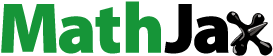
Abstract
In this effort, we provided comparative study on optimization of transfection conditions for AGS human gastric cancer cell line using two commercial non-liposomal cationic lipids. Using reporter vector pEGFP-N1, transfection efficiency of Attractene™ and X-tremeGENE HP™ transfection reagents in terms of cell densities and DNA/reagent ratios was determined in AGS cells by flow cytometry and fluorescence microscopy. In addition, influence of transfection reagents on direct cytotoxicity and cell viability was respectively, measured using lactate dehydrogenase (LDH) leakage and MTT assays. Provided that the transfection rate of 29% and the mean fluorescence intensity of 437.5, the DNA/reagent ratio of 0.4/1.5 was selected as the optimal condition using Attractene™, whereas the optimum condition using X-tremeGENE HP™ was obtained by the ratio of 1/2 with a higher transfection rate of 36.9% and an MFI of 833. Very low direct cytotoxicity (<5% and 6–9% using Attractene™ and X-tremeGENE HP™, respectively) and high cell viability (74.5–95.5% versus 68–75%) showed the biodegradable attribute for both transfection reagents. Altogether, X-tremeGENE HP™ exhibited superiority over Attractene™ as a transfection reagent for AGS cells. In the present research, we have established the optimized protocols for efficient transfection of AGS cells with potential applications in gene function and expression studies as well as gene therapy.
Introduction
Transfection is defined as a tool for introducing foreign genetic material (DNAs and RNAs) into nuclei of the target cells to produce genetically modified cells. Mammalian cell transfection is a potent tool used in biomedical and molecular biology researches. It is applicable to human therapeutic purposes as well as assisting study of gene function, gene expression and protein function. In general, strategies used for delivery of expression cassettes into mammalian cells can be categorized into three groups, including (i) viral vectors such as adenovirus, retrovirus and lentivirus; (ii) non-viral vectors (so-called chemical-mediated techniques) such as calcium phosphate, DEAE-dextran, cationic lipids and polymers and peptides; and (iii) physical methods for direct delivery of gene into the cytoplasm (e.g. electroporation, bombardment, and microinjection) [Citation1,Citation2]. Different parameters can influence the selection of gene delivery method, including transfection efficiency and performance, cell toxicity and viability, cell type (primary cell or cell line), cellular context (in vivo, in vitro, ex vivo), transgenic capacity, type of genetic material (DNA, siRNA and mRNA), application purpose(s), reproducibility, general safety, ease of use, and cost-and time-effectiveness [Citation3–5].
Chemical-mediated transfection methods are considered as one of the most commonly used approaches for gene expression in mammalian cells [Citation6]. This approach is based on ionic interaction between positively charged carrier molecules and negatively charged nucleic acids, resulting in complexation of two entities and its association with the negatively charged surface of target cells [Citation7]. The delivery of the DNA into the nucleus yet to be fully understood; however, it is supposed that the chemical/DNA complex is up-taken by cell through a receptor-mediated endocytosis and/or fluid-phase endocytosis/phagocytosis [Citation8,Citation9]. Among potential chemical carriers, cationic lipids have widely been used for the transfection of gene-based entities into mammalian cells [Citation10]. Simplicity of production and handling along with the low cost and high transfection efficiency approaching viral vectors make cationic lipids attractive vehicles for the gene delivery. They have potential application for transient and stable transfection of transgene with all size to nearly all kind of cells and tissues [Citation11]. Another advantage of these carriers is their relatively low cytotoxicity [Citation12,Citation13], even though some cationic lipids and polymers have been reported to intrinsically induce inadvertent toxicogenomics and genotoxicity [Citation14,Citation15]. Further, unlike viral vectors, they do not elicit immunologic reactions, and hence, are considered as relatively safe reagents for transfection process.
Cationic lipid transfection is based on either liposomal or non-liposomal interaction. Despite aforementioned profits, liposomal reagents entrapping designated nucleic acids may associate with some drawbacks such as serum interfering with transfection process [Citation16,Citation17], batch-to-batch variation and irreproducible outcomes. While non-liposomal reagents are able to form lipoplexes with DNA as micellar structures. Non-liposomal reagents, unlike liposomal reagents, allow the transfection process to be established in the presence of serum, thereby decreasing toxicity and increasing efficiency [Citation18]. Therefore, superior transfection efficiencies can be achieved in cell lines that are refractory to liposome-based transfection.
So far, various cationic lipids have been developed with various transfection efficiencies and cellular toxicities. Here, for the first time, we explored and compared optimal condition(s) of two commercial transfection reagents, Attractene™ and X-tremeGENE HP™, for the transfection of human gastric adenocarcinoma AGS cells. Transfection efficiencies (e.g. rate and intensity of reporter GFP expression), cell toxicity and viability were evaluated.
Material and methods
Cell culture
AGS cell line (gastric adenocarcinoma cell) was obtained from National Cell Bank of Iran (Pasteur Institute, Tehran, Iran). The cells were cultivated in Dulbecco's Modified Eagle's Medium (DMEM/F12) supplemented with 10% foetal bovine serum (FBS, Gibco, Waltham, MA), 100× GlutaMAX (Gibco, Waltham, MA). They were maintained at 37 °C in a humidified 5% CO2 incubator. The culture media were replenished every 2–3 d and regular subculture was carried out every 6–7 d by 0.25% trypsin–EDTA.
Transfection
Reporter vector pEGFP-N1 (Clontech, Mountain View, CA), encoding enhanced green fluorescence protein (EGFP), was purified from overnight culture of transformed DH5α cells using Endo-Free Plasmid Maxi Kit (Qiagen, Hilden, Germany) according to the instructions from the supplier, and then employed for the transfection of AGS cells. For this purpose, two commercial transfection reagents of Attractene™ (Qiagen) and X-tremeGENE HP™ (Roche, Basel, Switzerland) were utilized. Then, the optimal transfection conditions (i.e. cell density and DNA/transfection reagent ratio) were determined according to own manufacturer's protocols. In brief, 24 h before transfection, the cells at 80% confluence were plated into a 24-well microplate at different seeding densities of 4.0–7.0 × 104 cells/well to give 50–90% confluence on the transfection day. As shown in , different ratios of DNA to transfection reagents were prepared in serum-free Opti-Mem medium (Gibco, Waltham, MA) and incubated at room temperature for 15 min to allow the formation of polyplexes. Meanwhile, cell culture media were replaced with fresh complete media. The transfection complexes were added onto the cells in drop-wise manner and then the cells were incubated further for 48 h until the transfection efficiency analysis. All transfection experiments were performed in triplicate.
Table 1. Transfection conditions according to companies' instructions.
Flow cytometry and fluorescent microscopy
Transfection efficiency of EGFP gene was quantified by flow cytometry. Forty-eight hours post-transfection, the medium was removed and the cells were washed two times with cold phosphate-buffered saline (PBS). Next, the cells were harvested by 0.25% trypsin–EDTA, washed with PBS and resuspended in 0.5 mL PBS to be read out by flow cytometry, FACSCalibur™ (Becton Dickinson, Franklin Lakes System, NJ). Two negative controls were included for each transfection experiment as the lipid-based transfection reagents can influence the natural fluorescence of cells. Untransfected and mock transfection (cells transfected with a vector lacking of EGFP) control cells representing auto-fluorescence were included as EGFP-negative controls to adjust cutoff thresholds for the measurement of EGFP-positive cell population. For each transfection experiment, 10,000 live cells (excluding dead cells using propidium iodide staining) were analyzed using CellQuest Pro software (BD Biosciences, Franklin Lakes, NJ). Then, the transfection efficiency was represented as both transfection rate (% EGFP-positive cells to the total number of cells) and mean fluorescent intensity (MFI, level of EGFP expression per cells). In parallel, EGFP-expressing cells were visualized by an inverted fluorescence microscope, Olympus IX81 (Shinjuku, Tokyo, Japan).
Lactate dehydrogenase (LDH) cytotoxicity assay
LDH-mediated cytotoxicity of Attractene™ and X-tremeGENE HP™ reagents was performed by LDH leakage detection kit (Roche, Basel, Switzerland) according to the manual protocol from the manufacturer. Initially, the optimal cell density was determined for the subsequent assays. Briefly, various ranges of 340–100,000 cells/well in 100 μL of assay medium (i.e. RPMI 1640 containing 1% BSA) were plated across the 96-well microplate. Then, 100 μL of assay medium containing 2% Triton X-100 and 100 μL of assay medium alone, respectively, correspond to high and low LDH activity controls were added onto the cells. After 24 h incubation, the culture supernatants were collected to assay their LDH leakage activity at an absorbance of 490 nm in a microplate reader, BioTek ELx808 (BioTek, Winooski, VT). The cell density at which the difference between the low and high control is at a maximum was considered as optimal cell density.
For cytotoxicity study of transfection reagents, the cells were plated in 100 μL/well of assay medium at optimum seeding density, which was determined in the preliminary experiment. On the next day, the assay medium was removed and then fresh assay medium containing different amounts of transfection reagents was added on to the cells (), denoted as test samples. The treated cells (i.e. test samples, high and low LDH activity controls) were cultured for 24 h and then amount of LDH released into culture media was assayed at an absorbance of 490 nm in the microplate reader. The percentage of cytotoxicity was calculated by the subtraction of average absorbance values of treated samples from the absorbance values of background at 630 nm. The resulting values are substituted in the following equation, and data were presented as percentage of the control values:
(1)
(1)
Viability assay
The 3-(4,5-dimethylthiazol-2-yl)-2,5-diphenyl tetrazolium bromide (MTT) viability assay was applied to study effect of transfection reagents on the cell metabolic activity. After removing cell media required for the cytotoxicity assay, the cultured cell plates were retained for MTT assay by means of Cell Proliferation Kit (Roche, Basel, Switzerland). Based on the protocol instruction, 100 μL/well of MTT solution was added and incubated at 37 °C for 4 h. After the addition of 100 μL/well of solubilizing solution and incubation of the plates overnight, the absorbance of soluble formazan was quantified at 570 nm and then subtracted from absorbance values of background at 630 nm. The relative cell viability (%) calculated by the following equation and results were presented as percentage of control values:
(2)
(2)
Statistical analysis
Data are represented as mean ± SD of triplicate for each experiment. One-way analysis of variance (ANOVA) or Student's t-test was applied to compare differences among the experimental groups using GraphPad Prism 5.0 (GraphPad Software, La Jolla, CA). A p value less than .05 was considered statistically significant.
Results
Transfection efficiency analysis
As a standard method of measuring transfection efficiency, a reporter gene construct such as pEGFP-N1 consisting of GFP driven by the constitutively active CMV early promoter was used in all the experiments. Two key parameters of cell seeding density and DNA/transfection reagent ratio affecting transfection efficiency were optimized for gene delivery into AGS cells using two different commercial reagents, Attractene™ and X-tremeGENE HP™. Transfection efficiency was evaluated in terms of both transfection rate and MFI by flow cytometry. illustrates the flow cytometry profiles obtained from a typical transfection experiment. The live single-cell population was analyzed on side scatter (SSC) versus EGFP (FL1) density plot. Density plot of mock DNA transfection, like untransfected cells, exhibited a single EGFP-negative population indicating that both the transfection reagents had no effect on the auto-fluorescence of AGS cells. Based on these controls, gates were adjusted to locate all the un-transfected cells in region R1 and EGFP transfected cells in region R2. Subsequently, the EGFP-transfected samples were quantified by FACS and the results are presented in as transfection rate and MFI to explore the optimal transfection condition in view of cell density and DNA/transfection reagent ratio.
Figure 1. Methods used for analyzing flow cytometry data. Two control cells were prepared for data acquisition and analysis: untransfected cell control for optimizing SSC (side scatter) and FSC (forward scatter); and mock DNA control (the cells transfected by a vector without insert DNA) to subtract auto-fluorescence emitted by the transfection regents. After optimizing amplifiers, untransfected cell and mock DNA controls, and EGFP-transfected cells were read out by flow cytometry as density plot. Mock DNA density plots were analyzed based on gate regions of untransfected cell control plots to omit the auto-fluorescence of the cells, while the transfected cells were analyzed based on mock DNA gates in order to subtract fluorescence emitted by the transfection reagents. Gate regions R1 and R2 depict GFP negative and positive cells, respectively. Statistics of transfection rate and expression intensity (MFI) for each sample test were calculated and displayed as diagrams in .
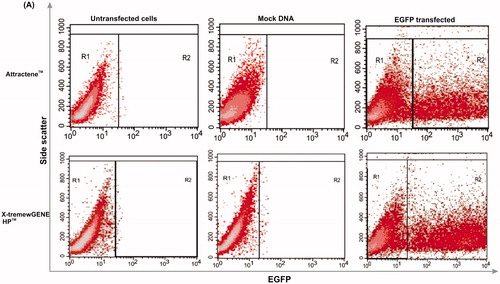
Figure 2. Transfection efficiency. Two key parameters of cell density and DNA/transfection reagent ratio were considered to evaluate transfection efficiency of AGS cells by means of transfection rate and MFI for Attractene™ (panels A and B) and X-tremeGENE HP™ (panels C and D) reagents, using BD FACSCalibur system. 48 h after transfection, the cells were trypsinized and then percentage of EGFP-expressing cells (transfection rate) and mean intensity of EGFP expression per cells (MFI) were analyzed with CellQuest Pro software (BD Biosciences, Franklin Lakes, NJ). Each value represents standard error of the mean (SEM) of triplicates. Asterisks (*) indicate statistical significance; *p < .05, **p < .01, ***p < .001 and ****p < .0001.
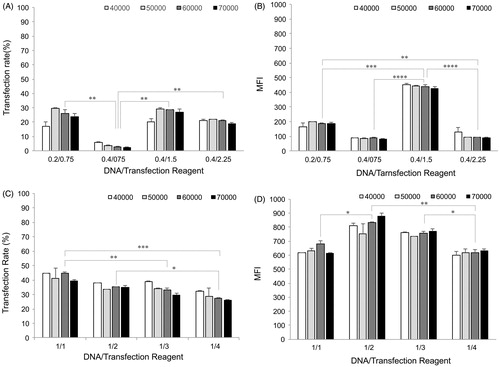
For transfection of proliferating cells, unlike stationary cell types, the optimal cell density should be determined [Citation19]. Transfection performed at different cell seeding densities (4.0–7.0 × 104 cells/well) had no significant effect (p ≥ .05) on transfection efficiency when comparing within each ratio (). This observation may be explained by transfection of all seeding densities with the same amount of transfection complex (60 and 50 μL, respectively, for Attractene™ and X-tremeGENE HP™). In this line, it was reported that the optimal DNA/transfection reagents ratio depends on the number of cells to be transfected and so with the large number of proliferating cells, higher concentrations of transfection reagents were required to obtain the maximal transfection efficiency [Citation20]. Further, higher cell seeding density can resemble stationary cells status through cell–cell contact inhibition of proliferation, and hence requires higher amount of DNA/transfection reagents [Citation21]. Taken together, a seeding density of 6.0 × 104 cells/well was selected and applied for the subsequent statistical analyses and comparisons as it provided 60–70% confluence to avoid contact inhibition and so allowing actively dividing cells.
Additionally, different DNA/transfection reagent ratios were examined to determine the highest transfection efficiency. The best condition for transfection of AGS cells using Attractene™ reagent was considered to be 0.4 μg DNA/1.5 μL reagent ratio based on the transfection rate of 29% and an MFI of 437.5 (). Among conditions used, the lowest transfection rate (2.5%) and the highest MFI (437.5) belong to DNA/reagent ratio of 0.4 μg/0.75 μL and 0.4 μg/1.5 μL, respectively, without any significant differences between other ratios. Cellular DNA uptake, the first limiting step for transfection, depends on two crucial parameters: (i) DNA–reagent condensation that indicates quantity of input DNA accessible for uptake and (ii) DNA–reagent complex concentration as a function of balance between enough DNA and reagent concentration that determines the cellular internalization capacity. It has been demonstrated that high amount of DNA is accompanied with inefficient DNA–reagent condensation because plasmid interaction and aggregation during encapsulation cause the formation of large particles difficult to be taken up by cells. Besides, high reagent concentration might promote the formation of the large number of DNA–reagent complex with lower DNA content.
The results for transfection efficiency of X-tremeGENE HP™ reagent are represented in . By combining transfection rate and MFI, the optimal condition for the transfection of AGS cells was considered to be 1/2 reagent ratio with a transfection rate of 36.9% and an MFI of 833. There was no significant difference between transfection rate of the DNA/reagent ratios of 1/1 and 1/2; however, the ratio of 1/2 enabled to transfect the cells with an MFI value higher than 1/1 ratio. Despite displaying comparable transfection efficiency with the 1/2 ratio, the ratio of 1/3 was not selected as an optimal condition because it required more transfection reagent for the cell transfection and exhibited significantly less transfection rate than the ratio of 1/1. Visually, not significantly, the transfection rate was decreased as the volume of transfection reagent increased. This could be due to the usage of limited amount of DNA with excess volumes of transfection reagent that could reduce the efficient formation of DNA–reagent complex.
EGFP expression was also visualized by fluorescence microscopy at 48 h post-transfection with Attractene™ and X-tremeGENE HP™. As shown in , X-tremeGENE HP™ resulted in both high transfection rate and intensity of EGFP expression than Attractene™ when comparing at their optimal transfection conditions.
Figure 3. Fluorescence microscopic imaging of EGFP-transfected AGS cells. Transfection efficiency of the optimal transfection conditions for Attractene™ and X-tremeGENE HP™ reagents was studied under fluorescence microscopy. Phase contrast (top panel) and fluorescence (bottom panel) micrographs at 20× magnification.
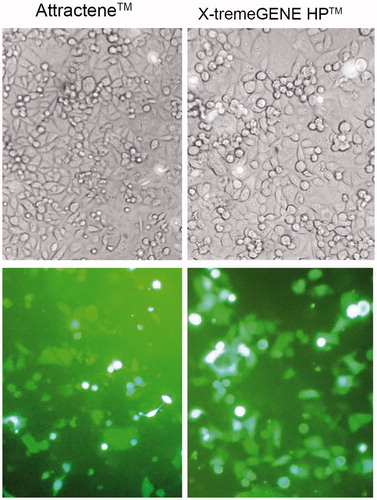
Toxicity analysis
A comparative in vitro cytotoxicity study was performed on two different transfection reagents for the gene delivery into AGS cells. The cell toxicity associated to direct membrane damage which is a consequence of potential interaction between cationic macromolecules and the cell membrane was measured by the release of cytosolic enzyme LDH to the extracellular environment (LDH leakage assay). The optimal cell density applied for LDH leakage and subsequent MTT assay was about 25,000 cells/well.
Both the transfection reagents were judged to be slightly toxic for the cells with regard to the toxicity for different ratios of Attractene™ () and X-tremeGENE HP™ () which ranged from 1.2 to 4.6% and 6 to 9%, respectively. In addition, there is no significant difference (p ≥ .05) between cell toxicities of various ratios in both transfection reagents tested.
Figure 4. Cell toxicity assay. Influence of the Attractene™ (A) and X-tremeGENE HP™ (B) transfection reagents on the cell membrane integrity was studied by LDH release into culture media. The cells were incubated with different ratios of DNA/transfection reagent for 24 h, and then, culture supernatants were applied for the assay using LDH leakage assay kit (Roche, Basel, Switzerland). Each value represents the SEM of triplicates.
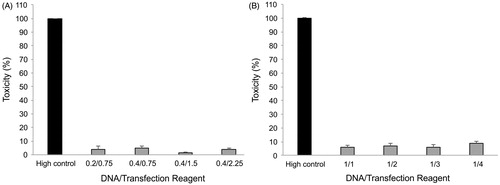
MTT viability assay
The colorimetric MTT assay was used to determine adverse intracellular effects of transfection regents on the mitochondria and metabolic activity. In the case of Attractene™, ratios of 0.2/0.75 and 0.4/0.75 had not adverse effect on the cell viability (about 95% or above), while at the lower ratios of 0.4/1.5 and 0.4/2.25, the cell viability was significantly reduced to 74.5% and 81%, respectively (). On the contrary, as shown in , X-tremeGENE HP™ was found to significantly decrease the cell viability (varying from 68% to 75% of control) at all DNA/reagent ratios. Unlike Attractene™, no significant differences in cell viability were observed with increasing transfection reagent concentration.
Figure 5. MTT viability assay. Effects of Attractene™ (A) and X-tremeGENE HP™ (B) on metabolic activity of AGS cells was determined by MTT viability assay using Cell Proliferation Kit (Roche, Basel, Switzerland). The cells were incubated with different ratios of DNA/transfection reagent for 24 h and after removing culture media for LDH leakage assay the same culture plates were applied for MTT test according to manufacturer's instructions. Each value represents the SEM of triplicates. Asterisks (*) indicate statistical significance when comparing with viability of control cells; **p < .01 and ***p < .001.
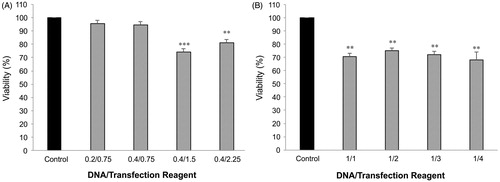
Discussion
The delivery of genes into primary and immortalized cell lines is a groundwork of molecular biology and bio-medical research and has become increasingly important in therapeutic development. Defining and optimizing parameters necessary for the transfection are critical step to evaluate gene delivery efficiency in a wide range of cells. Cell density and DNA/transfection reagent ratio along with cytotoxicity are the most important parameters to be considered for the optimization of cationic lipid-based gene delivery into target cells.
In the current study, due to straightforward practice, transfection in the presence of serum, and a very low toxicity [Citation22], we have selected two commercial non-liposomal cationic lipids (i.e. Attractene™ and X-tremeGENE HP™) for the transfection of AGS cells, as a hard-to transfect cell line, and compared their transfection efficiency, cell toxicity and viability.
Regardless of conditions used, X-tremeGENE HP™ transfection reagent showed superior transfection rate and MFI. Also considering the optimal conditions, X-tremeGENE HP™ transfection reagent produced higher transfection rate and MFI than Attractene™ reagent (36.9% and 833 versus 29% and 437.5, respectively). Despite the usage of the same cell line, discrepancy between the transfection efficiency of two cationic lipids might be attributed to the specific characteristics of the reagents (such as size, lipid type/structure, surface charge, surface hydrophobicity, material, shape and formulation) which can influence the lipid/DNA lipoplex formation and internalization mechanisms used to entry into target cells [Citation23–26]. There are a large body of studies investigating the relationship between the physicochemical characteristics of transfection reagents and efficiency of DNA delivery to cells. For example, lipoplexes with nearly neutral charge were shown to provide efficient aggregation and fusion of lipoplex with the cell membrane [Citation27]. Besides, cationic lipids were reported to produce lipoplex with DNA, and then, based on the physicochemical characteristics, internalized by the target cells through distinct endocytic mechanisms [Citation28] which could also influence the efficiency of DNA transfer. It should be noted that the size of lipoplex is a key parameter that determines internalization mechanisms and the intracellular fate. Lipoplexes, at a size range of 300 nm or less, preferentially use the clathrin-mediated pathway. However, particles with a size range of 500 nm or over can internalized into cells through caveolae-mediated pathways by which they can escape from the lysosomal degradation and hence enhance transfection efficiency [Citation29,Citation30]. Additionally, lipid type/structure is another factor specifying internalization and transcytosis pathways. Lipid transfection reagents containing neutral lipids (e.g. DOPE, DOPC and cholesterol), the so-called fusogenic lipids, could increase transfection efficiency through destabilizing endosomal membrane and releasing DNA from early endosome into cytoplasm prior to lysosomal digestion [Citation31,Citation32].
The cytotoxicity of the transfection reagents was studied by both the LDH leakage and MTT viability assays. Both transfection reagents were well tolerated by the AGS cells because of the very low LDH leakage (direct cytotoxicity) and relatively high viability. When comparing the optimal conditions, Attractene™ reagent was found to be less cytotoxic than X-tremeGENE HP™ (1.2% versus 7%) for AGS cells, although both the transfection reagents resulted in the similar viability (74.5% versus 75%). Such dissimilarities observed between the results from LDH leakage and MTT viability assays were expected. This phenomenon can be attributed to the nature of each assay employed and multiple mechanism(s) of toxicity were used by the transfection reagents. The LDH leakage assay evaluates cell membrane damage through the detection of cytoplasmic LDH enzyme released into the culture medium, while MTT viability assay indicates the number of metabolically active cells with intact and healthy mitochondria [Citation33,Citation34]. In consistence with this line, cationic lipid-based transfection reagents are able to mediate their cytotoxicity effects on cells through multiple mechanisms including direct cell membrane damage as well as adverse effect on the cellular metabolism by incorporation of non-biodegradable lipids into the biological membranes such as mitochondria [Citation35]. Consequently, when evaluating cytotoxicity of transfection reagents, in particular cationic lipid-based transfection reagents, different cytotoxicity assays enabling to identify cell compartment-specific toxicities should be applied to avoid any underestimation or overestimation.
Conclusion
Over recent decades, development of efficient chemical-mediated transfection strategies, especially, non-liposomal cationic lipids, has been extensively increased in order to improve in vitro and in vivo gene delivery procedures. The main advantages of non-liposomal cationic lipids over the most commonly used viral-based gene delivery are their straightforward practice, low toxicity, no carcinogenicity and no immunogenic reactions. In this paper, we have investigated the optimal transfection conditions of two commercially available cationic lipids for transfection of AGS cells using reporter vector pEGFP-N1. Our results demonstrate that both the cationic lipid reagents used for transfection of AGS cells possess excellent transfection performances and well-tolerable toxicity. However, X-tremeGENE HP™ was considered to be outperformed over Attractene™ in terms of transfection efficiency for AGS cells. In addition, when analyzing toxicity of transfection reagents, it would be privileged to apply different cell healthy assays in order to increase validity of the results obtained. With safety and efficacy confirmed here for X-tremeGENE HP™ transfection of AGS as a difficult-to-transfect cell line, this study emphasizes promising role for non-liposomal cationic lipids as suitable vectors for functional analysis of gene expression as well as genetic modifications.
Acknowledgements
The authors are very grateful for the technical support provided by the members of the Research Center for Pharmaceutical Nanotechnology at Tabriz University of Medical Sciences.
Disclosure statement
The authors report no conflicts of interest.
Additional information
Funding
References
- Harris J. Gene delivery and therapy strategies. Lancet. 1993;342:234.
- Walthers CM, Seidlits SK. Gene delivery strategies to promote spinal cord repair. Biomark Insights. 2015;10:11–29.
- Zhou Q, Chen JL, Chen Q, et al. Optimization of transfection parameters for ultrasound/SonoVue microbubble-mediated hAng-1 gene delivery in vitro. Mol Med Rep. 2012;6:1460–1464.
- Srinivasan C, Burgess DJ. Optimization and characterization of anionic lipoplexes for gene delivery. J Control Release. 2009;136:62–70.
- Singh A, Trivedi P, Jain NK. Advances in siRNA delivery in cancer therapy. Artif Cells Nanomed Biotechnol. 2017;19. DOI:10.1080/21691401.2017.1307210.
- Felgner PL, Ringold GM. Cationic liposome-mediated transfection. Nature. 1989;337:387–388.
- Hsu CY, Uludag H. Cellular uptake pathways of lipid-modified cationic polymers in gene delivery to primary cells. Biomaterials. 2012;33:7834–7848.
- Koynova R, Tenchov B. Cationic lipids: molecular structure/transfection activity relationships and interactions with biomembranes. Top Curr Chem. 2010;296:51–93.
- Singh J, Michel D, Chitanda JM, et al. Evaluation of cellular uptake and intracellular trafficking as determining factors of gene expression for amino acid-substituted gemini surfactant-based DNA nanoparticles. J Nanobiotechnol. 2012;10:7.
- Gheisari Y, Soleimani M, Azadmanesh K, et al. Multipotent mesenchymal stromal cells: optimization and comparison of five cationic polymer-based gene delivery methods. Cytotherapy. 2008;10:815–823.
- Zhao DD, Watarai S, Lee JT, et al. Gene transfection by cationic liposomes: comparison of the transfection efficiency of liposomes prepared from various positively charged lipids. Acta Med Okayama. 1997;51:149–154.
- Balasubramaniam RP, Bennett MJ, Aberle AM, et al. Structural and functional analysis of cationic transfection lipids: the hydrophobic domain. Gene Ther. 1996;3:163–172.
- Pozzi D, Marchini C, Cardarelli F, et al. Mechanistic evaluation of the transfection barriers involved in lipid-mediated gene delivery: interplay between nanostructure and composition. Biochim Biophys Acta. 2014;1838:957–967.
- Omidi Y, Barar J, Akhtar S. Toxicogenomics of cationic lipid-based vectors for gene therapy: impact of microarray technology. Curr Drug Deliv. 2005;2:429–441.
- Omidi Y, Barar J, Heidari HR, et al. Microarray analysis of the toxicogenomics and the genotoxic potential of a cationic lipid-based gene delivery nanosystem in human alveolar epithelial a549 cells. Toxicol Mech Methods. 2008;18:369–378.
- Zellmer S, Gaunitz F, Salvetter J, et al. Long-term expression of foreign genes in normal human epidermal keratinocytes after transfection with lipid/DNA complexes. Histochem Cell Biol. 2001;115:41–47.
- Keogh MC, Chen D, Lupu F, et al. High efficiency reporter gene transfection of vascular tissue in vitro and in vivo using a cationic lipid–DNA complex. Gene Ther. 1997;4:162–171.
- Faneca H, Simoes S, de Lima MC. Evaluation of lipid-based reagents to mediate intracellular gene delivery. Biochim Biophys Acta. 2002;1567:23–33.
- Lascombe I, Mougin P, Vuillermoz C, et al. Gene transfer into subcultured endometrial cells using lipofection. Biotechniques. 1996;20:88–91.
- Pelisek J, Engelmann MG, Golda A, et al. Optimization of nonviral transfection: variables influencing liposome-mediated gene transfer in proliferating vs. quiescent cells in culture and in vivo using a porcine restenosis model. J Mol Med (Berl). 2002;80:724–736.
- Simoes S, Pires P, Düzgünes N, et al. Cationic liposomes as gene transfer vectors: barriers to successful application in gene therapy. Curr Opin Mol Ther. 1999;1:147–157.
- Karmali PP, Chaudhuri A. Cationic liposomes as non-viral carriers of gene medicines: resolved issues, open questions, and future promises. Med Res Rev. 2007;27:696–722.
- Vercauteren D, Vandenbroucke RE, Jones AT, et al. The use of inhibitors to study endocytic pathways of gene carriers: optimization and pitfalls. Mol Ther. 2010;18:561–569.
- Goncalves C, Mennesson E, Fuchs R, et al. Macropinocytosis of polyplexes and recycling of plasmid via the clathrin-dependent pathway impair the transfection efficiency of human hepatocarcinoma cells. Mol Ther. 2004;10:373–385.
- Clark PR, Hersh EM. Cationic lipid-mediated gene transfer: current concepts. Curr Opin Mol Ther. 1999;1:158–176.
- Keith MB, Farrell PJ, Iatrou K, et al. Use of flow cytometry to rapidly optimize the transfection of animal cells. Biotechniques. 2000;28:148–154.
- Almofti MR, Harashima H, Shinohara Y, et al. Cationic liposome-mediated gene delivery: biophysical study and mechanism of internalization. Arch Biochem Biophys. 2003;410:246–253.
- Kirkham M, Parton RG. Clathrin-independent endocytosis: new insights into caveolae and non-caveolar lipid raft carriers. Biochim Biophys Acta. 2005;1746:349–363.
- Hoekstra D, Rejman J, Wasungu L, et al. Gene delivery by cationic lipids: in and out of an endosome. Biochem Soc Trans. 2007;35:68–71.
- Prabha S, Arya G, Chandra R, et al. Effect of size on biological properties of nanoparticles employed in gene delivery. Artif Cells Nanomed Biotechnol. 2016;44:83–91.
- Hui SW, Zhao YL, Ross P, et al. The role of helper lipids in cationic liposome-mediated gene transfer. Biophys J. 1996;71:590–599.
- Panahi Y, Farshbaf M, Mohammadhosseini M, et al. Recent advances on liposomal nanoparticles: synthesis, characterization and biomedical applications. Artif Cells Nanomed Biotechnol. 2017;45:788–799.
- Fotakis G, Timbrell JA. In vitro cytotoxicity assays: comparison of LDH, neutral red, MTT and protein assay in hepatoma cell lines following exposure to cadmium chloride. Toxicol Lett. 2006;160:171–177.
- Lochmann D, Weyermann J, Georgens C, et al. Albumin-protamine-oligonucleotide nanoparticles as a new antisense delivery system. Part 1: physicochemical characterization. Eur J Pharm Biopharm. 2005;59:419–429.
- Leventis R, Silvius JR. Interactions of mammalian cells with lipid dispersions containing novel metabolizable cationic amphiphiles. Biochim Biophys Acta. 1990;1023:124–132.