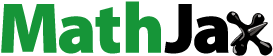
Abstract
Mesoporous silica nanoparticles (MSNs) have been widely used in biomedical applications. However, most studies have been limited to spherical MSNs, while non-spherical MSNs have never been rigorously studied. In this study, we fabricated mesoporous silica nanospheres (MSNSs) and mesoporous silica nanorods (MSNRs), with different aspect ratios (ARs) but identical surface chemistries to explore the shape effects of MSNs on oral delivery. The results of cellular studies demonstrated that MSNRs exhibited a higher cellular uptake than MSNSs. Mechanistic studies showed that caveolae-mediated endocytosis was involved in the uptake of MSNRs, while the clathrin-dependent pathway contributed to the endocytosis of MSNSs. Meanwhile, the apparent permeability coefficient value (Papp) of doxorubicin hydrochloride (Dox)-loaded MSNRs was approximately 1.8-, 3.2- and 6.3-fold higher than that of Dox-loaded MSNS1, Dox-loaded MSNS2 and Dox solution, respectively. The in vivo pharmacokinetics study showed that the area under the plasma concentration–time curve (AUC) achieved by Dox-loaded MSNRs was 1.9-, 3.4- and 5.7-fold higher than the corresponding values for Dox-loaded MSNS1, MSNS2 and Dox solution, respectively. Taken together, our results demonstrated that tuning nanoparticle shape potentially determines the biological fate of nanoparticles with higher delivery efficiency, such as enhanced cellular uptake and oral bioavailability.
Introduction
Mesoporous silica nanoparticles (MSNs), exhibiting attractive properties, such as large surface area [Citation1], easy surface modification [Citation2,Citation3] and multiple morphology [Citation4], have attracted extensive attention for their application in oral drug delivery [Citation5,Citation6]. Recently, numerous studies have synthesized MSNs with various properties [Citation7,Citation8], and they demonstrated that the physical parameters of mesoporous silica materials have played an important role in both in vitro and in vivo biological performance [Citation9–13]. Hu et al. [Citation14] revealed that mesoporous silica microspheres could exhibit an excellent dissolution-enhancing effect for poorly soluble drugs. Zhang et al. [Citation15] proposed that MSNs with particle diameters less than 100 nm have the potential to achieve enhanced oral bioavailability of poorly soluble drugs. Notably, these studies generally focused on the modification of particle size, while there remained limited information in regard to the effect of particle shape on the oral absorption. Hence, it is important to explore favourably shaped MSNs as drug carriers for effective oral delivery.
Inspired by an intriguing phenomenon that most microbial inhabitants in the gastrointestinal (GI) tract are rod-shaped [Citation16], some researches have emerged to explore the effects of nanoparticle shape on oral delivery [Citation6,Citation13,Citation17–19]. For example, Yu et al. [Citation20] reported that rod-shaped nanoparticles displayed faster diffusion than their spherical counterparts in the intestinal mucus, leading to deeper mucus penetration and a longer retention time in the GI tract. Banerjee et al. [Citation21] demonstrated that the intestinal cellular uptake of nanorods was significantly higher than spheres. Therefore, we assumed that the bioavailability of oral administration based on MSN delivery can be improved by rod-shaped nanoparticles.
In this study, we first fabricated MSNs with different aspect ratios (ARs) and sizes (MSNRs, MSNS1 and MSNS2) but with identical surface chemistries and zeta potentials. The Caco-2 cellular uptake results showed that mesoporous silica nanorods (MSNRs) exhibited higher cellular internalization both than the mesoporous silica nanospheres (MSNSs) with a similar size and the MSNs with a smaller size. The cellular uptake mechanisms study revealed that the difference of cellular internalization of differently shaped MSNs may be ascribed to the pathway involved in the uptake of nanoparticles. When loaded with doxorubicin hydrochloride (Dox), a poorly permeable drug, we observed that MSNRs could exhibit significantly enhanced oral bioavailability of Dox compared to MSNSs and Dox solution, which may be due to the higher cellular uptake of MSNRs. These findings help to characterize the effect of MSN-based oral administration and provide new insights into particle geometry for the future design of efficient drug delivery systems.
Materials and methods
Materials
Cetyltrimethylammonium bromide (CTAB) was purchased from Yu Wang Chemical Reagent Corporation (Shandong, China). Tetraethoxysilane (TEOS) and 3-aminopropyltriethoxysilane (APTES) were purchased from Aladdin (Shanghai, China). All other chemicals were of analytical grade as required and were used without further purification. Deionized water was prepared by ion exchange.
Preparation of MSNs
The MSNs were prepared according to the previous method with little modification [Citation20]. For the fabrication of MSNRs, 0.22 g CTAB was dissolved in 54 mL of deionized water. Next, 850 μL NH4OH was added to alkalify the solution. After stirring for 1 h, 470 μL of TEOS was dropped into the solution, and the reaction was kept stirring for 3 h at room temperature. The above sample was centrifuged and washed with ethanol three times, and the particles were resuspended in ethanol. To remove the CTAB, 1 mL HCl was added to stir overnight. The final samples were produced by lyophilization. For MSNS2, briefly, 0.686 g KH2PO4 and 0.116 g NaOH were dissolved in 100 mL deionized water, and then 0.32 g CTAB and 9 mL propanetriol were added. After forming micelles, 1.8 mL TEOS was introduced and the reaction was maintained for 8 h. The subsequent steps were the same as those of MSNR.
Additionally, we applied another type of spherical MSNs (MSNS1) with smaller diameter according to method previously reported [Citation20]. First, NaOH (0.28 g) and CTAB (1 g) were dissolved in 480 mL of deionized water on 80 °C water bath. TEOS (5 mL) was dropped into the solution and then stirred for 2 h. The subsequent steps were the same as those of MSNR.
For the FITC labelling, FITC was covalently linked to APTES in ethanol, and 20 mg of three types of prepared MSNs were added to form the conjugation, respectively. The system was stirred overnight, and the solution was centrifuged and washed with ethanol to remove the unconjugated FITC and APTES until the supernatants were colourless.
Drug loading
Briefly, 25 mg Dox was dissolved in 1 mL absolute ethyl alcohol to obtain a drug solution, and this solution was mixed with 100 mg MSNs under stirring for 24 h. Samples were centrifuged, and the supernatant was removed. The drug loading capacity was measured by subtracting the drug in the supernatant using ultraviolet spectroscopy (UV-1750, Shimadzu, Japan) at the wavelength of 499 nm [Citation22].
Characterization
TEM
The mesoporous structures of MSNs were characterized using a Tecnai G2 20 TEM instrument (FEI, Hillsboro, OR). Before examination, the sample was dispersed in ethanol through sonication and subsequently deposited on carbon-coated copper grids. The grids were air-dried at room temperature for 12 h and observed by TEM with an acceleration voltage of 200 kV.
Size and zeta potential measurement
The particle size and zeta potential of MSNRs, MSNS1 and MSNS2 were determined at 25 °C in distilled water by dynamic light scattering (DLS; Nano ZS, Malvern, UK). The experiments were conducted in triplicate.
Cellular studies
Cell culture and cytotoxicity
Caco-2 cells were maintained in Dulbecco's Modified Eagle's Medium (DMEM; Gibco, Carlsbad, CA), 10% (v/v) foetal bovine serum (FBS; HyClone, Conroe, TX), 1% (v/v) non-essential amino acid, 1% (v/v) l-glutamine and 1% penicillin and streptomycin (100 IU/mL) at 37 °C in 5% CO2. The cytotoxicity of MSNs was evaluated by measuring the cell viability using an MTT (3-(4,5-dimethylthiazol-2-yl)-2,5-diphenyltetrazolium bromide) assay. For the MTT test of MSNs, the cells were seeded into 96-well plates at a density of 2 × 104 cells/well. After 24 h of incubation, the culture medium was removed and different concentrations of cell medium containing MSNs (10, 20, 50, 100, 200, 500 and 1000 μg/mL) were added. After incubating for 24 h, 20 μL MTT solution (5 mg/mL) was added into each well, and the plates were incubated for another 4 h to quantify the living cells. Afterwards, the DMEM was removed, and DMSO (150 μL) was added to dissolve the MTT formazan crystals. Finally, the 96-well plates were placed in a Thermo Fisher Microplate Reader (Waltham, MA) to measure the absorbance of formazan product at 490 nm.
Cellular uptake
For the quantitative cellular uptake study performed using flow cytometry (FACS) analysis, the cells were seeded into 12-well culture plates at a density of 1 × 105 cells/cm2. After 2 h of incubation with MSNs, the cells were washed three times with PBS and trypsinized for 2 min. Trypsinization was terminated by adding cold culture medium. The cells were extracted gently by pipetting. After centrifugation, the treated samples were transferred to FACS tubes. The cells were analysed by FACS using BD FACS Calibur fluorescent-activated flow cytometry and BD CellQuest software (San Jose, CA). The experiment was performed in triplicate, and a total of 10,000 cells per sample were analysed.
To study the cellular uptake of MSNs qualitatively, Caco-2 cells were seeded into 12-well plates and allowed to attach in growth medium at 37 °C in a 5% CO2 incubator for 24 h. Next, the medium was removed and replaced with fresh medium containing FITC-labelled MSNs (10 μg/mL). After an incubation of 2 h, the cells in each well were washed with PBS three times and fixed with 4% formaldehyde, and the nuclei were later stained by DAPI (4,6-diamidino-2-phenylindole) for 10 min. Subsequently, cells were washed twice with PBS and observed by a confocal laser scanning microscope (FluoView FV1000, Olympus, Japan).
To investigate the effect of the MSNs on drug delivery ability, the cellular uptake efficiency of the Dox loaded in MSNs was compared. The Caco-2 cells were seeded into a density of 1 × 105 cells/mL on a polycarbonate membrane (0.4 μm in pore size) in Costar Transwell 12 wells/plate (Corning Costar Corp., Corning, NY). The integrity of cell monolayer was monitored by an electrical resistance metre (Millicell ERS-2, Millipore, Billerica, MA) to ensure the transepithelial electrical resistance (TEER) values were 400–600 Ω cm2. After incubation with test samples (Dox solution, suspension of MSNRs-Dox, MSNS1-Dox and MSNS2-Dox with the equivalent drug concentration of 2 μg/mL) for 4 h at 37 °C, cell monolayers were washed three times with PBS, polycarbonate membranes with test cell monolayers were removed to glass slides. Later, cells were fixed with 4% paraformaldehyde solution, stained with DAPI, and examined via CLSM.
Cellular uptake mechanisms
To study the behaviour of the MSNs as they were taken up by cells, endocytotic mechanisms of MSNs were performed in the presence of specific inhibitors. Caco-2 cells were first incubated in PBS with specific inhibitors for 1 h at 37 °C and subsequently exposed to the MSNs for 2 h at 37 °C. A control group was performed in PBS without inhibitors for MSNs. Methyl-β-cyclodextrin 10 mmol/L in the presence of lovastatin (1 μg/mL) was used to deplete cholesterol [Citation23], which had been demonstrated to inhibit caveolae-independent and clathrin-independent endocytosis. Chlorpromazine 10 μg/mL was used to inhibit clathrin-mediated endocytosis [Citation24]. The inhibition of caveolae-dependent endocytosis was achieved by incubation of cells with 10 μg/mL nystatin.
Cellular transport
Cell monolayers were incubated with PBS on a polycarbonate membrane located between the donor and receptor chambers. The receptor chamber was filled with PBS, and the donor chambers was replaced with test solutions. At predetermined time intervals, receptor chambers were sampled and an equivalent amount of fresh PBS was replaced. The concentration of Dox was measured using a microplate reader by transferring the withdrawn samples into a 96-well plate [Citation25].
The apparent permeability coefficient (Papp) was calculated using the following equation:
where dQ/dt is the flux rate of Dox from the donor chamber to the receptor chamber, C0 is the initial concentration of Dox in the donor chambers and A is the membrane area (cm2).
Drug absorption in rat small intestine
Animal experiments were performed in accordance with the Guidelines for Animal Experimentation of Shenyang Pharmaceutical University (Shenyang, China) and the protocol was approved by the Animal Ethics Committee of the institute. Prior to the experiment, rats were fasted overnight with free access to water. To investigate the intestinal absorption of Dox, male Sprague–Dawley rats were anaesthetized with urethane solution, Dox formulations (MSNRs-Dox, MSNS1-Dox, MSNS2-Dox and Dox solution, 5 mg/kg) were orally administered using an oral feeding needle, respectively. Two hours later, rats were sacrificed, and the sections of the middle small intestine were excised [Citation26]. The intestinal tissues were washed with PBS, immediately frozen in OCT, and sliced at a depth of 20 μm using a cryostat (LEICA, Wetzlar, Germany). The tissue slices were subsequently fixed with 4% paraformaldehyde solution, stained with DAPI, and examined via CLSM.
In vivo pharmacokinetic study
The Male Sprague–Dawley rats (200 ± 20 g) were randomly divided into four groups (n = 3 for each studied group). All rats were fasted overnight prior to dosing with free access to water. The four groups were orally administered Dox solution and the water suspension of MSNRs-Dox, MSNS1-Dox and MSNS2-Dox at a dose of 10 mg/kg, respectively. An approximately 0.5 mL aliquot of blood sample was collected via the retro-orbital venous sinus at specified time intervals of 0.25, 0.5, 1, 2, 4, 6, 8, 12 and 24 h in microcentrifuge tubes containing heparin. The blood samples were immediately centrifuged for 10 min at 5000×g to collect the plasma, and the plasma samples were modified for the HPLC analysis of Dox.
Plasma samples were processed as follows: a 100 μL plasma sample was mixed with 5 μL of an internal standard (daunomycin) solution (5 μg/mL) and 400 μL acetonitrile and vortexed for 2 min. After centrifugation at 13,000 rpm for 10 min, the top organic layer was extracted and then evaporated under a gentle stream of nitrogen gas at room temperature. The residue was reconstituted in 100 μL mobile phase. After vortex mixing and centrifugation at 8000 rpm for 10 min, a 20 μL aliquot was subjected to HPLC analysis.
Statistical analysis
Statistical analysis was carried out with Student’s t-test using SPSS software (Chicago, IL), with p < .05 being considered to be statistically significant differences and p < .01 being considered to be very statistically significant differences.
Results
Synthesis and characterizations of MSNs
MSNs with different shapes were synthesized. As shown in the TEM images (), it was obvious that the MSNRs showed a rod-like structure (AR = 3), whereas the MSNSs (, MSNS1 and MSNS2) presented as spherical (AR = 1). The hydrodynamic diameters of the MSNs were approximately 90, 190 and 200 nm for MSNS1, MSNS2 and MSNRs, respectively (). We note that the hydrodynamic diameter of MSNS1 was approximately half of that of MSNS2 and MSNRs. The average ζ-potentials of the three types of NPs were approximately –10 mV (), which ensured the same surface charge on all of these samples.
Cellular studies
Cytotoxicity and cellular uptake of MSNs
As shown in , the cell viability of Caco-2 incubated with MSNs for 24 h remained above 80%, indicating the influence of MSNs on cell viability could be neglected at the tested concentrations (10, 20, 50, 100, 200, 500 and 1000 μg/mL). The results illustrated that both MSNSs and MSNRs produced no significant cytotoxicity, and they could be applied as safe drug delivery systems.
Figure 2. (a) Viability of Caco-2 cells after incubation with MSNRs or MSNSs for 24 h. (b) The MFI of FITC labelled MSNRs and MSNSs was measured by FACS in Caco-2 cells after treatment for 0.5, 1 and 2 h, respectively. (c) Confocal microscopy images of Caco-2 cells after treatment with FITC labelled MSNRs and MSNSs for 2 h. Scale bar represents 50 μm. (d) Cellular uptake mechanism of MSNRs and MSNSs. The data are presented as the mean ± standard error of the mean (n = 3). *p < .05 compared to control group; **p < .01 compared to control group.
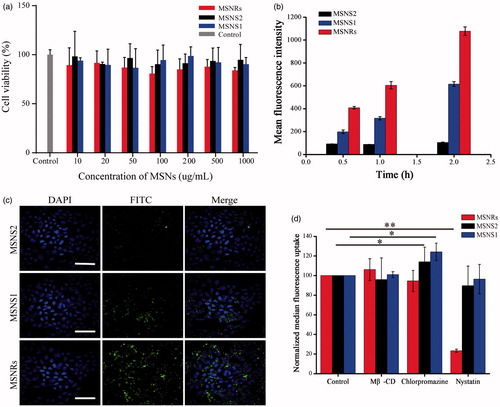
To study quantitatively the cellular uptake of nanoparticles by Caco-2 cells, the FACS method was adopted. As it can be seen in , the cellular uptake of MSNRs in Caco-2 cells occurred in a time-dependent manner, and prolonged time is beneficial for the internalization of MSNRs. However, there was slight improvement in mean fluorescence intensity (MFI) of MSNS2 and MSNS1 as the treatment time increased. MSNS1 displayed better cellular internalization, demonstrating that decreasing the size of NPs could improve the cellular uptake. The MFI of MSNSs was persistently dramatically weaker than that of MSNRs at different treatment time points. Next, we observed the cellular uptake of MSNs by confocal laser scanning microscopy. shows that the fluorescence intensity originating from FITC-labelled MSNRs was significantly higher than that of MSNS1 and MSNS2. These results demonstrated that MSNRs with rod-like shape possessed better cellular uptake performance than MSNSs, and the effect of shape to NPs on cellular uptake seemed to be more important than that of particle size.
We further explored the specific mechanisms involved in the cellular internalization of differently shaped nanoparticles. As shown in , the cellular internalization of MSNRs was significantly influenced by nystatin with a reduction in cellular uptake of approximately 70%, while the effects of methyl-β-cyclodextrin/lovastatin and chlorpromazine on the cellular internalization were negligible. This finding indicated that caveolae-mediated endocytosis was involved in the uptake of MSNRs by Caco-2 cells. For MSNS1 and MSNS2, pre-incubation with chlorpromazine resulted in a distinct increase of the cellular uptake (approximately 20%), while the other inhibitors showed no significant effects on the cellular uptake of MSNSs, indicating that clathrin-mediated endocytosis was involved in the cellular uptake of MSNSs [Citation27]. To the best of our knowledge, nanoparticles internalized via clathrin-mediated endocytosis will be transformed to endosomes, in which a fraction of internalized particles could be recycled back to the cell exterior. However, caveolae-mediated endocytosis transports nanoparticles either through the endosomal pathway, in which particles suffer the same fate as ones internalized via clathrin-mediated endocytosis, or through caveosome pathway, in which a portion of particles could be translocated to the endoplasmic reticulum/Golgi body. Therefore, compared with clathrin-mediated endocytosis, caveolae-mediated endocytosis involved in the uptake of MSNRs may perform better at internalizing nanoparticles [Citation28,Citation29].
Cellular uptake of Dox
Based on the results of cellular uptake studies, we further investigated the effect of particle shape on drug delivery for poorly permeable drugs. The three-dimensional fluorescent images of Caco-2 cell monolayer treated with Dox formulations were obtained as depicted in . Dox-loaded MSNRs exhibited the most enhancement in cellular uptake for Dox compared to MSNSs and Dox solution. In contrast, the fluorescence of Dox loaded by MSNSs were seldom observed in deep layers along the z-direction, indicating the weak cellular internalization of MSNSs. In addition, clearly, very little Dox could be observed internalizing the cell monolayer in group Dox solution. The results of 2D visualization confirmed the best Dox uptake efficiency of Dox-loaded MSNRs (), and it was worth noting that MSNRs could facilitate the delivery of loaded Dox to nuclei; therefore, Dox could perform better to treat malignancies by disturbing DNA double helix [Citation22,Citation30].
Cellular transport of Dox
The Papp and the permeation enhancement ratio of four types of Dox formulation are displayed in . The results showed that MSNRs exhibited the best enhanced permeation of Dox compared with MSNSs. The Papp of Dox-loaded MSNRs, Dox-loaded MSNS1 and Dox-loaded MSNS2 was almost 6.3-, 3.5- and 2.0-fold higher than that of Dox solution, respectively, which was consistent with the result of the cellular uptake of Dox loaded in mesoporous silica carriers.
Table 1. Permeability value for MSNRs-Dox, MSNS1-Dox, MSNS2-Dox and Dox solution transported through Caco-2 cells.
Drug absorption in the intestine
The absorption of Dox in the intestine was investigated in male SD rats. demonstrates that only slight Dox fluorescence could be observed crossing the epithelium via the enterocytes after treatment with Dox solution. The Dox loaded in MSNS1 and MSNS2 were increased across the epithelial barrier compared to Dox solution. However, the Dox fluorescence from Dox-loaded MSNRs presented the highest among the four groups indicating the promising efficiency of MSNRs in facilitating the intestinal absorption of Dox. Additionally, a single intestinal villus from each sample at a higher magnification was carefully examined. Obviously, the Dox was delivered efficiently into the intestinal tissue by MSNRs, while MSNS1 and MSNS2 performed worse in the regions of interest. These results demonstrated that MSNRs exhibited better intestinal Dox delivery than that of MSNSs, which were in good agreement with the findings of our in vitro studies.
In vivo pharmacokinetic study
To evaluate the application of MSNRs capable of enhancing drug absorption in vivo, a pharmacokinetics study was performed. The mean plasma concentration time profiles of different Dox formulations after oral administration are shown in . The pharmacokinetic parameters are reported in . It is worth noting that MSNRs-Dox achieved the highest Cmax, which was approximately 5.8-, 2.9- and 1.6-fold higher than Dox solution, MSNS2-Dox and MSNS1-Dox, respectively. Similarly, the area under the plasma concentration–time curve (AUC) for Dox-MSNRs was approximately 5.7-, 3.4- and 1.9-fold higher than that of Dox solution, MSNS2-Dox and MSNS1-Dox, respectively. Therefore, the significantly enhanced bioavailability of Dox ensured the potential of MSNRs as the next-generation oral drug delivery system for poorly absorbed drugs.
Figure 5. Plasma concentration vs time profile of different Dox formulations after oral administration (n = 3).
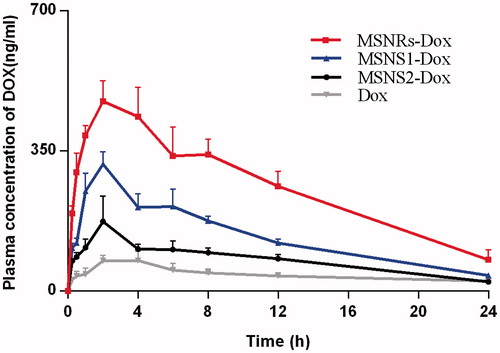
Table 2. Pharmacokinetic parameters of Dox after oral administration of different Dox formulations at a dose of 10 mg/kg to rats (n = 3).
Discussion
Oral administration is the most convenient and extensively used route for drug delivery. Over the past several decades, MSNs have shown enhanced efficacy for the oral delivery of insoluble therapeutics and biologics. The size, surface charge and material compositions of MSNs can greatly impact the cellular uptake and efficacy of MSN-based oral delivery [Citation21]. However, the effect of MSN shape is less thoroughly understood, especially for oral drug delivery. To this end, we fabricated rod- and sphere-shaped MSNs to study the influence of shape on oral delivery, especially on cellular uptake and bioavailability.
In this study, we studied the effect of particle shape on the internalization of Caco-2 cells, a typical type of epithelium cells. Interestingly, we observed that the efficiency and mechanisms of cellular uptake are both shape-dependent, and MSNRs performed better in efficiency of cellular uptake compared with MSNSs. Specifically, caveolae-mediated endocytosis was involved in the uptake of MSNRs, while the endocytosis of MSNSs was clathrin-dependent, which was consistent with the conclusions reported previously [Citation17]. We assumed that the different mechanisms involved in the cellular internalization may contribute to the uptake results of MSNs with different shapes. These results offer crucial insights on the effect of particle shape on cell uptake and provide a new perspective on designing nanoparticles as drug delivery systems.
MSNs hold the potential to improve the oral bioavailability of poorly permeable drugs. When pharmacokinetic studies were conducted in male SD rats, both MSNRs and MSNSs exhibited significantly enhanced Dox plasma concentrations over that of Dox solution. Interestingly, the Cmax and AUC of MSNRs were considerably higher than those of MSNSs, indicating a greater bioavailability enhancement of MSNRs. Collectively, we attributed this phenomenon to the improved epithelial uptake of MSNRs compared with MSNSs.
Conclusions
MSNs with different shapes were facilely prepared. Based on the fundamental point that the shape is the only variable, it is worth noting that MSNRs exhibited excellent cellular uptake and provide Dox with the highest oral bioavailability. Our work demonstrates that particle shape is an important parameter that should be carefully investigated in order to develop highly effective MSNs as drug delivery systems.
Disclosure statement
The authors report no conflicts of interest.
Additional information
Funding
References
- Zhu M, Wang H, Liu J, et al. A mesoporous silica nanoparticulate/β-TCP/BG composite drug delivery system for osteoarticular tuberculosis therapy. Biomaterials. 2011;32:1986–1995.
- Li X, Xing L, Zheng K, et al. Formation of gold nanostar-coated hollow mesoporous silica for tumor multimodality imaging and photothermal therapy. ACS Appl Mater Interfaces. 2017;9:5817–5827.
- Ahmadi NN, Hassani Kumleh H, Beygzadeh M, et al. Delivery of curcumin by a pH-responsive chitosan mesoporous silica nanoparticles for cancer treatment. Artif Cells Nanomed Biotechnol. 2017;23:1–7.
- Wang Y, Zhao QF, Hu YC, et al. Ordered nanoporous silica as carriers for improved delivery of water insoluble drugs: a comparative study between three dimensional and two dimensional macroporous silica. Int J Nanomed. 2013;8:4015–4031.
- Hartono SB, Hadisoewignyo L, Yang YN, et al. Amine functionalized cubic mesoporous silica nanoparticles as an oral delivery system for curcumin bioavailability enhancement. Nanotechnology. 2016;27:505605.
- Biswas N. Modified mesoporous silica nanoparticles for enhancing oral bioavailability and antihypertensive activity of poorly water soluble valsartan. Eur J Pharm Sci. 2017;99:152–160.
- Du X, He JH. Elaborate control over the morphology and structure of mercapto-functionalized mesoporous silicas as multipurpose carriers. Dalton Trans. 2010;39:9063–9072.
- Yildirim A, Bayindir M. A porosity difference based selective dissolution strategy to prepare shape-tailored hollow mesoporous silica nanoparticles. J Mater Chem A. 2015;3:3839–3846.
- Slowing II, Trewyn BG, Giri S, et al. Mesoporous silica nanoparticles for drug delivery and biosensing applications. Adv Funct Mater. 2007;17:1225–1236.
- Giri S, Trewyn BG, Lin VSY. Mesoporous silica nanomaterial-based biotechnological and biomedical delivery systems. Nanomedicine-UK. 2007;2:99–111.
- He QJ, Zhang JM, Shi JL, et al. The effect of PEGylation of mesoporous silica nanoparticles on nonspecific binding of serum proteins and cellular responses. Biomaterials. 2010;31:1085–1092.
- McCarthy CA, Faisal W, O'Shea JP, et al. In vitro dissolution models for the prediction of in vivo performance of an oral mesoporous silica formulation. J Control Release. 2017;250:86–95.
- Hao N, Li L, Tang F. Roles of particle size, shape and surface chemistry of mesoporous silica nanomaterials on biological systems. Int Mater Rev. 2017;62:57–77.
- Hu YC, Zhi ZZ, Zhao QF, et al. 3D cubic mesoporous silica microsphere as a carrier for poorly soluble drug carvedilol. Micropor Mesopor Mater. 2012;147:94–101.
- Zhang YZ, Wang JC, Bai XY, et al. Mesoporous silica nanoparticles for increasing the oral bioavailability and permeation of poorly water soluble drugs. Mol Pharm. 2012;9:505–513.
- Backhed F, Ley RE, Sonnenburg JL, et al. Host-bacterial mutualism in the human intestine. Science. 2005;307:1915–1920.
- Agarwal R, Singh V, Jurney P, et al. Mammalian cells preferentially internalize hydrogel nanodiscs over nanorods and use shape-specific uptake mechanisms. Proc Natl Acad Sci USA. 2013;110:17247–17252.
- Li LL, Liu TL, Fu CH, et al. Biodistribution, excretion, and toxicity of mesoporous silica nanoparticles after oral administration depend on their shape. Nanomed-Nanotechnol. 2015;11:1915–1924.
- Dias DR, Moreira AF, Correia IJ. The effect of the shape of gold core-mesoporous silica shell nanoparticles on the cellular behavior and tumor spheroid penetration. J Mater Chem B. 2016;4:7630–7640.
- Yu MR, Wang JL, Yang YW, et al. Rotation-facilitated rapid transport of nanorods in mucosal tissues. Nano Lett. 2016;16:7176–7182.
- Banerjee A, Qi JP, Gogoi R, et al. Role of nanoparticle size, shape and surface chemistry in oral drug delivery. J Control Release. 2016;238:176–185.
- Li J, Du XT, Zheng N, et al. Contribution of carboxyl modified chiral mesoporous silica nanoparticles in delivering doxorubicin hydrochloride in vitro: pH-response controlled release, enhanced drug cellular uptake and cytotoxicity. Colloid Surf B. 2016;141:374–381.
- Garcion E, Lamprecht A, Heurtault B, et al. A new generation of anticancer, drug-loaded, colloidal vectors reverses multidrug resistance in glioma and reduces tumor progression in rats. Mol Cancer Ther. 2006;5:1710–1722.
- Makhlof A, Fujimoto S, Tozuka Y, et al. In vitro and in vivo evaluation of WGA-carbopol modified liposomes as carriers for oral peptide delivery. Eur J Pharm Biopharm. 2011;77:216–224.
- Zhu QL, Song WY, Xia DN, et al. Poly-l-glutamic acid functionalized nanocomplex for improved oral drug absorption. J Mater Chem B. 2015;3:8508–8517.
- Maisel K, Ensign L, Reddy M, et al. Effect of surface chemistry on nanoparticle interaction with gastrointestinal mucus and distribution in the gastrointestinal tract following oral and rectal administration in the mouse. J Control Release. 2015;197:48–57.
- Li X, Chen D, LeC, et al. Novel mucus-penetrating liposomes as a potential oral drug delivery system: preparation, in vitro characterization, and enhanced cellular uptake. Int J Nanomed. 2011;6:3151–3162.
- Doherty GJ, McMahon HT. Mechanisms of endocytosis. Annu Rev Biochem. 2009;78:857–902.
- Hao NJ, Li LL, Zhang Q, et al. The shape effect of PEGylated mesoporous silica nanoparticles on cellular uptake pathway in HeLa cells. Micropor Mesopor Mater. 2012;162:14–23.
- Han N, Zhao QF, Wan L, et al. Hybrid lipid-capped mesoporous silica for stimuli-responsive drug release and overcoming multidrug resistance. ACS Appl Mater Interfaces. 2015;7:3342–3351.