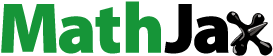
Abstract
In the present study, silver nanoparticles (PJB-AgNPs) have been biosynthesized employing Prosopis juliflora bark extract. The biosynthesis of silver nanoparticles was monitored on UV-vis spectrophotometer. The size, charge and polydispersity index (PDI) of PJB-AgNPs were determined using dynamic light scattering (DLS). Different parameters dictating the size of PJB-AgNPs were explored. Nanoparticles biosynthesis optimization studies suggested efficient synthesis of highly dispersed PJB-AgNPs at 25 °C when 9.5 ml of 1 mM AgNO3 was reduced with 0.5 ml of bark extract for 40 min. Characterization of PJB-AgNPs by SEM showed spherical-shaped nanoparticles with a size range ∼10–50 nm along with a hydrodynamic diameter of ∼55 nm as evaluated by DLS. Further, characterizations were done by FTIR and EDS to evaluate the functional groups and purity of PJB-AgNPs. The antibacterial potential of PJB-AgNPs was tested against E. coli and P. aeruginosa. The PJB-AgNPs remarkably exhibited anticancer activity against A549 cell line as evidenced by Alamar blue assay. The dye degradation activity was also evaluated against 4-nitrophenol that has carcinogenic effect. The results thus obtained suggest application of PJB-AgNPs as antimicrobial, anticancer and catalytic agents.
Introduction
In recent years, nanotechnology has emerged as promising strategy that can surmount problems of existing mode of therapies. Owing to their unique chemical and physical properties, nanoparticles have been implicated in the field of optics, catalysis, biology, medicine and pharmaceutical science. Silver nanoparticles (AgNPs) have been reported to be non-toxic, environmentally safe and exhibit antimicrobial property [Citation1,Citation2]. AgNPs have also been observed to possess anticancer activity [Citation3,Citation4]. Further, AgNPs demonstrated excellent catalytic efficacies in degradation of anthropogenic pollutants present in environment [Citation5]. Biosynthesis or green synthesis of nanoparticles is an eco-friendly and alternative strategy for synthesis of AgNPs [Citation3].
Various methodologies have been developed for the preparation of AgNPs, broadly categorized as (1) top-down and (2) bottom-up approach. Top-down method includes physical processes that include the breakdown of solid into nanoparticles, whereas bottom-up method include synthesis of nanoparticles from silver ions by employing chemical, photochemical and biological reactions. Generally, these methods generate toxic chemicals that are expensive and have high energy requirements. To mask these issues of toxicity in biological applications, plant extracts have evolved as one of the most promising options in the biosynthesis of AgNPs. Several phytoconstituents possess both protective and reductive properties that are mainly responsible for the reduction of silver ions to AgNPs [Citation3]. Several studies have been done for biosynthesis of AgNPs from plant extracts including Cinnamon zeylancium bark, Coleus amboinicus lour, Medicago sativa, Citrus sinensis peel, Panax ginseng, Weissella oryza, Terminalia arjuna, Cordia dichotoma, Canarium ovatum [Citation6–14].
In the present investigation, AgNPs have been synthesized using aqueous bark extract of plant Prosopis juliflora. This plant is a member of Fabaceae family and has been known for its use in several digestive, rheumatic and skin diseases. These medicinal properties have been attributed to the presence of several bioactive compounds such as flavonoids, alkaloids and phenolic compounds, which are further considered as essential elements in the biosynthesis of AgNPs [Citation15,Citation16]. Herein, the biosynthesis of AgNPs was done under very mild reaction conditions. Parameters influencing the synthesis of AgNPs such as reaction time, AgNO3 concentration, temperature, extract to AgNO3 ratios, have also been explored. Further, the antibacterial potential of PJB-AgNPs was tested against E. coli and P. aeruginosa, followed by anticancer activity against lung adenocarcinoma cell line, A549. Finally, the catalytic activity was also evaluated against 4-nitrophenol that has carcinogenic effect.
Materials and methods
Material
Silver nitrate (extra pure), Luria broth, Luria agar, kanamycin and 4-nitrophenol were purchased from Central Drug House (Delhi, India). Dulbecco's modified Eagle medium high glucose (DMEM HG), foetal bovine serum (FBS) and 0.25% trypsin–EDTA were procured from Life Technologies (Carlsbad, CA). Dulbecco’s phosphate-buffered saline (PBS) without calcium and magnesium chloride, sodium bicarbonate and resazurin sodium salt was purchased from Sigma-Aldrich, New Delhi, India. Filtration was done using Whatman No. 1 filter paper and double distilled water (in house prepared) was used for the preparation of extract and other solution.
Plant sampling, identification and extract collection
The barks of P. juliflora plant were collected from the campus of Central University of Rajasthan, Ajmer, India, during the month of December. Barks were washed with tap water followed by rinsing with ddH2O and shade dried. Further, barks were chopped and finely grounded using mortar pestle. 100 ml of ddH2O was boiled with 10 g of powdered bark at 60 °C for 20 min on continuous stirring at 500 rpm (Stuart hot plate stirrer, model UC152). The obtained mixture was filtered under reduced pressure condition and stored at 4 °C for further biosynthesis of AgNPs from silver nitrate.
Biosynthesis of silver nanoparticles
In a typical reaction procedure, bark extract was added dropwise to the solution of AgNO3 under continuous stirring at 500 rpm at room temperature (Stuart hot plate stirrer, model UC152). Reaction was continuously performed until the colour change was observed. Thereafter, the AgNPs were collected by centrifugation at 10,000 rpm for 10 min followed by washing thrice with ddH2O (Hanil Combi 514 R table top refrigerated centrifuge). Synthesis of PJB-AgNPs was then confirmed by UV-visible spectrophotometer analysis.
UV-visible spectrophotometer analysis
The first physicochemical characterization of synthesized PJB-AgNPs was done with UV-vis spectroscopy by scanning from 300 nm to 700 nm due to unique optical properties of AgNPs (Thermo Scientific Evolution 201, Madison, USA). This study gives a confirmation data for the synthesis due to the characteristic absorbance of AgNPs around 400 nm to 440 nm. These data also suggest approximate size, shape and yield of synthesized particles.
Optimization of reaction parameters
The biosynthesis reaction of AgNPs is very sensitive and depends on certain important parameters, i.e., time of reaction, concentration of AgNO3, volume of extract to AgNO3 and temperature of the reaction [Citation12,Citation14]. These parameters help in qualitative determination of the size, shape, yield and agglomeration state of the AgNPs. Here in this study, we have optimized these parameters for the green synthesis reaction of PJB-AgNPs.
Firstly, for time optimization, reactions were performed for different time durations, i.e., 10, 20, 40, 80, 120, 160 and 200 min by keeping 1 mM AgNO3 concentration and volume of extract fixed. After that, all reactions were subjected to centrifugation followed by three times washing and monitored by UV-visible spectrophotometer. The obtained data from UV-visible scan were further considered as the base for next optimization study.
Similarly, the concentration of AgNO3 was optimized with different silver nitrate concentration, i.e., 0.5 mM, 1 mM, 1.5 mM and 2 mM by keeping the other two parameters fixed. Further, volume of extract and the temperature of reaction were optimized in the same manner by keeping different ratios of extract to AgNO3 (1:40, 1:20, 1:10, 1:6.5 and 1:5) and various temperature conditions (4 °C, 25 °C, 40 °C, 60 °C and 80 °C) for biosynthesis reaction.
Microwave-assisted synthesis of silver nanoparticles
Here the synthesis reaction was carried out by irradiating the reaction mixture using microwave oven at regular time intervals, i.e., at 30 s, 60 s, 90 s and 120 s with other optimized conditions (Samsung, model MW73AD/B-HTL, New Delhi, India). AgNPs were separated and analyzed by UV-visible spectrophotometer.
Stability study of AgNPs
In the present study, the stability of synthesized AgNPs was examined. The master reaction was performed using optimized parameter. Reaction mixture was subjected to centrifugation followed by washing thrice and dispersed in ddH2O for storage and analyzed by UV-visible spectrophotometer.
Physicochemical characterization of AgNPs
The optimized master reaction was further employed for the characterization of the AgNPs. DLS and zeta analyzer were used to determine the hydrodynamic size and charge along with the polydispersity index of the particles. For this, freshly prepared suspension of AgNPs in ddH2O was taken after centrifugation and washing. This was followed by sonication at 25°C and measurement taken with nominal 5mW HeNe laser operating at 633 nm wavelength and scattered light was detected at 173° angle.
For confirmation of actual size and shape, morphological study was carried out by SEM and compositional study was done by EDAX (energy-dispersive X-ray analysis) using FESEM, JEOL JSM-7400 F, Tokyo, Japan. 1 ml suspension of AgNPs was vortexed, deposited on an aluminium stub and measured from a working distance of 3.2 mm and a SEI detector was used to take images at 15 kV.
Further, functional groups that are involved in the synthesis of AgNPs, as well as in the protection through capping, were confirmed by Fourier transform infrared (FTIR) spectroscopic analysis. This was carried out by concentrating both AgNPs and extract using rotary evaporator (Hei-VAP Precision, Heidolph, Schwabach, Germany), followed by drying in a desiccator. The dried powder was mixed with KBr to prepare pellet and scanned against the blank KBr pellet using FT-IR spectrometer (PerkinElmer, Yokohama, Japan) in the range of 4500–400 cm−1 at 2°cm−1 resolution. The data give information about the possible interactions occurring between the biomolecules of plant extract and Ag ions for the synthesis of AgNPs and capping process.
Evaluation of antibacterial activity using disc diffusion assay
To study the potent activity of the PJB-AgNPs towards bacteria, disc diffusion assay was performed against E. coli and P. aeruginosa [Citation17,Citation18]. The culture inoculums of both bacteria were streaked on separate LB agar plates and sterile six paper discs (5 mm in diameter) were placed on the streaked plate. The discs were then impregnated with different concentration of AgNPs. Negative and positive control was used by saturating the discs with deionized water and antibiotic kanamycin, respectively. The plates were then incubated at 37° C for 24 h followed by observation for the zone of inhibition around the discs.
Evaluation of anticancer activity against A549 cell line
The anticancer activity of PJB-AgNPs was assessed by Alamar blue assay against A549 cells (a human lung carcinoma cell line). Cells were grown in DMEM media with 10% FBS and 1% pen–strep, and maintained at 37 °C at 5% CO2 in CO2 incubator. For the experiment, the cells grown up to 80% confluency were trypsinized and seeded separately in 96-well plate with a cell density of 1 × 104 cells/well. After 24 h of incubation period, the used media were discarded and cells were washed twice with PBS. Cells were then treated with different concentration (10 μg, 50 μg, 100 μg, 150 μg and 200 μg) of synthesized AgNPs suspended in complete media and incubated for 24 h. Thereafter, the mixture of 90 μl fresh media and 10 μl Alamar blue reagent (resazurin 0.15 mg/ml) was added in each well after giving a wash using PBS and incubated for duration of 4 h. Later, the absorbance was read using micro-plate reader at 570 nm and 600 nm.
Evaluation of catalytic activity
The synthesized PJB-AgNPs have been utilized as nano-catalyst against the reduction of nitro-aromatic compound, 4-nitrophenol. To evaluate this, reactions were carried out in three sets. 200 μl of 4-nitrophenol (2 mM) was added to 2 ml of dH2O in first culture tube. In second reaction, 200 μl of 4-nitrophenol was added to 2 ml dH2O along with 1 ml of NaBH4 (0.03 M). In the third reaction, 30 μg/ml of the synthesized AgNPs was mixed with the solution obtained from the second reaction [Citation19]. All the three reactions were then monitored by UV-visible spectroscopy. However, the three reactions were periodically scanned at different time interval after centrifugation. The percent of degradation of each of the reaction was calculated by following equation
Results and discussion
The present study shows novel synthesis of AgNPs from the bark extract of P. juliflora plant. The synthesized PJB-AgNPs were shown to have medicinal value as it showed potent antibacterial and anticancer activity. Also, the biosynthesized AgNPs can aid in protecting the environment owing to their catalytic activity against organic pollutants.
Preparation of extract from P. juliflora bark
The aqueous extract of bark was prepared and filtered under reduced pressure condition to obtain a clear light brown colour filtrate. The filtrate was then used for synthesis of AgNPs via reduction of AgNO3 and kept at 4° C for further use.
Visual confirmation of synthesis of silver nanoparticles
The PJB-AgNPs synthesized from the bark extract was visually confirmed by change of colour from light yellow to dark brown (). This change in colour was due to the reduction of Ag+ to AgNPs. After that, reaction mixture was subjected to centrifugation at 10,000 rpm for 10 min followed by washing thrice and re-dispersion in ddH2O for further analysis.
UV-visible spectrophotometer analysis
UV-visible spectroscopy analysis is the immediate characterization method done after synthesis procedure by scanning AgNPs in the range of 300–700 nm. In general, this study is an indirect method to confirm synthesis of silver nanoparticles. It gives a qualitative estimation about size, shape and yield along with agglomeration state of AgNPs in suspension due to their unique optical properties.
The PJB-AgNPs were also scanned for confirmation of their synthesis and were found to show characteristic band near 420 nm (). The band observed is due to the surface plasmon resonance of the collectively oscillating electron present in the conduction band [Citation20,Citation21]. Plethora of studies has revealed that the AgNPs showing absorption maxima around 420 nm tend to have spherical shape. A bathochromic shift in the absorption peak indicates larger size of particles due to aggregation and broader peak for smaller size particles. On the other hand, particles with larger size become narrow and show increase in the absorption intensity [Citation22,Citation23].
Further, the study also revealed that increase in the absorption peak height indicates an increase in concentration of AgNPs. But as the yield of AgNPs increases, aggregation is likely to occur due to increase in collision frequency of nanoparticles [Citation6,Citation23]. So, based on above study, PJB-AgNPs synthesized herein were of spherical shape as the absorption maxima are near 420 nm.
Optimization of reaction parameters
The biosynthesis reaction of AgNPs is very sensitive and depends on certain important parameters, i.e., time of the synthesis reaction, concentration of AgNO3, volume of extract to AgNO3 and temperature of the reaction. These parameters control size, shape, yield and agglomeration state of the AgNPs due to their unique characteristic property. Here in this study, we have optimized these parameters for the synthesis reaction of PJB-AgNPs.
In case of reaction time optimization, all the reactions were performed separately at different time points starting from 10 min to 200 min. The AgNPs were centrifuged and subjected to UV-visible spectroscopy. The data revealed that at 200, 160, 180 and 80 min, large particles were obtained (). The reason for this large size could be the aggregation of smaller particles at long incubation period. Further, lower absorption peaks after 10 and 20 min indicate lower yield of synthesis of AgNPs. So, the suggested optimized time for synthesis of AgNPs could be 40 min that was used as the base for further optimization.
Figure 2. UV-vis spectra of biosynthesized PJB-AgNPs recorded as a function of (A) time, (B) concentration of AgNO3 solution, (C) concentration of extract, (D) temperature, (E) microwave and (F) after storage for 8 months.

Next optimization was done for AgNO3 concentration by taking 0.1 mM, 0.5 mM, 1 mM, 1.5 mM and 2 mM. Reactions were then analyzed by UV-vis spectroscopic scanning. In this case, the growth rate increased with increase in AgNO3 concentration. The scan data revealed that the optimum concentration of AgNO3 was expected to be 1 mM, as above this concentration, the reaction got saturated and higher peak at 2 mM AgNO3 concentration could be due to large size particles after aggregation ().
Similarly, the ratio of extract and temperature of the reaction was optimized and according to the explanation given above the extract to AgNO3 ratio of 1:20 () and 25 °C temperature () was considered suitable for synthesis of PJB-AgNPs.
Microwave-assisted synthesis of silver nanoparticles
Further, using the optimized parameters, the reactions with bark extract were conducted by irradiated microwaves. The reactions were performed at different time intervals as 30, 60, 90 and 120 s followed by scanning the samples using UV-vis spectrophotometer (). From the obtained data, it can be discerned that AgNPs can be synthesized by microwave within few seconds.
Stability study of silver nanoparticles
The stability of the PJB-AgNPs was observed after 8 months of synthesis. The UV-vis spectrophotometer scan data () suggest efficient stability of the particles with slight increase in the size due to aggregation.
Physicochemical characterization of silver nanoparticles
For physicochemical characterization, freshly synthesized AgNPs were subjected to zetasizer analysis. This study revealed the average size and charge of AgNPs in suspension, i.e., the hydrodynamic size and zeta potential. It was found that the average size of the particles was ∼54 nm along with a poly dispersive index of 0.2 which clearly indicates that the synthesized PJB-AgNPs are homogeneous in terms of size (). The obtained zeta potential (−16 mV) of these nanoparticles were found to be in considerable range ().
Figure 3. Physicochemical characterization of PJB-AgNPs. (A) Representative DLS spectrum of AgNPs, here hydrodynamic radius is ∼55 nm, (B) zeta potential of AgNPs is −16 mV, (C) SEM of AgNPs, the size of nanoparticles is ∼10–50 nm, and (D) EDS pattern of AgNPs showing prominent peak of silver.
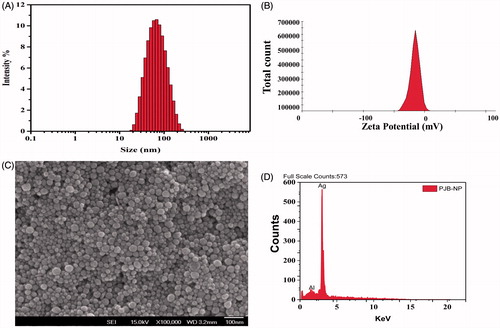
The data acquired from DLS technique are a quantitative measure of average size of thousands of particles per second. On the other hand, SEM gives qualitative data of size and morphology of small number of particles at single time point. Accordingly, SEM data revealed that synthesized nanoparticles were around 10–50 nm (). Image clearly shows that almost all these nanoparticles were spherical in shape and are not in agglomerated form. Absorption spectrum was observed at 3KeV that gave a strong peak at silver region confirming the presence of AgNPs [Citation24]. A strong peak in EDS spectra depicts characteristic absorption of silver metal (). A single peak of silver element in the graph suggests that PJB-AgNPs were synthesized without any contaminations. The presence of weak signal of Al along with strong silver peak could be due to the aluminium stub in which sample was loaded for the analysis.
Further, FTIR spectroscopy of both PJB extract and PJB-AgNPs was done. The obtained data were compared to identify the possible interaction of various functional groups that were involved in the synthesis of AgNPs as well as capping. The dried samples were used to prepare translucent disc by taking 10 mg of dried sample and 100 mg of KBr. IR scanning was done from 450 to 4000 cm−1 to obtain the spectra. IR spectrum of PJB extract shows peak at 3445 cm−1 depicting the presence of alcoholic and phenolic compound with N–H stretching of amino groups (, band A). Other functional groups present are alkanes (2908 cm−1), aromatic compounds (1422.9 cm−1), aliphatic amines (1070.6 cm−1) and alkyl halides (658 cm−1). IR spectrum of PJB-AgNPs (, band B), also indicates the presence of phenolic compounds (3477 cm−1) in synthesis of AgNPs but the upward shift in this peak indicates that phenolic compounds are responsible for the reduction into AgNPs. Aldehydes (2403.9 cm−1) and aromatic compounds (1590.9 cm−1) are also involved. Peak at 832 cm−1 depicts that primary and secondary amines are present which are responsible for the capping and stabilization of synthesized AgNPs [Citation25].
Evaluation of antibacterial activity
In vitro antibacterial assay was performed using disc diffusion method against E. coli and P. aeruginosa (PA) on LB agar plates. The zones of inhibition on each plate with various concentrations of synthesized nanoparticles were examined for the inhibitory effect on growth of bacteria. In case of E. coli, region “A” and “B” indicates negative and positive control, respectively (). The remaining discs show different concentration of AgNPs from 0.25 μg to 1 μg. The diameter of zone of inhibition was presented in mm and taken as mean ± SD of duplicates. The graph of zone of inhibition of E. coli shows that growth rate decreases as the concentration of AgNPs increases from 0.25 μg to 1 μg.
Figure 5. Antibacterial activity of PJB-AgNPs showing zone of inhibition of (A) E. coli and (B) P. aeruginosa. (C) Comparative graph for zone of inhibition of both. Here, kanamycin and autoclaved ddH2O were taken as positive and negative control, respectively.
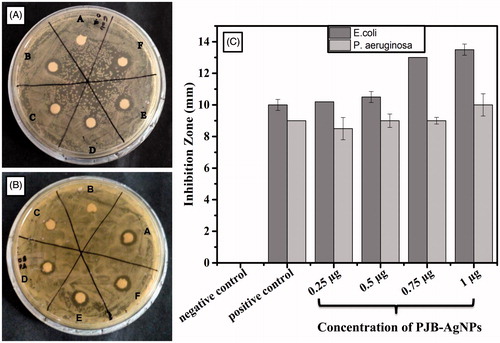
In case of PA, region “A” and “B” indicates positive and negative control, respectively (). Here the growth rate was affected in the same manner as with E. coli. It can be clearly observed from that E. coli and PA were susceptible to PJB-AgNPs in a very appreciable manner. These AgNPs show antibacterial activity as these are 10–55 nm in size so it can easily enter the nuclear material and interfere its normal processing. This study reveals that synthesized AgNPs can also surmount the problem of multi-drug resistance. From the results, it can be concluded that the antibacterial activity of these nanoparticles is dose dependent.
Evaluation of anticancer activity
The result of in vitro anticancer activity of PJB-AgNPs was evaluated against A549 cells at different concentrations (10 μg, 50 μg, 100 μg, 150 μg, 200 μg) using Alamar blue assay [Citation26]. This is one of the most sensitive and referenced assay for cytotoxicity and viability assay. It was evident from the results that PJB-AgNPs possess excellent anticancer activity. showed a decrease in cell viability when treated with AgNPs in comparison with untreated cells which was taken as control (). The graph depicts a 70% decrease in viability against A549 cells at 200 μg concentration (. This activity of AgNPs showed dose-dependent cytotoxicity, i.e., increase in anticancer activity with increase in the concentration of silver nanoparticles from 10 to 200 μg. These data suggest that PJB-AgNPs could be of immense use in medical field against cancer to certain extent.
Figure 6. Anticancer activity against A549 cells treated with different concentrations of PJB-AgNPs, showing dose-dependent anticancer activity as determined by Alamar blue assay. (A) Untreated A549 cells as control and (B) A549 cells treated with PJB-AgNPs (C) Representative graph of anticancer activity.
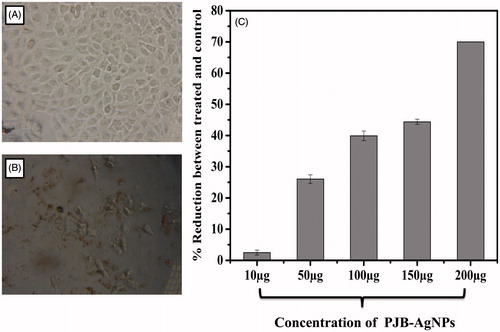
Evaluation of catalytic activity
To evaluate the catalytic activity of synthesized PJB-AgNPs, reduction reaction of 4-NP was monitored. All sets of the reactions were performed in 5-ml culture tubes. Degradation of 4-NP to 4-AP (aminophenol) occurs via reaction intermediate 4-nitrophenolate. Therefore, the reactions were performed here in three sets and monitored using UV-visible spectrophotometer by scanning 200–600 nm as shown (. The first reaction (control) possessed a mixture of only 4-NP and dH2O imparting light yellow colour that showed an absorbance peak at around 320 nm. Second reaction (control) having NaBH4 showed change in colour from light yellow to bright yellow. This was due to the formation of 4-nitro sodium phenolate that leads red shift in absorption peak to ∼400 nm [Citation27]. This reaction was then monitored for several times but no change was observed in the absorption intensity peak, suggesting that no reduction takes place in the absence of catalyst. In case of third reaction, addition of ∼30 μg of AgNPs leads to rapid decrease in the intensity of the absorption peaks (400 nm) at periodic time interval from 0 to 80 min due to the reduction of 4-NP. Here, NaBH4 acts as hydrogen donor to catalyst AgNPs that in turn act as hydrogen carrier for reduction reaction. Along with this, a new peak appears at around 296 due to the formation of 4-AP that increases periodically with solution colour changing from yellow to colourless [Citation28,Citation29]. The percent reduction of 4-NP was also shown with time that indicates 90% degradation done in 80 min. These data represent the catalytic activity of our synthesized PJB-AgNPs.
Conclusion
The work represents efficient synthesis and optimization of silver nanoparticles from bark extract of P. juliflora. To the best of our knowledge, we are the first to use P. juliflora bark for synthesis of AgNPs. The study proved the anticancer activity of AgNPs synthesized from PJB extract that can help in overcoming the problem of present scenario to some extent. Our study gives an immense benefit towards the environment through green synthesis approach. Also, the catalytic activity of PJB-AgNPs can promote its role in combating organic pollutants.
Acknowledgements
GA acknowledges receipt of RGNF fellowship from UGC, India. NG and SN acknowledge the financial assistance from Science and Engineering Research Board (SERB) and Department of Biotechnology (DBT) (grant nos. SB/FT/LS-441/2012 and 6242-P82/RGCB/PMD/DBT/SNMH/2015, Government of India.
Disclosure statement
The authors declare that they have no competing interests.
Additional information
Funding
References
- Qu D, Sun W, Chen Y, et al. Synthesis and in vitro antineoplastic evaluation of silver nanoparticles mediated by Agrimoniae herba extract. Int J Nanomed. 2014;9:1871.
- Sun W, Ding Qu Y, Chen M, et al. Enhanced stability and antibacterial efficacy of a traditional Chinese medicine-mediated silver nanoparticle delivery system. Int J Nanomed. 2014;9:5491.
- Ahmed S, Ahmad M, Swami BL, et al. A review on plants extract mediated synthesis of silver nanoparticles for antimicrobial applications: a green expertise. J Adv Res. 2016;7:17–28.
- Kathiravan V, Ravi S, Ashokkumar S. Synthesis of silver nanoparticles from Melia dubia leaf extract and their in vitro anticancer activity. Spectrochim Acta Part A Mol Biomol Spectrosc. 2014;130:116–121.
- Edison TJ, Sethuraman MG. Biogenic robust synthesis of silver nanoparticles using Punica granatum peel and its application as a green catalyst for the reduction of an anthropogenic pollutant 4-nitrophenol. Spectrochim Acta Part A Mol Biomol Spectrosc. 2013;104:262–264.
- Sathishkumar M, Sneha K, Won SW, et al. Cinnamon zeylanicum bark extract and powder mediated green synthesis of nano-crystalline silver particles and its bactericidal activity. Colloids Surfaces B Biointerfaces. 2009;73:332–338.
- Narayanan KB, Sakthivel N. Extracellular synthesis of silver nanoparticles using the leaf extract of Coleus amboinicus Lour. Mater Res Bull. 2011;46:1708–1713.
- Lukman AI, Gong B, Marjo CE, et al. Facile synthesis, stabilization, and anti-bacterial performance of discrete Ag nanoparticles using Medicago sativa seed exudates. J Colloid Interface Sci. 2011;353:433–444.
- Kaviya S, Santhanalakshmi J, Viswanathan B, et al. Biosynthesis of silver nanoparticles using citrus sinensis peel extract and its antibacterial activity. Spectrochim Acta Part A Mol Biomol Spectrosc. 2011;79:594–598.
- Singh P, Kim YJ, Wang C, et al. Weissella oryzae DC6-facilitated green synthesis of silver nanoparticles and their antimicrobial potential. Artif Cells Nanomed Biotechnol. 2016;44:1569–1575.
- Singh P, Kim YJ, Wang C, et al. The development of a green approach for the biosynthesis of silver and gold nanoparticles by using Panax ginseng root extract, and their biological applications. Artif Cells Nanomed Biotechnol. 2016;44:1150–1157.
- Ahmed Q, Gupta N, Kumar A, et al. Antibacterial efficacy of silver nanoparticles synthesized employing Terminalia arjuna bark extract. Artif Cells Nanomed Biotechnol. 2016;45:1192–1200,
- Mankamna Kumari R, Thapa N, Gupta N, et al. Antibacterial and photocatalytic degradation efficacy of silver nanoparticles biosynthesized using Cordia dichotoma leaf extract. Adv Nat Sci Nanosci Nanotechnol. 2016;7:045009.
- Arya G, Kumar N, Gupta N, et al. Antibacterial potential of silver nanoparticles biosynthesized using Canarium ovatum leaves extract. IET Nanobiotechnol. 2016. [DOI 10.1049/iet-nbt.2016.0144 6].
- Dave PN, Bhandari J. Prosopis juliflora: a review. Int J Chem Stud. 2013;1:181–196.
- Muzammil Ahmed S, Ahmed S, Tasleem F, et al. Acute systemic toxicity of four mimosaceous plants leaves in mice. IOSRPHR. 2012;2:291–295.
- Al-Shmgani HSA, Mohammed WH, Sulaiman GM, et al. Biosynthesis of silver nanoparticles from Catharanthus roseus leaf extract and assessing their antioxidant, antimicrobial, and wound-healing activities. Artif Cells Nanomed Biotechnol. 2017;45:1234–1240.
- Singh P, Kim YJ, Wang C, et al. Biogenic silver and gold nanoparticles synthesized using red ginseng root extract, and their applications. Artif Cells Nanomed Biotechnol. 2016;44:811–816.
- Al-Marhaby FA, Seoudi R. Preparation and characterization of silver nanoparticles and their use in catalytic reduction of 4-nitrophenol. WJNSE. 2016;06:29–37.
- Pradeepa M, Harini K, Ruckmani K, et al. Extracellular Bio-inspired synthesis of silver nanoparticles using raspberry leaf extract against human pathogens. Int J Pharm Sci Rev Res. 2014;25:160–165.
- Ajitha B, Reddy YAK, Reddy PS, et al. Instant biosynthesis of silver nanoparticles using Lawsonia inermis leaf extract: innate catalytic, antimicrobial and antioxidant activities. J Mol Liq. 2016;219:474–481.
- Stamplecoskie KG, Scaiano JC. Light emitting diode irradiation can control the morphology and optical properties of silver nanoparticles. J Am Chem Soc. 2010;132:1825–1827.
- Zhang W, Qiao X, Chen J. Synthesis of silver nanoparticles: Effects of concerned parameters in water/oil microemulsion. Mater Sci Eng B. 2007;142:1–15.
- Vijayaraghavan K, Nalini SPK, Prakash NU, et al. Biomimetic synthesis of silver nanoparticles by aqueous extract of Syzygium aromaticum. Mater Lett. 2012;75:33–35.
- Zayed MF, Eisa WH, Shabaka AA. Malva parviflora extract assisted green synthesis of silver nanoparticles. Spectrochim Acta Part A Mol Biomol Spectrosc. 2012;98:423–428.
- Alsalhi MS, Devanesan S, Alfuraydi AA, et al. Green synthesis of silver nanoparticles using Pimpinella anisum seeds: antimicrobial activity and cytotoxicity on human neonatal skin stromal cells and colon cancer cells. IJN. 2016;11:4439–4449.
- Wunder S, Polzer F, Lu Y, et al. Kinetic analysis of catalytic reduction of 4-nitrophenol by metallic nanoparticles immobilized in spherical polyelectrolyte brushes. J Phys Chem C. 2010;114:8814–8820.
- Prasad CH, Srinivasulu K. Catalytic reduction of 4-nitrophenol using biogenic silver nanoparticles derived from papaya (Carica papaya) peel extract. Ind Chem. 2015;1:104.
- Premkumar T, Lee K, Geckeler KE. Shape-tailoring and catalytic function of anisotropic gold nanostructures. Nanoscale Res Lett. 2011;6:547.