Abstract
Green synthesis of silver nanoparticles (AgNPs) has received many interests as a simple, cost-effective, and environmentally friendly method. This study reported the use of sericins extracted from non-mulberry (Samia cynthia ricini) and mulberry (Bombyx mori) silkworms for green syntheses of AgNPs. Both sericins possessed the reducing activity, which the reducing activity of S. c. ricini sericin was significantly higher than that of B. mori sericin. The formation of AgNPs facilitated by S. c. ricini sericin was greater than B. mori sericin as determined by the intensity of the surfacing plasmon resonance peak of silver at 412 nm. The synthesized AgNPs using both sericins were spherical and uniform in size with the average diameter of ∼13 nm. The silver component and the crystalline structure was determined by energy-dispersive X-ray and X-ray diffraction analyses. The synthesized AgNPs exhibited the antibacterial activity against both Gram-positive Staphylococcus aureus and Gram-negative Escherichia coli, suggesting their potential application as an effective antibacterial agent.
Introduction
Silver nanoparticles (AgNPs) have received increasing interests as a biocide agent to replace antibiotics in health, food and textile applications [Citation1]. There are three major routes for synthesizing AgNPs: chemical, physical and biological. Although chemical and physical approaches offer quick and mass production of AgNPs with a good control of size distribution, a high production cost, toxicity of used reductants, and aggregation of produced particles are the limitation of these approaches [Citation2]. Green synthesis has come forth as an upsurge and alternative method to avoid the problems and limitations of physical and chemical approaches for nanoparticle synthesis. The green synthesis of metal nanoparticles has become a prominent area of interest in the field of nanoscience and technology, as it offers non-toxic, economically viable and eco-friendly processes. Most reports of green synthesis of AgNPs were the uses of plant extracts, which mainly contained phytochemicals such as terpenoids, flavonoids, phenols, and alkaloids to function as reducing agents [Citation3]. In addition to phytochemicals, other natural biomolecules, especially proteins, could assist the formation and stabilization of AgNPs. However, there are still few reports on uses of proteins for green syntheses of AgNPs; egg white [Citation4], casein-hydrolytic peptides [Citation5], bovine serum albumin [Citation6], and silk fibroin of Bombyx mori silkworm [Citation7]. Some amino acids, especially tyrosine and tryptophan, are proposed to serve as reducing agents, while a protein structure can stabilize the formed particles [Citation8].
Sericin is a glue-like coating protein capping the silk core, which is removed in a degumming process of silk thread production to improve the luster, softness, smoothness, whiteness and dye-staining ability of the silk threads. In each year, sericin was discarded as waste ∼50,000 tons out of 1 million tons of fresh cocoons produced [Citation9]. Thus, value adding of sericin has received many research interests, including its application for green synthesis of AgNPs. In sericultrue industries, most silk threads are produced from mulberry B. mori. In last few years, non-mulberry silkworms, especially eri (Samia cynthia ricini), was promoted in sericulture industries. Thus, sericin of S. c. rinici is also questionable for its applications in a similar manner of sericin of B. mori, especially for a green synthesis of AgNPs in this work. The sericin of B. mori was initially reported to function as only a stabilizing agent and an addition of sodium borohydride as a reducing agent was required for a green synthesis of AgNPs [Citation10]. Later, there were reported that B. mori sericin could be functioned as both reducing and stabilizing agents for a production of AgNPs, but the synthesis reactions occurred under an alkaline condition (pH 9.5–11.0) by adding NaOH. Under this condition, sericin was hydrolyzed into very small peptides and free amino acids, which can reduce Ag+ to Ag0 [Citation11,Citation12]. However, an alkaline trace could affect an application of AgNPs that required a neutral condition. Therefore, this work is interested in studying a green synthesis of AgNPs under a neutral condition. Since a green synthesis of AgNPs using S. c. ricini sericin has not been studied and its differences of amino acid compositions and protein structures may affect the production of AgNPs, this work also investigates on the green synthesis of AgNPs using S. c. ricini sericin compared with B. mori sericin. The characterization and antibacterial activity of the produced AgNPs were also studied.
Materials and methods
Materials
Fresh silk cocoons (B. mori and S. c. ricini) were purchased from the Pongpet farm (Kalasin, Thailand). Silver nitrate (AgNO3), d-glucose, and Muller-Hinton (MH) medium were from Sigma-Aldrich Corporation (St. Louis, MO), VWR International (Leuven, Belgium), and Merck (Dramstadt, Germany), respectively. All other chemicals used were of analytical grade.
Extraction of sericins
Fresh silk cocoons (5 g) of B. mori and S. c. ricini were cut into pieces and extracted in boiling water (200 ml) for 1 h. After removing the remaining cocoons, each extracted solution was filtered through a Whatman paper No. 1. The protein concentration was determined by a Bio-Rad protein assay (Bio-Rad, Hercules, CA). The extraction yield was determined by the dried weight of the extract per 1 g silk cocoon. The pattern of the extracted proteins was analyzed on a 4–15% gradient sodium dodecyl sulphate–polyacrylamide gel electrophoresis (SDS–PAGE) gel and stained with Coomassie brilliant blue R-250 dye.
Determination of reducing power
The reducing power of the extracted sericin was evaluated by a modified method of Ferreira et al. [Citation13]. The extracted sericin (2.5 ml) was mixed with 200 mM sodium phosphate buffer (2.5 ml) and 1% potassium ferricyanide (2.5 ml). The reaction mixture was incubated at 50 °C for 20 min. Then, 10% (w/v) trichloroacetic acid (2.5 ml) was mixed before centrifuging at 3000g for 10 min. The supernatant (1.25 ml) was removed to a new tube before mixing with distilled water (1.25 ml) and 0.1% of ferric chloride (0.25 ml). The reduce activity of each sample was determined by the absorbance at 700 nm. All determinations were performed in five replications.
Syntheses of AgNPs
The reaction was carried out in a total volume of 220 ml, containing 190 ml of extracted sericin (derived from B. mori or S. c. ricini) at 0.15 mg/ml and 10 ml of 1 M AgNO3. The reaction might contain or not contain 20 ml of 2 M glucose as an additional reducing agent. The mixture was continuously stirred at 60 °C for 24 h under light protection. The formation of AgNPs was monitored by the changed color to yellow and the surface plasmon resonance (SPR) band by measuring the absorbance at 300–900 nm using a UV–Vis spectrophotometer (Jena, Germany). To study the effect of protein concentrations, the reactions that contained 10 ml of 1 M AgNO3 and 190 ml of different protein concentrations (0.05, 0.10, 0.15, and 0.20 mg/ml) with and without the presence of glucose, were incubated at 60 °C for 24 h. To study a formation of AgNPs in a time course of 48 h, the similar reaction containing the optimal concentration of the protein, AgNO3, and glucose was prepared. The reaction at 0, 6, 12, 24, and 48 h was aliquot to measure at the absorbance at 300–900 nm.
Characterization of the synthesized AgNPs
The morphology and size of AgNPs were determined from the transmission electron microscope (TEM) images using a Tecnai G2 20S-Twin (FEI, Hillsboro, OR) microscope at an accelerating voltage of 200 kV. The suspension of AgNPs (10 μl) was dropped on a carbon-coated copper grid and dried at room temperature prior to TEM analysis. The average size of AgNPs was determined from 120 particles of TEM images using an image J software (National Institutes of Health, USA). The histogram of size distribution was plotted using OriginPro 8.0 (OriginLab Corporation, Northampton, MA) software.
The identity of the synthesized AgNPs was determined by energy-dispersive X-ray (EDX), selected area electron diffraction (SAED) and X-ray diffraction (XRD) analyses. The element chemical composition of the synthesized AgNPs was analyzed by an EDX spectroscopy equipped on TEM with an EDAX r-TEM SUTW detector (FEI) operating at an accelerating voltage of 10 kV. The crystalline nature of the synthesized AgNPs was characterized by a SAED pattern using TEM, which operated at 200 kV with a LaB6 filament. Images were recorded by a Gatan Orius 200 CCD Camera (Gatan, Inc., Houston, TX). The lattice fringe spacing of the AgNPs was measured using an image J software. The crystallinity of the synthesized AgNPs was investigated by XRD spectrometer (D8 Advance, Bruker (UK) Ltd, Coventry, UK) with Cu Kα radiation (λ = 1.5418 Å) operating at 40 kV and 40 mA. The diffracted intensities were recorded from 30 to 80° of 2θ angles. The sample was coated on a glass coverslip and dried at room temperature prior to use.
Evaluation of antibacterial activity
The antibacterial activity of the synthesized AgNPs was evaluated by the standard broth microdilution method [Citation14] to determine the minimum inhibitory concentration (MIC) and the minimum bactericidal concentration (MBC) against Gram-positive (Staphylococcus aureus, ATCC 25923) and Gram-negative (Escherichia coli, ATCC 25922) bacteria. The suspension of AgNPs was serially two-fold diluted by MH broth. The suspension of AgNPs (100 μl) was mixed with the tested bacteria (100 μl) at a concentration of 5 × 105 colony-forming units/ml (CFU/ml) in each well of a 96-well plate. The cultures were incubated at 37 °C with shaking at 80 rpm. Bacterial growth was examined by measuring the optical density (OD) at 600 nm. The MIC was determined by the lowest concentration of the AgNPs that visually inhibited 99% growth of the microorganisms, seen as a transparent culture without turbidity of bacterial cells. To determine the MBC, the aliquots (100 μl) of the bacterial cultures at the MIC value and two higher concentrations were spread on MH agar plates. After incubating at 37 °C for 24 h, the number of the colony grown on each agar plate was counted. The MBC value was determined by the lowest concentration of AgNPs killing 100% of the initial bacterial population.
Statistical analysis
All of the quantitative data were expressed as mean ± standard deviation (SD). Statistical comparisons were performed using one-way analysis of variance (ANOVA) with SPSS 11.5 for Windows software (SPSS Inc., Chicago, IL). The p values of <.05 was considered statistically significant.
Results and discussion
The extracted sericins of S. c. ricini and B. Mori
Silk cocoons of S. c. ricini and B. mori were extracted in distilled water to obtain sericins. The extraction yielded 4.05 ± 2.44 and 8.56 ± 1.47 mg sericins per 1 g silk cocoon, respectively. Although the extraction of sericins by the method using 20 mM sodium carbonate can give a higher yield, the extraction process is time-consuming due to a requirement of dialysis for at least 72 h to remove salts [Citation15]. In this work, sericins extracted by boiling water was used without a dialysis step for a synthesis of AgNPs. The extracted sericin proteins were size-separated on a 4–15% gradient SDS–PAGE gel as seen in . For S. c. ricini sericin, faint protein bands of 18, 22, and 27 kDa were observed, while most proteins were observed as smear bands, suggesting their partial degradation. Similarly, the faint protein bands of 13, 22, 26, 32, and 122 kDa and smear proteins of B. mori sericin were also observed. Similar to other works, the extraction of B. mori sericin by water resulted in smear bands of proteins, while the extraction by urea yielded the intact proteins [Citation16].
Figure 1. Images of (a) the extracted sericins of S. c. ricini and B. mori visualized on a 12.5% SDS–PAGE gel and (b) their reducing activity.
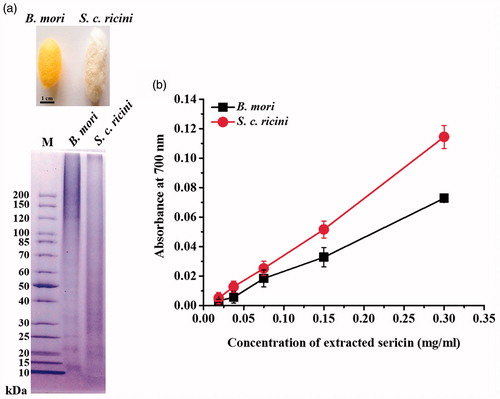
Reducing activities of B. mori and S. c. ricini sericins are illustrated in . The reducing power of both sericins was increased according to the increased protein concentrations, indicating their potential activity as a reducing agent for a synthesis of AgNPs. In addition, the reducing activity of S. c. ricini sericin was significantly higher than that of B. mori sericin. The reducing capacity of a compound suggests its ability to donate electrons to other compounds and reduce them into more stable forms [Citation17].
Green synthesis of AgNPs using sericins
To determine its reducing and stabilizing functions, the formation of AgNPs was studied using the reactions containing sericins (S. c. ricini or B. mori) and AgNO3 in a presence and absence of glucose as the additional reducing agent. shows the formation of AgNPs in the reactions containing sericins in the presence and absence of glucose, as indicated from light yellow to brown color of the reactions. However, the obvious SPR peak of silver was detected only in the reactions containing glucose and both sericins, suggesting that the reducing activity of the extracted sericins was not sufficient to promote a high formation of AgNPs in 24 h. The maximum SPR peaks of the reactions using S. c. ricini and B. mori sericins were 419 and 408 nm, respectively, whereas no SPR peak was detected in the reactions contained AgNO3 alone and AgNO3 with glucose. In addition, the S. c. ricini sericin significantly induced the greater formation of AgNPs than the B. mori sericin as determined by its higher intensity of SPR peak.
Figure 2. UV–Vis spectra of AgNPs synthesized by using (a) sericin of S. c. ricini and (b) sericin of B. mori.
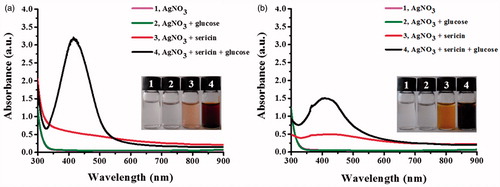
shows the UV–Vis spectra of the formation of AgNPs under the conditions using different concentrations (50–200 μg/ml) of S. c. ricini and B. mori sericins. With increasing sericin concentrations, more AgNPs were produced as determined by the increased SPR intensity. Similar to the previous observation, the production of AgNPs was higher in the reactions using the S. c. ricini sericin.
Figure 3. UV–Vis spectra of the synthesized AgNPs using different concentrations of (a) sericin of S. c. ricini and (b) sericin of B. mori.
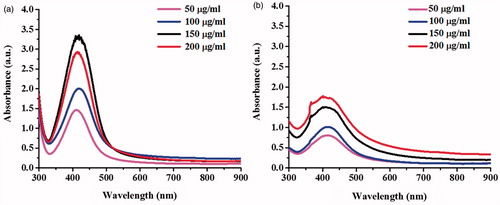
The formation of AgNPs was also monitored in a time course of 48 h (). With increasing incubation times, the formation of AgNPs was increased as determined by the increased intensity of SPR peak. The greater formation of AgNPs in the reaction using sericin of S. c. ricini was also observed in these reactions. As the reducing activity of sericin of S. c. ricini was greater than that of B. mori, it might contribute to the greater production of AgNPs. The content of reducing amino acids (phenylalanine, cysteine, tyrosine, and histidine) of S. c. ricini sericin was reported of 7.88%, while that of B. mori sericin was only 2.21% [Citation18,Citation19]. In addition, the extracted sericin contained the functional groups of cysteine, tyrosine and histidine, and the phenolic compounds that could contribute to a reduction of Ag+ to Ag0 [Citation20].
Characterization of the synthesized AgNPs
The produced AgNPs of the reactions using sericins of S. c. ricni and B. mori were characterized and compared. TEM images revealed that AgNPs from both reactions had similar morphology and size (). The synthesized AgNPs derived from sericins of S. c. ricini and B. mori were predominantly spherical-shaped particles with the average diameters of 13.6 ± 5.1 nm and 13.3 ± 6.5 nm, respectively. The sizes of AgNPs derived from S. c. ricini sericin was ranged from 5.5 to 30.5 nm, while those of B. mori sericin was ranged from 2.4 to 38.1 nm. The good dispersion of AgNPs as seen in TEM images were likely resulted from the negative charges of sericin surrounding the particles [Citation10].
Figure 5. TEM images of AgNPs synthesized by using (a) sericin of S. c. ricini and (b) sericin of B. mori.
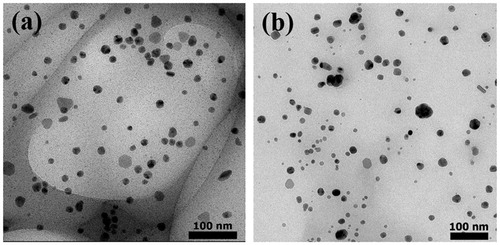
The identity of the produced AgNPs was further confirmed by TEM-SAED, TEM-EDX and XRD analyses. Similar analysis results were obtained by the produced AgNPs using both sericins, which the representative data of the produced AgNPs using S. c. ricini sericin are presented in . shows the TEM-SAED result, which the produced AgNPs exhibited the bright circular rings corresponding to the plane families (1 1 1) and (3 1 1) of pure face-centered cubic (fcc) silver structure according to JCPDS, file no. 4–0787 [Citation21]. shows the elemental composition profile of the produced AgNPs, which revealed the major silver component. The trace detection of oxygen and copper atoms was likely from the atmosphere and the carbon-coated copper grid [Citation22]. The observed signal from silicon atom was possibly due to elements from a glass sample holder [Citation23]. shows the XRD analysis of the produced AgNPs, which demonstrated four diffraction peaks at 2θ values (38.11°, 44.29°, 64.41°, and 77.38°), corresponding to planes of (1 1 1), (2 0 0), (2 2 0), and (3 1 1) of the face-centered cubic (fcc) lattice of silver (JCPDS file no. 04–0783), respectively [Citation24]. Some unassigned peaks of XRD patterns were also detected, which were likely due to the organic compounds occurring on the surface of the AgNPs [Citation25].
Antibacterial activity of the synthesized AgNPs
The antibacterial activity of the synthesized AgNPs against E. coli and S. aureus, the representative Gram-negative and Gram-positive bacteria, was investigated. shows the growth curves of E. coli and S. aureus in response to AgNPs synthesized by using S. c. ricini sericin, which the antibacterial activity of AgNPs against both bacteria was dose dependent. The MIC and MBC against E. coli were determined at 15.60 μg/ml and 62.50 μg/ml, respectively, and those against S. aureus were 31.25 μg/ml and 62.50 μg/ml, respectively. Similarly, AgNPs produced by using B. mori sericin exhibited the antibacterial activity against both bacteria in a dose-dependent manner (data not shown). Their MIC and MBC against E. coli were 6.25 μg/ml and 12.50 μg/ml, respectively, while those against S. aureus were 12.50 μg/ml and 25.00 μg/ml, respectively. The proposed bactericidal mechanism of AgNPs is via a disruption of membrane permeability, interference of cell division, interruption of protein and enzyme functions, and induction of oxidative stress-induced apoptosis [Citation26]. In addition, the different sensitivity of Gram-negative and Gram-positive bacteria to AgNPs is possibly due to the different thickness of the peptidoglycan layer in their cell wall [Citation27]. With thinner cell wall, the Gram-negative bacteria are more susceptible to AgNPs [Citation28].
Conclusions
This work reported the green synthesis of AgNPs using the aqueous extract of sericin of non-mulberry silkworm (S. c. ricini) compared to sericin of mulberry silkworm (B. mori). Despite the differences in amino acid composition, both sericins facilitate a formation of spherical AgNPs with a similar size of ∼13 nm, but at different efficacies. The identity of the synthesized AgNPs was confirmed by TEM-SAED, TEM-EDX and XRD analyses. This proposed green synthesis method was simple, rapid and eco-friendly. Furthermore, the synthesized AgNPs showed potent antibacterial activity against both E. coli and S. aureus, suggesting their potential application as an antibacterial agent.
Acknowledgements
Funding of this work is supported by Suranaree University of Technology-National Research Fund (Grant No. SUT-1–104-57–24-20).
Disclosure statement
No potential conflict of interest was reported by the authors.
Additional information
Funding
References
- Liu C, Wang L, Xu H, et al. “One pot” green synthesis and the antibacterial activity of g-C3N4/Ag nanocomposites. Mater Lett. 2016;164:567–570.
- Verma DK, Hasan SH, Banik RM. Photo-catalyzed and phyto-mediated rapid green synthesis of silver nanoparticles using herbal extract of Salvinia molesta and its antimicrobial efficacy. J Photochem Photobiol B. 2016;155:51–59.
- Rafique M, Sadaf I, Rafique MS, et al. A review on green synthesis of silver nanoparticles and their applications. Artif Cells Nanomed Biotechnol. 2016 [cited 2017 May 11]:[20 p.]. DOI: 10.1080/21691401.2016.1241792.
- Lu R, Yang D, Cui D, et al. Egg white-mediated green synthesis of silver nanoparticles with excellent biocompatibility and enhanced radiation effects on cancer cells. Int J Nanomed. 2012;7:2101–2107.
- Ghodake G, Lim S-R, Lee DS. Casein hydrolytic peptides mediated green synthesis of antibacterial silver nanoparticles. Colloids Surf B Biointerfaces. 2013;108:147–151.
- Singh AV, Bandgar BM, Kasture M, et al. Synthesis of gold, silver and their alloy nanoparticles using bovine serum albumin as foaming and stabilizing agent. J Mater Chem. 2005;15:5115–5121.
- Fei X, Jia M, Du X, et al. Green synthesis of silk fibroin-silver nanoparticle composites with effective antibacterial and biofilm-disrupting properties. Biomacromolecules. 2013;14:4483–4488.
- Kumar, U, Ranjan, AK, Sharan, C, et al. Green approach towards size controlled synthesis of biocompatible antibacterial metal nanoparticles in aqueous phase using lysozyme. CNANO. 2012;8:130–140.
- Lamboni L, Gauthier M, Yang G, et al. Silk sericin: a versatile material for tissue engineering and drug delivery. Biotechnol Adv. 2015;33:1855–1867.
- Bhat PN, Nivedita S, Roy S. Use of sericin of Bombyx mori in the synthesis of silver nanoparticles, their characterization and application. Indian J Fibre Text Res. 2011;36:168–171.
- Yue X, Lin H, Yan T, et al. Synthesis of silver nanoparticles with sericin and functional finishing to cotton fabrics. Fibers Polym. 2014;15:716–722.
- Aramwit P, Bang N, Ratanavaraporn J, et al. Green synthesis of silk sericin-capped silver nanoparticles and their potent anti-bacterial activity. Nanoscale Res Lett. 2014;9:79.
- Ferreira ICFR Baptista P, Vilas-Boas M, et al. Free-radical scavenging capacity and reducing power of wild edible mushrooms from northeast Portugal: individual cap and stipe activity. Food Chem. 2007;100:1511–1516.
- Khamhaengpol A, Siri S. Fluorescent light mediated a green synthesis of silver nanoparticles using the protein extract of weaver ant larvae. J Photochem Photobiol B. 2016;163:337–344.
- Chirila TV, Suzuki S, McKirdy NC. Further development of silk sericin as a biomaterial: comparative investigation of the procedures for its isolation from Bombyx mori silk cocoons. Prog Biomater. 2016;5:135–145.
- Aramwit P, Kanokpanont S, Nakpheng T, et al. The effect of sericin from various extraction methods on cell viability and collagen production. Int J Mol Sci. 2010;11:2200–2211.
- Bursal E, Köksal E. Evaluation of reducing power and radical scavenging activities of water and ethanol extracts from sumac (Rhus coriaria L.). Food Res Int. 2011;44:2217–2221.
- Komatsu K, Yamada M. Chemical studies on sericin. II. Amino acid composition of wild and domestic cocoon sericins. J Seric Sci Jpn. 1975;44:105–110.
- Mirsky AE, Anson ML. The reducing groups of proteins. J Gen Physiol. 1936;19:451–459.
- Fan J-B, Wu L-P, Chen L-S, et al. Antioxidant activities of silk sericin from silkworm Bombyx mori. J Food Biochem. 2009;33:74–88.
- Talib A, Hui Fen W. Biomimetic synthesis of lotus leaf extract-assisted silver nanoparticles and shape-directing role of cetyltrimethylammonium bromide. J Mol Liq. 2016;220:795–801.
- Korbekandi H, Mohseni S, Mardani Jouneghani R, et al. Biosynthesis of silver nanoparticles using Saccharomyces cerevisiae. Artif Cells Nanomed Biotechnol. 2016;44:235–239.
- Varadavenkatesan T, Selvaraj R, Vinayagam R. Phyto-synthesis of silver nanoparticles from Mussaenda erythrophylla leaf extract and their application in catalytic degradation of methyl orange dye. J Mol Liq. 2016;221:1063–1070.
- Dong C, Zhang X, Cai H, et al. Green synthesis of biocompatible silver nanoparticles mediated by Osmanthus fragrans extract in aqueous solution. Optik. 2016;127:10378–10388.
- Ibrahim HMM. Green synthesis and characterization of silver nanoparticles using banana peel extract and their antimicrobial activity against representative microorganisms. J Radiat Res Appl Sci. 2015;8:265–275.
- Singh H, Du J, Yi T-H. Green and rapid synthesis of silver nanoparticles using Borago officinalis leaf extract: anticancer and antibacterial activities. Artif Cells Nanomed Biotechnol. 2016 [cited 2017 May 11]:[7 p.]. DOI: 10.1080/21691401.2016.1228663.
- Moteriya P, Chanda S. Synthesis and characterization of silver nanoparticles using Caesalpinia pulcherrima flower extract and assessment of their in vitro antimicrobial, antioxidant, cytotoxic, and genotoxic activities. Artif Cells Nanomed Biotechnol. 2016 [cited 2017 May 11]:[12 p.]. DOI: 10.1080/21691401.2016.1261871.
- Salari Z, Danafar F, Dabaghi S, et al. Sustainable synthesis of silver nanoparticles using macroalgae Spirogyra varians and analysis of their antibacterial activity. J Saudi Chem Soc. 2016;20:459–464.