Abstract
Mosquitoes act as key vector for transmission of devastating parasites and pathogens which affect millions of people globally. In this research, the green synthesis of silver nanoparticles of Cassia fistula fruit pulp as an innovative and operative tool against vector mosquitoes is presented. Silver nanoparticles were characterized by a series of techniques including Fourier transform infrared spectroscopy, Transmission Electron Microscope and confirmed by Scanning Electron Microscope, UV-Vis spectrophotometry and X-ray diffraction. Silver nanoparticles were highly effective against the larvae (I–IV instar) and pupae of Aedes albopictus and Culex pipiens pallens after 24, 48 and 72 h of treatment. Ae. albopictus had LC50 values ranging from 8.3 mg/L (I instar) to 17.3 mg/L (pupae) and LC50 ranging from 1.1 mg/L (I instar) to 19.0 mg/L (pupae) against Cx. pipiens pallens. The systemic effect of AgNPs was further assessed in the fourth instar larvae of Ae. albopictus and Cx. pipiens pallens by measuring the levels of total proteins and activity of two important marker enzymes: Acetylcholinesterase and α- and β-carboxylesterase. Overall, the findings of the study suggest that the use of Cassia fistula-fruit pulp extract mediated synthesis of silver nanoparticles can be used for controlling vector mosquitoes. This is the first report on the mosquito larvicidal and pupicidal activity of AgNPs synthesized by Cassia fistula fruit pulp and its possible mechanism of action.
Introduction
People may contract debilitating and life-threatening diseases due to bites from infected mosquitoes. As mosquito vectors are solely responsible for transmitting diseases such as malaria, dengue, chikungunya, Japanese encephalitis and lymphatic filariasis [Citation1,Citation2]. Among all, malaria remains a serious public health problem in many tropical and subtropical countries, affecting millions of individuals annually. In Southern China, major endemic cases were reported for falciparum malaria [Citation3]. The Asian tiger mosquito, Aedes albopictus, is highly anthropophilic and a day-biter mosquito which resides in peri-domestic habitats, serving as important vector of arboviruses throughout the world [Citation4]. Similarly, Culex mosquitoes are painful and persistent biters that are responsible for lymphatic filariasis (which is a neglected tropical disease). The infection occurs when filarial parasites are transmitted to humans through mosquitoes [Citation5,Citation6]. Currently, more than 1.4 billion people in 73 countries are living in areas where lymphatic filariasis is transmitted and a bigger population is at the risk of being infected [Citation2]. Furthermore, the recent outbreak of Zika virus infections, occurring in South America, Central America and the Caribbean, represents the most recent of four arrivals of important arboviruses in the Western Hemisphere. Zika virus follows dengue which slyly arrived in the hemisphere over decades and became more aggressive in the 1990s, West Nile virus emerged in 1999 and chikungunya emerged in 2013. Notably, there are no specific treatments for these arboviruses [Citation7].
The synthetic insecticides occupy leading position in mosquito control practices creating unwarranted ecological problems, developing physiological resistance in insects and causing environmental imbalances. The long use of insecticides against mosquito in China has been monitored. However, recently in Shandong province of China, insecticide resistance was observed in vector mosquito population [Citation8,Citation9]. In most cases, mosquito larvae targeted using organophosphates, insect growth regulators, microbial agents and insecticide-treated bed nets. In fact, these chemicals have a negative impact on human health and the environment. Hence, the development of new and safe tools to control mosquitoes, mainly the improvement of behavior-based strategy (sterile insect technique, “SIT”) and botanical products (mosquitocidal nanoparticles, MNP) are critically important. Few parts per million of different MNP are highly toxic against the malaria vector Anopheles stephensi, the dengue vector Aedes aegypti, and the filariasis mosquito Culex quiquefasciatus. However, moderate efforts have been carried out to draw attention to their possible non-target effects against mosquito’s natural enemies and other aquatic organisms [Citation10–12].
The synthesis of metal nanoparticles and their polymer composites have attracted great attention because of their unusual physical and chemical properties. In the field of nanotechnology, the most important Nano product is silver nanoparticles (AgNPs) which has enormous applications in various fields [Citation13]. Thus, biological materials, namely, fungi, bacteria, biomolecules [Citation14,Citation15] and plant extracts [Citation16] are under much investigation. In earlier reports, biosynthesized AgNPs were reported to have potential antimicrobial, antiplasmodial and larvicidal properties [Citation17,Citation18].
In the early twentieth century, the ability of plant extracts to reduce metal ions has been known. In view of its simplicity, and use of plant’s extract and tissue as source of nanoparticles synthesis has caught the attention of the researchers [Citation19,Citation20].
Cassia fistula L. (Leguminosae, commonly known as: Golden Shower) is a plant widely cultivated in India, Pakistan, Bangladesh and Tropical Africa and has been used in traditional medicine by local people since long time [Citation21]. Moreover, different parts of C. fistula (leaves, seed, flower, etc.), were reported previously to have high medicinal value [Citation18]. In the present study, a new trend for the green synthesis of AgNPs using fruit pulp extract of C. fistula as reducing and stabilizing agent has been described. The obtained particles were characterized by UV–vis spectroscopy, scanning electron microscope (SEM), transmission electron microscope (TEM), Fourier transform infrared spectroscopy (FTIR), energy dispersive X-ray (EDX) and X-ray diffraction (XRD). In addition, the mosquito larvicidal and pupicidal activity of AgNPs in laboratory conditions was investigated. To the best of our knowledge, this is the first report for the synthesis, characterization, larvicidal and pupicidal potential of silver nanoparticles from C. fistula L fruit pulp against mosquito vectors. Furthermore, the effect on enzyme levels in the larval system after treatment with AgNPs will give an exact understanding about the mechanism of its action.
Materials and methods
Chemicals and plant materials
The used silver nitrate (AgNO3) in this research was received from Sigma–Aldrich Chemicals (St Louis, MO). The dry fruits of C. fistula were collected from Mansoura University, Mansoura, Egypt. The plant materials were authenticated and confirmed at the Department of Botany, Mansoura University Egypt and kept in a well-dried place for further processing.
Preparation of plant fruit extract
The pulp was collected after breaking dry fruits and it was dried at ambient temperature and then ground manually. The fruit pulp powder (10 g) was taken and mixed with distilled water (100 ml) and it was kept in a water bath at 60 °C for 20 min. The extracts were purified through Whatman No. 1 filter paper, and the aqueous extract was used as a starting material for the synthesis of silver nanoparticles according to the method described by Das et al. [Citation22].
Preliminary phytochemical testing
The mature fruit pulp extract was subjected to preliminary phytochemical testing to detect different chemical groups of compounds by applying the methods described elsewhere [Citation23]. Air-dried and powdered pulp was screened for the presence of alkaloids, flavonoids, glycosides, anthraquinones, coumarins, and carbohydrates according to the method described by Arokiyaraj et al. [Citation24].
Synthesis of silver nanoparticles
To synthesize AgNPs, 50 mL of fruit pulp extract was mixed with 200 mL of aqueous solution of AgNO3 (3mmol/L) and it was stirred for 1 h at 60 °C. The reduction occurred rapidly as indicated by a reddish brown colour of the sample after 24 h indicating the formation of AgNPs. The mixture of AgNPs with proteins, carbohydrates and flavonoids materials obtained was purified two times from impurities by centrifugation (10,000 rpm/30 min) and the pellet was collected and dried according to the method described by Poopathi et al. [Citation25].
Characterization of synthesized C. fistula/AgNPs
UV–Vis spectral analysis
The samples used for analysis were diluted with 2 mL double distilled water and were characterized by operating UV-2550 spectrophotometer (UV-Visible spectrophotometer Shimadzu, Japan) at the resolution of 1 nm with ranging from 190 nm to 700 nm according to the method described elsewhere [Citation14,Citation24].
FT-IR analysis
FT-IR spectra were recorded under identical conditions of 4000–400 cm−1 region at a resolution of 4 cm−1 using Fourier transform infrared spectrometer (VECTORE 22, BRUKER, Germany) according to the method described elsewhere [Citation26]. The AgNPs (1 mg) was mixed with KBr (300 mg). The thin AgNPs pellet was prepared by pressing with the hydraulic pellet press and was analyzed by FT-IR.
SEM analysis
The size of the synthesized AgNPs was analyzed by Scanning Electron Microscopic (SEM) (TM-1000, Hitachi, Japan). Thin film samples were prepared on a carbon-coated copper grid (mesh size, 40 × 4 μm) by dropping the sample on the grid and extra solution was removed using a blotting paper. Then, the film on the SEM grid was allowed to dry by putting the grid under a mercury lamp for 5 min.
TEM analysis
The structural characterization of AgNPs was carried out by Transmission Electron Microscopy (TEM) (JEOL- JEM-1230, Japan). The extra sample was removed from carbon coated copper grid using the cone of a blotting paper and sample was placed on the carbon-coated copper grid to make a thin film of the sample and then it was kept in grid box sequentially. The instrument was equipped with an Energy Dispersive Spectrum (EDS) to confirm the presence of silver metal.
X-ray diffraction analysis of AgNPs
To investigate the crystalline structure of AgNPs, XRD analysis was conducted using Siemens X-ray diffractometer. The operation conditions described by Murugan et al. [Citation27] were followed and these were 45 kV and 20 mA current with CuKα radiation as an X-ray source.
Mosquito larvicidal and pupicidal activity of AgNPs
Larvae and pupae collection
Aedes albopictus and Culex pipiens pallens eggs were collected from the laboratory of Urban Entomology, Institute of Insect Sciences, Zhejiang University. To develop the colony after hatching, the larvae were preserved in plastic and enamel trays containing tap water. First instar larvae were fed with a small amount of rat food until reaching the fourth instar form. The transformed pupae were separated manually and relocated by transport tool into glass beaker (100 ml) containing distilled water. The beaker was introduced into cages for the emergence of adult mosquitoes. Cotton soaked in 5% glucose solution was used as glucose meal for adult mosquitoes and periodically they were provided with the mouse to serve as a blood source for laying eggs. The experimental method was according to the practice adopted by [Citation14]. Second and third instars were used directly and first instar was reared up to fourth instar in the laboratory before using them in the experiment.
Larvicidal and pupicidal bioassay
With minor modifications, standard methods for testing the toxicity of aqueous plant extract and biologically synthesized nanoparticles on mosquito larvae and pupae were performed as stipulated by [Citation28]. The setup was maintained at 27 ± 1 °C and 70 ± 4% RH, a photoperiod of 14:10 L: D. The desired concentrations of aqueous plant extract such as 250, 500, 750 and 1000 mg/L were prepared in a 250 mL beaker (Borosil) using deionized water as a solvent with twenty insect per one replicate. The control was set up with dechlorinated tap water. Synthesized AgNPs toxicity tests were conducted using a multi-concentration test which consisted of a control and different concentrations of AgNPs. The AgNPs toxicity test was carried out by using twenty larvae of each instar (I–IV instar) and pupae per one replicate into 200 ml of Milli-Q water. Silver nanoparticle solution was diluted using double distilled water as a solvent according to the desired concentrations (2.5, 5, 7.5, and 10 mg/L). Each test including a set of the control group (distilled water) was tested with four replicates for each individual concentration. The effectiveness of silver nanoparticles and aqueous plant extract as mosquito larvicidal and pupicidal were determined from all the twenty larvae/replicate (I–IV instar) and pupae by exposing them to different time periods. The AgNPs and aqueous plant extract toxicity was assessed against the larvae of Ae. albopictus and Cx. pipiens pallens at 24, 48 and 72 h of exposure time. The numbers of dead larvae and pupae were counted with four replicates. The LC50 and LC90 values were calculated from the average of four replicates.
Examination for the mechanism of AgNPs for mosquito larvicidal potential
We studied the action of AgNPs on the functioning of essential enzymes involved in the metabolism of mosquito larvae. The enzymes studied were acetylcholinesterase, α- and β-carboxylesterase. All the tests were carried with fourth instar larvae.
Preparation of whole body homogenates
Biochemical and physiological changes were analyzed by biochemical experiments using the procedures outlined by WHO [Citation29] with suitable modifications. Silver nanoparticles and plant extract (test) and untreated (control) larvae (30 individuals), after 24 h, were washed with double distilled water and their body surface was blotted with tissue paper to remove the adhering water. The larvae then were collected and homogenized in EppendorfTM tubes (held in ice) using a Teflon hand homogenizer in 1 ml of 0.05 phosphate buffer (pH 7.0) for eventual estimation of total proteins, acetylcholine esterase, α- and β-carboxylesterase assay. The whole body homogenates were centrifuged at 10,000 rpm for 10 min at 4 °C and the clear supernatants were kept at 4 °C until usage for biochemical analysis.
Determination of protein concentration
The proteins in the larval homogenates of both test and control were first precipitated by 80% ethanol [Citation30] and the protein concentrations were estimated by the method of Lowry et al. [Citation31].
Acetylcholinesterase assays
Acetylcholinesterase activity in the whole body of control and test larval homogenate was spectrophotometrically measured according to Wierenga and Hollingworth [Citation32] with slight modifications using acetylcholine iodide as a substrate. Each aliquot of homogenate (200 μl) was mixed with 200 μl of sodium phosphate buffer (100 mM, pH 7.5). Following preincubation for 10 min at 30 °C, 50 μl of 10 mM 5,5-dithio-bis-(2-nitrobenzoate) and 50 μl of 12.5 mM acetylthiocholine iodide were added for a total volume of 1 ml. After incubation for 5 min at room temperature, the reaction was measured at absorbance at 400 nm using Shimadzu UV spectrophotometer (Kyoto, Japan). Readings were taken at every 33 s for 10 min against suitable reagent blank.
Carboxylesterase assays
The activity of α- and β-carboxylesterase in the larval homogenates was measured by the method of van Asperen [Citation33] with suitable modifications. Briefly, 200 μl of control and test larval homogenate were incubated with 2 ml of alpha or beta naphthyl acetate solution for 30 min at room temperature. To each reaction mixture, 500 μl of fast blue SDS reagent was added [22.5 mg fast blue salt in 2.25 ml distilled water and 5% w/v SDS in 0.2M phosphate buffer (pH 7.2)] to stop the enzymatic reaction, and the colour was allowed to develop for 15 min at room temperature. The optical density of the sample was measured at 588 nm in the spectrophotometer against the respective reagent blank.
Statistical analysis
The percentage of mortality for larvae and pupae treated with AgNPs and aqueous plant extract were subjected to probit analysis [Citation34] for calculating LC50, LC90 statistics at 95% confidence limits of lower and upper values. The difference in the levels of various biochemical parameters between control and experimental larvae were tested for statistical significance using mean difference Student’s t-test (SPSS software). Chi-square values were calculated using the SPSS software package 16.0 version (SPSS Inc., Chicago, IL). The acceptance level of statistical significance was p ≤ .05 for all instances.
Results and discussion
Preliminary phytochemical testing
The preliminary phytochemical analysis was carried out to detect the main constituents in the fruit pulp extract of C. fistula. shows that C. fistula fruit pulp extract contained alkaloids, flavonoids, anthraquinones, coumarins, and glycosides/carbohydrates in relatively high amounts. In plants, the presence of carbohydrate, flavone glycosides and anthraquinones compounds are expected and these are to be held responsible for the reduction of Ag+ to Ag0 [Citation35].
Table 1. Phytochemical screening of C. fistula fruit pulp extract.
In previous studies, the presence of saponins, tannins, alkaloids, flavonoids, triterpenoids, steroids, glycosides/carbohydrates, anthraquinones, reducing sugars, starch, protein and amino acids in C. fistula leaves have been reported [Citation36]. The plant C. fistula seems to be a potential source of carbohydrate, flavone glycosides and anthraquinones [Citation37]. These compounds are expected to be responsible for the reduction of AgNO3 to Ag0 and plant proteins are believed to be active molecules for nanoparticles synthesis [Citation22].
UV–Vis spectroscopy
Using plant extracts, several methods can be employed for biosynthesis of AgNPs [Citation38]. UV–vis spectroscopy is an initial step for analyzing the formation of silver nanoparticles in aqueous solution. In the present study, the pulp extract along with AgNO3 underwent bio-reduction and the change of colour to yellowish brown indicated the formation of AgNPs (). The formation of plant-based on AgNPs has also been reported by other researchers [Citation22,Citation39]. The characteristics of silver surface plasmon resonance (SPR) bands were detected at around 400–450 nm [Citation40]. Even though, the actual mechanism is not properly understood. As previously reported, the AgNPs have free electrons which create a surface plasmon resonance at 448 nm wavelength. This may be due to the combined vibration of electrons of the metal nanoparticles in resonance [Citation41,Citation42]. In our study, the SPR was observed at 448 nm which is the characteristics of the silver particle (). In agreement with our results, a broad peak with maximum wavelength (410 nm) was also described in the Aloe vera based-study on the biosynthesis of AgNPs [Citation43]. The current results are also comparable with Roopan et al. [Citation44] with main difference of plant types used.
The reduction of silver ions to nanoparticles using an extract of C. fistula could be due to the presence of the phenolics, alkaloids, flavonoids, amino acids and polysaccharides. Phytochemical analysis of C. fistula revealed flavonoids, steroids, coumarins and glycosides which could have functional groups responsible for AgNPs formation. These results were in accordance with earlier research works [Citation45].
FT-IR analysis
FT-IR spectroscopy is one of the most important and widely used analytical methods for obtaining information about the availability of certain functional groups present in the structure of the silver nanoparticles. A typical FTIR spectra was carried out to identify the possible interactions between silver and bioactive molecules, which may be responsible for synthesis and stabilization (capping) of silver nanoparticles. shows the representative spectra of AgNPs in most cases: 3419 cm−1 for O–H bond and N–H bond are located in the region 1 stretching, 2923 cm−1 was associated with stretching vibration of C–H, 1623cm−1 for C = O of –NH = C=O bond stretching. The relative absorption peaks at 1558 cm−1 and 1457 cm−1 for N–H and methylene bending vibration might be related to the content of protein. It is quite clear that proteins can bind to the silver nanoparticle through the free amine groups in the proteins [Citation46]. The stronger absorption peak at 1069 cm−1showed stretching vibration of C–OH bond [Citation47]. It can be seen that the peak at 1260 cm−1was observed most probably due to the C–O group of polyphenols in hydroxyl avones [Citation48].
SEM, TEM analyses and EDX spectrum
Most of SEM images of AgNPs were spherical as indicated by the micrograph. In this study, the size of the AgNPs synthesized by fruit pulp extract of C. fistula was in the range of 148–938 nm (). During SEM analysis, it was revealed that the various grain sizes of AgNPs might have been produced by the agglomeration of Ag particles. Additionally, the sizes of the synthesized AgNPs were characterized using TEM. The TEM images of AgNPs synthesized also showed that the particles are nearly spherical (). The size and shape of NPs play an important role in many of biological applications, especially in vector management program. It has been confirmed using electron microscopy that the AgNPs and AuNPs mostly are spherical [Citation16,Citation49]. The shape of plant-mediated AgNPs was mostly spherical with the exception of Azadirachta indica which yielded both spherical and flat plate-like morphology [Citation50]. SEM images of AgNPs from Emblica officinalis were also predominantly spherical ranging from 7.5 to 25 nm with an average size of 16.8 nm [Citation51].
Figure 3. (A) SEM, (B) TEM images of AgNPs synthesized by reducing 3 mM AgNO3 using aqueous pulp extract of C. fistula, (C) EDX results of the AgNPs formation.
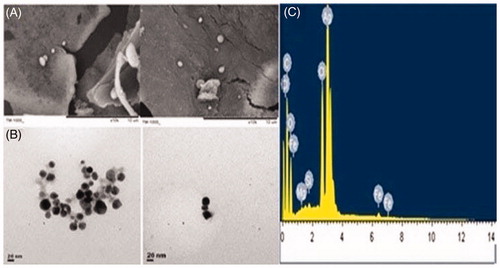
From (), it could be noted that the AgNPs have also given a characteristic strong peak at 3 keV, which indicates the reduction of Ag+ to Ag0. The other peaks of C, O, Cl, Mg, Si and a small peak of Fe were observed which were possibly due to the presence of phytochemical elements in the pulp extract of C. fistula. These elements might be capped with the AgNPs, which is an added advantage in the green chemical synthesis. The EDX profile shows the presence of a strong characteristic silver signal at almost 3 keV, which is perfect for the absorption of metallic silver nanocrystals due to surface plasmon resonance. It has been reported that synthesized nanoparticles using plant extracts were enclosed by a thin coat of some capping organic material from the plant leaf broth and are stable in solution up to 6 months [Citation22]. This is another feature of nanoparticles synthesized using plant extracts over those synthesized using chemical methods. The EDX spectrum has shown the characteristic silver peaks on the surface of the nanoparticles, suggesting successful silver nanoparticles synthesis using plant fruit extract.
X-ray diffraction analysis of AgNPs
The XRD is an effective method for determining the crystal structure of AgNPs and can provide very useful information about the physical and chemical form of the silver nanoparticles [Citation52]. shows the XRD pattern of AgNPs of vacuum dried C. fistula. After the reaction, the diffraction peaks at 2θ = 38.27°, 46.27°, 64.64°, and 77.66°could be assigned to the 111, 200, 220 and 311 planes of a faced centre cubic lattice of silver [Citation53]. Unidentified crystalline peaks (27.89°, 32.30° and 54.79°) are also apparent in many research works in which the XRD pattern included the relevant 2θ range [Citation54]. These peaks came as a result of the organic compounds which are present in the extract of the plant [Citation44]. So, overall XRD pattern revealed that C. fistula mediated AgNPs were really crystalline.
Mosquito larvicidal and pupicidal activity
In the present study, the larvicidal and pupicidal activity of different concentrations of aqueous plant extract and synthesized silver nanoparticles of Cassia fistula fruit pulp were tested against the first to fourth instar larvae and pupae of two vector mosquitoes in 24, 48 and 72 h intervals. The LC50 and LC90 values of aqueous extract against (I–IV) larvae and pupae such as: A. albopictus had LC50 values of 624, 822, 966, 1288 and 3871 mg/L and LC90 values of 19241, 25739, 16026, 21806 and 33556 mg/L after 72 h, respectively (); Cx. pipiens pallens had LC50 values of 493, 716, 896, 1218 and 6126 mg/L and LC90 values of 3925, 12167, 21804, 26979 and 185186 mg/L after 72 h, respectively (). Previously, several plant crude extracts have been reported as potential larvicidal agents against target mosquitoes, namely, Cannabis sativa and Humulus lupulus [Citation55], Clausena dentata [Citation16] with least LC50 and LC90 values and these findings support the outcome of present study. The mortality effect of silver nanoparticles on larvae and pupae may be due to the internal toxic effects of tiny particle inside cuticle. As the cross movement of small particles targeting individual cell inside and interfere with melting and other physiological processes have been described elsewhere [Citation56]. The present results show that synthesized AgNPs had shown an excellent activity against (I-IV) instar larvae and pupae of Ae. albopictus and Cx. pipiens pallens with low LC50 and LC90 values ( and ). The LC50 and LC90 values of AgNPs against (I-IV) larvae and pupae such as: A. albopictus had LC50 values of 8.3, 9.3, 12.0, 16.5 and 33.1 mg/L and LC90 values of 51.3, 47.1, 56.0, 78.0 and 519.3 mg/L respectively after 24 h (); Cx. pipiens pallens had LC50 values of 1.1, 1.2, 4.6, 9.7 and 18.8 mg/L and LC90 values of 4.7, 11.6, 94.0, 234.0 and 523.4 mg/L, respectively after 24 h (). The value of χ2 was significant at p ≤ .05 level. Recently, significant effects on different vector mosquitoes have been documented using silver nanoparticles. Though the aqueous extract of the plant has effect on larval instar of both mosquito species but it needs high concentrations to cause mortality of larvae as compared with AgNPs treatments which appeared lethal effect vividly on mosquito larvae and pupae with very low concentrations. The regression equation suggested that the linear relationship between the rates of mortality and doses of silver nanoparticles have positive relationship between the higher doses of silver nanoparticles and the larval body's absorption of high quantity of nanoparticles that caused their death at the end. Additionally, AgNPs in the intracellular spaces bind to sulphur containing proteins or to phosphorus containing compounds like DNA, leading to the denaturation of some organelles and enzymes [Citation57]. In other studies, the LC50 values of the synthesized AgNPs using Rhizophora mucronata leaf extract were identified as 0.585, 2.615 mg/l and LC90 values as 0.891 and 6.291 mg/l against the larvae of A. aegypti and C. quinquefasciatus [Citation58]. Similarly, the larvicidal activities of synthesized AgNPs against Cx. pipiens pallens and Cx. quinquefasciatus were assessed with LC50 = 3.62 ppm; LC90 = 38.71 ppm and LC50 = 49.89 ppm; LC90 = 84.85 ppm, respectively [Citation14,Citation44]. Furthermore, latex-producing plant Pergular iadaemia AgNPs showed excellent larvicidal activity for first, second, third and fourth larval instars. However, after either 24 or 48 h of exposure, the larvae of Aedes aegypti and A. stephensi did not exhibit any noticeable mortality effects [Citation59].
Table 2. Larval and pupal toxicity using fruit pulp aqueous extract of C. fistula against Ae. albopictus.
Table 3. Larval and pupal toxicity using fruit pulp aqueous extract of C. fistula against Cx. pipiens pallens.
Table 4. Larval and pupal toxicity of silver nanoparticles synthesized using C. fistula fruit pulp extract against Ae. albopictus.
Table 5. Larval and pupal toxicity of silver nanoparticles synthesized using C. fistula extract against Cx. pipiens pallens.
The mechanism of AgNPs and plant extract on mosquito larvae
Considering the larvicidal activity of AgNPs, it is also necessary to know its mechanism of action. The effects of AgNPs and plant extract on the biochemical constituents of the fourth instar larvae of Ae. albopictus and cx. pipiens pallens were measured. In general, it was found that the tested samples caused variation in normal biochemical constituents with either an increase or decrease in their activity as compared to control (). For this, we carried out a study involving the effect of AgNPs on different enzymes involved in biological processes of mosquito larvae. It involves the estimation of total protein, acetycholinesterase, α- and β-carboxylesterase. The exposure of the larvae of Ae. albopictus and cx. pipiens pallens to silver nanoparticles for 24 h significantly decreased the total protein level from the control value of 2.07 to 1.28 and 1.58 to 0.96 mg protein/ml of homogenate, respectively (). Besides, the level of acetylcholinesterase activity in the control larvae of Ae. albopictus and cx. pipiens pallens was 1.80 and 2.01 μM ACT/mg/min respectively, and it significantly decreased to 1.12 and 1.65 μM ACT/mg/min respectively, in the larvae exposed to AgNPs for 24 h (). A similar effect was also observed with α-carboxylesterase, wherein its activity decreased from 0.39 to 0.32 and 0.40 to 0.33 μM α-naphthol released/mg/min, respectively on Ae. albopictus and cx. pipiens pallens. On the other hand, a slight, but insignificant, reduction in the level of β-carboxylesterase activity was detected in AgNPs-exposed larvae of Ae. albopictus and cx. pipiens pallens in which it declined from 0.65 to 0.62 and 0.65 to 0.57 μM β-naphthol released/mg/min, respectively (). In the present study, the protein metabolism in the larvae of Ae. albopictus and cx. pipiens pallens exposed to AgNPs was found to be disturbed as evident from 61% and 60% reduction in level of total protein in the larval homogenates. This observation suggests a direct toxic effect of silver nanoparticles on the protein synthetic machinery of the mosquito larvae. In mosquitoes, esterases are the primary enzymes involved in development of resistance mechanisms to synthetic chemical insecticides by cleaving the carboxylester and phosphodiester bonds [Citation60]. Resistant insects usually show a very high activity of esterases [Citation61,Citation62]. The elevated level of esterases in various insect species including mosquitoes has been primarily associated with the enhancement of resistance against insecticidal compounds [Citation60,Citation63]. It is pertinent to note that exposure of a wide range of insect targets to various plant secondary metabolites has been reported to cause decrease in the levels of esterases [Citation64,Citation65]. Aqueous extract of kernel from the soapnut with proven anti-mosquito activity [Citation66] was found to significantly reduce the levels of both acetylcholinesterase and carboxylesterase in the larvae of A. aegypti [Citation67]. Acetylcholine esterase breaks down the neurotransmitter acetylcholine at the synaptic cleft so that the nerve impulse can be transported across the gap. Neurotransmitters must be cleaned immediately after the message is passed, and if not, it causes paralysis. This work is done by acetylcholine esterase in the insect’s nervous system. In the test sample acetylcholine esterase was found to be decreased by 62% and 82% when compared to control.
Conclusions
Vector control requires improvement of new mosquito control methods that are cheap and eco-friendly. In this study, biosynthesized AgNPs were characterized by FTIR, TEM and confirmed by SEM, EDX and UV-vis spectrophotometry techniques. Green synthesis of nanoparticle from aqueous extracts of C. fistula may be a suitable alternative vector control approach in this regard. Green synthesis provides advantages over chemical and physical methods as it can be easily scaled up for large-scale synthesis. In green synthesis method, there is no need to use high pressure, energy, temperature and toxic chemicals. In the next steps of our research we will try to find the exact mechanism for the reduction of AgNO3 by the plant bioactive compound and the new possible applications in the field of mosquito such as the mechanism of the death.
Ethical statement
All applicable international, national, and/or institutional guidelines for the care and use of animals were followed.
Disclosure statement
We declare that we have no conflict of interest.
Additional information
Funding
References
- Jensen M, Mehlhorn H. Seventy-five years of Resochin in the fight against malaria. Parasitol Res. 2009;105:609–627.
- WHO. Factsheet on the World Malaria Report 2012. [cited 2014 Mar 28]. 2014.
- Wei G, Zhang L, Yan H, et al. Evaluation of the population structure and genetic diversity of Plasmodium falciparum in southern China. Malaria J. 2015;14:283.
- Suman DS, Shrivastava AR, Pant SC, et al. Differentiation of Aedes aegypti and Aedes albopictus (Diptera: Culicidae) with egg surface morphology and morphometrics using scanning electron microscopy. Arthropod Struct Dev. 2011;40:479–483.
- Soni N, Prakash S. Microbial synthesis of spherical nanosilver and nanogold for mosquito control. Ann Microbiol. 2014;64:1099–1111.
- Suresh U, Murugan K, Benelli G, et al. Tackling the growing threat of dengue: Phyllanthus niruri-mediated synthesis of silver nanoparticles and their mosquitocidal properties against the dengue vector Aedes aegypti (Diptera: Culicidae). Parasitol Res. 2015;114:1551–1562.
- Benelli G, Mehlhorn H. Declining malaria, rising of dengue and Zika virus: insights for mosquito vector control. Parasitol Res. 2016;115:1747–1754.
- Dai Y, Huang X, Cheng P, et al. Development of insecticide resistance in malaria vector Anopheles sinensis populations from Shandong province in China. Malaria J. 2015;14:62.
- Wang D, Xia Z, Zhou S, et al. 2013. A potential threat to malaria elimination: extensive deltamethrin and DDT resistance to Anopheles sinensis from the malaria-endemic areas in China. Malaria J. 12:164.
- Benelli G. Research in mosquito control: current challenges for a brighter future. Parasitol Res. 2015;114:2801–2805.
- Benelli G. Plant-mediated biosynthesis of nanoparticles as an emerging tool against mosquitoes of medical and veterinary importance: a review. Parasitol Res. 2016;115:23–34.
- Muthukumaran U, Govindarajan M, Rajeswary M, et al. Synthesis and characterization of silver nanoparticles using Gmelina asiatica leaf extract against filariasis, dengue, and malaria vector mosquitoes. Parasitology Res. 2015;114:1817–1827.
- Ahmad MB, Lim JJ, Shameli K, et al. Antibacterial activity of silver bionanocomposites synthesized by chemical reduction route. Chem Cent J. 2012;6:1–9.
- Fouad H, Hongjie L, Yanmei D, et al. Synthesis and characterization of silver nanoparticles using Bacillus amyloliquefaciens and Bacillus subtilis to control filarial vector Culex pipiens pallens and its antimicrobial activity. Artif Cells Nanomed Biotechnol. 2016;18:1–10.
- Rajakumar G, Rahuman AA, Roopan SM, et al. Fungus-mediated biosynthesis and characterization of TiO(2) nanoparticles and their activity against pathogenic bacteria. Spectrochim Acta A Mol Biomol Spectrosc. 2012;91:23–29.
- Sowndarya P, Ramkumar G, Shivakumar MS. Green synthesis of selenium nanoparticles conjugated Clausena dentata plant leaf extract and their insecticidal potential against mosquito vectors. Artif Cells Nanomed Biotechnol. 2016;10:1–6.
- Salunke BK, Sawant SS, Kim BS. Potential of Kalopanax septemlobus leaf extract in synthesis of silver nanoparticles for selective inhibition of specific bacterial strain in mixed culture. Appl Biochem Biotechnol. 2014;174:587–601.
- Suman DS, Wang Y, Bilgrami AL, et al. Ovicidal activity of three insect growth regulators against Aedes and Culex mosquitoes. Acta Tropica. 2013;128:103–109.
- Borase HP, Salunke BK, Salunkhe RB, et al. Plant extract: a promising biomatrix for ecofriendly, controlled synthesis of silver nanoparticles. Appl Biochem Biotechnol. 2014;173:1–29.
- Gan PP, Li SFY. Potential of plant as a biological factory to synthesize gold and silver nanoparticles and their applications. Rev Environ Sci Bio/Technol. 2012;11:169–206.
- Duraipandiyan V, Ignacimuthu S. Antibacterial and antifungal activity of Cassia fistula L.: an ethnomedicinal plant. J Ethnopharmacol. 2007;112:590–594.
- Das J, Paul Das M, Velusamy P. Sesbania grandiflora leaf extract mediated green synthesis of antibacterial silver nanoparticles against selected human pathogens. Spectrochim Acta A Mol Biomol Spectrosc. 2013;104:265–270.
- Tona L, Kambu K, Ngimbi N, et al. Antiamoebic and phytochemical screening of some Congolese medicinal plants. J Ethnopharmacol. 1998;61:57–65.
- Arokiyaraj S, Dinesh Kumar V, Elakya V, et al. Biosynthesized silver nanoparticles using floral extract of Chrysanthemum indicum L.–potential for malaria vector control. Environ Sci Pollut Res Int. 2015;22:9759–9765.
- Poopathi S, De Britto LJ, Praba VL, et al. Synthesis of silver nanoparticles from Azadirachta indica–a most effective method for mosquito control. Environ Sci Pollut Res Int. 2014;22:2956–2963.
- Santhosh SB, Ragavendran C, Natarajan D. Spectral and HRTEM analyses of Annona muricata leaf extract mediated silver nanoparticles and its Larvicidal efficacy against three mosquito vectors Anopheles stephensi, Culex quinquefasciatus and Aedes aegypti. J Photochem Photobiol B. 2015;153:184–190.
- Murugan K, Dinesh D, Paulpandi M, et al. Nanoparticles in the fight against mosquito-borne diseases: bioactivity of Bruguiera cylindrica-synthesized nanoparticles against dengue virus DEN-2 (in vitro) and its mosquito vector Aedes aegypti (Diptera: Culicidae). Parasitology Res. 2015;114:4349–4361.
- WHO. 2004. Geneva: WHO Estimated incidence, prevalence and TB mortality [homepage on the internet][one screen]. Available from:http://www.who.int/mediacentre/factsheets/fs104/en
- WHO. 1998. Techniques to detect insecticide resistance mechanisms (field and laboratory manual), Geneva.
- Subhashini M, Ravindranath M. Haemolymph protein concentration of Scylla serrata: assessment of quantitative methods and intra-individual variability. Arch Int Physiol Biochim. 1980;88:47–51.
- Lowry OH, Rosebrough NJ, Farr AL, et al. Protein measurement with the Folin phenol reagent. J Biol Chem. 1951;193:265–275.
- Wierenga JM, Hollingworth RM. Inhibition of insect acetylcholinesterase by the potato glycoalkaloid α‐chaconine. Nat Toxins. 1992;1:96–99.
- Van Asperen K. A study of housefly esterases by means of a sensitive colorimetric method. J Insect Physiol. 1962;8:401–416.
- Finney DJ. Probit analysis. 3rd ed. London: Cambridge University Press; 1971. p. 68–72.
- Mithraja MJ, Marimuthu J, Mahesh M, et al. Phytochemical studies on Azolla pinnata R. Br., Marsilea minuta L. and Salvinia molesta Mitch. Asian Pac J Trop Biomed. 2011;1:S26–S29.
- Lloyd JR, Byrne JM, Coker VS. Biotechnological synthesis of functional nanomaterials. Curr Opin Biotechnol. 2011;22:509–515.
- Yadav R, Verma V. New biologically active flavone glycoside the seed of Cassia fistula Linn. Has been identified. J Asian Nat Prod Res. 2003;5:57–61.
- Rajakumar G, Abdul Rahuman A. Larvicidal activity of synthesized silver nanoparticles using Eclipta prostrata leaf extract against filariasis and malaria vectors. Acta Trop. 2011;118:196–203.
- Jeeva K, Thiyagarajan M, Elangovan V, et al. Caesalpinia coriaria leaf extracts mediated biosynthesis of metallic silver nanoparticles and their antibacterial activity against clinically isolated pathogens. Ind Crops Prod. 2014;52:714–720.
- Njagi EC, Huang H, Stafford L, et al. Biosynthesis of iron and silver nanoparticles at room temperature using aqueous sorghum bran extracts. Langmuir. 2011;27:264–271.
- Gengan RM, Anand K, Phulukdaree A, et al. A549 lung cell line activity of biosynthesized silver nanoparticles using Albizia adianthifolia leaf. Colloids Surf B Biointerfaces. 2013;105:87–91.
- Piryaei M, Abolghasemi MM, Nazemiyeh H. Fast determination of Ziziphora tenuior L. essential oil by inorganic–organic hybrid material based on ZnO nanoparticles anchored to a composite made from polythiophene and hexagonally ordered silica. Nat Prod Res. 2015;29:833–837.
- Dinesh D, Murugan K, Madhiyazhagan P, et al. Mosquitocidal and antibacterial activity of green-synthesized silver nanoparticles from Aloe vera extracts: towards an effective tool against the malaria vector Anopheles stephensi? Parasitol Res. 2015;114:1519–1529.
- Roopan SM, Madhumitha G, Rahuman AA, et al. Low-cost and eco-friendly phyto-synthesis of silver nanoparticles using Cocos nucifera coir extract and its larvicidal activity. Ind Crops Prod. 2013;43:631–635.
- Kesharwani J, Yoon KY, Hwang J, Rai M. Phytofabrication of silver nanoparticles by leaf extract of Datura metel: hypothetical mechanism involved in synthesis. J Bionanosci. 2009;3:39–44.
- Gole A, Dash C, Soman C, et al. On the preparation, characterization, and enzymatic activity of fungal protease-gold colloid bioconjugates. Bioconjug Chem. 2001;12:684–690.
- Huang G, Zhang H, Shi JX, et al. Adsorption of chromium (VI) from aqueous solutions using cross-linked magnetic chitosan beads. Ind Eng Chem Res. 2009;48:2646–2651.
- Begum NA, Mondal S, Basu S, et al. Biogenic synthesis of Au and Ag nanoparticles using aqueous solutions of Black Tea leaf extracts. Colloids Surf B Biointerfaces. 2009;71:113–118.
- Lallawmawma H, Sathishkumar G, Sarathbabu S, et al. Synthesis of silver and gold nanoparticles using Jasminum nervosum leaf extract and its larvicidal activity against filarial and arboviral vector Culex quinquefasciatus Say (Diptera: Culicidae). Environ Sci Pollut Res. 2015;22:17753–17768.
- Shankar SS, Rai A, Ahmad A, et al. Rapid synthesis of Au, Ag, and bimetallic Au core-Ag shell nanoparticles using Neem (Azadirachta indica) leaf broth. J Colloid Interface Sci. 2004;275:496–502.
- Ankamwar B, Damle C, Ahmad A, et al. Biosynthesis of gold and silver nanoparticles using Emblica officinalis fruit extract, their phase transfer and transmetallation in an organic solution. J Nanosci Nanotechnol. 2005;5:1665–1671.
- Velu K, Elumalai D, Hemalatha P, et al. Evaluation of silver nanoparticles toxicity of Arachis hypogaea peel extracts and its larvicidal activity against malaria and dengue vectors. Environ Sci Pollut Res. 2015;22:17769.
- Sheny DS, Mathew J, Philip D. Phytosynthesis of Au, Ag and Au-Ag bimetallic nanoparticles using aqueous extract and dried leaf of Anacardium occidentale. Spectrochim Acta A Mol Biomol Spectrosc. 2011;79:254–262.
- Kumar R, Roopan SM, Prabhakarn A, et al. Agricultural waste Annona squamosa peel extract: biosynthesis of silver nanoparticles. Spectrochim Acta A Mol Biomol Spectrosc. 2012;90:173–176.
- Bedini S, Flamini G, Cosci F, et al. Cannabis sativa and Humulus lupulus essential oils as novel control tools against the invasive mosquito Aedes albopictus and fresh water snail Physella acuta. Ind Crops Prod. 2016;85:318–323.
- Sap-Iam N, Homklinchan C, Larpudomlert R, et al. UV irradiation-induced silver nanoparticles as mosquito larvicides. J Appl Sci. 2010;10:3132–3136.
- Sundaravadivelan C, Nalini Padmanabhan M, Sivaprasath P, et al. Biosynthesized silver nanoparticles from Pedilanthus tithymaloides leaf extract with anti-developmental activity against larval instars of Aedes aegypti L. (Diptera; Culicidae). Parasitol Res. 2013;112:303–311.
- Gnanadesigan M, Anand M, Ravikumar S, et al. Biosynthesis of silver nanoparticles by using mangrove plant extract and their potential mosquito larvicidal property. Asian Pac J Trop Med. 2011;4:799–803.
- Rawani A, Ghosh A, Chandra G. Mosquito larvicidal and antimicrobial activity of synthesized nano-crystalline silver particles using leaves and green berry extract of Solanum nigrum L. (Solanaceae: Solanales). Acta Tropica. 2013;128:613–622.
- Brogdon WG, McAllister JC. Insecticide resistance and vector control. Emerg Infect Diseases. 1998;4:605–13.
- Hemingway J, Ranson H. Insecticide resistance in insect vectors of human disease. Annu Rev Entomol. 2000;45:371–391.
- Lemos FJ, Cornel AJ, Jacobs-Lorena M. Trypsin and aminopeptidase gene expression is affected by age and food composition in Anopheles gambiae. Insect Biochem Mol Biol. 1996;26:651–658.
- Polson KA, Brogdon WG, Rawlins SC, et al. Characterization of insecticide resistance in Trinidadian strains of Aedes aegypti mosquitoes. Acta Tropica. 2011;117:31–38.
- Breuer M, Hoste B, De Loof A, et al. Effect of Melia azedarach extract on the activity of NADPH-cytochrome c reductase and cholinesterase in insects. Pest Biochem Physiol. 2003;76:99–103.
- Nathan SS, Choi MY, Seo HY, et al. Effect of azadirachtin on acetylcholinesterase (AChE) activity and histology of the brown planthopper Nilaparvata lugens (Stål). Ecotoxicol Environ Saf. 2008;70:244–250.
- Koodalingam A, Mullainadhan P, Arumugam M. Antimosquito activity of aqueous kernel extract of soapnut Sapindus emarginatus: impact on various developmental stages of three vector mosquito species and nontarget aquatic insects. Parasitol Res. 2009;105:1425.
- Koodalingam A, Mullainadhan P, Arumugam M. Effects of extract of soapnut Sapindus emarginatus on esterases and phosphatases of the vector mosquito, Aedes aegypti (Diptera: Culicidae). Acta Tropica. 2011;118:27–36.