Abstract
Context: Due to their renewal and potency, umbilical cord blood (UCB) stem cells have the ability to proliferate and serve as an attractive alternative source for bone marrow transplantation. However, insufficient number of haematopoietic stem cells (HSCs) in UCB is still a major constraint in clinical applications.
Objective: In vitro expansion of stem cells on fibronectin (Fn)-coated poly-L-lactic acid (PLLA) scaffold can be a proper way to overcome this limitation.
Materials and method: UCB CD133 + cells were isolated by magnetic cell sorting (MACS), and then the flowcytometry method was used for analysing CD133 + cells. Confirmed cells were seeded on the Fn-coated PLLA scaffold; also, collagen-coated PLLA scaffold, PLLA scaffold and two-dimensional (2D) culture system were expanded for 7 days. During this time, we used the flowcytometric method for analysing CD133 + cells and real-time PCR for the expression level of CXCR4 gene. The number of total cells and CD133 + cells, as well as MTT assay and colony-forming unit (CFU) assay were evaluated.
Results: Flowcytometry data indicated that the purity of CD133 + before expansion was 93%. After 7 days, there was higher number of CD133 + cells on the Fn-coated PLLA scaffold compared to other groups. Moreover, results of MTT and colony assays showed higher viability and proliferation of CD133 + on the Fn-coated PLLA scaffold. Also, the quantity of CXCR4 gene expression increased compared to other groups.
Discussion: The Fn-coated scaffold was the most effective scaffold for proliferation and improved the adhesiveness to the scaffold.
Conclusion: The Fn-coated PLLA scaffold could be a suitable in vitro mimicry niche over a 2D system.
Introduction
Haematopoietic stem cells (HSCs) are ancestors of most kinds of blood cells that have the opportunity both for self-renewal and differentiation. They are obtained from bone marrow, peripheral blood and umbilical cord blood (UCB) [Citation1]. Recently, HSCs transplantation (HSCsT) from UCB has been considered for treating both malignant and non-malignant haematologic disorders. UCB was previously considered as a waste material, but today, it is regarded as a beneficial supply of blood stem cells and provides an alternative method to bone marrow transplantation. UCB transplantation has at the very least two benefits over bone marrow transplantation. The first benefit is the lower rate of rejection reactions, graft-versus-host disease (GVHD) in patients receiving UCB, as compared to bone marrow; second is its lesser requirement for human leucocyte antigen (HLA)-antigen matching [Citation2–4]. A significant disadvantage of UCB transplantation, however, is low quantity of cells in one single unit. The blood obtained from an individual umbilical cord does not contain as numerous HSCs as does the blood obtained from a bone marrow donation [Citation5]. Thus, to overcome these problems and enhance the amount of cord blood stem cells, ex vivo expansion of these cells is the important strategy [Citation6–8].
It must be considered for ex vivo or in vitro expansion of UCB HSCs that in nature HSCs come in the microenvironment on an extracellular matrix (ECM) named “niche”. The niche features a main role in physical and chemical symptoms; it can control the amount of stem cells within the body and also prevent them from multiplying [Citation9–12]. Three-dimensional (3D) scaffolds in tissue engineering research by utilizing biochemical materials such as nanoscaffolds are rapidly growing. 3D scaffolds are similar to the niche and possess some major properties, including biocompatiblity, injectablity and biodegradability. They are employed for providing progressed surface to volume ratio of cultures. These factors allow scaffolds to enhance the efficiency of the culture, provide structural support, improve cell-to-cell contact, mimic the in vivo environment and allow cells to influence their private microenvironments [Citation13,Citation14]. Here, we made an effort to imitate HSCs’ microenvironment or niche in 3D scaffolds. These nanoscaffolds might be fabricated from different polymers such as polydimethylsiloxane, poly-L-lactic acid (PLLA), poly lactic-co-glycolic acid, polycaprolactone and polyethylene terephthalate.
PLLA is a biodegradable polymer, degradation of which results in lactic acid. In this study, this scaffold has been used as a 3D scaffold for ex vivo or in vitro expansion of HSCs. Furthermore, as 3D scaffolds modification with ECM molecules has been introduced to boost cell adhesion and control the next cellular reactions [Citation6,Citation15,Citation16], the common strategy in modifying cell dependency and proliferating subsequent cellular response including pre-coating these surfaces with molecules such as fibronectin (Fn) and collagen was used [Citation15,Citation17–19].
We also hypothesized that modifying the PLLA scaffolds surface with such adhesion molecules had a beneficial influence on in vitro expansion of UCB CD133 + stem cells.
Materials and methods
Sample collection and preparation
UCB units were collected from normal full-term pregnant women after obtaining informed consent from the Iranian Blood Transfusion Organization. The collected samples were diluted with hydroxyethyl starch (HES) in the ratio of 1:6 to eradicate red blood cells (RBCs).Then, by utilizing Ficoll-Hypaque(Pharmacia-Amersham, Piscataway, density 1.077 g/mL) with centrifuge at 300 g for 10 min at room temperature, the diluted sample was separated for some layers. Following that, the low-density mononuclear (MNC) jointing layer was collected and washed twice with phosphate buffer saline (PBS)/Ethylenediaminetetraacetic acid (EDTA).
Isolation of umbilical cord blood CD133 + cells
CD133 + cells were isolated using magnetic cell sorting (MACS) technology (MiltenyiBiotec, CA, USA) based on the manufacturer’s instruction. MNCs were incubated with 100 μl blocking solution and 100 μl CD133 microbeads (MiltenyiBiotec, CA, USA) for 1 h at 4 °C in dark. The incubated cells with CD133 microbeads were centrifuged at 300 g for 5 min at 4 °C and resuspended in PBS buffer.
Positive selection was carried out by Large separation (LS) columns. Purity of the isolated CD133 + cells was certified by flowcytometry analysis.
Flowcytometry analysis
Flowcytometry analysis was performed to ascertain the confirmation of the isolated CD133 + cells before and after expansion.
About 1 × 105 isolated CD133 + cells were subjected to 10 µl phycoerythrin conjugated CD133 monoclonal antibody (mAb) (MiltenyiBiotec, Auburn, CA). After incubation at 4 °C in dark for 30 min with mAb, the cells were fixed with 2% paraformaldehyde. Isotope control was used to set compensation and confirm the specificity. The CD133 + labelled fixed cells analysis was performed using a Fluorescence-activated cell sorting (FACS) calibre (BD Biosciences, USA).
PLLA scaffold preparation and surface modification
Nanofibrous PLLA scaffolds were fabricated using an electrospinning technique. PLLA(Sigma) was dissolved in chloroform (9% w/w) and added directly to N,N-dimethylformamide (Sigma, Steinheim, Germany) solution (10:1). Random nanofibrous scaffolds were obtained using low (100 rpm) speed rotating disk.
For modifying the surface of the PLLA scaffolds, plasma treatment was performed by a low frequency plasma generator of 40 kHz frequency with a cylindrical quartz reactor (Diener Electronics, Germany). Pure oxygen was introduced into the reaction chamber of the system at 0.4 mbar pressure and purged for 5 min before beginning the treatment; subsequently, the glow discharge was ignited for 5 min.
Then, the PLLA scaffolds were sterilized in 70% ethanol, washed by PBS and dried overnight under sterile condition. The scaffolds were placed in 24-well polystyrene plates. For protein grafting, the plasma-coated scaffolds were covered in 1–(3-dimethylaminopropyl)-3-ethylcarbodiimide/N-hydroxysuccinimide (Merck, Germany), crosslinking an agent solution (5 mg/ml) for 24 h and rinsed with distilled water. Moreover, one category of the scaffolds was coated in 50 μg/ml Fn solution (Nutacon BV, Netherlands) and another category of the scaffolds was coated in 50 μg/ml collagen solution (Nutacon BV, Netherlands) for 24 h. Following that, the scaffolds were rinsed with distilled water.
Expansion of CD133 + cells on PLLA scaffolds and 2D culture system
The CD133 + cells were cultured in an Fn-coated PLLA scaffold, a collagen-coated PLLA scaffold, an unmodified PLLA scaffold and a 2D culture system with a 250 µl serum-free cell culture medium stem span (stem cell technologies, USA) containing 100 ng/ml stem cell factor, 100 ng/ml fms related tyrosine kinase 3 (FLT-3) ligand and 100 ng/ml thrombopoietin (Gibco, USA) and antibiotic/antimycotic (100 μg/ml penicillin G sodium and 100 μg/ml streptomycin) at density of about 5 × 104 cells, and then incubated for 7 days in a humidified atmosphere with 5% CO2 at 37 °C.
Scanning electron microscopy (SEM)
To judge the cell scaffold constructs, i.e. proliferation and adhesion of the cells, the cell loaded scaffold specimens from day 0 as a control and day 7 cultures were prepared for SEM. For this purpose, the CD133 + cells were washed with phosphate buffered saline (PBS). The cell-seeded scaffolds were rinsed with PBS and fixed in gelutaraldehyde 2.5% for 1 h. For dehydration, the scaffolds were put into some gradient alcohol concentration and then dried; gold was splattered in vacuum, and examined using SEM.
Colony assay
The 5 × 103 scaffold-expanded cells were harvested from each well before (day 0) and after expansion (day 7). Then, these cells were utilized in 3 ml of methylcellulose medium (H4435, stem cell technology, Canada) plated in a 35-mm culture dish (Grainger). The cell’s dish with two uncovered dishes containing 3–4 ml sterile water was put into a 100-mm culture dish and covered. The sterile water dish was served to steadfastly keep up the humidity essential for colony development. H4435 contains 1% methylcellulose in Iscove's Modified Dulbecco's Medium (IMDM) (IMDM, sigma, Germany). After 14 days incubation at 37°C and 5% CO2, colony-forming unit (CFU)-GM, BFU-E/CFU-E and CFU-GEMM (colony-forming unit of granuiocyte/erythrocyte]macrophage/megakaryocyte; the multilineage HSCs) colonies were counted.
MTT assay
To determine the CD133 + cells viability, MTT assay was performed 3, 5 and 7 days after cell culturing. Briefly, 5 mg/ml 3–(4, 5-dimethylthiazol-2-yl)-2, 5-diphenyltetrazolium bromide (MTT) (Sigma) was added to 3 × 103 cells seeded on the PLLA scaffold and the Fn-coated and collagen-coated PLLA scaffolds in each well of the 96-well plate. Then, the culture was maintained for another 4 h. The formazan was dissolved in dimethyl sulphoxide and the optical density at 570 nm was measured employing a microplate reader (Thermo Scientific, Waltham, MA, USA).
Gene expression analysis
To evaluate the adhesion ability of the expanded UCB CD133 + cells to the scaffold, CXCR4 gene expression was assessed by qPCR. RNA isolated by Trizol (Sigma, Germany) protocol. Briefly, 1 ml Trizol was added and mixed well with every 106 cell; then, chloroform addition and centrifugation were carried out. Isopropanol was added to the aqueous phase, and after the centrifugation, the supernatant was removed and only the RNA plate was washed by 75% ethanol. Also, the cDNA synthesis was performed by Thermo Scientific Kit. After the synthesis of cDNA, 1 μlcDNA was added and mixed by a real-time master mix (Ampilicon, Denmark), gene-specific primers for CXCR4 and a glyceraldehyde 3-phosphate dehydrogenase (GAPDH) control gene. Subsequently, it was absolutely watered up to 10 μl and put into the real-time PCR (ABI, USA). The primer sequences were as follows for the CXCR4 gene:
CXCR4-F TGAACCCCATCCTCTATGCTT
CXCR4-R GATGAATGTCCACCTCGCTTT
And for the GAPDH gene (as the intrinsic control),
GAPDH-F: TGCACCACCAACTGCTTAGC
GAPDH -R: GGCATGGACTGTGGTCATGAG
Statistical analysis
Statistical analysis was performed using SPSS (V. 17.0) software and p < .05 was considered significant.
Results
Isolation of CD133 + cells and confirmation
After isolation of the CD133 + cells, they were counted. The CD133 + cells were about 2 × 106 (), meaning that the CD133 + cells constitute 25% of the total MNC ().
Figure 1. (A) Isolated CD133 + cells at day 0 counted by Haemocytometer. (B). Comparison of total number of MNC and CD 133+ after isolation.

Purity of the isolated CD133 + cells was evaluated by the flowcytometry method just after the isolation. The acquired result was 93% that was proper enough for continuing the downstream steps (). After the 7-day expansion, the CD133 + cells purity was measured in the 3D scaffolds and 2Dculture system, as shown in ).
CD133 + cells expansion on 3D PLLA scaffold and 2D culture system
The effects of the Fn and collagen scaffolds on the expansion and adhesion to the scaffold CD133 + cells were evaluated in a 0-, 3-, 5-, and 7-day culture. During this period, the count of the CD133 + cells cultured in the 2D culture system increased (by trypan blue). Cell counting in the Fn and collagen-coated PLLA scaffolds showed significant decrease in the number of the CD133 + cells () (p < .05).
Scaffold morphology and cell culture on scaffold
The morphology of the PLLA scaffolds was demonstrated by SEM micrographs. showed a well-fabricated PLLA scaffold, representing a huge surface to volume ratio like the natural ECM.
Figure 4. (A). SEM photographs of the PLLA scaffold before expansion. (B). SEM micrographs of CD133+adhesion on Fn-coated PLLA scaffold on day 7. (C). SEM micrographs of CD133+adhesion on collagen-coated PLLA scaffold on day 7. (D). SEM micrographs of CD133+adhesion on PLLA scaffold on day 7.
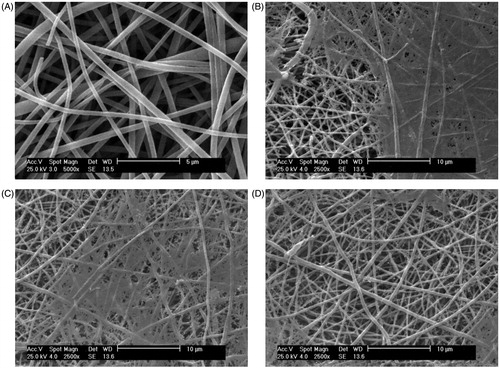
Moreover, showed the CD133 + cells’ growth and adhesion to the Fn- and collagen-coated PLLA scaffolds on the day 7. The SEM micrographs showed that the cells adhered and spread on the surface of the nanofibre. This was an obvious evidence for the cells’ good interaction and integration with the surrounding fibres.
MTT assay
After 3, 5 and 7 days of cell culturing on the 3D culture systems, the viability and proliferation of the CD133 + cells were determined by the MTT assay. The test showed that more viable cells existed in the scaffolds after a week of culture, making them quite suitable for the CD133 + cells growth. After the day7 of the culture, the value of absorbance in all the 3D scaffolds was highest in comparison to the other days. This indicated that the viability, growth and proliferation of the CD133 + cells increased on the 3D scaffolds during this period ().
Colony assay
Colony assay was performed to evaluate clonogenic potential of the expanded cells (). CFU assays were performed on the cells expanded on the Fn-coated PLLA scaffold, collagen-coated PLLA scaffold, PLLA scaffold and 2D culture system before and after the expansion. In this assay, 5000 expanded cells at the days 0 and 7 of the expansion were harvested and added to 2 ml of methylcellulose medium. After 14 days, results revealed that the cells expanded on the 3D nanofibre scaffold before and after the expansion yielded higher total colony counts compared with the 2D plate condition ().
Figure 6. (A). Colony assays from experiments on Fn-coated PLLA. (B). On collagen-coated PLLA. (C). PLLA scaffold and.
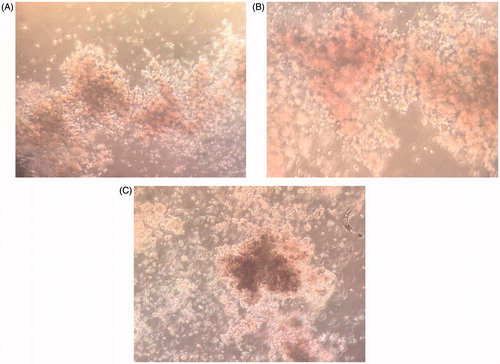
Figure 7. (A). CFU numbers (CFU-GM, CFU-GM, CFU-G, and CFU-GEMM) based on total CFU analysed for day-0 cells expanded in different culture system after 14 days. (B). CFU numbers (CFU-GM, CFU-GM, CFU-G, CFU-GEMM) based on total CFU analysed for day-7 cells expanded in different culture system after 14days. (C). Effects of culture system on 2D culture system and PLLA nanofibre scaffold (3D) (2D versus 3D) on CFU colony count.
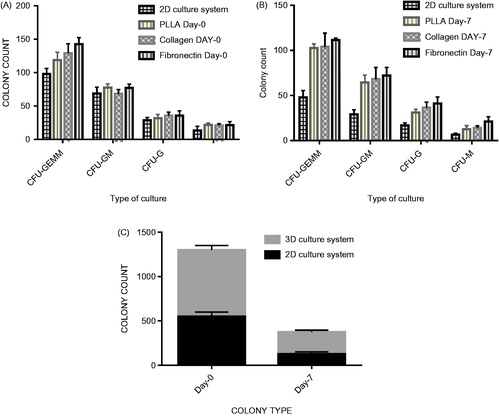
Table 1. CFU Colony count of CD133 + expanded cells on Fn-coated, collagen-coated, PLLA scaffold and 2D culture system.
Assessment of CXCR-4 expression
To estimate the expression level of adhesion agents in the expanded cells, evaluating the CXCR4 gene expression could display the homing condition. The CXCR-4 relative expression in the Fn-coated scaffold, collagen-coated scaffold, PLLA scaffold and 2D culture system increased 8.5-, 6.8- and 2.3-fold, respectively ().
Discussion
HSCsT could be the transplantation of multipotent HSCs and has been used as a typical treatment for haematological disorders. Given that cord blood is easier to collect than bone marrow and considering the presence of stem cells and an immature immune system in cord blood, transplant immunology has low rate of rejection. Despite all the benefits, UCB contains a limited number of stem cells. One of the best solutions is the ex vivo or in vitro expansion of UCB stem cells, focusing on maintaining the self-renewal ability and stemness. Designing 3D systems for the in vitro expansion of UCB HSCs should incorporate a minimum of one of many naive options to stimulate the HSC niche because UCB HSCs cannot stay alive for a number of years without the niche [Citation4,Citation5,Citation9,Citation20]. To optimize the in vitro expansion of UCB HPCs, this 3D culture system must mimic the niche via coating systems with ECM components.
In conventional 2D culture systems performed in plates, the importance of cell–cell interaction and stem cell niches in cell expansion was not considered [Citation12,Citation13,Citation21].
In this study, in vitro-like situations comprised of Fn and collagen-coated 3D PLLA scaffolds were compared with the 2D culture system. After the 7-day expansion period, the percentage of the expanded CD133 + cells on 3D systems as well as the PLLA scaffold, collagen-coated PLLA scaffold and Fn-coated PLLA scaffold was 0.71, 0.66, 0.61 compared to 2D culture systems, respectively. However, the MTT assay absorbance value of the expanded cells on the above-mentioned scaffolds at 570 nm was 2.2, 3.6 and 5.5 higher than those cultured on 2D culture systems, respectively. At first glance, it seemed that the 3D cultured UCB CD133 + cells were found to proliferate more slowly compared with 2D ones; however, the MTT assay data showed opposite results; higher absorbance at 570 nm demonstrated higher viability in the 3D scaffold in comparison to the 2D group. A possible reason could be that the nanosize structures promoted cell adhesion, function, proliferation and attachment to the scaffold. Thus, they could not be suspended on the culture medium and also, were not counted by trypan blue. Cell-cell interactions and mimicking of the niche physiochemical properties in the 3D scaffold, which elevated the surface to volume ratio in order to increase cell interaction, might be the causes of this process. This is while in the 2D cell culture system, the interaction capacity between adjacent cells was low and limited. In a similar study, Zeltinger et al. investigated the effect of pore size and void fraction on cellular adhesion and proliferation, the results of which were consistent with our results [Citation12,Citation22–25]. In addition, flowcytometric analyses illustrated less differentiation in 3D scaffold cells in comparison to the 2D culture system.
Also, results of CFU assays confirmed that a 3D culture has greater ability of colonization compared with a 2D culture. In fact, the Fn-coated scaffold was more able to maintain the stemness of HSCs. Among the 3D scaffolds, the Fn- and collagen-coated scaffolds had more total colonies than the 2D cell culture system, and the CFU-GEMM output was higher in 3D cultures when compared with their 2D counterparts. Ehring et al. appointed the frequency of CFU-granulocyte macrophages (GM) in cells recovered from cytomatrix cultures using traditional CFU assays and observed a 2.6-fold increase in the number of CFU-GMs compared with their baseline number.
Also, as collagen and Fn are regarded as major ECM proteins, and given that the principal components found in the endosteal part of the bone marrow niche comprise the tissue stroma and basement membrane, are associated with cell ECM interactions and interact with cell surfaces, we coated our scaffold with these proteins. Some other properties of these proteins are low immunogenicity, porous structure, good permeability, biocompatibility and biodegradability. Thus, all our above results were reasonable. Our data might also partially explain the tendency of HSCs to migrate into the Fn- and collagen-coated 3D scaffolds, proliferate on them and improve adhesiveness. It seems that the 3D scaffold coated with Fn had great convenience in HSCs expansion in comparison to the collagen-coated 3D scaffold. However, both of them showed better capability of cells proliferation when compared to the 2D culture system. In a similar study, Celebi et al. investigated the effects of ECM proteins on the growth of haematopoietic progenitor cells [Citation26,Citation27]. The results also confirmed that the Fn-coated nanofibre had more ability than the collagen-coated nanofibre to steadfastly continue cells adhesion. It was suggested that the collagen was a very important ECM for CD133 + cells adhesion. However, Fn, besides that, could supply better environment for these cells, as the SEM images prepared direct evidence for this claim [Citation17,Citation19,Citation27].
CXCR4 gene is a crucial factor for homing and engraftment of stem cells; therefore, investigation of its expression is very important. Denning et al. showed that the expression of CXCR4 decreased after a 10-day culture period in the 2D culture system. In contrast, Ehring et al. showed that this receptor was expressed at high level of CD34 cells in the 3D cytomatrix cell culture mode [Citation21,Citation28,Citation29]. In this study, we evaluated the CXCR4 expression in the PLLA, Fn and collagen-coated scaffold in comparison to the 2D culture system. We observed the CXCR4 expression after 7 days of expanding on 3D scaffolds increased significantly. Our results confirmed Denning et al.’s results about increase in the CXCR4 expression in 3D scaffolds compared to the 2D cell culture model [Citation28,Citation29].
In conclusion, results of our investigations confirmed that the efficiency of 3D systems to expand cell numbers is more than that of traditional plastic culture dishes. We also proved employment of ECM molecules and the PLLA scaffold as a simple, efficient, and cost-effective system for the expansion and/or manipulation of human HSCs. PLLA scaffolds were also very efficient for the proliferation and adhesion of UCB CD133 + cells. Therefore, these coated scaffolds could mimic the role, structure and environment pattern of ECMs, which might be useful as natural molecules for regenerative medicine and therapeutic purposes.
Acknowledgements
The authors thank Dr. Atashi from Stem Cell and Tissue Engineering Research Center, Shahroud University of Medical Sciences, for his help and suggestions and Bonyakhte Company Headquarter for providing labratory facilities. All authors read and approved the final manuscript. The authors have no conflict of interest in this article.
Disclosure statement
No potential conflict of interest was reported by the authors.
References
- Soufizomorrod M, Soleimani M, Hajifathali A, et al. Expansion of CD133(+) umbilical cord blood derived hematopoietic stem cells on a biocompatible microwells. Int J Hematol-Oncol Stem Cell Res. 2013;7:9–14.
- Mousavi SH, Abroun S, Soleimani M, et al. Expansion of human cord blood hematopoietic stem/progenitor cells in three-dimensional nanoscaffold coated with fibronectin. Int J Hematol-Oncol Stem Cell Res. 2015;9:72–79.
- Eskandari F, Allahverdi A, Nasiri H, et al. Nanofiber expansion of umbilical cord blood hematopoietic stem cells. Iran J Pediatr Hematol Oncol. 2015;5:170–178.
- Aggarwal R, Lu J, Pompili VJ, et al. Hematopoietic stem cells: transcriptional regulation, ex vivo expansion and clinical application. Curr Mol Med. 2012;12:34–49.
- Guillot PV, Cui W, Fisk NM, et al. Stem cell differentiation and expansion for clinical applications of tissue engineering. J Cell Mol Med. 2007;11:935–944.
- Lu J, Aggarwal R, Pompili VJ, et al. A novel technology for hematopoietic stem cell expansion using combination of nanofiber and growth factors. Recent Pat Nanotechnol. 2010;4:125–135.
- Ferreira MS, Jahnen-Dechent W, Labude N, et al. Cord blood-hematopoietic stem cell expansion in 3D fibrin scaffolds with stromal support. Biomaterials. 2012;33:6987–6997.
- Mehta RS, Rezvani K, Olson A, et al. Novel techniques for ex vivo expansion of cord blood: clinical trials. Front Med (Lausanne). 2015;2:89.
- Kelly SS, Sola CB, de Lima M, et al. Ex vivo expansion of cord blood. Bone Marrow Transplant. 2009;44:673–681.
- Wilson A, Trumpp A. Bone-marrow haematopoietic-stem-cell niches. Nat Rev Immunol. 2006;6:93–106.
- Pineault N, Abu-Khader A. Advances in umbilical cord blood stem cell expansion and clinical translation. Experimental Hematology. 2015;43:498–513.
- Ellis SJ, Tanentzapf G. Integrin-mediated adhesion and stem-cell-niche interactions. Cell Tissue Res. 2010;339:121–130.
- Carletti E, Motta A, Migliaresi C. Scaffolds for tissue engineering and 3D cell culture. Methods Mol Biol. 2011;695:17–39.
- Lee ST, Yun JI, Jo YS, et al. Engineering integrin signaling for promoting embryonic stem cell self-renewal in a precisely defined niche. Biomaterials. 2010;31:1219–1226.
- Ma Z, Gao C, Shen J. Surface modification of poly-L-lactic acid (PLLA) membrane by grafting acrylamide: an effective way to improve cytocompatibility for chondrocytes. J Biomater Sci Polym Ed. 2003;14:13–25.
- Lee IC, Young T-H. Preparation of PLLA membranes with different morphologies for culture of ligament cells. Biomed Eng Appl Basis Commun. 2006;18:185–189.
- Connor NS, Aubin JE, Sodek J. Independent expression of type I collagen and fibronectin by normal fibroblast-like cells. J Cell Sci. 1983;63:233–244.
- Mansourizadeh F, Asadi A, Oryan S, et al. PLLA/HA Nano composite scaffolds for stem cell proliferation and differentiation in tissue engineering. Mol Biol Res Commun. 2013;2:1–10.
- Dong C, Lv Y. Application of collagen scaffold in tissue engineering: recent advances and new perspectives. Polymers. 2016;8:42.
- Pineault N, Abu-Khader A. Advances in umbilical cord blood stem cell expansion and clinical translation. Exp Hematol. 2015;43:498–513.
- Ehring B, Biber K, Upton TM, et al. Expansion of HPCs from cord blood in a novel 3D matrix. Cytotherapy. 2003;5:490–499.
- Zeltinger J, Sherwood JK, Graham DA, et al. Effect of pore size and void fraction on cellular adhesion, proliferation, and matrix deposition. Tissue Eng. 2001;7:557–572.
- McCarthy J, Turley EA. Effects of extracellular matrix components on cell locomotion. Crit Rev Oral Biol Med. 1993;4:619–637.
- Skerra A. Engineered protein scaffolds for molecular recognition. J Mol Recognit. 2000;13:167–187.
- Brizzi MF, Tarone G, Defilippi P. Extracellular matrix, integrins, and growth factors as tailors of the stem cell niche. Curr Opin Cell Biol. 2012;24:645–651.
- Celebi B, Mantovani D, Pineault N. Effects of extracellular matrix proteins on the growth of haematopoietic progenitor cells. Biomed Mater. 2011;6:055011.
- Dubey G, Mequanint K. Conjugation of fibronectin onto three-dimensional porous scaffolds for vascular tissue engineering applications. Acta Biomaterialia. 2011;7:1114–1125.
- Heino J, Kapyla J. Cellular receptors of extracellular matrix molecules. Curr Pharm Des. 2009;15:1309–1317.
- Denning-Kendall P, Singha S, Bradley B, et al. Cytokine expansion culture of cord blood CD34+ cells induces marked and sustained changes in adhesion receptor and CXCR4 expressions. Stem Cells. 2003;21:61–70.