Abstract
Curcumin is a promising wound healing agent but its clinical application is limited due to hydrophobicity and lack of stability. In this article, we report the results of a study on wound healing efficacy of curcumin conjugated to hyaluronic acid (HA) which is a natural polysaccharide known to influence the healing process. Studies on proliferation, antioxidant activity and scratch wound healing carried out in human keratinocyte cells revealed that HA-conjugated curcumin treatment enhanced cell proliferation, decreased oxidative damage induced by H2O2 and also improved migration of cells in scratch wounds as compared to treatment with native curcumin. HA conjugated curcumin exhibited bactericidal activity in dark and phototoxicity when irradiated with blue light against antibiotic resistant bacteria. Furthermore, wound healing efficacy studied in diabetic mice demonstrated that topical application of the conjugate on wounds led to better healing as compared to treatment with HA-free curcumin and HA alone. These results suggest that HA conjugation is a promising formulation of curcumin for enhancing its healing efficacy.
Curcumin, a low molecular weight polyphenolic compound found in the Indian spice turmeric (Curcuma longa) is well known for its multiple pharmacological applications including wound healing [Citation1,Citation2]. Healing effects are attributed due to its antioxidant, anti-inflammatory and antimicrobial properties [Citation3–5]. Additionally, curcumin exhibits photodynamic properties. This has been exploited for enhancing its anti-microbial efficacy and hastening healing of infected wounds [Citation6]. Studies have shown that application of curcumin on normal and burn wounds improve healing by enhancing epithelial regeneration, fibroblast proliferation, vascular density, collagen deposition and reorganization [Citation7,Citation8]. Curcumin has also been shown to accelerate healing of cutaneous wounds in streptozotocin induced diabetic rats and genetically modified diabetic mice by reducing inflammatory cytokines, elevating anti-inflammatory cytokines, antioxidant enzymes and improving neovascularization [Citation9,Citation10].
Despite potential wound healing benefits, therapeutic efficacy of curcumin is limited when it is administered in conventional form because of its poor aqueous solubility and low stability under physiological condition [Citation11]. To address these issues, various nanoformulations of curcumin have been prepared for wound healing applications. These include curcumin incorporated nanocomposite hydrogels [Citation12], polymeric micelles prepared from poly(ethylene glycol)-b-poly(-caprolactone) [Citation13], poly(caprolactone) nanofibres and poly (lactic-co-glycolic acid) polymer [Citation14]. Nevertheless, use of biomaterials which have inherent wound healing properties may have an added advantage.
Hyaluronic acid (HA), a naturally occurring polysaccharide, composed of N-acetyl-d-glucosamine and d-glucuronic acid has gained a lot of attention in tissue regeneration and wound healing applications [Citation15]. It is one of the main components of the extra cellular matrix, has good viscoelastic properties and also facilitates cell migration [Citation16]. Additionally, being hydrophilic it helps in hydration of skin. Nevertheless, biological effect of HA is dependent on its molecular weight [Citation17]. High molecular weight HA species with >1000 kDa are space-filling, anti-angiogenic and immunosuppressive [Citation17–20], whereas lower molecular weight HA-oligosaccharides (∼1.35–7.2 kDa) and polymers fragments (20–600 kDa) have been reported to stimulate angiogenesis [Citation21], induce inflammation [Citation22], regulate cell adhesion [Citation23] and promote proliferation of keratinocytes [Citation24] and fibroblasts [Citation25].
Recently, healing efficacy of HA based nano carrier gel core hyalurosome incorporating curcumin was studied in burn wounds of rats [Citation26]. Results showed that nanocarrier accelerated healing by increasing skin penetration and dermal localization of curcumin also reduced the scar formation due to combined effect of HA and curcumin. In another study, it was demonstrated that curcumin incorporated in nanovesicles prepared using polyanion sodium hyaluronate could repair the chemically induced skin damage in mice faster as compared to free curcumin and curcumin loaded in liposomes due to improvement in its anti-inflammatory activity [Citation27].
In addition to curcumin incorporated HA based nano carriers/vesicles, there is a considerable interest in use of HA conjugates of curcumin [Citation28,Citation29]. Studies have shown that conjugation of curcumin with HA improve water solubility as well as stability of curcumin at physiological pH. This has been attributed to formation of nanomicelles in aqueous conditions [Citation28,Citation29]. Conjugate of curcumin and high molecular HA has also shown enhanced cytotoxicity against fibroblast cells as compared to native curcumin [Citation28]. Additionally, compared to nano carriers, preparation of these nanoformulations is simpler. Since lower molecular weight HA (∼1.35–−600 kDa) influence angiogenesis, inflammation and proliferation of skin cells, it is of interest to investigate the wound healing activity of curcumin conjugated to HA. The effect of HA conjugated curcumin on healing of wounds have not been studied so far.
In the present study, we have investigated the wound healing efficacy of curcumin conjugated to HA in in vitro and in vivo models of wound. Effect of HA–cur on cell proliferation, cell migration and its antioxidant efficacy was studied in human keratinocyte (HaCaT) cells. We also evaluated the antimicrobial potential of HA conjugate on methicillin resistant Staphylococcus aureus (MRSA) in dark and after exposure to blue light. In vivo efficacy of the conjugate was evaluated in excisional wound of diabetic mice. For this study, HA of molecular weight 250 kDa was used as this molecular weight polymer has shown beneficial effect on the wound healing [Citation30].
Materials and methods
Materials
Hyaluronic acid (HA, molecular weight 250 kDa) was purchased from Creative PEG Works. Curcumin, N, N-Dicyclohexylcarbodiimide (DCC) 4-dimethylaminopyridine (DMAP), ketamine, xylazine and streptozotocin (STZ) were obtained from Sigma–Aldrich, India. Dimethyl sulfoxide (DMSO) was purchased from Spectrochem Pvt. Ltd., Mumbai, India. Dulbecco’s minimum essential medium/Nutrient Mixture F-12 Ham (DMEM/F12, 1:1 mixture), foetal bovine serum (FBS), antibiotic and antimycotic solutions, 3(4,5-dimethylthiazolyl-2)-2,5 diphenyltetrazolium bromide (MTT) and tryptose soya broth (TSB) were procured from Himedia, Mumbai, India. All other chemicals used were of analytical grade.
Synthesis and characterization of hyaluronic acid–curcumin conjugate (HA–cur)
HA–cur conjugate was synthesized according to the procedure described in [Citation28] with some modifications. Briefly, 1% (W/V) HA was prepared in 1:1 water/DMSO mixture. To this, DCC and DMAP were added. The ratio of HA: DCC: DMAP was maintained as 10:1:0.5. The carboxylic groups of HA were activated by stirring it for 1 h at room temperature. To this mixture, 1 ml of 0.4 mM of curcumin prepared in DMSO was added and the mixture was stirred for about 24 h at room temperature. Subsequently, the mixture was poured into a solution of ethanol to precipitate the HA–cur conjugate. Supernatant and the precipitate were separated. The precipitated conjugate was dialyzed against distilled water for 48 h to remove the unbound entities and then the sample was lyophilized. The concentration of curcumin in the conjugate was estimated by dissolving a known quantity of HA–cur in DMSO and the absorbance of the conjugate was measured using absorption spectrophotometer (Cintra 20, GBC, Australia). The values were compared with the standard calibration curve obtained by measuring absorbance of known concentrations of curcumin dissolved in DMSO.
The average size, polydispersity and zeta potential of the HA–cur conjugate (1 mg/ml) dissolved in distilled water were measured by dynamic light scattering measurements using a Brookhaven 90Plus particle and zeta potential analyzer (USA).
Antioxidant activity
The antioxidant activity of HA, curcumin and HA–cur was evaluated by phosphomolybdenum method as described by Prieto et al. [Citation31]. Briefly, an aliquot (0.1 ml) of different concentrations of HA, curcumin/HA–cur was mixed with 1 ml of reagent solution (0.6 M sulphuric acid, 28 mM sodium phosphate and 4 mM ammonium molybdate). All the samples were incubated at 90 °C for 90 min. After cooling, absorbance of the samples was measured at 695 nm. Blank solution contained 1 ml of reagent solution and the solvent used for dissolving the samples. For calibration, ascorbic acid was used as standard. Antioxidant activities of curcumin and HA–cur were estimated from the standard graph; the antioxidant activity is expressed as the number of equivalents of ascorbic acid (µmol/g).
Effect of HA–cur on cell proliferation and antioxidant activity
HaCaT cells (NCCS, Pune, India) were maintained in high glucose (25 mM) containing DMEM/F12 (1:1) media supplemented with 10% FBS, antibiotic and antimycotic solutions at 37 °C in humidified CO2 incubator. For toxicity studies, 25,000 cells/well were seeded into 96-well plates. After 24 h, growth medium was replaced with medium containing different concentrations of curcumin, or HA–cur. Subsequent to incubation for 24 h, viability of cells was determined by MTT assay following the method described by Mosmann [Citation32]. Briefly, 100 µl of medium containing 10 µl MTT (5 mg/ml) was added into each well and incubated for 4 h. Subsequently, MTT was removed and formazan crystals formed were dissolved by adding 150 µl of DMSO into each well. The absorbance of the resulting solution was recorded at 570 nm using micro plate reader (Bio-Tek Instruments, Vermont). Change in absorbance of treated cells was compared with untreated cells (control) and represented as relative change in % viability with respect to control.
For studying antioxidant activity, cells grown in 96-well microplate for 24 h were incubated in a medium containing hydrogen peroxide (H2O2, 300 µM) for 3 h along with HA (42 or 84 µg/ml; concentration equivalent to HA present in the conjugate), curcumin or its HA conjugate containing equivalent concentration of curcumin. Following incubation, cells were washed with PBS and MTT assay was performed as described above. The antioxidant effect was evaluated by comparing the viability of H2O2 treated cells incubated with and without curcumin/HA–cur.
Antibacterial activity
Antibacterial activity of HA–cur in dark and in combination with blue light was assessed on methicillin resistant Staphylococcus aureus (MRSA, ATCC 43300) and compared with native curcumin. Bacteria were maintained routinely by subculturing on tryptone soya agar (TSA) plates. For experiments, a colony of the bacteria was inoculated into TSB and grown aerobically at 37 °C for 20 h using a shaker incubator. 1 ml of overnight culture was centrifuged at 8000 rpm and the cell pellet was resuspended in PBS. The optical density of culture was adjusted to 0.4 (108cells/ml) at 600 nm and the cell suspension was divided into four aliquots (1 ml each). One of the aliquots was incubated with curcumin (25 µM, prepared in DMSO), the other with HA–cur containing similar concentration of curcumin for 2 h in dark at 37 °C under aerobic conditions along with untreated control. For phototoxic experiments, subsequent to incubation, 0.5 ml of cell suspensions treated with curcumin or HA–cur and untreated controls were transferred to 24-well plate and each well was exposed to blue light (25 J/cm2). The light source used for irradiation was a LED (Applied Optical Technologies, India) emitting light of wavelength ∼420 nm. Power output measured by a power meter (Ophir) at the cell surface was 36 mW. All the treated and untreated cultures were diluted serially in PBS. An aliquot of different dilutions of bacterial suspensions were plated on TSA and incubated for 37 °C for 24 h. The number of colonies formed was counted and surviving fraction was expressed as the ratio of colony forming units (CFU) counted in samples treated with curcumin/HA–cur in dark or after irradiation with light to CFU in untreated sample (control).
In vitro scratch-wound healing assay
HaCaT Cells (1 × 105) were seeded in petri dishes (35 mm) in complete medium (10% FBS) and grown until the monolayer was confluent. The medium of the monolayer was replaced with medium containing 1% FBS and incubated for 2–4 h. Subsequently, wounds of size ∼1.0 mm were created by mechanically scratching the monolayer with a 100 µl sterile pipette tip. Detached cells and debris were washed with medium not containing serum. The scratched monolayers were incubated with HA (42 µg/ml), curcumin (5.0 µM) or HA–cur conjugate containing similar concentration of curcumin in a medium containing 2% FBS and incubated for different time intervals (24–96 h). The wound closure of the treated cells was monitored using an inverted phase contrast microscope (Leica, Germany) equipped with a CCD camera. The wound images of the treated groups were recorded at different time points and wound area was measured using image J with 4X objective. Results were recorded in square pixels and then converted to area.
In vivo wound healing
Animals
All the experimental procedures involving animals were approved by the Institutional Animal Ethics Committee, in accordance with the guidelines of the Committee for Purpose of Care and Supervision of Experimental Animals (CPCSEA), Department of Environment and Forests, Government of India. The animals were housed individually in cages with free access to food, water and maintained on a 12 h light/dark cycle at 22 °C (± 2 °C). All the procedures involving wounds were carried out in anesthetized conditions and animals were kept on warm cotton pads for recovery from anaesthesia. Animals were euthanized by cervical dislocation. All research animals were treated humanely.
Diabetes induction, wound creation and application of HA–cur
A total of twenty six Swiss albino mice (male, 25–28 g) were used for all experiments. Diabetes was induced by injecting multiple doses of STZ intraperitoneally according to the protocol described in Ref. [Citation33]. The blood glucose concentration was measured by a commercial glucometer (One touch horizon, Johnson and Johnson) for 3 months to ensure sustained hyperglycaemia. Mice with blood glucose level >250 mg/dl were considered as diabetic. The diabetic mice at 4 weeks post-STZ treatment were used for wound creation. For this, in mice anaesthetized by intraperitoneal injection of Ketamine (80 mg/kg) and Xylazine (10 mg/kg) cocktail, the dorsal skin was shaved, treated with a depilatory cream, and then cleaned with povidone–iodine solution followed by 70% alcohol wipe. A wound of dimension ∼1.2 × 1 cm was created at the back of each mouse by excising the skin down to panniculus carnosus. Mice with wounds were divided into (I) untreated control, (II) HA alone, (III) curcumin (native) and (IV) HA–cur groups. Wounds of mice belonging to groups II, 20 µl of 210 µg/ml of HA, for groups III and IV, 20 µl of the 25 µM of curcumin or HA–cur were applied topically at 24 h intervals on day 1–3 post-wounding (p.w.). Treated and untreated wounds of the animals were photographed to monitor the changes. Wound closure for treated and untreated animals was calculated from the images recorded on day 0 and day 14 using image J.
Histological analysis
For wound healing studies after recording the images of wound on day 14, treated and untreated animals were scarified under anaesthesia and wounds with surrounding tissues were collected on day 14th post-treatment. Specimens were fixed in 10% formaldehyde, embedded in paraffin, tissue sections of 5 µm were prepared using microtome and stained with haematoxylin and eosin as per the standard method and visualized under a light microscope (Olympus, Japan) at 4 × magnification.
Statistical analysis
Data was represented as mean ± SEM of three experiments. Student t-test and one way ANOVA was used to compare the statistical difference among the groups. p Values <.05 was considered as statistically significant.
Results and discussion
Preparation and characterization of HA–cur conjugate
Low molecular weight HA (oligomers ∼1.5–7.2 kDa and fragmented HA polymers ∼20–600 kDa) have shown positive influence on endothelial cells [Citation21], macrophages [Citation22], keratinocytes [Citation24] and fibroblasts [Citation25]. As these cells play an important role in different phases of wound healing it was of interest to study the effect of HA conjugated curcumin on healing of diabetic wounds. HA was conjugated to curcumin as reported previously [Citation28]. Conjugation was confirmed by observations of red shift in the absorption peak of curcumin (Supplementary Figure S1), signature of ester linkage around ∼1340 nm due to C–O stretching frequency in the FTIR spectra (Supplementary Figure S2) [Citation28] and changes in the fluorescence life time of curcumin (Supplementary Table S1). The average content of curcumin per mg of conjugate estimated from the standard calibration curve was about 0.043 mg. The average size of HA–cur in PBS was about 320 nm and polydispersity was 0.36. Zeta potential of the conjugate was −53 ± 1.0 mV. As reported previously [Citation28], stability of HA–cur in aqueous media was found to be higher as compared to HA free curcumin.
Effect of HA–cur on cell viability
To investigate if HA–cur conjugate has any effect on keratinocyte viability/proliferation, HaCaT cells were incubated with different concentrations of HA–cur for 24 h and viability was measured by MTT assay. Comparison of viability of cells treated with either HA–cur or free curcumin and untreated (control) cells for 24 h is shown in . Viability of cells treated with low concentrations of curcumin (up to 5 µM) did not change significantly. However, significant (p < .05) loss of viability (∼45%) was observed in cells treated with curcumin at a concentration of 20 µM. This observation was consistent with other reports wherein it has been shown curcumin at high concentration exhibits antiproliferative effect by promoting apoptosis [Citation34,Citation35]. In contrast, viability of cells treated with HA–cur increased with increase in curcumin concentration up to 10 µM as compared to untreated control and native curcumin. Beyond this concentration there was no significant change in viability. To further verify if HA alone had any effect on cell proliferation, cells were incubated for 24 h with HA (curcumin free) equivalent to the concentrations (2.5–10 µM curcumin) present in the conjugate (). About 10–15% enhancement in proliferation was observed in cells treated with HA as compared to untreated cells. These results were similar to that of HA–cur treated group. Observed enhancement at low concentrations of HA–cur appeared to be due to the additive effect of curcumin and HA. However, at higher concentrations (10 and 20 µM) effect was comparable to HA (alone) treated cells. This suggests that HA conjugation reduces the antiproliferative effect of native curcumin. Previous studies have shown that HA binding to CD44 receptors induces redistribution of lipid rafts due to which multiple signalling molecules gets recruited and facilitate transduction of signals for cell proliferation [Citation36,Citation37]. It has been shown that HA activate P2X7 purinergic receptor upon binding [Citation38]. Activation of this receptor has been shown to increase mitochondrial calcium and promote cell proliferation [Citation39]. Taken together our results, suggest that curcumin bound to HA enhances cell proliferation.
Figure 1. Viability of HaCaT cells incubated for 24 h with different concentrations of (A) free curcumin and HA–cur conjugate, (B) HA present in the conjugate. Viability was assessed by MTT assay. Viability represented is relative to untreated control. Data represented are mean ± SE of three experiments. *,#p < .05 compared to untreated control group.

Antioxidant activity
Since several studies carried out previously have shown that HA exhibits antioxidant properties under in vitro and in vivo conditions [Citation40,Citation41], it was of interest to study the antioxidant activity of HA–cur. Antioxidant capacitates of curcumin, its HA conjugate and HA alone were determined by measuring the formation of phosphomolybdenum complex and were compared with ascorbic acid, a standard antioxidant (). HA alone (42 µg/ml) showed antioxidant activity corresponding to ∼5 µmol ascorbic equivalents. With increase in HA concentration (84 µg/ml), activity increased to ∼9 µmol ascorbic equivalents. For curcumin, antioxidant capacity was ∼16 and ∼29 µmol ascorbic equivalents at 5 and 10 µM concentration, respectively. Compared to HA alone and native curcumin, antioxidant activity of HA–cur was significantly (p < .05) higher. At 5 µM antioxidant capacity for HA–cur was ∼22 µmoles which increased to ∼38 µmoles ascorbic equivalents at 10 µM. These results suggest that HA conjugation have additive effect on antioxidant activity of curcumin.
Figure 2. (A) Antioxidant activity of HA, curcumin and HA–cur determined by phosphomolybdenum method. (B) Viability of HaCaT cells treated with H2O2 (300 µM) for 3 h along with HA/curcumin HA–cur. Cell viability was determined by MTT assay. Data represented are mean ± SE of three experiments. #p > .5 with respect to H2O2 treated cells; *p < .05 with respect to H2O2 treated cells.
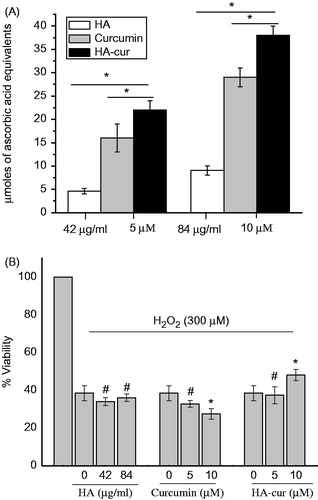
Antioxidant activity of curcumin and its HA conjugate and HA (curcumin free) was also evaluated in cells subjected to oxidative stress by H2O2. shows the viability of cells treated with H2O2 (300 µM) for 3 h with and without curcumin or HA–cur. Treatment of cells with only 5 and 10 µM of curcumin or HA–cur for 3 h did not affect the viability (data not shown). However, viability of cells treated with H2O2 reduced to 40% of the control after 3 h incubation. While no significant change in viability (p > .05) was noted in cells incubated with H2O2 along with 5 µM curcumin viability reduced slightly when concentration of curcumin was increased to 10 µM. Decrease in cell viability in curcumin treated cells at higher concentration (10 µM) suggests that curcumin do not have protective effect against H2O2 and these results are consistent with previous study wherein it has been shown that curcumin enhances H2O2 induced cell injury by promoting apoptosis [Citation42]. Interestingly, no significant change in viability was observed in H2O2 treated cells incubated with HA alone at both the concentrations (42 or 84 µg/ml). Lack of antioxidant effect of HA could be due to degradation of HA by H2O2 [Citation43]. On the other hand, when H2O2 treated cells were incubated with HA–cur (10 µM), viability increased by ∼15% as compared to cells treated without the conjugate although no change was observed at lower concentration (5 µM). This suggests that HA–cur protect the cells from oxidative damage and however effect is dependent on the concentration of HA present in the conjugate. These results are consistent with the enhanced antioxidant activity of HA–cur observed in cell free system (). However, protective effect observed by HA–cur conjugate seem to be due to synergetic effect of HA and curcumin and not due to an additive effect as observed in the cell free system. This difference could be due to the variation in the reaction system, reactive oxygen species generated and their quenching by HA, curcumin and HA–cur.
Antibacterial activity of HA conjugate in dark and after exposure to light
In order to study if conjugation of curcumin to HA has any influence on its anti-bacterial effect and phototoxicity, bactericidal efficacy of HA conjugate was evaluated in dark and after exposure to blue light. Antibacterial activity of the conjugate was evaluated in MRSA as this is the common bacteria responsible for causing infection in wounds of diabetic subjects and also difficult to treat [Citation44]. shows the surviving fraction of cells treated with 25 µM of curcumin and its HA–cur conjugate for 2 h in dark and after exposure to light fluence 25 J/cm2. Treatment of bacteria with curcumin and HA–cur in dark for 2 h reduced the surviving fraction to ∼99.5% (>1 log) and ∼90% (1 log), respectively. It should be noted that HA alone (210 µg/ml) in dark did not exhibit any bactericidal effect. Exposure of bacteria to light alone led to about ∼95% killing. However, when curcumin and HA–cur treated bacteria were exposed to blue light reduction in bacteria was more than ∼5 log and 4 log, respectively. Curcumin induces bactericidal effect by inhibiting cellular proteins, FtsZ [Citation45] and sortase A, that are involved in bacterial cytokinesis and cell adhesion [Citation46]. Higher toxicity observed in curcumin treated cells as compared to HA–cur could have been contributed by DMSO which is used as solvent for dissolving curcumin and also because size of conjugate is larger their uptake may be slower. Observed enhancement in toxicity of curcumin and HA–cur when irradiated with blue light is due to photochemical generation of reactive oxygen species in the presence of oxygen [Citation47] and confirm that phototoxicity of curcumin is not affected by HA conjugation.
In vitro scratch wound assay
To study the effect of curcumin, HA–cur and HA alone on migration of cells scratch wound assay was carried out in HaCaT cells grown in monolayer. Treatment induced effect on wound closure was evaluated by measuring the differences in area of wounds at 0 h (immediately after wounding) and at different time points (). It is evident from the results that as compared to untreated control and curcumin, HA and HA–cur treated scratch wounds healed faster. At 24 h, wound closure was ∼23, ∼21 and ∼26% in untreated control, HA alone and HA–cur treated groups, respectively, whereas in curcumin treated group change in wound area was only about ∼18%. At 96 h, in curcumin treated wounds, wound closure was lower (∼75%) than the untreated control (∼86%), which might be due to curcumin induced inhibition on migration of Keratinocytes [Citation48]. On the other hand, wound closure was ∼98 and ∼99% in HA (alone) and HA–cur treated group. Faster wound closure observed in HA–cur and HA treated group could be mainly due to increase in migration of cells induced by HA and not due to increase in proliferation as in these experiments serum concentration was kept very low. It has been shown previously that dermal wounds treated with HA of 250 kDa enhance expression of CD44 and RHAMM receptors which are known to activate pathways that promote cellular migration and proliferation [Citation30]. Also, studies have shown that HA enhances expression of tight junction proteins like ZO-1 which play crucial role in cell-cell adhesion and formation of the epidermal barrier during wound healing [Citation38].
Figure 4. Phase contrast microscopic images of HaCaT cell scratch wounds with different treatments. Monolayer of cells was wounded manually using 100 µl of pipette tip, after washing, incubated with HA (42 µg/ml), curcumin (5 µM) and HA–cur (containing 5 µM curcumin) in culture medium containing 2% FBS for different durations along with untreated control.
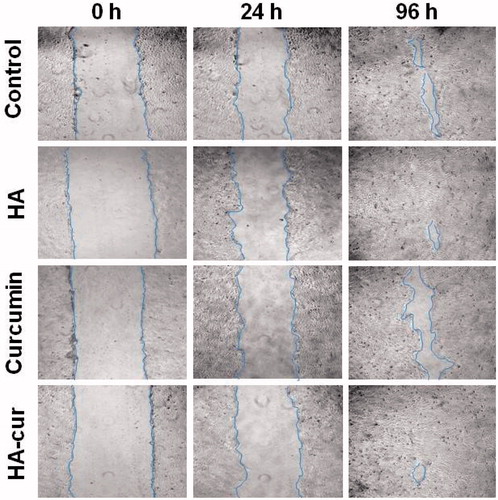
Effect of curcumin and HA–cur on healing of diabetic wounds
In order to further understand the efficacy of HA–cur for healing impaired wounds under in vivo conditions, curcumin and its HA conjugate and HA were topically applied on wounds of diabetic mice. shows the comparison of untreated wounds and wounds of mice treated with HA (210 µg/ml) and 25 µM of curcumin and its HA conjugate on day 0 and 14. In untreated wounds, wound closure was only ∼24% (with respect to initial wound area, day 0), whereas in curcumin treated group change in wound area was ∼55% and in HA treated group wound closure was ∼81%. On the other hand, in HA–cur treated group almost complete healing was observed and wounds closure was ∼96% ().
Figure 5. Effect of HA, curcumin and HA–cur conjugate on healing of wounds of diabetic mice. (A) Representative images of wounds on day 0 and 14; (a, b) untreated, treated with (c, d) curcumin, (e, f) HA, (g, h) HA–cur conjugate. Scale bar: 1 cm. (B) Wound closure (%) on day 14 of different treatment groups. Wound closure (%) was calculated with respect to initial wound area on day 0. In each group, six mice were used, **p < .05 (ANOVA) between different groups. (C) Histological evaluation of untreated and wounds treated with curcumin, HA and HA–cur conjugate. HA (210 µg/ml), curcumin (25 µM) or HA–cur containing equivalent concentration of curcumin was applied topically on excisional wounds of diabetic mice for 3 days at an interval of 24 h. Scale bar: 200 µm.
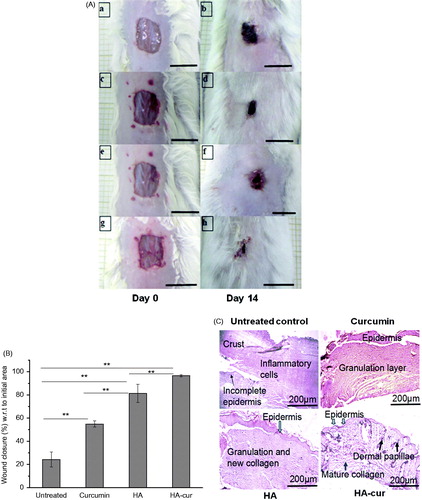
To further confirm the healing efficacy of different formulations of curcumin histological investigations were carried out on wounds subjected to different treatments on day 14 (). Histology of untreated wounds showed lot of inflammatory cells on upper regions, crust, incomplete epidermis even on day 14. On the other hand, curcumin treated wounds showed fewer inflammatory cells and increased deposition of dermal layers. These signatures implied improvement in healing as compared to untreated wounds and were consistent with previous studies wherein it has been shown that curcumin due to its anti-inflammatory and antioxidant activities [Citation9], improves wound healing by increasing collagen deposition [Citation10], fibronectin production, myofibroblast contraction [Citation49]. In comparison to curcumin treated wounds, HA treated group showed well-formed granulation tissue rich in fibroblast, collagen and re-epithelialization was almost complete. These observations were consistent with the previous report wherein it has been shown that HA (250 kDa) improves wound repair due to increase in proliferation of cells of epidermis and dermis through activation of CD44 receptors [Citation30]. Compared to HA and curcumin, HA–cur treated group showed well-formed dermal layer with mature collagen, dermal papillae and complete re-epithelialization. Improved wound healing activity of curcumin conjugated to HA could be due to the combined effect of HA and curcumin on proliferation and migration of skin cells as well as collagen deposition in dermis. Consistent with this we have also observed increased proliferation of cells () as well as migration of keratinocytes in scratch wounds () and antioxidant activity (). Also, well-formed dermal layer observed in HA–cur treated wounds could be due to HA induced increase in collagen deposition. It has been shown that HA of MW 250 kDa enhances wound repair by increasing synthesis of collagen I and II as well as TGF-β1 expression which promote myofibroblast differentiation to fibrogenic phenotype [Citation30].
In summary, our findings on in vitro wound model of human keratinocytes cells and wound model of diabetic mice show that by conjugating HA of molecular weight (250 kDa) to curcumin, its wound healing activity is improved. The results of in vitro studies suggest that improvement in wound healing activity of curcumin is due to HA induced increase in proliferation, migration of cells and enhancement in antioxidant activity. These effects contribute to faster healing of wounds. Also, HA conjugated curcumin shows antibacterial efficacy which is enhanced when irradiated with light. Results suggest that HA conjugated curcumin if incorporated in suitable matrix has a potential utility for treatment of diabetic wounds.
Supplementary_information.doc
Download MS Word (616.5 KB)Disclosure statement
No potential conflict of interest was reported by the authors.
References
- Gupta SC, Patchva S, Aggarwal BB. Therapeutic roles of curcumin: lessons learned from clinical trials. AAPS J. 2013;15:195–218.
- Pulido-Moran M, Moreno-Fernandez J, Ramirez-Tortosa C, et al. Curcumin and health. Molecules. 2016;21:264.
- Akbik D, Ghadiri M, Chrzanowski W, et al. Curcumin as a wound healing agent. Life Sci. 2014;116:1–7.
- Thangapazham RL, Sharad S, Maheshwari RK. Skin regenerative potentials of curcumin. Biofactors. 2013;39:141–149.
- Teow SY, Liew K, Ali SA, et al. Antibacterial action of curcumin against Staphylococcus aureus: a brief review. J Trop Med. 2016;2016:2853045.
- Hegge AB, Andersen T, Melvik JE, et al. Formulation and bacterial phototoxicity of curcumin loaded alginate foams for wound treatment applications: studies on curcumin and curcuminoides XLII. J Pharm Sci. 2011;100:174–185.
- Sidhu GS, Singh AK, Thaloor D, et al. Enhancement of wound healing by curcumin in animals. Wound Repair Regen. 1998;6:167–177.
- Mehrabani D, Farjam M, Geramizadeh B, et al. The healing effect of curcumin on burn wounds in rat. World J Plast Surg. 2015;4:29–35.
- Kant V, Gopal A, Pathak NN, et al. Antioxidant and anti-inflammatory potential of curcumin accelerated the cutaneous wound healing in streptozotocin-induced diabetic rats. Int Immunopharmacol. 2014;20:322–330.
- Sidhu GS, Mani H, Gaddipati JP, et al. Curcumin enhances wound healing in streptozotocin induced diabetic rats and genetically diabetic mice. Wound Repair Regen. 1999;7:362–374.
- Sharma RA, Gescher AJ, Steward WP. Curcumin: the story so far. Eur J Cancer. 2005;41:1955–1968.
- Mehanny M, Hathout RM, Geneidi AS, et al. Exploring the use of nanocarrier systems to deliver the magical molecule; Curcumin and its derivatives. J Control Release. 2016;225:1–30.
- Merrell JG, McLaughlin SW, Tie L, et al. Curcumin -loaded poly(epsilon-caprolactone) nanofibres: diabetic wound dressing with anti-oxidant and anti-inflammatory properties. Clin Exp Pharmacol Physiol. 2009;36:1149–1156.
- Chereddy KK, Coco R, Memvanga PB, et al. Combined effect of PLGA and curcumin on wound healing activity. J Control Release. 2013;171:208–215.
- Neuman MG, Nanau RM, Oruña-Sanchez L, et al. Hyaluronic acid and wound healing. J Pharm Pharm Sci. 2015;18:53–60.
- Dicker KT, Gurski LA, Pradhan-Bhatt S, et al. Hyaluronan: a simple polysaccharide with diverse biological functions. Acta Biomater. 2014;10:1558–1570.
- Karbownik MS, Nowak JZ. Hyaluronan: towards novel anti-cancer therapeutics. Pharmacol Rep. 2013;65:1056–1074.
- Papakonstantinou E, Roth M, Karakiulakis G. Hyaluronic acid: a key molecule in skin aging. Dermatoendocrinol. 2012;4:253–258.
- Kavasi RM, Berdiaki A, Spyridaki I, et al. HA metabolism in skin homeostasis and inflammatory disease. Food Chem Toxicol. 2017;101:128–138.
- Monslow J, Govindaraju P, Puré E. Hyaluronan – a functional and structural sweet spot in the tissue microenvironment. Front Immunol. 2015;6:231.
- Slevin M, Kumar S, Gaffney J. Angiogenic oligosaccharides of hyaluronan induce multiple signaling pathways affecting vascular endothelial cell mitogenic and wound healing responses. J Biol Chem. 2002;277:41046–41059.
- McKee CM, Lowenstein CJ, Horton MR, et al. Hyaluronan fragments induce nitric-oxide synthase in murine macrophages through a nuclear factor kappaB-dependent mechanism. J Biol Chem. 1997;272:8013–8018.
- Oertli B, Beck-Schimmer B, Fan X, et al. Mechanisms of hyaluronan induced up-regulation of ICAM-1 and VCAM-1 expression by murine kidney tubular epithelial cells:hyaluronan triggers cell adhesion molecule expression through a mechanism involving activation of nuclear factor-kappa B and activating protein-1. J Immunol. 1998;161:3431–3437.
- Bourguignon LY, Wong G, Xia W, et al. Selective matrix (hyaluronan) interaction with CD44 and RhoGTPase signaling promotes keratinocyte functions and overcomes age-related epidermal dysfunction. J Dermatol Sci. 2013;72:32–44.
- David-Raoudi M, Tranchepain F, Deschrevel B, et al. Differential effects of hyaluronan and its fragments on fibroblasts: relation to wound healing. Wound Repair Regen. 2008;16:274–287.
- El-Refaie WM, Elnaggar YS, El-Massik MA, et al. Novel curcumin-loaded gel-core hyaluosomes with promising burn-wound healing potential: development, in-vitro appraisal and in-vivo studies. Int J Pharm. 2015;486:88–98.
- Manca ML, Castangia I, Zaru M, et al. Development of curcumin loaded sodium hyaluronate immobilized vesicles (hyalurosomes) and their potential on skin inflammation and wound restoring. Biomaterials 2015;71:100–109.
- Manju S, Sreenivasan K. Conjugation of curcumin onto hyaluronic acid enhances its aqueous solubility and stability. J Colloid Interface Sci. 2011;359:318–325.
- Li J, Shin GH, Chen X, et al. Modified curcumin with hyaluronic acid: combination of pro-drug and nano-micelle strategy to address the curcumin challenge. Food Res Int. 2015;69:202–208.
- Damodarasamy M, Johnson RS, Bentov I, et al. Hyaluronan enhances wound repair and increases collagen III in aged dermal wounds. Wound Repair Regen. 2014;22:521–526.
- Prieto P, Pineda M, Aguilar M. Spectrophotometric quantitation of antioxidant capacity through the formation of a phosphomolybdenum complex: specific application to the determination of vitamin E. Anal Biochem. 1999;269:337–341.
- Mosmann T. Rapid colorimetric assay for cellular growth and survival: application to proliferation and cytotoxicity assays. J Immunol Methods. 1983;65:55–63.
- Sahu K, Sharma M, Dube A, et al. Topical antimicrobial photodynamic therapy improves angiogenesis in wounds of diabetic mice. Lasers Med Sci. 2015;30:1923–1929.
- Zhao R, Yang B, Wang L, et al. Curcumin protects human keratinocytes against inorganic arsenite-induced acute. Oxid Med Cell Longev. 2013;2013:412576.
- Balasubramanian S, Eckert RL. Keratinocyte proliferation, differentiation, and apoptosis – differential mechanisms of regulation by curcumin, EGCG and apigenin. Toxicol Appl Pharmacol. 2007;224:214–219.
- Föger N, Marhaba R, Zöller M. Involvement of CD44 in cytoskeleton rearrangement and raft reorganization in T cells. J Cell Sci. 2001;114:1169–1178.
- Ghatak S, Misra S, Toole BP. Hyaluronan constitutively regulates ErbB2 phosphorylation and signaling complex formation in carcinoma cells. J Biol Chem. 2005;280:8875–8883.
- Ghazi K, Deng-Pichon U, Warnet JM, et al. Hyaluronan fragments improve wound healing on in vitro cutaneous model through P2X7 purinoreceptor basal activation: role of molecular weight. PLoS One. 2012;7:e48351.
- Adinolfi E, Callegari MG, Ferrari D, et al. Basal activation of the P2X7 ATP receptor elevates mitochondrial calcium and potential, increases cellular ATP levels, and promotes serum-independent growth. Mol Biol Cell. 2005;16:3260–3272.
- Ke C, Sun L, Qiao D, et al. Antioxidant acitivity of low molecular weight hyaluronic acid. Food Chem Toxicol. 2011;49:2670–2675.
- Campo GM, Avenoso A, Campo S, et al. The antioxidant and antifibrogenic effects of the glycosaminoglycans hyaluronic acid and chondroitin-4-sulphate in a subchronic rat model of carbon tetrachloride-induced liver fibrogenesis. Chem Biol Interact. 2004;148:125–138.
- Chen X, Zhong Z, Xu Z, et al. No protective effect of curcumin on hydrogen peroxide-induced cytotoxicity in HepG2 cells. Pharmacol Rep. 2011;63:724–732.
- Rinaudo M, Lardy B, Grange L, et al. Effect of mannitol on hyaluronic acid stability in two in vitro models of oxidative stress. Polymers. 2014;6:1948–1957.
- Reveles KR, Duhon BM, Moore RJ, et al. Epidemiology of methicillin-resistant Staphylococcus aureus diabetic foot infections in a large academic hospital: implications for antimicrobial stewardship. PLoS One. 2016;11:e0161658.
- Rai D, Singh JK, Roy N, et al. Curcumin inhibits FtsZ assembly: an attractive mechanism for its antibacterial activity. Biochem J. 2008;410:147–155.
- Park BS, Kim JG, Kim MR, et al. Curcuma longa L. constituents inhibit sortase A and Staphylococcus aureus cell adhesion to fibronectin. J Agric Food Chem. 2005;53:9005–9009.
- Araújo NC, de Menezes RF, Carneiro VS, et al. Photodynamic inactivation of cariogenic pathogens using curcumin as photosensitizer. Photomed Laser Surg. 2017;35:259–263.
- Krausz AE, Adler BL, Cabral V, et al. Curcumin-encapsulated nanoparticles as innovative antimicrobial and wound healing agent. Nanomedicine. 2015;11:195–206.
- Mani H, Sidhu GS, Kumari R, et al. Curcumin differentially regulates TGF-beta1, its receptors and nitric oxide synthase during impaired wound healing. Biofactors. 2002;16:29–43.