Abstract
Candida species are pleomorphic, commensal fungi associated with candidiasis. The extracellular hydrolytic-secreted aspartyl proteinases are recognized as chief agents for pathogenesis of Candida species, involved in the degradation of proteins and adhesion during biofilm formation. This study aimed at exploring inhibitory effect of mycogenic silver nanoparticles (Ag NPs) against C. albicans and non-albicans’ biofilm growth and aspartyl proteinase enzyme activity in-vitro. Biofilm forming, drug-resistant clinical isolates of C. albicans (n = 25) and non-albicans (n= 20) were assessed for their ability to reduce the metabolic and aspartyl proteinase activities using XTT assay and spectrophotometric analysis at different concentrations of mycogenic Ag NPs. After 24 h of incubation, significant reduction (>50%) in metabolic activity was observed with 100 ppm mycogenic Ag NPs. Incubation time has greater inhibitory effect against Candida spp. biofilms secreted aspartyl proteinase after treatment with 100 ppm mycogenic Ag NPs. Inhibition of secreted aspartyl proteinase by mycogenic Ag NPs provides an insight towards the mechanism for the treatment of Candida-associated infections involving biofilms-related infections.
Introduction
Candidiasis, in majority of the cases is caused by Candida albicans. Among non-albicans, C. tropicalis, C. glabrata, C. parapsilosis species are often associated with candidiasis [Citation1]. Candida spp. have also developed a battery of putative virulence factors which include adherence to host tissues, yeast hyphal transitions, and secretion of hydrolytic enzymes especially secretion of proteinases and phospholipases known to be frequently associated with pathogenicity [Citation2]. Secreted aspartyl proteinases (S aspartyl proteinases) by Candida spp. have been demonstrated to be one of the virulence determinants [Citation3]. S aspartyl proteinases are encoded by family of SAP genes (SAP1–SAP10) which are associated with virulence in Candida spp. Mainly, secreted aspartyl proteinases are involved in the degradation of proteins but their role in the adhesion of Candida to mucosal surfaces is also recognized [Citation3]. Elevated levels of S aspartyl proteinases are released during biofilm formation in comparison to the planktonic form of Candida spp. [Citation4]. Among different SAPs, SAP 4–6, are mostly expressed during formation of hypha, the predominant structure during biofilm growth. Whereas, SAP 9–10 encoded proteins are involved in maintaining the cell integrity by processing cell wall proteins thereby facilitate biofilm formation [Citation5]. Most prominently, S aspartyl proteinase enzyme is the key factor of C. albicans biolfilms but the SAP genes’ expression in other non-albicans including C. tropicalis, and C. parapsilosis is also reported [Citation6–8]. Similarly, complex biofilm structures confer biofilms with more resistance than the planktonic forms of the pathogens [Citation9].
Biological synthesis of metallic nanoparticles (NPs) using fungal isolates has found significantly more advantages compared to other microorganisms [Citation10]. One of the main advantages of involving fungi for NPs synthesis as compared to bacteria, is ease of cultivation at large scale associated with an increase in biomass resulting in an increased secretion of metabolites typically extracellular enzymes that aid in NPs’ synthesis [Citation11].
Recently, the role of silver nanoparticles (Ag NPs) is observed for gaining much importance in controlling fungal biofilm infestation [Citation12]. The green synthesis of Ag NPs with antibacterial and activity against bacterial isolates and C. albicans is reported in near past. Furthermore, Ag NPs activity against bacterial biofilm was also reported [Citation13]. Mycogenic silver nanoparticles were recently used for deterrence in metabolic and biofilms forming activity of clinical Candida species [Citation14]. It can be speculated that differential SAP genes’ expression during biofilm formation is linked to the biofilm associated infections caused by Candida spp. In present study, we aim to evaluate for the first time the potent anti-biofilm activity displayed by mycogenic Ag NPs y inhibition of S aspartyl proteinases’ secretion. In recent studies, triangular gold nanoparticles (Au NPs) have demonstrated their efficacy against planktonic C. albicans by inhibition of aspartyl proteinase activity [Citation15,Citation16]. Inhibition of S aspartyl proteinase by Ag NPs provides an insight towards the treatment of Candida associated infections typically biofilms-related infections.
Materials and methods
Mycogenic Ag NPs’ synthesis
Mycogenically synthesized Ag NPs using Aspergillus spp. were obtained from the Microbiology Research Laboratory at Quaid-i-Azam University, Islamabad, Pakistan. The AgNPs were characterized previously using UV-Vis spectroscopy, X-Ray Diffraction (XRD) and Transmission Electron Microscopy (TEM) [Citation17]. A 1000 ppm stock solution of Ag NPs was prepared by dissolving 1 mg of synthesized Ag NPs in 1 ml of autoclaved deionized water. Before use, this suspension was sonicated for approximately 20 min in order to make proper suspension and to avoid Ag NPs’ accumulation.
Microorganisms
Biofilm forming clinical isolates of C. albicans (n = 25) and non-albicans (n = 21), isolated from different clinical specimens were included in the study. The non-albicans include C. glabarata, C. krusei, C. parapsilosis and C. tropicalis. These isolates were resistant against amphotericin B, fluconazole and voriconazole.
Inhibitory effect of Mycogenic Ag NPs against Candida spp. Biofilms’ metabolic activity
Candida spp. biofilms were developed in 90 well polystyrene microtiter plates (Corning Inc., Corning, NY) using SDB (sabouraud dextrose broth) supplemented with 10% glucose. The overnight fungal cell cultures (OD600 of 0.1) were treated with Ag NPs of 25 to 100 ppm concentration. Hundred microliters of the suspension was added to each well of microtiter plates, and incubated at 37 °C for 24 h, subsequently washed with PBS. Inhibition in the metabolic activity of Candida spp. biofilms by mycogenic Ag NPs was tested by XTT assay [Citation18]. The mixture of Candida spp. cell suspension with Ag NPs was cultured at 37 °C for 24 h. The assay was performed in duplicate.
Evaluation of mycogenic Ag NPs against S-aspartyl proteinase
For the evaluation of S aspartyl proteinase activity, spectrophotometric analysis was performed with slight modification on Candida spp. biofilms grown before 24 and 48 h. The bovine serum albumin (BSA) was utilized as a substrate for enzyme activity’s evaluation. The effect of Ag NPs on the S aspartyl proteinase activity was evaluated by culturing biofilm forming Candida spp. in 5 ml yeast peptone dextrose (YPD) medium. Inoculation was followed by incubation for 24 and 48 h at 37 °C in the glass tubes. After incubation, biofilms were washed with PBS twice and centrifugation at 5000 rpm for 5 min. The cell suspension (107 cells/ml) was inoculated in 5 ml of YPD medium. 200 μL of 100 ppm mycogenic Ag NPs were added to medium containing Candida cell suspension and incubated at 37 °C for 24 and 48 h, respectively. After incubation, 0.1 ml of medium was transferred to other Eppendorf and 0.4 ml of 0.1 M sodium citrate buffer (pH 3.2) containing BSA 1% w/v was added and incubated for 15 min at 37 °C. After 15 min, 0.5 ml of 5% TCA was added to stop the reaction. The mixture was centrifuged at 3000 g for 10 min and OD was measured at 280 nm against distilled water. Enzyme activity was estimated as an amount of tyrosine released (μM) per min per ml in the culture supernatant. Culture without Ag NPs served as negative control [Citation15].
Statistical analysis
SPSS software was utilized (SPSS 16.0, Statistical Package for the Social Sciences, Inc., Chicago, IL) for evaluating the efficacy of Ag NPs against Candida biofilms and S aspartyl proteinase activity. The statistical tests used include student’s t-test and one-way ANOVA for analysis.
Results
The effect of mycogenic Ag NPs on C. albicans biofilms was determined using different Ag NPs concentrations. The Candida spp. biofilm formation was significantly inhibited that varied between <20% to >70% after treatment with different doses of Ag NPs. At 25 ppm, biofilm inhibition was approx. 20%. The increase in the concentration of Ag NPs to 100 ppm reduced the biofilm growth to >70% (. Thus, 100 ppm Ag NPs concentration was selected for further investigation.
Figure 1. %inhibition of C. albicans biofilm (24 h) at different concentrations of Ag NPs. Error bars represent % error.
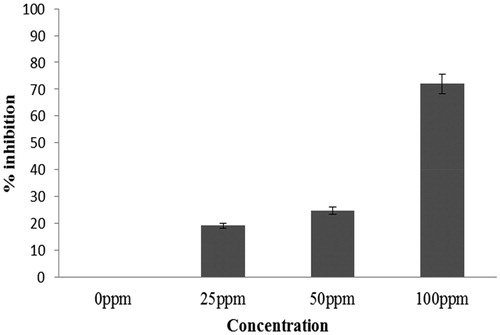
Quantification of biofilm metabolic activity
In C. albicans and non-albicans, inhibition in metabolic activity was observed by mycogenic Ag NPs against 24 h old biofilms. Overall, >50% inhibition in metabolic activity was found among C. albicans (n = 16). However, the highest inhibition in metabolic activity in C. albicans (CA 29) was shown to be 87% at 100 ppm Ag NPs (). The reduction in metabolic activity >50% was observed in non-albicans (n = 11). The reduction in metabolic activity was observed as highest in C. glabarata 83% (CG 55) followed by 80% (CK 117) C. krusei, 74% (CP 31) C. parapsilosis and 56% (CT 51) C. tropicalis ().
Figure 2. (A) Reduction in metabolic activity of Candida albicans (n = 25) biofilm after treatment with mycogenic AgNPs at 24 h by XTT assay. Error bars represent standard deviation from the mean. (B) Reduction in metabolic activity (%age) of non-albicans spp. (n = 20) biofilm (24 h) after treatment with mycogenic AgNPs by XTT assay. Error bars represent standard deviation from the mean.
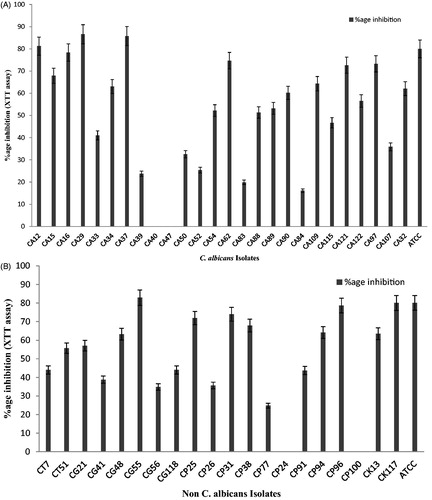
Effect of Mycogenic Ag NPs on S Aspartyl Proteinase activity at 24 h and 48 h Candida spp. Biofilms
The impact of 100 ppm of mycogenic Ag NPs on biofilm growth was further investigated by S aspartyl proteinase activity analysis at 24 and 48 h. Statistical analysis indicated significant inhibition (p < .05) in S aspartyl proteinase activity after interaction of Ag NPs with C. albicans and non-albicans biofilms. The inhibition in enzyme was increased at 48 h compared to 24 h during biofilm growth. Inhibition in S aspartyl proteinase secretion varied within the range of 54 μM to 76 μM at 24 h during C. albicans biofilm growth (). Reduction was not observed in CA115. S aspartyl proteinase reduction in term of tyrosine equivalent release was reported 108 μM (CA115), 68 μM (CA62), 67 μM (CA72) and 64 μM (CA84), respectively in 48 h old C. albicans biofilms after 100 ppm mycogeinc Ag NPs treatment ().
Figure 3. (A) Effect of mycogenic AgNPs on the secreted aspartyl proteinase activity of clinical isolates of biofilm forming C. albicans (24 h). Error bar represents %age error. (B) Effect of mycogenic AgNPs on the secreted aspartyl proteinase activity of clinical isolates of biofilm forming C. albicans (48 h). Error bar represents %age error.
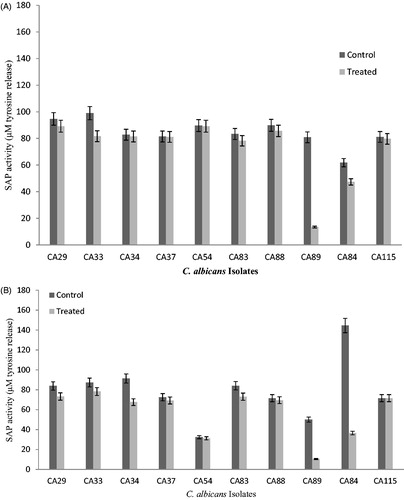
Among non-albicans, inhibition of S aspartyl proteinase secreted from the cells in a biofilms varied significantly after interaction with mycogenic Ag NPs. In non-albicans, highest inhibition in S aspartyl proteinase activity was observed to be 89 μM of tyrosine release in (CP25) followed by 79 μM in (CG 48) and 55 μM (CT7) at 24 h of biofilms. However, an increase in the S aspartyl proteinase releases was also reported in non-albicans isolates which was 5 μM (CP 94) and 1 μM (CP100) after Ag NPs treatment in 24 h biofilm (). The treatment of 48 h old non-albicans biofilms also showed reduction in S aspartyl proteinase activity. The maximum inhibition was 203 μM (CP77) and 129 μM (CT7), respectively, after 48 h of Ag NPs treatment compared to the controls (). Candida glabrata showed minimum inhition in S aspartyl proteinase activity at 48 h old biofilms compared to other non-albicans isolates.
Figure 4. (A) Effect of mycogenic AgNPs on the secreted aspartyl proteinase activity of clinical isolates of biofilm (24 h) forming non-albicans spp. Error bars showed %age error. (B) Effect of mycogenic AgNPs on the secreted aspartyl proteinase activity of clinical isolates of biofilm (48 h) forming non-albicans spp. Error bars showed %age error.
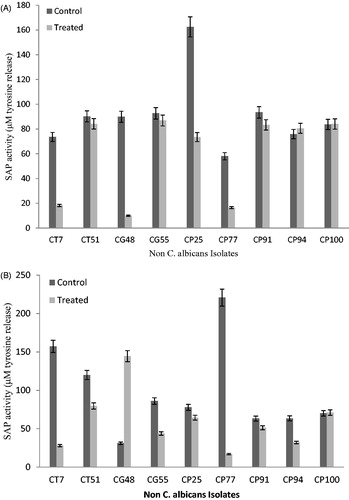
Discussion
Due to the increase in emergence of drug resistance, the Candida associated infections are on continual rise. There is a dire need for the new agents that can be used for the treatment. For this purpose, this study utilized the mycogenic Ag NPs as therapeutics against drug resistant clinical isolates of C. albicans and non-albican species. As biofilm is considered an important factor for pathogenesis, potential of Ag NPs against biofilm formation was evaluated. Furthermore, S aspartyl proteinase is also a key enzyme associated with virulence, thus, effect of the mycogenic Ag NPs against secreted aspartyl proteinase during biofilm formation was evaluated for the first time for limiting Candida spp. biofilms growth and associated infections.
The method of nanoparticles’ synthesis used in this study was biological, i.e. using Aspergillus spp, and C. albicans isolates were found to be susceptible to biologically synthesized Ag NPs with an average inhibition of >70% (. Previous studies have also reported similar findings of biofilm inhibition with Ag NPs but with varied concentration of NPs applied thus affecting the degree of inhibition [Citation16,Citation19,Citation20]. For the synthesis of Ag NPs, biological method poses certain advantages over physical and chemical methods which include safety, stability, polydispersity, reducing the requirement of stabilizing agents such as agents used for capping [Citation17,Citation21]. Thus it can be inferred that difference in the inhibitory effect of Ag NPs may be due to the different parameters of Ag NPs have an implication in degree of inhibition which includes synthesis method, size and concentration. Furthermore, certain experimental conditions such as temperature, incubation period, pH, type of media used for microbial growth have role in the varied results [Citation18,Citation22,Citation23].
Candida albicans, C. parapsilosis, and C. tropicalis displayed proteolysis in this study as reported by previous study but the degree of S aspartyl proteinase production is different among different isolates [Citation24]. However, C. glabrata is also reported to produce S aspartyl proteinase in this study along with C. albicans, C. parapsilosis, and C. tropicalis. S aspartyl proteinase is regarded as key factor that contribute to candida infection that facilitates microbial invasion by damaging mucosa [Citation3].
Previously, Au NPs have been shown to exhibit significant inhibition against C. albicans biofilms and S aspartyl proteinase. The lowest S aspartyl proteinase activity was observed at 64 ppm against planktonic strains after 48 h of treatment [Citation15,Citation25,Citation26]. However, the study used C. albicans isolates associated with vaginal candidiasis whereas present study incorporated C. albicans and non-albicans associated with candidiasis from different sources. The inhibition in S aspartyl proteinase activity of biofilms treated with 100 ppm mycogenic Ag NPs was 68 μM (CA62), 67 μM (CA72) and 64 μM (CA84), respectively after 48 h. S aspartyl proteinase comprises of conserved aspartyl residues in the active site and also cysteine for structural integrity [Citation27]. It can be speculated that Ag NPs act against aspartyl proteinase can be due to an interaction of Ag NPs with either aspartyl residues within active site or the conserved cysteine residues thereby blocking active site or altering structural integrity.
Till date, data is not available regarding the effect of Ag NPs on S aspartyl proteinase activity of biofilm forming clinical isolates of non-albicans species. In this study, reduction in S aspartyl proteinase activity of biofilm forming clinical isolates of non-albicans was observed with mycogenic Ag NPs treatment after 24 h and 48 h () with slight variation. C. glabrata showed minmum inhition in S aspartyl proteinase activity at 48 h of biofilm formation. After 48 h, Ag NPs treated biofilms (CG 48 and CP100) showed higher S aspartyl proteinase activity compared to the controls. There might be different possibilities for the variation in S aspartyl proteinase activities against C. albicans and different non-albicans spp. which include C. glabrata, C. tropicalis, C. parapsilosis. Sequence variations in S aspartyl proteinase enzyme protein sequnce might also be responsible for different inhibitory responses among C. albicans and non-albicans and even within different species of non-albicans. Insertion and deletion of sequence modifies the primary secreted aspartyl proteinases sequence ultimately modifying the enzyme structure which then alters the substrate-binding capacity and specificity of enzymes [Citation28]. Another possible mechanism associated with the inhibition is that Candida cells in biofilms recognized Ag NPs as stress signals and thus can activate the quorum sensing mechanism. Farnesol, a quorum sensing molecule inhibits biofilm formation by modulating various morphological changes in Candida biofilms along with the down regulation of different S aspartyl proteinases mRNA expression thus anti-biofouling effect is produced [Citation29].
The results of the study revealed critically that time of exposure of biofilms to Ag NPs have implications in its inhibitory effect. The mycogenic Ag NPs are significantly associated with the inhibitory effect against metabolic activity of Candida spp. biofilms. The significant inhibition in the S aspartyl proteinase secreted by the Candida cells in a biofilm was also observed. Ag NPs provide to be potent agent for reducing Candida spp. associated infections in a dose response manner. The targets of mycogenic Ag NPs are numerous ranged from biofilm growth to enzyme inhibition. Further studies are needed to address the different parameters like time of exposure, pH and temperature affecting the Ag NPs activity against S aspartyl proteinase before using as medication with greater sample size.
Acknowledgements
The authors would like to thank the Department of Microbiology, Quaid-i-Azam University, Islamabad, Pakistan for providing the funding and facilities to carry out the research.
Disclosure statement
No potential conflict of interest was reported by the author(s).
Additional information
Funding
References
- Filler SG, Sheppard DC. Fungal invasion of normally non-phagocytic host cells. PLoS Pathog. 2006; 2:e129.
- Khatoon N, Mishra A, Alam H, et al. Biosynthesis, characterization, and antifungal activity of the silver nanoparticles against pathogenic Candida species. BioNanoSci. 2015;5:65–74.
- Naglik JR, Challacombe SJ, Hube B. Candida albicans secreted aspartyl proteinases in virulence and pathogenesis. Microbiol Mol Biol Rev. 2003;67:400–428.
- Řičicová M, Kucharíková S, Tournu H, et al. Candida albicans biofilm formation in a new in vivo rat model. Microbiology. 2010;156:909–919.
- Joo MY, Shin JH, Jang H-C, et al. Expression of SAP5 and SAP9 in Candida albicans biofilms: comparison of bloodstream isolates with isolates from other sources. Med Mycol. 2013;51:892–896.
- De Viragh PA, Sanglard D, Togni G, et al. Cloning and sequencing of two Candida parapsilosis genes encoding acid proteases. Microbiology. 1993;139:335–342.
- Zaugg C, Borg-von Zepelin M, Reichard U, et al. Secreted aspartic proteinase family of Candida tropicalis. Infect Immun. 2001;69:405–412.
- Kalkanci A, Bozdayi G, Biri A, et al. Distribution of secreted aspartyl proteinases using a polymerase chain reaction assay withSAP specific primers in Candida albicans isolates. Folia Microbiol. 2005;50:409.
- Nobile CJ, Johnson AD. Candida albicans biofilms and human disease. Annu Rev Microbiol. 2015;69:71–92.
- Narayanan KB, Sakthivel N. Biological synthesis of metal nanoparticles by microbes. Adv Colloid Interface Sci. 2010;156:1–13.
- Das K, Thiagarajan P. Mycobiosynthesis of metal nanoparticles. Int J Nanotechnol Nanosci. 2012;1:1–10.
- Wang H, Xie B. Study on how nanosilver-based inorganic antibacterial agent functions on biofilm formation of Candida albicans, inside the oral cavity. Artif Cells Nanomed Biotechnol. 2016;44:1429–1433.
- Singh P, Kim YJ, Wang C, et al. Biogenic silver and gold nanoparticles synthesized using red ginseng root extract, and their applications. Artif Cells Nanomed Biotechnol. 2016;44:811–816.
- Hamid S, Zainab S, Faryal R, Ali N, et al. Deterrence in metabolic and biofilms forming activity of Candida species by mycogenic silver nanoparticles. J Appl Biomed. 2017. https://doi.org/10.1016/j.jab.2017.02.003
- Haji Esmaeil HF, Jebali A, Hekmatimoghaddam S. The inhibition of Candida albicans secreted aspartyl proteinase by triangular gold nanoparticles. Nanomed J. 2015;2:54–59.
- Wady A, Machado AL, Zucolotto V, et al. Evaluation of Candida albicans adhesion and biofilm formation on a denture base acrylic resin containing silver nanoparticles. J Appl Microbiol. 2012;112:1163–1172.
- Naqvi SH, Zainab S, Hameed A, et al. Mycogenesis of silver nanoparticles by different Aspergillus species. Sci Iran. 2014;21:1143.
- Serrano-Fujarte I, López-Romero E, Reyna-López GE, et al. Influence of culture media on biofilm formation by Candida species and response of sessile cells to antifungals and oxidative stress. BioMed Res Int. 2015;2015:1–15.
- Monteiro DR, Takamiya AS, Feresin LP, et al. Susceptibility of Candida albicans and Candida glabrata biofilms to silver nanoparticles in intermediate and mature development phases. J Prosthodont Res. 2015;59:42–48.
- Vazquez-Muñoz R, Avalos-Borja M, Castro-Longoria E. Ultrastructural analysis of Candida albicans when exposed to silver nanoparticles. PLoS One. 2014;9:e108876.
- Ge L, Li Q, Wang M, et al. Nanosilver particles in medical applications: synthesis, performance, and toxicity. Int J Nanomedicine. 2014;9:2399.
- Chauhan NM, Shinde RB, Karuppayil SM. Effect of alcohols on filamentation, growth, viability and biofilm development in Candida albicans. Braz J Microbiol. 2013;44:1315–1320.
- Weerasekera MM, Wijesinghe GK, Jayarathna TA, et al. Culture media profoundly affect Candida albicans and Candida tropicalis growth, adhesion and biofilm development. Mem Inst Oswaldo Cruz. 2016;111:697–702.
- Dostál J, Hamal P, Pavlíčková L, et al. Simple method for screening Candida species isolates for the presence of secreted proteinases: a tool for the prediction of successful inhibitory treatment. J Clin Microbiol. 2003;41:712–716.
- Jebali A, Hajjar FHE, Hekmatimoghaddam S, et al. Triangular gold nanoparticles conjugated with peptide ligands: a new class of inhibitor for Candida albicans secreted aspartyl proteinase. Biochem Pharmacol. 2014;90:349–355.
- Yu Q, Li J, Zhang Y, et al. Inhibition of gold nanoparticles (AuNPs) on pathogenic biofilm formation and invasion to host cells. Sci Rep. 2016;6:26667.
- Jebali A, Hajjar FHE, Pourdanesh F, et al. Silver and gold nanostructures: antifungal property of different shapes of these nanostructures on Candida species. Med Mycol. 2014;52:65–72.
- Miranda I, Rocha R, Santos MC, et al. A genetic code alteration is a phenotype diversity generator in the human pathogen Candida albicans. PLoS One. 2007;2:e996.
- Décanis N, Tazi N, Correia A, et al. Farnesol, a fungal quorum-sensing molecule triggers Candida albicans morphological changes by downregulating the expression of different secreted aspartyl proteinase genes. Open Microbiol J. 2011;5:119–126.