysfunction. In recent years, several lines of evidence support the dominance of Hb oxidative reactions in the overall toxicology of HBOCs [Citation28,Citation29]. Autoxidation and oxidative modification of Hb ultimately lead to unfolding of the molecule and heme loss, which could trigger a cascade of inflammatory responses [Citation30,Citation31]. In order to overcome the defects of HBOCs, we crosslinked Hb and Tempol using a one-step polymerization reaction. The PolyHb-Tempol has similar P50 to normal red blood cells, so they have similar oxygen releasing abilities.
In addition, it is reported that hemogloblin lower than 64 kD molecular weight in HBOCs may lead to vasoconstriction and induce hypertension [Citation32], which is why we used a cut-off of 64 kD Hb in the preparation. Researchers currently think that the optimal particle size for HBOCs is 128 kD or higher [Citation33], but there is no consensus as to the appropriate range of their molecular weights. For example, the average molecular weight of HBOC-201 (Biopure Co. Ltd, Cambridge, MA) is 250 kD and that of Zero-link (oxyVita Inc.) is higher than 20 MD [Citation34,Citation35].
The one-step polymerization method described herein is simple and effective, with high recovery. In particular, it is remarkable that the PolyHb-Tempol has a significant ability to inhibit or eliminate oxidative stress induced by superoxide free radicals. Together, these results suggest that PolyHb-Tempol is a potentially suitable HBOC for further development.
Disclosure statement
No potential conflict of interest was reported by the authors.
Additional information
Funding
References
- Ratanasopa C, Bülow L. Possibilities of using fetal hemoglobin as a platform for producing hemoglobin-based oxygen carriers (HBOCs). Adv Exp Med Biol. 2016;876:445–453.
- Bäumler H, Zhi Zhao Liu YX, Patzak A, et al. Novel hemoglobin particles—promising new-generation hemoglobin-based oxygen carriers. Artif Organs. 2014;38:708–714.
- Toma VA, Roman I, Sevastre B, et al. Comparative in vivo effects of hemoglobin-based oxygen carriers (HBOC) with varying prooxidant and physiological reactivity. PLoS One. 2016;11:1–16.
- Dunne J, Caron A, Menu P, et al. Ascorbate removes key precursors to oxidative damage by cell-freehaemoglobin in vitro and in vivo. Biochem J. 2006;399:513–524.
- BorettiFS BP, D’Agnillo F, Kluge K, et al. Sequestration of extracellular hemoglobin within a haptoglobin complex decreases its hypertensive and oxidative effects in dogs and guinea pigs. J Clin Invest. 2009;119:2271–2280.
- Butt OI, Buehler PW, D’Agnillo F. Differential induction of renal heme oxygenase and ferritin in ascorbate and nonascorbate producing species transfused with modified cell-free hemoglobin. Antioxid Redox Signal. 2010;12:199–208.
- Ma L, Zhu X, Wu YY, et al. Protective effect of propyl gallate against oxidized low-density lipoprotein-induced injury of endothelial cells. Chin J Integr Med. 2015;21:299–306.
- Karanovic D, Grujic-Milanovic J, Miloradovic Z, et al. Effects of single and combined losartan and Tempol treatments on oxidative stress, kidney structure and function in spontaneously hypertensive rats with early course of proteinuric nephropathy. PLoS One. 2016;11:1–21.
- Vorobjeva NP, Pinegin BV. Effects of the antioxidants Trolox, Tiron and Tempol on neutrophil extracellular trap formation. Immunobiology. 2016;221:208–219.
- Nan L, Min L. GW25-e1582 impact of SOD mimetic tempol and exercise training on NOS in spontaneously hypertensive rats. J Am Coll Cardiol. 2014;64:C80.
- Yu V, Yushkova EIC, Yu F, et al. Synthesis of spin-labeled amides of 6-hydroxy-2,5,7,8-tetramethylchroman-2-carboxylic acid (trolox). Chem Nat Compd. 2013;49:253–257.
- Buehler PW, Haney CR, Gulati A, et al. Polynitroxyl hemoglobin: a pharmacokinetic study of covalently bound nitroxides to hemoglobin platforms. Free Radic Biol Med. 2004;37:124–135.
- Weinberg AN, Karen D, Yan C, et al. Prevention of catecholaminergic oxidative toxicity by 4-hydroxy-2,2,6,6-tetramethylpiperidine-1-oxyl and its recycling complex with polynitroxylated albumin, TEMPOL/PNA. Brain Res. 2004;1012:13–21.
- Scurtu F, Zolog O, Iacob B, et al. Hemoglobin – albumin cross-linking with disuccinimidyl suberate (DSS) and/or glutaraldehyde for blood substitutes. Artif Cells Nanomed Biotechnol. 2014;42:13–17.
- Peng George Wang MX, Tang X. Nitric oxide donors: chemical activities and biological applications. Chem Rev. 2002;102:1091–1134.
- Ricci A. Electron paramagnetic resonance (EPR) study of spin-labeled camptothecin derivatives: a different look of the ternary complex. J Med Chem. 2011;54:1003–1009.
- Lu M, Zhao L ,Wang Y, et al. Measurement of the methemoglobin concentration using Raman spectroscopy. Artif Cells Nanomed Biotechnol. 2014;42:63–69.
- Kimura S, Inoguchi T, Yamasaki T, et al. A novel DPP-4 inhibitor teneligliptin scavenges hydroxyl radicals: in vitro study evaluated by electron spin resonance spectroscopy and in vivo study using DPP-4 deficient rats. Metabolism. 2016;65:138–145.
- Dey M, Li X, Kunz RC, et al. Detection of organometallic and radical intermediates in the catalytic mechanism of methyl-coenzyme M reductase using the natural substrate methyl-coenzyme M and a coenzyme B substrate analogue. Biochemistry. 2010;49:10902–10911.
- Bilmin K, Kopczynska B, Grieb P. Influence of serum and albumin on the in vitro anandamide cytotoxicity toward C6 glioma cells assessed by the MTT cell viability assay: implications for the methodology of the MTT tests. Folia Neuropath. 2013;51:44–50.
- Kim YS. Antioxidant activity and protective effects of Trapa japonica pericarp extracts against tert-butylhydroperoxide-induced oxidative damage in Chang cells. Food Chem Toxicol. 2014;64:49–56.
- Auclair C, Torres M, Hakim J. Superoxide anion involvement in NBT reduction catalyzed by NADPH-cytochrome P-450 reductase: a pitfall. FEBS Lett. 1978;89:26–28.
- Sellards AW, Minot GR. Injection of hemoglobin in man and its relation to blood destruction, with especial reference to the anemias. J Med Res. 1916;34:469–494.
- Bunn HF, Esham WT, Bull RW. The renal handling of hemoglobin. Trans Assoc Am Physicians. 1968;81:147–152.
- Luo Z, Chen Y, Chen S, et al. Comparison of inhibitors of superoxide generation in vascular smooth muscle cells. Br J Pharmacol. 2009;157:935–943.
- Ono T, Tsuruta R, Fujita M, et al. Xanthine oxidase is one of the major sources of superoxide anion radicals in blood after reperfusion in rats with forebrain ischemia/reperfusion. Brain Res. 2009;1305:158–167.
- Masuokaa N, Niheib K, Maetaa A, et al. Inhibitory effects of cardols and related compounds on superoxide anion generation by xanthine oxidase. Food Chem. 2015;166:270–274.
- Alayash AI. Hemoglobin-based blood substitutes: oxygen carriers, pressor agents, or oxidants? Nat Biotechnol. 1999;17:545–549.
- Alayash AI. Oxygen therapeutics: can we tame haemoglobin? Nat Rev Drug Discov. 2004;3:152–159.
- Buehler PW. Toxicological consequences of extracellular hemoglobin: biochemical and physiological perspectives. Antioxid Redox Signal. 2010;12:275–291.
- Alayash AI. Blood substitutes: why haven’t we been more successful? Trends Biotechnol. 2014;32:177–185.
- Yu B, Shahid M, Egorina EM. Endothelial dysfunction enhances vasoconstriction due to scavenging of nitric oxide by a hemoglobin-based oxygen carrier. Anesthesiology. 2010;112:586–594.
- Greenburg AG, Kim HW. Civilian uses of hemoglobin-based oxygen carriers. Artif Organs. 2004;28:795–799.
- Stumpa DG, Holsonb JF, Harrisc C. Developmental toxicity in rats of a hemoglobin-based oxygen carrier results from impeded function of the inverted visceral yolk sac. Reprod Toxicol. 2015;52:108–117.
- Jia Y, Alayash AI. Effects of cross-linking and zero-link polymerization on oxygen transport and redox chemistry of bovine hemoglobin. Biochim Biophys Acta. 2009;1794:1234–1242.