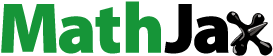
Abstract
Curcumin, a natural polyphenol, exhibits anti-oxidant, anti-inflammatory, anti-neoplastic and chemopreventive properties. In fact, targeting of this natural anticancer agent has received a great deal of attention during the recent years. In this study, we proposed that curcumin conjugation with lactoferrin molecules may lead to a potential drug delivery system targeted toward cancerous cells through both active and passive targeting. In this regard, curcumin conjugated lactoferrin was developed via a carbodiimide-based coupling reaction and the resulting conjugates were appraised for their molecular properties as a potential targeted drug delivery system. The mean diameter of the designed nanostructure was about 165 ± 26 nm with a PDI of 0.308 ± 0.045. The conjugated nanostructures showed a considerably improved cytotoxicity on HCT116 cells as illustrated by MTT assay along with a higher level of cellular uptake. Cellular uptake and targeting capability of conjugated samples were further investigated by confocal microscopy and the conjugated curcumin nanostructures showed an enhanced efficacy compared to curcumin. Furthermore, flow cytometry analysis proved that early apoptosis occurred in HCT116 cell line, after 24 h incubation with conjugated curcumin.
Graphical Abstract
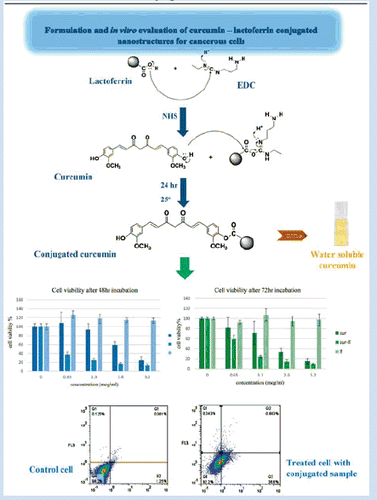
Introduction
Curcumin, is a small, unsaturated natural hydrophobic, polyphenolic β-ketone compound which is commonly found in the rhizomes of Curcuma longa. In 1870, curcumin was purified in its crystal form and its chemical structure was clarified as 1,6-heptadiene-3, 5-dione-1, 7-bis (4-hydroxy-3-methoxyphenyl)-(1E, 6E) or diferuloylmethane and further attempts were made to chemically synthesize this molecule [Citation1–3]. The potency for the suppression of tumour necrosis factor (TNF)-induced nuclear factor-kappaB (NF-kB) activation for curcumin and its related compounds is rated curcumin > demethoxycurcumin > bisdemethoxycurcumin offering a significant role for the methoxy groups existing on the phenyl rings [Citation2,Citation4]. Food and drug administration (FDA), has considered curcumin as “Generally Recognized as Safe (GRAS)” [Citation5] and it has been demonstrated that curcumin presents an extensive range of pharmacological functions which are beneficial in several chronic disorders such as multiple sclerosis, type II diabetes, atherosclerosis, rheumatoid arthritis and Alzheimer’s disease. The anti-cancer activity of curcumin is of particular interest and it has been recommended as a potential therapeutic for both inhibition and treatment of a major variety of various cancers, including gastrointestinal, melanoma, genitourinary, breast, lung, haematological, head and neck, neurological and sarcoma cancers [Citation2, Citation5–8]. It has been demonstrated that curcumin can inhibit different tumour cell lines growth including prostate cancer, osteosarcoma, lung cancer, breast cancer and colorectal cancer cell lines [Citation9–11].
Various pre-clinical animal samples and human surveys have confirmed that curcumin is nontoxic and well tolerated even at very extraordinary doses [Citation10]. Thus, curcumin has become an extremely favourable candidate for curing various cancers, due to its acceptable safety profile, low adverse effects and good efficiency on several cancer cell species. Unfortunately, this outstanding therapeutic potential of curcumin is widely limited by its very low water solubility (10–20 μg/ml), negligible tissue absorption, and fast metabolism which indicate a necessary demand for designing novel drug delivery systems to effectively deliver this beneficial neutraceutical [Citation10,Citation12].
To overcome these pharmaceutical limitations, we tried to develop a novel drug delivery system by conjugating curcumin molecules to lactoferrin (Lf). Lf a molecule with an approximately 80 kDa molecular weight, is a glycoprotein which demonstrates high affinity towards iron. Because of the 60% similarity between sequences of Lf’s amino acids and serum transferrin, Lf has been considered as a member of transferrin family [Citation13]. Lf is capable to access the cell nucleus, due to its nuclear localization sequence. The procedure of the recent action is now unidentified, but is supposed to take place by Lf receptors [Citation14].
Conjugating curcumin with small molecules such as amino acids and natural and synthetic hydrophilic polymers has been previously utilized to enhance its water solubility [Citation15,Citation16]. Enhanced water solubility was achieved by conjugation of curcumin to hyaluronic acid as a hydrophilic polymer where the conjugated structures formed micelles in aqueous media with a diameter larger than 500 nm. Although a higher cytotoxicity was achieved by this modification, the large size of the formed micelles may limit their successful internalization. Furthermore, hyaluronic acid does not specially target tumour tissues and would be equally distributed throughout the body [Citation15]. Other researchers have tried to develop a targeted and water soluble formulation of curcumin by loading it in human serum albumin based nanoparticles [Citation16]. These nanoparticles showed relative tumour accumulation and better solubility but their targeting strategy for tumour tissue was solely based on passive targeting.
In this paper, nano-structures of curcumin are proposed as a targeted drug delivery system to deliver curcumin to tumour sites and to specifically develop the therapeutic effects in the target tissues [Citation2]. Lf was utilized as a vector here due to its capability for actively targeting tumour tissues through receptor mediated transcytosis along with passively targeting such tissues by enhanced permeation and retention effect (EPR). Lf ability to access cell nucleus would provide an additional advantage for better chemotherapeutic intervention in multiple cellular levels. In the current paper the approaches utilized for formulation and development of curcumin–lactoferrin (Cur-Lf) conjugates are discussed and the results obtained from various in vitro tests are discussed. The injectable curcumin conjugated Lf nanostructure are proposed as a potential targeted drug delivery system for improving the aqueous solubility and cytotoxicity of the drug, and thus as a successful approach to improve tumour cell targeting and specific drug uptake.
Materials and methods
Materials
Curcumin powder, research grade, was purchased from Serva Company (Islandia, NY). Lf from bovine milk, N-hydroxy-3-succinimde (NHS) and 1-(3-dimethylaminopropyl) ethyl carbodiimide (EDC) were purchased from Sigma Aldrich (St. Louis, MO). High performance liquid chromatography (HPLC) grade acetonitrile, HPLC grade methanol, sodium hydroxide, sodium azide, sodium hydrogen phosphate, dimethylsulfuoxide (DMSO) and dimethylformamide (DMF) were from Merck (Darmstadt, Germany). Every other reagent if not stated was from Merck (Darmstadt, Germany).
Preparation and purification of lactoferrin conjugates with curcumin
Lf was dissolved in a phosphate buffered solution (0.1 M, pH 7.3 plus 50 mM NaCl). In addition, 4.5 μmole curcumin (1.65 mg), was dissolved in 1 ml DMSO and was added to Lf solution (50 μM) with 10 to 40 times molar excess. 9 μmole NHS (1.03 mg) and 9 μmole EDC (2 mg) were dissolved in a DMSO-DMF solution (70:30) and the first mixture was added to the NHS-EDC solution and was stirred over night at room temperature and in dark condition. The final solution was subsequently centrifuged (5000 g, 10 min), and the supernatant was concentrated and purified by Amicon tubes (Millipore, Germany, 30 kDa cut off). The final solution was then infused into dialysis membranes (Eppendorf, USA, cutoff 12 kDa) to remove any unattached curcumin molecules. The resulting purified conjugated samples were consequently freeze-dried and stored at 4 °C until required.
Characterization of conjugation ratio and protein recovery
UV analysis of curcumin
Standards with different concentrations were prepared from the stock solution by serial dilution to obtain 2, 3, 5, 8, 10 μg/ml concentrations. The UV absorbance of these standard solutions were determined by a Scinco UV spectrophotometer at 425 nm wavelength and the calibration curve was obtained and was used for determining curcumin concentration in conjugated samples. Free Lf did not demonstrate any interaction in this wavelength. In addition, the absorption spectra of curcumin before and after conjugation was compared by scanning the samples in a UV range between 300–600 nm. To validate the UV method, accuracy and precision data for the UV analysis of curcumin solutions were determined by analyzing triplicate samples at five different concentrations.
Solubility improvement of conjugated curcumin
Solubility of free and conjugated curcumin was determined by adding an excess amount of free curcumin’s powder and lyophilized powder of conjugated sample to a certain amount of deionized water. The mixtures were vortexed for 5 min and centrifuged at 20,000 rpm for 5 min. The supernatant was separated and analyzed by UV spectrophotometer to calculate the curcumin concentration.
Lactoferrin quantification by modified Bradford method
A modified Bradford method was utilized for determining the protein concentration. In this method, UV absorbance was measured at 450 nm and 595 nm wavelengths in the presence of Bradford indicator and the absorbance ratio at these two wavelengths was used to obtain a calibration curve where bovine serum albumin served as standard. Consequently, the concentration of Lf was determined using the obtained calibration curve.
Conjugation ratio determination
The conjugation ratio was determined by calculating the number of moles of curcumin attached to each mole of Lf.
Determination of protein recovery in conjugated samples
The protein recovery in various conjugation conditions was calculated by the following formula:
HPLC analysis of curcumin
To further make sure that no free curcumin has remained in the conjugated mixture after extensive dialysis, an HPLC method was developed to quantify the level of free curcumin concentration. The stationary phase used was a 25 cm*0.46 cm C18 column with a particle size of 5 μm mounted on a Kneuer HPLC system (Berlin, Germany) consisting of a Well chrome K1001 pump, a Rheodyne injector armed with a 20 μL sample loop and a K2600 UV detector. Curcumin was detected at 425 nm and Acetonitrile/Methanol/Water (40:23:37, v/v/v) was used as the mobile phase with a flow rate of 1 ml/min. The pure HPLC grade methanol was used as blank sample.
Calibration curve was plotted for curcumin solutions with different concentrations of 2, 3, 5, 8, 10 μg/ml in methanol. Accuracy was determined by analyzing triplicate samples from five calibration standards with the above mentioned concentrations and was expressed as relative error, RE%, calculated with the following equation:
Precision values were determined by analyzing three replicates of the calibration standards and was expressed as the relative standard deviation (RSD %).
Evaluation of molecular weight of conjugated species by gel electrophoresis
Sodium dodecyl sulfate polyacrylamide gel electrophoresis (SDS-PAGE) technique was performed in non-reducing condition with a 10% polyacrylamide gel and a standard ladder from 24,000 to 93,000 Da and was used to determine any changes in the molecular weight of Lf molecules after the conjugation practice.
Preparation of nanostructures from conjugated curcumin
In order to make nanostructures from the conjugated species, the samples were homogenized by high pressure homogenizer, 20,000 rpm for 5 min. The samples were then sonicated by probe sonicator for 20 min (4 W) and were finally ultra-sonicated for 15 min.
Particle size analysis and field emission scanning electron microscopy imaging
Size distribution and particle size of conjugated samples were determined by dynamic light scattering (Zetasizer Nano ZS, Malvern, UK). The samples were prepared with a suitable concentration and were analyzed for their mean diameter and poly-dispersity index (PDI). The formation of nanoparticles, their size and morphology were further evaluated via field emission scanning electron microscopy (FESEM) (HITACHI S-4160).
In vitro cytotoxicity assay
In order to evaluate the impact of curcumin nanostructures on cell growth, MTT assay was utilized with Human Colon Cancer cells (HCT116). Cell viability (MTT) assay was carried out using comparable dosages of free curcumin, free Lf and conjugated curcumin where untreated cells attended as control. For this assay, cells, were plated in 96 well-plates and were treated with 0.62, 1.3, 2.5 and 5.2 μg/ml of free curcumin, free Lf and conjugated curcumin nanostructures according to their curcumin concentration. The assay was completed after 48 and 72 h and the colorimetric determination of cell viability was performed and relative growth prevention was calculated at 540 nm. All experiments were setup in quadruplicate.
Cellular uptake studies
To study the cellular uptake, HCT116 cells were cultured on glass slides alternatively where each well was surrounded by free wells to prevent any interaction among fluorescence emitted from neighbouring wells. In order to prepare Fluorescein isothiocyanate (FITC) labelled Lf, 9.45 μg Lf was dissolved in 1 ml sodium carbonate-bicarbonate buffer (pH =9) then added to the FITC (1 mg/ml) solution. The labelled Lf was stirred for 8 h at 2–8 °C in dark condition. Cells were incubated with FITC labelled Lf for 2 h. Each well was washed three times with phosphate buffered saline (PBS) after 2 h incubation at 37 °C and received 100 μl of fresh medium plus 50 μl of Triton. The cells were then studied with a fluorescence microscope (Partec pas II hast, made in Germany). All stages of this experiment were performed under light protected conditions.
Apoptosis test
In order to determine the apoptotic cells from intact cells, the flow cytometry analysis with annexin V-FITC/propidium iodide (PI) staining was utilized. The treated cells with conjugated samples at IC50 concentration were trypsinated and then washed by sterile phosphate buffer. The marked cells were then placed on ice and protected from light till analyzed by flow cytometer. Signal from FITC/annexin V was measured at 525 nm and red signal from PI was measured at 630 nm in a Cyflow flow cytometry machine (Partec pas II hast, made in Germany). Outcomes were evaluated using FlowJo software.
Results
Synthesis of conjugated curcumin
As shown in Scheme 1, Lf was attached to the phenolic OH group of curcumin by means of covalent bond. In the presence of EDC as a water-soluble carbodiimide-based coupling agent and NHS as a catalyzer of this reaction, the carboxyl group of Lf was activated and attached to the curcumin’s OH group in an aqueous medium. Accordingly, the carboxyl group of Lf was attached to the phenolic OH of curcumin by esteric bond (Scheme 1).
As it is demonstrated, by increasing the Cur-Lf molar ratio, the conjugation ratio was also increased and the highest conjugation ratio was achieved at 40 times molar excess of curcumin.
Conjugated samples characterization and quantification
Validation of UV analysis method
Curcumin was quantified by a UV spectrophotometry method which was validated and was found to be accurate and precise with an R2 of 0.9871 (, ). The conjugation ratio was determined as the number of moles of curcumin conjugated to each mole of Lf and was found to be between 0.85 and 4.52 when 10 to 40 times molar excess of curcumin was used respectively (). The comparison between absorption spectra of free and conjugated curcumin proves that the lambda max of curcumin has not been changed after conjugation practice. ()
Table 1. Accuracy and precision data for UV spectrophotometric method of curcumin assay.
Table 2. Conjugation ratio and protein recovery with various curcumin: lactoferrin molar ratios.
The protein recovery was also calculated and the results are shown in . As it can be observed in , protein recovery has a reverse correlation with curcumin to Lf molar ratio where it is decreased by increasing this molar ratio.
Solubility improvement of conjugated curcumin
The solubility of free and conjugated curcumin was evaluated using UV spectrophotometry method. Results show that the solubility of conjugated curcumin is about 2.7 μg/ml, while the solubility of free curcumin in water is below the limit of detection.
HPLC validation
HPLC method was validated using various curcumin solutions with different concentrations and the obtained calibration curve was used for determining the free curcumin concentration in conjugated samples (). The calibration curve exhibited linearity over the concentration range of 2–10 μg/ml (). The retention time of curcumin in this method was about 11 min ().
Figure 4. (A) Calibration curve of HPLC assay of curcumin. (B) Curcumin (A) and blank sample (B) chromatogram in HPLC assay of curcumin. As it is demonstrated, there is no peak in the retention time of curcumin in the blank sample (11 min).
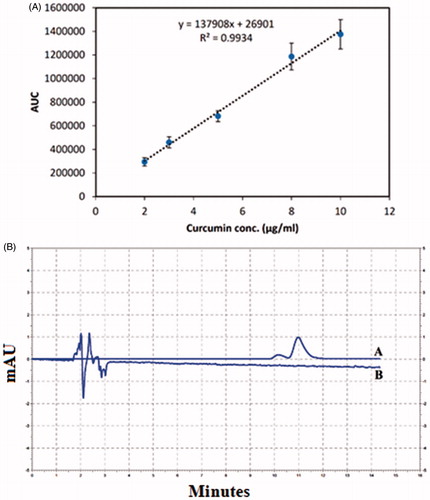
Table 3. Accuracy and precision data for HPLC assay of curcumin.
shows the chromatogram of a blank sample injected to the HPLC system with the same condition as the sample solution. As it is observed there are no peaks with the same retention time as curcumin which confirms that the HPLC method is selective for curcumin determination.
HPLC assay for conjugated curcumin did not detect any free curcumin in the related retention time which shows that after extensive dialysis no free curcumin molecules have remained in the reaction mixture and indicates that the dialysis process was efficient.
Gel electrophoresis
SDS-PAGE technique was used to determine any changes in the molecular weight of Lf molecules after the conjugation practice. As shown in , the band location for free Lf, mixture of curcumin and Lf and the conjugated samples were all approximately the same which means that all of the above species had approximately the same molecular weight.
Size analysis of conjugated sample
The mean diameter of the prepared conjugated nanostructures was about 165 ± 26 nm, and the PDI was 0.308 ± 0.045. The size and PDI were also examined after filtering by 0.45 μm syringe filter and the mean diameter was 183.5 ± 62.93 nm and the new PDI was 0.34 ± 0.01 after filtration. FESEM images further confirmed the successful formation of conjugated curcumin nanoparticles as demonstrated in .
MTT assay
The results of MTT assay after 48 h incubation with free Lf, free curcumin and conjugated form of curcumin are shown in . The IC50 of conjugated curcumin nanostructure was found to be 0.5 μg/ml, while that of free curcumin was calculated to be about 3.3 μg/ml.
Figure 7. Effect of various concentrations of curcumin, Lf and conjugated curcumin on cell viability after 48 h and 72 h. Viability was determined as the percentage of living cells in treated cultures to control.
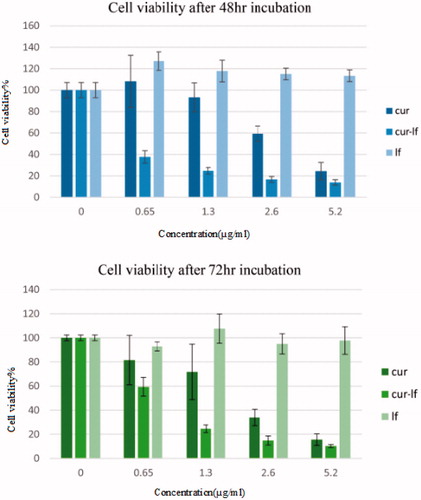
shows the results of MTT assay after 72 h incubation. The IC50 of conjugated samples after 72 h incubation was found to be 0.76 μg/ml, while that of free curcumin calculated to be about 2.03 μg/ml.
Cellular uptake
FITC was covalently attached to conjugated curcumin molecules to determine whether the conjugated formulation could enhance drug uptake by tumour cells or not. Subsequent to incubation for a determined time, cells were washed and then recognized using fluorescence or light microscopy. The mean fluorescence intensity (MFI) was calculated 36.6 for conjugated sample and 0.501 for control sample. As demonstrated in , graph A does not show any specific radiation, whereas in graph B most cells indicate significant radiation. As illustrated in the nucleoli are appeared as blue after colouring the cells with DAPI, and the green dots are associated with the conjugated samples which are labelled with FITC. This image shows that the conjugated species have entered into the cells.
Evaluation of apoptosis assay
Apoptosis was calculated via flow cytometric analysis of annexin V-FITC/PI staining. After 24 h treatment with conjugated curcumin, 36.6% cells were entered to early apoptosis stage, and 62.2% of treated cells were viable after 24 h incubation. Outcomes of a demonstrative test are displayed in . It recommends that apoptosis provides the curcumin-induced death of HCT116 cell lines.
Discussion
To improve the anticancer efficacy of the parent drug and to some extent avoid the systemic adverse effects, several anticancer drugs have been conjugated to various polypeptides and polymers [Citation17,Citation18]. As a matter of fact, tumours have vascular network specified by EPR effect, so various macromolecules such as Lf are known to accumulate in tumour tissues [Citation19]. In addition, conjugation with macromolecules leads to the constant release of the conjugated drug molecules because of enzymatic cleavage and also results in enhancement of water solubility of hydrophobic drug molecules [Citation17]. Furthermore, Lf can participate in targeted drug delivery actively through receptor-mediated transcytosis.
In order to increase the water solubility of curcumin and to improve its anticancer effects on tumoral tissues, we used Lf macromolecule as vector protein and chemically conjugated it to the drug molecule to obtain a novel drug delivery system.
As shown in Scheme 1, Lf was attached to the phenolic OH group of curcumin by means of covalent bond. In the presence of EDC as a water-soluble carbodiimide-based coupling agent, and NHS as the catalyzer of this reaction, the carboxyl moieties in Lf molecule were activated and subsequently attacked to the curcumin’s OH group. Accordingly, the carboxyl group of Lf was attached to the phenolic OH of curcumin by esteric bond (scheme 1). EDC/NHS have been chosen among the other catalysts cause we required to select a water-soluble coupling reagent to be able to interact with lactoferrin aqueous solution. EDC as a water-soluble carbodiimide is specifically appropriate for coupling reaction in aqueous media. In addition this carbodiimide reagent and its by-product are water soluble, so the byproduct and any excess reagent can be removed simply.
The conjugation ratio of various synthesized samples was determined by UV spectroscopy method. As demonstrated in the conjugation ratio of different conjugated samples was increased by increasing the Cur-Lf molar ratio [Citation17, Citation20,Citation21]. This may be the result of more curcumin molecules availability in the reaction medium. In this case, there are more hydroxyl groups available to go into interaction with activated carboxylic acid moieties of Lf. The protein recovery results indicated that the solubility of Lf was affected considerably by the hydrophobicity of curcumin. According to the protein recovery results, by increasing the molar ratio of Cur-Lf during the conjugation practice, the percent of protein recovery decreased. The reason lies behind the fact that the protein solubility may get affected by conjugating a hydrophobic drug molecule with basic or acidic amino acids. Therefore, by decreasing the solubility of protein, the protein recovery percent is reduced. As a result, for determining the conjugation ratio in conjugation practice, we tried to choose the conjugation ratio in a way that the protein does not precipitate and at the same time the highest level of conjugation ratio could be achieved.
The precision of HPLC and UV analysis as analytical procedures indicates the closeness of agreement between a series of measurements achieved from many samplings of the same homogenous samples, while the accuracy of an analytical procedure expresses the nearness of the test results achieved by the method to the normal value of the analyte. The quantities for precision and accuracy for curcumin were less than 15% that indicate a great consistency, precision and reproducibility of the assay technique over the concentration range examined [Citation22]. In this paper the indirect methods have been utilized to confirm the presence of chemical bond formation between the macromolecule and the curcumin molecule. The HPLC method was utilized to make sure that no free curcumin remained in the conjugated sample and the results showed that there was no unconjugated curcumin in the prepared sample after extensive dialysis and other purification steps which confirmed that the purification method used was efficient. The solubility of conjugated curcumin was further compared with free curcumin and the results confirmed that the solubility of curcumin has increased due to conjugation practice.
SDS-PAGE test was used to check whether any changes in the molecular weight of conjugated species has occurred after the conjugation practice or not [Citation23,Citation24]. Our results showed that the molecular weight of the conjugated species was approximately the same as free Lf where both bands migrated the same distance. This suggests that the molecular weight of conjugated lactoferrin does not change greatly after conjugation practice which supports that physicochemical properties of Lf molecules have remained unchanged after the conjugation practice [Citation17,Citation20].
Conjugated curcumin to Lf macromolecules formed nanostructures through self-assembly mechanism with a size of 165 ± 26.15 nm, and a PDI about 0.308 ± 0.045. The FESEM images proves that the particles are spherical and do not aggregate in the sample. In this technique, components form autonomously into templates or structures without any intervention by human. It should be mentioned that self-assembly is one of the numerable feasible strategies for creation ensembles of nanostructures. Two main approaches of self-assembly exist: static and dynamic. Static self-assembly deals with systems that are at global or local balance and have no energy dissipation. Formation of an ordered structure, in static self-assembly, may need energy (e.g. in the form of stirring), but once it is formed, it becomes permanent. In dynamic self-assembly, the interactions suitable for the formation of structures or patterns between components only happen if the system is consuming energy [Citation25]. In this work, it seems that the mechanism of preparation of conjugated nanostructures is static self-assembly, because, although energy was used to make the nanostructures, but the structures do not demand energy to maintain their structure. It also seems that inter molecular crosslinking may also participate in the formation of nanoparticles where EDC can contribute in internal crosslinking as well as conjugation practice.
The size and PDI results obtained before and after filtration were not significantly different which suggests that the sample does not have large particles which can be excluded by filtration practice. In comparison to Manju and Sreenivasan [Citation15], our obtained size is significantly smaller than their new conjugated formulation, which may increase the cellular uptake of our new conjugated nano-structure.
MTT assay is based on capability of viable cells to decrease a soluble yellow tetrazolium salt MTT into dark blue formazan crystals which are insoluble in water. MTT test was utilized to determine the cytotoxicity effect of developed conjugated samples on HCT116 cell line. Based on the results achieved from the cell viability test, Cur-Lf conjugates showed lower cell viability or equivalently, higher cytotoxicity versus free curcumin. The results of determining IC50 after 48 h and 72 h incubation, show that conjugated sample is more cytotoxic than free drug. The nanostructures which developed by our group is more toxic than, Tae Hyung Kim et al. formulation on the same cell line [Citation16]. This can indicate that our new conjugated nano-structure is more efficient on tumoral cells.
In order to determine the reason behind this more efficient cytotoxic effect of the conjugated curcumin when compared to free curcumin, an uptake study was performed. The results of flow cytometry showed that the conjugated sample had about 89% uptake to the HCT116 cell line and this enhanced cellular uptake may lay behind the higher cytotoxicity achieved. Since the conjugated drug can reach to the cancerous cells more effectively. Confocal microscopy demonstrated higher uptake of conjugated curcumin with Lf in comparison with non-conjugated curcumin on HCT116 cell line. The higher cellular uptake may be the result of nanostructures formation after conjugation practice which has facilitated its entrance in the cancerous cells due to their small size. As demonstrated in , the cells incubated with conjugated nanostructure formulation displayed noticeably greater fluorescence intensity than control cells. As cell death can be categorized into necrosis or apoptosis, it is necessary to realize the mode of cell death induced by curcumin. Apoptosis is more satisfactory than necrosis because it is a programmed cell death and does not trigger inflammatory responses. In this study, it was observed that the cells were going into early apoptosis and flow cytometry analysis proved the excitation of early apoptosis in the HCT116 cell lines.
Conclusions
To sum up, in this study, a conjugated form of curcumin with a suitable protein from the transferrin family was developed and characterized. Our studies show that the highest level of conjugation is reached when a 40 times molar excess amount of curcumin is adopted for reaction with Lf during conjugation practice. Results indicate that the size of the obtained conjugated structures is around 165 ± 26.15 nm. Moreover, the percentage of protein recovery after conjugation process was also investigated and demonstrated a decrease with an elevation of conjugation ratio. By developing Cur-Lf nanostructures, the insolubility of curcumin has been resolved and by passive targeting, toxic side effects of curcumin on tumoral cells are dramatically increased. MTT assay showed that conjugated curcumin shows a higher cytotoxic effect due to higher cellular uptake and a better solubility after conjugation practice. Results also showed that apoptosis cellular death pathway may be one of the main mechanisms involved in the observed cellular cytotoxicity. In conclusion, curcumin conjugated Lf represent a promising chemotherapeutic agent for targeting cancerous tissue and achieving better outcome.
Disclosure statement
There is no conflict of interest.
References
- Goel A, Kunnumakkara AB, Aggarwal BB. Curcumin as “Curecumin”: from kitchen to clinic. Biochem Pharmacol. 2008;75:787–809.
- Naksuriya O, Okonogi S, Schiffelers RM, et al. Curcumin nanoformulations: a review of pharmaceutical properties and preclinical studies and clinical data related to cancer treatment. Biomaterials. 2014;35:3365–3383.
- Qiao Q, Jiang Y, Li G. Curcumin improves the antitumor effect of X-ray irradiation by blocking the NF-κB pathway: an in-vitro study of lymphoma. Anti-Cancer Drugs. 2012;23:597–605.
- Sandur SK, Pandey MK, Sung B, et al. Curcumin, demethoxycurcumin, bisdemethoxycurcumin, tetrahydrocurcumin and turmerones differentially regulate anti-inflammatory and anti-proliferative responses through a ROS-independent mechanism. Carcinogenesis. 2007;28:1765–1773.
- Yallapu MM, Jaggi M, Chauhan SC. Curcumin nanomedicine: a road to cancer therapeutics. CPD. 2013;19:1994.
- Aditya N, Shim M, Lee I, et al. Curcumin and genistein coloaded nanostructured lipid carriers: in vitro digestion and antiprostate cancer activity. J Agric Food Chem. 2013;61:1878–1883.
- Aggarwal BB. Prostate cancer and curcumin: add spice to your life. Cancer Biol Ther. 2008;7:1436–1440.
- Singh S, Khar A. Biological effects of curcumin and its role in cancer chemoprevention and therapy. ACAMC. 2006;6:259–270.
- Anand P, Kunnumakkara AB, Newman RA, et al. Bioavailability of curcumin: problems and promises. Mol Pharm. 2007;4:807–818.
- Popat A, Karmakar S, Jambhrunkar S, et al. Curcumin-cyclodextrin encapsulated chitosan nanoconjugates with enhanced solubility and cell cytotoxicity. Colloids Surf B Biointerfaces. 2014;117:520–527.
- Wilken R, Veena MS, Wang MB, et al. Curcumin: a review of anti-cancer properties and therapeutic activity in head and neck squamous cell carcinoma. Mol Cancer. 2011;10:1–19.
- Friedman L, Lin L, Ball S, et al. Curcumin analogues exhibit enhanced growth suppressive activity in human pancreatic cancer cells. Anti-Cancer Drugs. 2009;20:444.
- Adlerova L, Bartoskova A, Faldyna M. Lactoferrin: a review. Veterinarni Medicina 2008;53:457–468.
- Baumrucker CR, Schanbacher F, Shang Y, et al. Lactoferrin interaction with retinoid signaling: cell growth and apoptosis in mammary cells. Domestic Animal Endocrinol. 2006;30:289–303.
- Manju S, Sreenivasan K. Conjugation of curcumin onto hyaluronic acid enhances its aqueous solubility and stability. J Colloid Interface Sci. 2011;359:318–325.
- Kim TH, Jiang HH, Youn YS, et al. Preparation and characterization of water-soluble albumin-bound curcumin nanoparticles with improved antitumor activity. Int J Pharm. 2011;403:285–291.
- Dosio F, Brusa P, Crosasso P, et al. Preparation, characterization and properties in vitro and in vivo of a paclitaxel-albumin conjugate. J Control Release. 1997;47:293–304.
- Mongelli N, Angelucci F, Pesenti E, Suarato A, Biasoli G. Polymer-bound paclitaxel derivatives. Google Patents; 1994.
- Iyer AK, Khaled G, Fang J, et al. Exploiting the enhanced permeability and retention effect for tumor targeting. Drug Discovery Today. 2006;11:812–818.
- Kamalinia G, Khodagholi F, Atyabi F, et al. Enhanced brain delivery of deferasirox–lactoferrin conjugates for iron chelation therapy in neurodegenerative disorders: in vitro and in vivo studies. Mol Pharmaceutics. 2013;10:4418–4431.
- Tanaka T, kaneo Y, miyashita M. Synthesis of transferrin-mitomycin C conjugate as a receptor-mediated drug targeting system. Biol Pharm Bull. 1996;19:774–777.
- D'Souza AA, Devarajan PV. Rapid and simultaneous hplc analysis of curcumin and its metabolite tetrahydrocurcumin from plasma and liver homogenates. J Liquid Chromatogr Related Technol. 2013;36:1788–1801.
- Rath A, Glibowicka M, Nadeau VG, et al. Detergent binding explains anomalous SDS-PAGE migration of membrane proteins. Proc Natl Acad Sci 2009;106:1760–1765.
- Sambrook J, Russell David W. Molecular cloning: a laboratory manual. Vol. 3: Cold Spring Harbor Laboratory Press; 1989.
- Whitesides GM, Grzybowski B. Self-assembly at all scales. Science. 2002;295:2418–2421.